- School of Biotechnology and Health Sciences, Wuyi University, Jiangmen, China
A metal-free catalytic approach for the remote C6-functionalization of 2,3-disubstituted indoles has been developed. In the presence of catalytic amounts of Brønsted acid, the β,γ-unsaturated α-ketoesters react with 2,3-disubstituted indoles at the C6 position selectively. Under mild reaction conditions, a range of C6-functionalized indoles were prepared with good yields and excellent regioselectivity. This methodology provides a concise and efficient route for the synthesis of C6-functionalized indole derivatives.
Introduction
Indole and its homologues and derivatives widely exist in nature, mainly in natural flower oils, such as jasmine, bitter orange flower, daffodil, vanilla, etc. This structure also ubiquitously exists as key structural framework in numerous natural products, functional materials, and medicines (Ishikura et al., 2015; Sherer and Snape, 2015; Zhang et al., 2015; Sravanthi and Manju, 2016; Gao et al., 2020) (Scheme 1A). For example, tryptophan is an essential amino acid of animals. Trikentrins, which have a fused cyclopenta[g]indole structures, were isolated from sponges and showed antimicrobial activity and cytotoxicity against KB cells (Capon et al., 1986; Herb et al., 1990). ABT-299 is a prodrug that is highly potent and specific platelet activating factor (PAF) antagonist (Vaden et al., 1996). Nintedanib is an oral tyrosine kinase inhibitor approved for the treatment of idiopathic pulmonary fibrosis (Wind et al., 2019).
Due to their important biological activities, the synthesis of functionalized indoles has received continuous attention. Although traditional methods for the indole synthesis can lead to indoles with different functionalizations (Fischer and Jourdan, 1883; Sugasawa et al., 1979; Gassman et al., 1984; Street et al., 1993; Fukukyama et al., 1994; Furstner and Ernst, 1995) these methods require multi-step substrate synthesis and the introduction of functional groups in many cases are less efficient. An alternative approach to access functionalized indoles is the direct C-H functionalization of indoles, (Wang et al., 2016; Fan et al., 2017; Gandeepan et al., 2019; Rej et al., 2020), which allows for the rapid and efficient introduction of specific groups into the indole backbone, thus providing a most straightforward and atom-economical access to the target indoles. Numerous efforts have been made in recent years to achieve selective functionalization of indoles. One of the major challenges of this area is the controlling of the site-selectivity of indole (Cacchi and Fabrizi, 2005; Humphrey and Kuethe, 2006; Sandtorv, 2015; Yang and Shi, 2018). Due to its inherent nucleophilic characteristics, the reaction of indoles usually take place at their very reactive N1, C3 and C2 positions. (Scheme 1B) various methods have been developed for the C3, C2, and N1-functionalization of indoles via organo- and transition-metal-catalysis.
Comparatively, the functionalization at the C4−C7 position of indoles has been less reported, most of them were based on transition metal catalysis using directing groups to achieve C-H bond activation (Poulsen et al., 2015; Rostoll-Berenguer et al., 2018; Xiao et al., 2018; Huang et al., 2019; Wen and Shi, 2021). Among them, the C6 position of indole is far away from the possible directing group, which makes the C6 functionalization of indole even more difficult (Liu et al., 2014; Zhou et al., 2014; Wu et al., 2019; Ling et al., 2019; Yan et al., 2020). In 2014, Yu and co-workers developed a removable “U-shaped” template to enable the orientation of indole C6-H to carry out alkenylation reactions (Yang et al., 2014) (Scheme 2A). In 2016, Larrosa developed a new strategy for indole C7 carboxyl group as a guiding group, causing indole C6 to undergo arylation (Scheme 2B). Simonetti et al. (2017) Frost’s group used C3 ester groups with auxiliary coordination orientation and strong coordination orientation of pyrimidine groups on N atoms as the reaction substrate, and achieved selective C-H bond alkylation of indole C6 in Ru catalysis (Scheme 2C). (Leitch et al. (2017) Recently, the groups of Zhang (Zhou et al., 2019) (Scheme 2D) and Zhou (Huang et al., 2021) (Scheme 2E) have independently developed C6-enantioselective C−H functionalization of 2,3-disubstituted indoles via chiral phosphoric acid catalysis. However, the development of efficient methods for the facile access to metal-free and highly selective C6 functionalization of indole in a sustainable fashion under mild conditions is still highly desirable. Herein, we developed a Brønsted acid catalyzed remote C6 functionalization of 2,3-disubstituted indoles with β,γ-unsaturated α-ketoester (Scheme 2F).
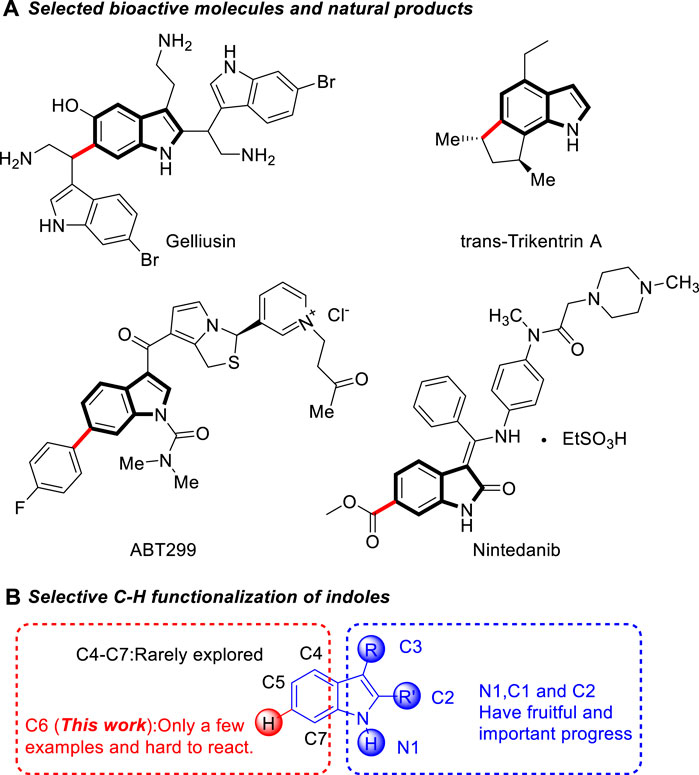
SCHEME 1. Selected bioactive molecules and natural products containing indole skeleton and selective C-H functionalization of indoles.
Initially, we examined this indole C6 functionalization reaction using β,γ-unsaturated α-ketoester 1a and 2,3-dimethyl-1H-indole 2a as model substrates. To our delight, when a mixture of 1a and 2a in toluene were treated with benzenesulfonic acid (BSA) at 30°C, the expected reaction proceeds smoothly and produced the desired C6 functionalized product 3aa in 42% yield (Table 1, entry 1). Inspired by this exciting result, we studied different reaction parameters for this reaction. Firstly, we screened the acid catalysts, including both Brønsted acids and Lewis acids (Table 1, entries 2–7). When p-toluenesulfonic acid (PTS) was used as the catalyst, the product 3aa could be obtained in a yield of 54% (Table 1, entry 2). Subsequently, different solvents were examined. DCM and DCE provided the desired product 3aa in moderate yields (Table 1, entries ht and 9). Other solvents such as THF and DMSO were ineffective for this reaction and only trace amounts of product were detected (Table 1, entries 10 and 11). MeCN was found to be the optimal solvent and the product 3aa was obtained in 85% yield (Table 1, entry 12). Then, the influence of the reaction temperature to this transformation was investigated. When the reaction was performed at 0°C, the yield of 3aa was decreased to 69% (Table1, entry 14). However, higher temperature also led to decreased yields (Table 1, entries 15 and 16). Finally, the ratio of the starting materials was screened (Table 1, entries 16–20). When 1.5 equivalent of 2,3-dimethyl indole was used, the desired product was obtained in an excellent yield of 92% (Table 1, entry 18).
With the optimal reaction conditions in hand, we turned our attention to examining the generality of that reaction. Firstly, as shown in Scheme 3, under optimal reaction conditions, various substituted β,γ-unsaturated α-ketoesters reacted with 2,3-dimethyl indole 2a and produced the corresponding products in good to excellent yields. Both electron-donating and electron-withdrawing group substituted β,γ-unsaturated α-ketoesters were tolerated in this reaction. The electronic properties of the substituents affect the efficiency of this reaction. Generally, β,γ-unsaturated α-ketoesters with electron-donating groups (3ba-3ea) gave higher yields than that with electron-withdrawing groups (3fa-3ka). The steric effect of the substituents has little influence to the yield of this reaction (3ba vs. 3da, 3ha vs. 3ja). Notably, halides including fluoro-, chloro- and bromo-groups were compatible in this reaction. Furthermore, heteroarenes such as 2-naphthyl (3la) and thienyl (3ma) substituted enoneates were also compatible in this reaction and produced the corresponding products in 83% and 98% yields, respectively. In addition, the reaction also gave good yields when ethyl and isopropyl esters were used (3na, 3oa).
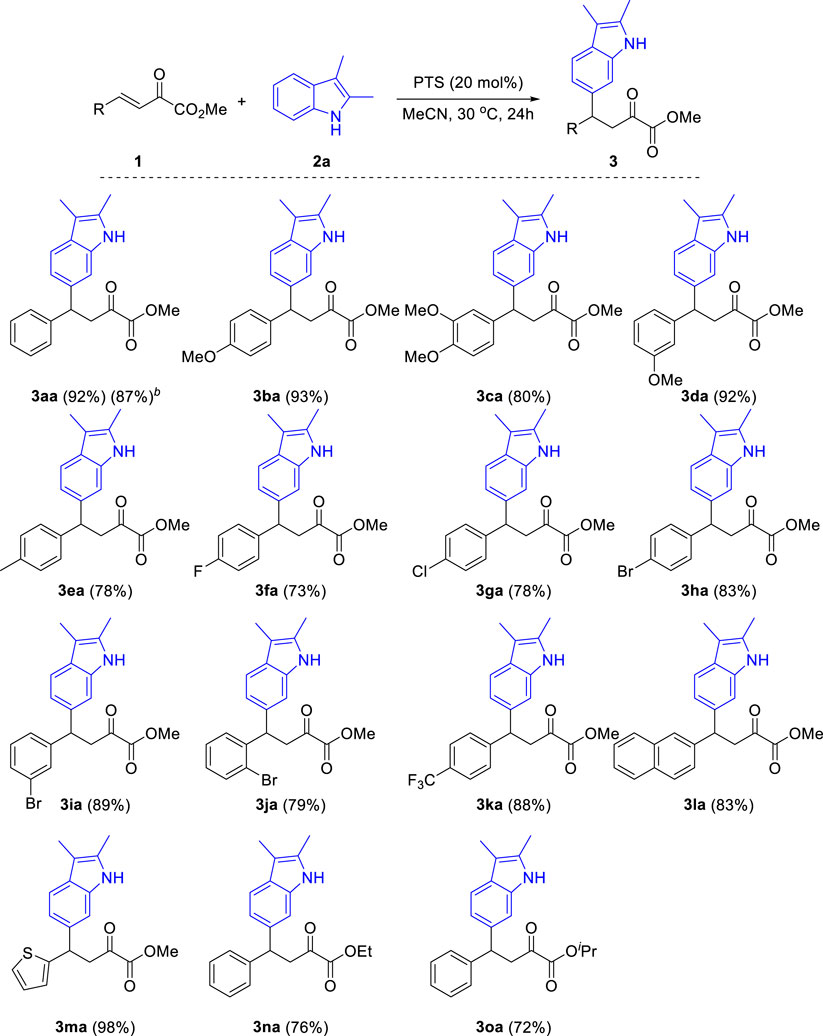
SCHEME 3. Substrate scope of β,γ-unsaturated α-ketoesters. aReaction conditions: β,γ-unsaturated α-ketoester 1 (0.2 mmol), Indoles 2a (0.3 mmol), PTS (20 mol%), 30°C, 24 h, isolated yields. b1a (3 mmol), Indoles 2a (4.5 mmol).
Then, we examined the scope of indoles for this reaction. As shown in Scheme 4, a range of 2,3-disubstituted indoles were successfully applied in this reaction, providing the corresponding C6 functionalized products in moderate to good yields. When tetrahydro-1H-carbazole was subjected to the optimized conditions, the desired product 3 ab was obtained in 71% yield. Other analogues with six-, seven- and five-membered fused rings were also tolerated in this reaction (3ac-3ae). Besides, several functional groups including halides (3ae-3ag), ester (3ah) and ketone (3ai) were compatible in this reaction. It should be noted that the substitution at both C2 and C3 positions of indole were essential for this reaction. When 2-methyl-1H-indole was used in this reaction, a mixture of isomers were obtained, while 3-methyl-1H-indole led to the formation of 9H-pyrrolo[1,2-a]indole (Sun et al., 2016). Other heterocycles such as benzofuran, benzothiophene, carbazole and quinoline were failed in this reaction.
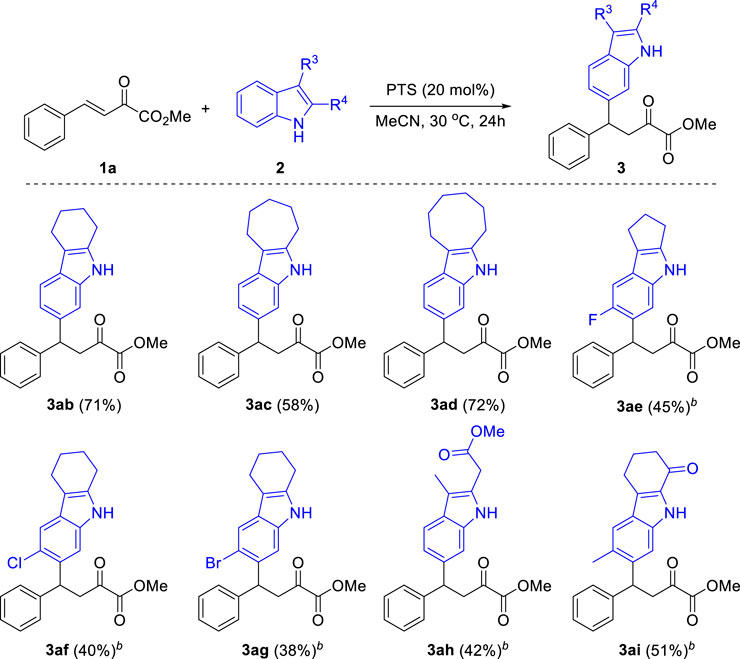
SCHEME 4. Substrate Scope of Indoles. aReaction conditions: β,γ-unsaturated α-ketoester 1a (0.2 mmol), Indoles 2 (0.3 mmol), PTS (20 mol%), 30°C, 24 h, isolated yields. b60°C.
On the basis of these results and the previous literatures, we proposed a plausible reaction model to illustrate the regioselectivity of this reaction. The Brønsted acid served as a bifunctional catalyst to activate both the indole and the β,γ-unsaturated α-ketoesters (Scheme 5).
In summary, we have developed a metal-free catalytic approach for the remote C6-functionalization of 2,3-disubstituted indoles. In the presence of catalytic amounts of Brønsted acid, the β,γ-unsaturated α-ketoesters react with 2,3-disubstituted indoles at the C6 position selectively. Under mild reaction conditions, a range of C6-functionalized indoles were prepared with good yields and excellent regioselectivity. This methodology provides a concise and efficient route for the synthesis of C6-functionalized indole derivatives.
Data availability statement
The original contributions presented in the study are included in the article/Supplementary Materials, further inquiries can be directed to the corresponding author.
Author contributions
J-BP conceived and directed the project. YY-Z performed the experiments. LL and X-ZZ participated in substrates synthesis and discussions. Y-YZ and J-BP wrote the manuscript and ESI.
Funding
Financial supports from the NSFC (21801225), the Wuyi University (2019td02, 2018TP018), the Guangdong Province Universities and Colleges Pearl River Scholar Funded Scheme (2020AL015), and the Department of Education of Guangdong Province (2020KCXTD036) are gratefully acknowledged.
Conflict of interest
The authors declare that the research was conducted in the absence of any commercial or financial relationships that could be construed as a potential conflict of interest.
Publisher’s note
All claims expressed in this article are solely those of the authors and do not necessarily represent those of their affiliated organizations, or those of the publisher, the editors and the reviewers. Any product that may be evaluated in this article, or claim that may be made by its manufacturer, is not guaranteed or endorsed by the publisher.
Supplementary material
The Supplementary Material for this article can be found online at: https://www.frontiersin.org/articles/10.3389/fchem.2022.992398/full#supplementary-material
References
Cacchi, S., and Fabrizi, G. (2005). Synthesis and functionalization of indoles through palladium-catalyzed reactions. Chem. Rev. 105, 2873–2920. doi:10.1021/cr040639b
Capon, R. J., Macleod, J. K., and Scammells, P. J. (1986). The trikentrins : Novel indoles from the sponge. Tetrahedron 42, 6545–6550. doi:10.1016/s0040-4020(01)88117-5
Fan, Z., Lu, H., Li, W., Geng, K., and Zhang, A. (2017). Rhodium-catalyzed redox-neutral coupling of phenidones with alkynes. Org. Biomol. Chem. 15, 5701–5708. doi:10.1039/c7ob01271c
Fischer, E., and Jourdan, F. (1883). Ueber die Hydrazine der Brenztraubensäure. Ber. Dtsch. Chem. Ges. 16, 2241–2245. doi:10.1002/cber.188301602141
Fukukyama, T., Chen, X., and Peng, G. (1994). A novel tin-mediated indole synthesis. J. Am. Chem. Soc. 116, 3127–3128. doi:10.1021/ja00086a054
Furstner, A., and Ernst, A. (1995). Syntheses of camalexin, indolopyridocoline and flavopereirine. Tetrahedron 51, 773–786. doi:10.1016/0040-4020(94)00987-6
Gandeepan, P., Müller, T., Zell, D., Cera, G., Warratz, S., and Ackermann, L. (2019). 3d transition metals for C–H activation. Chem. Rev. 119 (4), 2192–2452. doi:10.1021/acs.chemrev.8b00507
Gao, Y., Wang, X. N., Wei, Z. L., Cao, J. G., Liang, D. P., Lin, Y. J., et al. (2020). Asymmetric synthesis of spirooxindole-pyranoindole products via Friedel–Crafts alkylation/cyclization of the indole carbocyclic ring. New J. Chem. 44, 9788–9792. doi:10.1039/d0nj00074d
Gassman, P. G., Roos, J. J., and Lee, S. J. (1984). Use of [2, 3]-sigmatropic rearrangements in a one-step conversion of tetrahydroquinoline to substituted 1, 2, 5, 6-tetrahydro-4N-pyrrolo[3, 2, 1-ij]quinolin-2-one and 5, 6-dihydro-4H-pyrrolo[3, 2, 1-ij]quinoline. J. Org. Chem. 49 (4), 717–718. doi:10.1021/jo00178a033
Herb, R., Carroll, A. R., Yoshida, W. Y., Scheuer, P. J., and Paul, V. J. (1990). Polyalkylated cyclopentindoles: Cytotoxic fish antifeedants from a sponge, Axinella sp. Tetrahedron 46, 3089–3092. doi:10.1016/s0040-4020(01)88399-x
Huang, T., Zhao, Y., Meng, S., Chan, A. S. C., and Zhao, J. (2019). C7-Functionalization of indoles via organocatalytic enantioselective friedel-crafts alkylation of 4-aminoindoles with 2-butene-1, 4-diones and 3-aroylacrylates. Adv. Synth. Catal. 361, 3632–3638. doi:10.1002/adsc.201900377
Huang, W. J., Ma, Y. Y., Liu, L. X., Wu, B., Jiang, G. F., and Zhou, Y. G. (2021). Chiral phosphoric acid-catalyzed C6 functionalization of 2, 3-disubstituted indoles for synthesis of hetero-triarylmethanes. Org. Lett. 23, 2393–2398. doi:10.1021/acs.orglett.0c04002
Humphrey, G. R., and Kuethe, J. T. (2006). Practical methodologies for the synthesis of indoles. Chem. Rev. 106, 2875–2911. doi:10.1021/cr0505270
Ishikura, M., Abe, T., Choshi, T., and Hibino, S. (2015). Simple indole alkaloids and those with a nonrearranged monoterpenoid unit. Nat. Prod. Rep. 32, 1389–1471. doi:10.1039/c5np00032g
Leitch, J. A., McMullin, C. L., Mahon, M. F., Bhonoah, Y., and Frost, C. G. (2017). Remote C6-selective ruthenium-catalyzed C−H alkylation of indole derivatives via σ-activation. ACS Catal. 7 (4), 2616–2623. doi:10.1021/acscatal.7b00038
Ling, Y., An, D., Zhou, Y., and Rao, W. (2019). Ga(OTf)3-Catalyzed temperature-controlled regioselective friedel-crafts alkylation of trifluoromethylated 3-indolylmethanols with 2- substituted indoles: Divergent synthesis of trifluoromethylated unsymmetrical 3, 3′-and 3, 6’-Bis(indolyl)methanes. Org. Lett. 21, 3396–3401. doi:10.1021/acs.orglett.9b01135
Liu, H., Zheng, C., and You, S.-L. (2014). Catalytic C6 functionalization of 2, 3-disubstituted indoles by scandium triflate. J. Org. Chem. 79, 1047–1054. doi:10.1021/jo402511b
Poulsen, P. H., Feu, K. S., Paz, B. M., Jensen, F., and Jørgensen, K. A. (2015). Organocatalytic asymmetric 1, 6-addition/1, 4-addition sequence to 2, 4-dienals for the synthesis of chiral chromans. Angew. Chem. Int. Ed. Engl. 54, 8321–8325. doi:10.1002/ange.201503370
Rej, S., Ano, Y., and Chatani, N. (2020). Bidentate directing groups: An efficient tool in C−H bond functionalization Chemistry for the expedient construction of C−C bonds. Chem. Rev. 120 (3), 1788–1887. doi:10.1021/acs.chemrev.9b00495
Rostoll-Berenguer, J., Sanchez-García, R., Blay, G., Fernandez, I., Muñoz, M. C., and Pedro, J. R. (2018). Enantioselective synthesis of 2-amino-1, 1- diarylalkanes bearing a carbocyclic ring substituted indole through asymmetric catalytic reaction of hydroxyindoles with nitroalkene. J. Org. Chem. 83, 6397–6407.
Sandtorv, A. H. (2015). Transition metal-catalyzed C−H activation of indoles. Adv. Synth. Catal. 357, 2403–2435. doi:10.1002/adsc.201500374
Sherer, C., and Snape, T. J. (2015). Heterocyclic scaffolds as promising anticancer agents against tumours of the central nervous system: Exploring the scope of indole and carbazole derivatives. Eur. J. Med. Chem. 97, 552–560. doi:10.1016/j.ejmech.2014.11.007
Simonetti, M., Cannas, D. M., Panigrahi, M., Kujawa, S., Kryjewski, M., Xie, P., et al. (2017). Ruthenium-ruthenium-catalyzed C−H arylation of benzoic acids and indole carboxylic acids with aryl halides. Chem. Eur. J. 23 (3), 549–553. doi:10.1002/chem.201605068
Sravanthi, T. V., and Manju, S. L. (2016). Indoles-A promising scaffold for drug development. Eur. J. Pharm. Sci. 91, 1–10. doi:10.1016/j.ejps.2016.05.025
Street, L. J., Baker, R., Castro, J. L., Chambers, M. S., Guiblin, A. R., Hobbs, S. C., et al. (1993). Synthesis and serotonergic activity of 5-(oxadiazolyl)tryptamines: Potent agonists for 5-ht1d receptors. J. Med. Chem. 36 (11), 1529–1538. doi:10.1021/jm00063a003
Sugasawa, T., Adachi, M., Sasakura, K., and Kitagawa, A. (1979). Aminohaloborane in organic synthesis. 2. Simple synthesis of indoles and 1-acyl-3-indolinones using specific ortho .alpha.-chloroacetylation of anilines. J. Org. Chem. 44 (4), 578–586. doi:10.1021/jo01318a021
Sun, Y., Qiao, Y., Zhao, H., Li, B., and Chen, S. (2016). Construction of 9H-Pyrrolo[1, 2-a]indoles by a copper-catalyzed Friedel−Crafts alkylation/annulation cascade reaction. J. Org. Chem. 81, 11987–11993. doi:10.1021/acs.joc.6b02143
Vaden, S. L., Page, R. L., and Riviere, J. E. (1996). An in vitro-in vivo validation of the isolated perfused tumor and skin flap preparation as a model of cisplatin delivery to tumors. J. Pharmacol. Toxicol. Methods 35, 173–177. doi:10.1016/1056-8719(96)00044-5
Wang, H., Moselage, M., González, M. J., and Ackermann, L. (2016). Selective synthesis of indoles by cobalt(III)-Catalyzed C−H/N−O functionalization with nitrones. ACS Catal. 6 (4), 2705–2709. doi:10.1021/acscatal.5b02937
Wen, J., and Shi, Z. (2021). From C4 to C7: Innovative strategies for site-selective functionalization of indole C–H bonds. Acc. Chem. Res. 54 (7), 1723–1736. doi:10.1021/acs.accounts.0c00888
Wind, S., Schmid, U., Freiwald, M., Marzin, K., Lots, R., Ebner, T., et al. (2019). Clinical pharmacokinetics and pharmacodynamics of Nintedanib. Clin. Pharmacokinet. 58, 1131–1147. doi:10.1007/s40262-019-00766-0
Wu, Q., Li, G. L., Yang, S., Shi, X. Q., Huang, T.-A., Du, X.-H., et al. (2019). A chemo- and regioselective C6-functionalization of 2, 3-disubstituted indoles: Highly efficient synthesis of diarylindol-6-ylmethanes. Org. Biomol. Chem. 17, 3462–3470. doi:10.1039/c9ob00283a
Xiao, M., Xu, D., Liang, W., Wu, W., Chan, A. S. C., and Zhao, J. (2018). Organocatalytic enantioselective friedel-crafts alkylation/lactonization reaction of hydroxyindoles with methyleneoxindoles. Adv. Synth. Catal. 360, 917–924. doi:10.1002/adsc.201701089
Yan, J., Zhang, Z., Chen, M., Lin, Z., and Sun, J. (2020). A study of the reactivity of (Aza-)Quinone methides in selective C6-alkylations of indoles. ChemCatChem 12, 5053–5057. doi:10.1002/cctc.202000850
Yang, Y., and Shi, Z. (2018). Regioselective direct arylation of indoles on the benzenoid moiety. Chem. Commun. 54, 1676–1685. doi:10.1039/c7cc08752g
Yang, G. Q., Lindovska, P., Zhu, D. J., Kim, J., Wang, P., Tang, R. Y., et al. (2014). Pd(II)-Catalyzed meta-C-H olefination, arylation, and acetoxylation of indolines using a U-shaped template. J. Am. Chem. Soc. 136 (30), 10807–10813. doi:10.1021/ja505737x
Zhang, M. Z., Chen, Q., and Yang, G. F. (2015). A review on recent developments of indole containing antiviral agents. Eur. J. Med. Chem. 89, 421–441. doi:10.1016/j.ejmech.2014.10.065
Zhou, L. J., Zhang, Y. C., Zhao, J. J., Shi, F., and Tu, S. J. (2014). Organocatalytic arylation of 3-indolylmethanols via chemo- and regiospecific C6-functionalization of indoles. J. Org. Chem. 79, 10390–10398. doi:10.1021/jo501989x
Keywords: remote C6 functionalization of indoles, β,γ-unsaturated α-ketoester, brønsted acid, metal-free, 2,3-disubstituted indoles
Citation: Zhang Y-Y, Li L, Zhang X-Z and Peng J-B (2022) Brønsted acid catalyzed remote C6 functionalization of 2,3-disubstituted indoles with β,γ-unsaturated α-ketoester. Front. Chem. 10:992398. doi: 10.3389/fchem.2022.992398
Received: 12 July 2022; Accepted: 09 August 2022;
Published: 13 September 2022.
Edited by:
Shao-Hua Wang, Lanzhou University, ChinaReviewed by:
Sihua Hou, Shanghai Jiao Tong University, ChinaXinxin Qi, Zhejiang Sci-Tech University, China
Copyright © 2022 Zhang, Li, Zhang and Peng. This is an open-access article distributed under the terms of the Creative Commons Attribution License (CC BY). The use, distribution or reproduction in other forums is permitted, provided the original author(s) and the copyright owner(s) are credited and that the original publication in this journal is cited, in accordance with accepted academic practice. No use, distribution or reproduction is permitted which does not comply with these terms.
*Correspondence: Jin-Bao Peng, pengjb_05@126.com