- 1School of Chemistry and Chemical Engineering, Anshun University, Anshun, China
- 2College of Life Science, Sichuan Normal University, Chengdu, China
Environmental pollution caused by rapid industrial activities are becoming increasingly drastic, particularly its impact on soil and plant health. The present study was conducted to investigate the heavy metal (loid) (As, Cd, Cu, Hg, Pb, and Zn) concentrations in soils and food biomass crops and estimate the potential health risks of metals to humans via consumption of contaminated food biomass crops from Shifang, a periurban agricultural areas in the Chengdu Plain, Sichuan, China. Results revealed that the soils have been experiencing a substantial accumulation of heavy metals, especially for Cd, with a mean of 0.84 mg kg−1, about six times higher than the background values, of which 98% exceeded the pollution warning threshold of the China Soil Environmental Quality Standards. A total of 78% of all the grain part failed the national food standard for Cd. No significantly positive relationships between metal levels in food biomass crops and in the corresponding soils, indicated metals enrichment in soils were not entirely reflected to crops contaminant burdens. Estimated daily intake (EDI) of all the metals except for Pb, exceeded the oral reference dose (RfD) or the minimal risk levels recommended by USEPA and ATSDR. Target hazard quotients (THQs) of all the metals except for Cd was less than one indicated that potential health risk to the local inhabitant originated mainly from Cd exposure via cereals consumption. Mitigation strategies to curtail Cd-contaminated soils and crops Cd burdens need careful tailoring to meet the needs of health and safety in this region.
1 Introduction
Nowadays, environmental pollution and the greenhouse effect are becoming increasingly drastic. Among them, potential environmental and human risks of exposure to heavy metals through diet become an important issue of public health concern, but such information remains still fragmentary and scattered in an area of intensively cropping and heavily industrialized coexist (Masindi and Muedi, 2018; Briffa et al., 2020). Heavy metals are ubiquitous in the environment, with either natural or anthropogenic origin (Wu et al., 2016). Anthropogenic activities, such as mining, solid waste disposal, sludge applications, and industrial processing are the main sources of heavy metals soil contamination (Sodango et al., 2018; Timothy and Tagui Williams, 2019; Sharma et al., 2021). In addition, excessive use of pesticides and fertilizers, and wastewater irrigation also play an important role in the contamination of foodstuffs by heavy metals (Loutfy et al., 2012; Zwolak et al., 2019; Qin et al., 2021). Toxic heavy metals released by anthropogenic activities into ecosystems may lead to geo-accumulation, bio-accumulation, and biomagnification. In agricultural ecosystems, excessive accumulation of heavy metals in agricultural soils leads to elevated heavy metals uptake by food biomass crops, which is of great concern because of potential health risk to humans (Ali et al., 2019; Afonne and Ifediba, 2020; Hasan et al., 2020). Consumption of food biomass crops contaminated with heavy metals is a major food chain route for human exposure. Recently, there have been increasing interests in human health risk caused by consuming heavy metal contaminated food (Sall et al., 2020; Zheng et al., 2020; Ahmad et al., 2021; Alengebawy et al., 2021). The Food and Agricultural Organization of the United Nations (FAO), World Health Organization (WHO), United States Environmental Protection Agency (USEPA), the Agency for Toxic Substances and Disease Registry (ATSDR), and other regulatory bodies of various countries have established maximum permissible limits (MPL) of heavy metals in foodstuffs and offered some methods for health risk assessment. Based on these methods, numerous studies have been conducted on potential health risk assessment of heavy metals contaminated in soils and crops in different regions (Saha et al., 2016; Ishtiaq et al., 2018; Bello et al., 2019; Lien et al., 2021; Setia et al., 2021; Udom et al., 2022). But such information from an intensively cultivated areas remains still fragmentary (Bhatti et al., 2018; Zheng et al., 2020).
The Chengdu Plain, the“Land of Abundance” in China, is an important agricultural region and has also experiencing rapid change of socio-economic structur e changes. Urbanization, industrialization, and agricultural intensification have caused an increase of large amounts of metal-contained agrochemicals, wastes, and sewages in the agricultural environment. Previous studies in the Chengdu Plain reveal an obvious increase of heavy metals, especially cadmium (Cd) and mercury (Hg) in soils in the past decades (Qin et al., 2013; Wang et al., 2017; Deng et al., 2019; Wang et al., 2019). Increased heavy metals in soil results not only in soil quality deteriorating but may also affect agricultural product safety. There is a probable accumulation of heavy metals in crops grown in this region. However, studies have suggested that the knowledge of total concentration of metals alone is not sufficient to evaluate phytotoxic risk and human health risk (Yuswir et al., 2015; Tapia-Gatica et al., 2020); Huang et al. (2018) suggest that exposure to heavy metals through rice intake was the most important single health risk contributor. Dietary intake through contaminated foods has become the main route of heavy metal intake by humans (Chen et al., 2018). Therefore, the risk assessment of exposure to heavy metals through diet becomes an important health issue.
The coexist area of heavily industrialized and intensively cropping occupied a considerable proportion in the Chengdu Plain. Many previous studies only considered the levels of heavy metals in the soils and/or vegetables (Liu et al., 2004; Jin et al., 2008; Qin et al., 2013) and no studies have investigated the bio-accumulation of heavy metals in food crops from the soils in the coexist area. On the one hand, there are a large number of industrial pollution sources from such as the chemical industry, metal smelting, and cement production in the coexist areas, pollutants enter into farmland are inevitable through atmospheric deposition, wastewater, and solid waste discharge. On the other hand, highly intensive farming systems also bring a certain amount of heavy metals to the agricultural environment through the application of chemical fertilizers and pesticides. Health consequences of these pollution to local residents need to understand assessment, which has certain implications for changes in local planting structures and risk mitigation strategies or the safe usage of farmlands.
In order to enable the development of appropriate environmental and/or health guidelines, it is essential to have an understanding of the universal range of heavy metals concentrations in crops on the intensively cultivated area. Such data are also important to assist in assessing any potential risk to the environment or human health. The purpose of this study is to 1) identify the concentration of heavy metals in soils, rice, and maize; 2) evaluate the potential health risk associated with heavy metals through consumption of rice and maize using estimated daily intake (EDI) and target hazard quotient (THQ).
2 Materials and methods
2.1 Study area
The research area is composed of the whole flat area of Shifang city and parts of Guanghan city and Mianzhu city, situating at the northwest of the Chengdu Plain, Sichuan province, southwestern China (103°58′–104°22′E, 30°58′–31°23′N) (Figure 1). Climate is subtropical humid climate, with mean annual temperature of −16°C and mean annual rainfall of 940 mm. Parent materials of soils are mainly alluvial of the Min River. Major soil types are Hapli-Stagnic Anthrosols and Gleyi-Stagnic Anthrosols in the Chinese Soil Taxonomy (Gong and Li, 2001).As a heavily industrial activities and intensively farming coexist area, the study area has diverse industrial clusters, including food, metallurgical, construction materials, pharmaceutical, chemical, and leather industry in the flat part, whereas the mountainous/hill part being phosphorus ore, coal, and limestone mining.
Rice is cultivated on 90% of the total arable lands, rest be used for maize, wheat, vegetable, and mushroom cultivating (Wang M. et al., 2016). Agriculture depends on agrochemicals. It is a typical area that intensive crop farming mingled with heavily industrial operations in the Chengdu Plain.
2.2 Sample collection
The systematic random sampling method was used to collect samples. A sampling grid overlay on the study region (Figure 1), then the samples were collected at the node areas of a regular grid of about 3 km × 3 km. At each sampling site, a clean plastic shovel was used to collect 5–7 randomly subsamples at the 0–20 cm depth to form one representative composite sample of at least 500 g by the quartering method and put in a cleaned zip-lock plastic bag for the laboratory analysis. At each soil sampling sites, 3–5 subsamples of the edible part of maize mature seeds and/or the ear of rice were collected at random, and a composite sample at least 300 g was made for each crop. In total, the samples of 40 paddy soils, 10 dryland soils, 40 rice, and 10 maize were collected.
2.3 Analyses of samples
Soil samples were screened of debris and stones, air-dried, and crushed to pass through a 2-mm sieve. Each sample was homogeneized and quartered, representative subsamples of ≤2 mm size fraction were grounded in an agate mortar to pass a 0.149-mm sieve and prepared for chemical analysis. Samples of rice and maize were washed with deionized water to remove all visible soil particles or dusts, oven-dried at 60°C. After rice husks were removed, rice grains were grounded in a stainless steel mill to a fine powder and stored in plastic bags for further chemical analysis. Corn kernels were also prepared in the same way as analytical samples.
Soil pH was measured by a pH meter with soil/H2O ratio of 1:2.5 (soil:solution, dry w/v). The organic matter content was determined by the Walkley-Black procedure. Cation exchange capacity (CEC) was determined using NH4OAc at pH 7.0, the leaching method of the Soil Survey Staff (1996). Soil samples were digested by concentrated acid mixture (HNO3, HClO4, and HF), and food crop samples were digested with HNO3 and HClO4 in a 5:1 ratio. The acid digested soil and crop samples were filtered and diluted with distilled water to 50 and 10 ml, respectively. Concentration of total Pb, Cd, Cu, and Zn in the digests was measured using an atomic absorption spectrophotometer (Analyst 800 P.E.) equipped with a heated graphite furnace system (THGA-800 P.E.), while As and Hg were determined by atomic fluorescence spectrometer (AFS-830a).
2.4 Quality control and assurance
To ensure the quality of metals analysis, certified reference material (CRM) (from the National Research Center for Standards in China, Beijing) including Sichuan basin soil (GBW07428) and Sichuan rice flour (GBW10044) was used to validate the analysis. The average rice flour CRM recoveries ranged from 91 to 101%, 94 to 100%, 96 to 101%, 98 to 101%, 96 to 103%, and 90 to 105% for Cd, As, Pb, Cu, Zn, and Hg, respectively. The mean recoveries for soil CRM’s ranged from 89 to 100%, 97 to 104%, 98 to 102%, 97 to 100%, 98 to 101%, and 95 to 103% for Cd, As, Pb, Cu, Zn, and Hg.
2.5 Data analysis
2.5.1 Bio-accumulation factor
Metal concentrations of soils and grains were calculated on the basis of dry weight. The bio-accumulation factor (BAF), a ratio of the contaminant in food crops to the concentration in the soil substrate, was calculated using the following equation:
where Cplant and Csoil represent the heavy metal (loid) concentration in the edible part of food crops and soils on dry weight basis, respectively.
2.5.2 Estimated daily intake of metals
The estimated daily intake (EDI) of the specific metal depended on both the metal concentration in the edible part of food crops and the amount of consumption of the respective food. The EDI was determined by the following equation:
where Cmetal (mg kg−1, on dry weight basis) is the concentration of metals in contaminated crops; CF denotes the conversion factor, the Cmetal of both rice and maize were converted with a factor of 0.86 because home-stored rice and maize commonly contain water under 14% (w/w); Wfood represents the daily average consumption of food crops in the study area; and BW is the average body weight. According to the dietary intake surveyed by Zhu et al. (2000), the local inhabitants had an average consumption per person (average 65 kg in body weight) of 363 and 45 g/day for rice and maize, respectively, for children (average 30 kg in body weight), estimated intake account for about 60% of consumption for adults.
2.5.3 Target hazard quotient
Health risks for locals through the consumption of contaminated rice and maize was assessed based on the target hazard quotient (THQ). The THQ is a ratio of determined dose of a pollutant to a reference dose level. If the ratio is less than one, the exposed population is assumed to be safe (USEPA, 2012); THQ is described by the following equation:
where EF is the exposure frequency (365 days/year); ED is the exposure duration (70 years), equivalent to the average lifetime; FIR is the food ingestion rate (for adults, rice: 363 g/person/day, maize: 45 g/person/day; for children, rice: 218 g/person/day and maize: 27 g/person/day) (Zhu et al., 2000); C is the metal (loid) concentration in food (μg g−1); RfD is the oral reference dose (As = 0.3 μg kg−1 d−1, Hg = 0.16 μg kg−1 d−1, Cd = 1 μg kg−1 d−1, Pb = 4 μg kg−1 d−1, Cu = 10 μg kg−1 d−1, Zn = 300 μg kg−1 d−1) (USEPA, 2008; USEPA, 2013; ATSDR, 2013); BW is the average body weight (65 kg), and TA is the averaging exposure time for non-carcinogens (365 days/year ×ED).
2.5.4 Statistical analysis
Data were statistically analyzed using a statistical package SPSS 20. Shapiro–Wilk test is used to determine whether sample data have been drawn from a normally distributed population. When the assumption of normality was met, the mean was selected to test the statistical significance of the data, including comparison the mean of two and multiple groups and analysis of variance (ANOVA), with a significance level of p < 0.05, and the figures also presented with the mean values and standard errors. When the assumption of normality was violated, the median was selected to do Mann–Whitney test for two groups and Kruskal–Wallis test for multiple groups, and Spearman’s correlation analysis was used to test the correlation assumption.
3 Results
3.1 Heavy metals in soils
Basic soil characteristics and the concentrations of As, Cd, Hg, Pb, Cu, and Zn in soils are presented in Table 1. Soil is generally slightly acidic (mean pH 6.48), with a range of acidic (pH 4.54) to slightly alkaline (pH 7.99), in which pH value of 36 soil samples was less than seven, accounting for 72%. Soil organic matter (SOM) ranged between 54 and 112 g kg−1, with a mean of 101 g kg−1. Cation exchange capacity (CEC) varied considerably from 2.35 to 21.16 cmol kg−1, a difference of approximately nine times. The pH value in the rainfed lands that maize cultivated soils were not markedly different, comparing with the paddy fields that rice cultivated soils (p = 0.919). There was also no obvious difference in SOM between rainfed lands and paddy fields (p = 0.422). CEC in the paddy land was significantly lower than that in rainfed lands (p = 0.049).
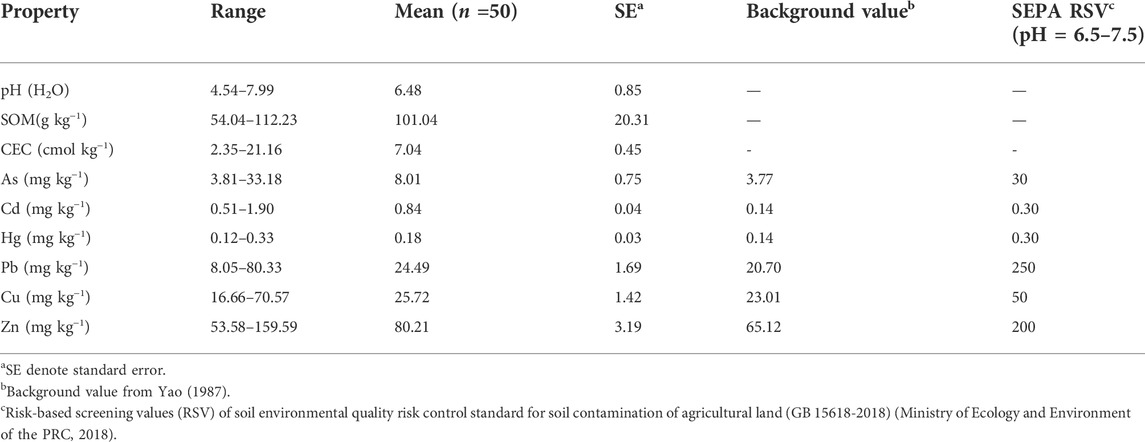
TABLE 1. Characteristics and metal levels of the soils collected from the study area (matters content on dry weight basis).
Mean concentrations of As, Cd, Hg, Pb, Cu, and Zn in soils were 8.01, 0.84, 0.19, 24.49, 25.72, and 80.12 mg kg−1, respectively (Table 1). Except for Cd, all other metals were below the risk control standard for soil contamination of agricultural land (Ministry of Ecology and Environment of the PRC, 2018) (Table 1). A considerable buildup for all the metals in the soils were observed when comparison with the background values, indicating that soils in the study area had a considerably contaminated by heavy metals, especially Cd and As. Concentration of metals was higher in rainfed soil than in paddy soil (Figure 2), with As (p = 0.001), Cd (p = 0.027), and Cu (p = 0.028) significantly higher in rainfed soil than paddy soil, and the rest [Pb (p = 0.156), Hg (p = 0.174), Zn (p = 0.47)] insignificantly different.
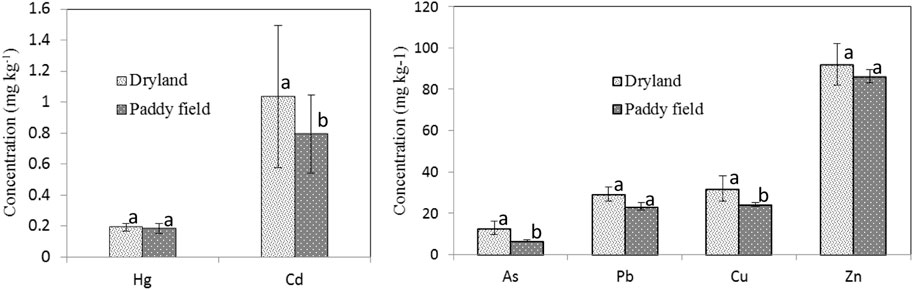
FIGURE 2. Metal concentrations (dry weight basis) in the soils from different types of farming in the study area. Data are mean ± 1SE, n = 40 for paddy soils and n = 10 for dryland soils. For each element, means with the same letter are not significantly (p > 0.05) different.
3.2 Heavy metals in food crops
The average concentrations and ranges of heavy metals (on dry weight basis) in the edible portions of food crops grown in the investigated soils are given in Table 2. The average concentrations of Cd in rice and maize were 0.46 and 0.26 mg kg−1, respectively (Table 2), 78% of crop samples exceeded the MPL for Cd of Chinese standard (Ministry of Health of the PRC, 2017). Mean content of Pb in rice and maize was 0.30 and 0.29 mg kg−1, respectively, also overtaking the MPL. The results indicated that both rice and maize in the study area exhibited a conspicuous Cd and Pb pollution. But As, Hg, Cu, and Zn concentrations were substantially lower than the MPL in rice and maize grown in the soils of the research area (Table 2). All the metal contents except for Cu (p = 0.001) and Cd (p =0.05) are insignificantly different between rice and maize, but the trends of heavy metal accumulations, in general, was in the order of rice > maize (Table 2).
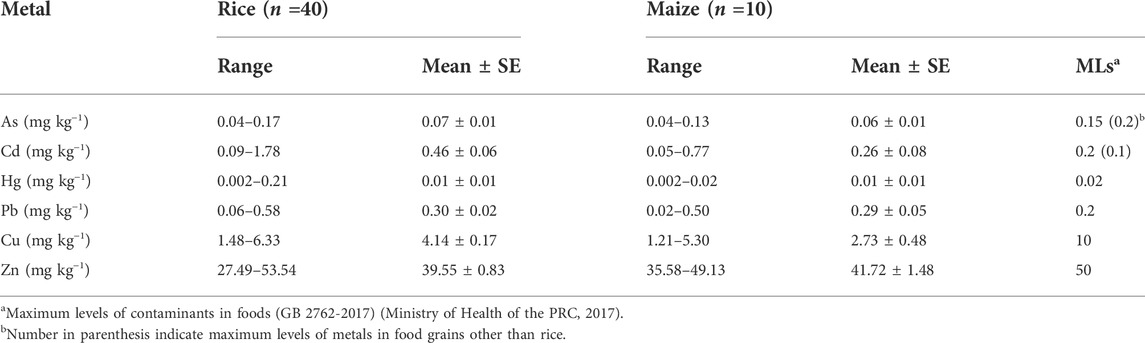
TABLE 2. Metals concentrations (on dry weight basis) in the edible parts of food crops collected from the study area.
3.3 Heavy metal transfer from soil to food crop
The bio-accumulation factor (BAF) for heavy metal transferring from soils to food crops tended to be in the order of Cd > Zn > Cu > Pb > Hg > As, and there was significantly difference in BAF values among metals (p < 0.001) (Figure 3). As, Cd, and Cu in the edible parts of rice were markedly higher than that of maize (Figure 3, all p < 0.05), whereas Hg, Pb, and Zn buildup in the corresponding parts of crops had no obvious difference. Correlation analysis reveals no significant correlation between metal concentrations in soils and in the edible parts of the plants except for Cu (p = 0.026), indicating that the metals content in plant does not fully reflect the total metal level in soils.
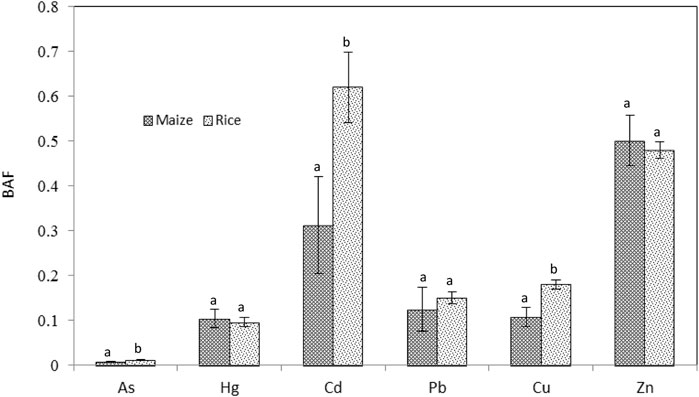
FIGURE 3. Bio-accumulation factors (BAF) for different metals, a ratio of heavy metals concentration in the edible part of maize and rice to that in the corresponding soil at the study area. Data are mean ± 1SE. For each element, means with the same letter are not significantly (p > 0.05) different.
3.4 Daily intake of metals through food consumption and human health risks
Daily intake of heavy metal was estimated based on the average food consumption in the study area. The estimated daily intake (EDI) via consumption of rice and maize for adults and children is given in Table 3. EDI of Hg, As, Pb, Cd, Cu, and Zn for adults was 0.11, 0.44, 1.62, 2.43, 21.52, and 214.83, respectively, whereas for children it was 0.31, 0.55, 2.11, 3.12, 38.02, and 379.52, respectively. According to the oral reference dose (RfD) recommended by USEPA, ASTDR and Cal EPA, EDI of As, Cd, and Cu for adults had exceeded the reference dose (Table 3), while EDI, except for that of Pb, for children, surpassed the recommended limit. Moreover, EDIs through the consumption of rice were significantly higher than through the consumption of maize because the dietary habits of local inhabitant are centered on rice. In particular, EDI values to the local children tended to be higher over adults, indicating that the children had a relatively significant health risks via the consumption of metals contaminated foods.
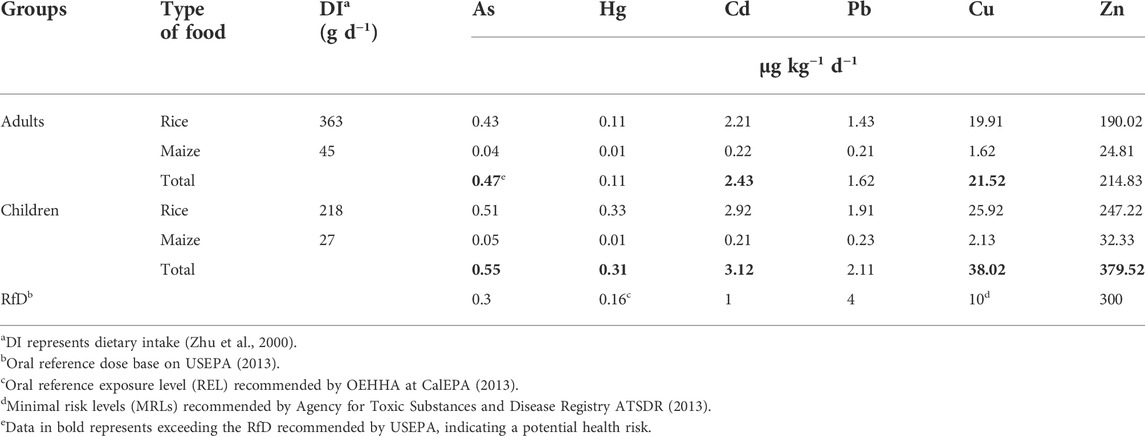
TABLE 3. Estimated daily intake (EDI) of metals by consumption of rice and maize at the investigation area (the EDI values based on the body weight of 65 and 30kg for the adults and children, respectively).
Target hazard quotient (THQ) of metals through the ingestion of rice and maize for adults and children is shown in Figure 4. While THQ of As, Hg, Pb, Zn, and Cu for adults was below one, indicating health risk was low, THQ of Cd was close to one, suggesting a potential health threat. Analogously, THQ of As, Hg, Pb, and Zn for children from consumption of rice and maize was below one, which suggested that health risk was insignificant. Conversely, THQ of Cd and Cu was bigger than one, indicating that health risk these two metals was of a concern.
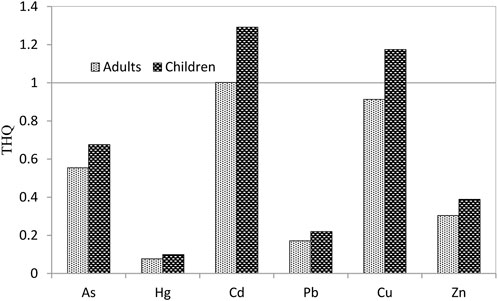
FIGURE 4. THQ values of metals through consumption of rice and maize grown at the sampling sites of the study area (a histogram above the reference line may be subjected to potentially higher health risk).
4 Discussion
4.1 Heavy metals in soils and crops
Increasing evidence (Ren et al., 2006; Tang et al., 2007; Jin et al., 2008; Qin et al., 2013) indicate that high Cd concentration in paddy soil and rice in the Chengdu Plain is a problem. All the metal concentrations except for Cd, although were still below the Grade II of the EQSS (SEPA, 2018), a substantial accumulation of metals in the soils was found when compared with the background values (Yao, 1987), on average concentrations of As, Hg, Pb, Cu, and Zn increasing up to 112, 30, 18, 12, and 23%, respectively (Table 1). These results agreed with the findings of previous studies in the Chengdu Plain (Qin et al., 2013; Wang et al., 2017; Deng et al., 2019). Cd concentrations in 49 soils were above the pollution warning threshold of the EQSS, accounting for about 98%, indicating that soils suffered generally from Cd contamination. This may have been due to the fact that diversity industries embedded in an intensively cultivated area, such as phosphorus chemicals, leather chemicals, old or currently active mining or ore processing facilities, with mine waste runoff or overspill tainted irrigation water, atmospheric deposition resulting from ore smelting, and application of agrochemicals, all may contribute to Cd contamination and others metals buildup in soils (Qin et al., 2013). Many previous studies suggested that long-term wastewater irrigation led to elevated levels of heavy metals in soils (Elbana et al., 2013; Christou et al., 2014; Meng et al., 2016; Abuzaid and Fadl, 2018) and revealed that the heavy metals content in soils were markedly influenced by stationary sources such as non-ferrous metal smelter, coal-fired power plant (Reza et al., 2015; Yang et al., 2017; Semenov et al., 2019; Wang et al., 2020), and non-point sources as use of fertilizers, pesticides, and bactericides (Ouyang et al., 2016; Zhang et al., 2021). These activities are inevitable in an intensively farming and heavily industrial activities coexist area.
Concentrations of Cd, As, and Cu in rainfed soils are significantly different from that in paddy soils (Figure 2), suggesting that different farming styles may potentially impact on metal concentrations in soil. It is a fact that rainfed lands are commonly used to cultivate vegetables, with a high ratio of rotation and increasing the input of agrochemicals, and more potential metals of anthropic sources being added to rainfed soils in compare to paddy soils. Metal elements may have different behaviors such as bioavailability, leachability, and mobility in various environments. An extractable form by DTPA is commonly applied to evaluate availability in previous many studies (Kim et al., 2015; Zahedifar et al., 2017; Kaninga et al., 2020). It was reported that Cd and Cu were readily extracted by DTPA compared to Pb and Zn (Singh et al., 1998), suggesting that Cd and Cu have more bioavailability in the same field condition, and therefore it should be possible to incur more leachability and/or mobility in a wet–dry cropping rotation due to the function of water. The mobile behavior of As in soils was affected by many factors such as pH and amorphous Al and Fe contents (Violante et al., 2010; Álvarez-Ayuso et al., 2016), especially, reduction condition possibly facilitates As releasing from soil because of reducing As (Ⅴ) to As (Ⅲ) (Pigna et al., 2015; Guénet et al., 2017). These behaviors would show why that As levels was different between rainfed soils and paddy soils.
Heavy metal accumulation in crops is a serious concern due to potential public health implications. The data from present study indicated that the average concentration of Cd in rice and maize was 2.3 and 2.6-fold higher than the MPL (Table 2), respectively. Similarly, Pb contents in rice and maize also surpassed the MPL by about1.5 times (Table 2). The concentration of Cd in rice from the Chengdu Plain was in balance with the findings of previous studies in a mining-affected area of Hunan province (Du et al., 2013; Chen et al., 2016; Wang X. et al., 2016) but lower than those in the Dabaoshan mine area in Guangdong province (Zhuang et al., 2009). While the Cd level in corn grains was beyond that of the corn grown at Qingchengzi Pb/Zn mine soil in Liaoning province (Li et al., 2014). On average buildup of Pb in the edible part of both rice and maize reached up to about 0.30 mg kg−1, ranging from 0.06 to 0.58 mg kg−1 for rice and 0.02–0.50 mg kg−1 for maize (Table 2), which was less than those in mining-affected areas (Zhuang et al., 2009). Analysis showed that no strong positive relationship between metals in the soils and in the crops. Conversely, a negative relationship between soil metal contents and crops for Cu, in addition to Cd were observed. Such inverse relationships were also reported by Khan et al. (2008) for vegetables. This may suggest that knowing total metal levels in soils use to assess health risk is inappropriate.
Soil-to-plant transfer is one of key pathways of human exposure to metals through food chain (Loutfy et al., 2006). Our results showed that BAF differ significantly among metals (p < 0.001) or between crops (Figure 3). Seemingly, Cd, As, and Cu transfer from soil to rice were easier than to maize (all p < 0.05), but the rest of the metals did not like such trend. However, the Cd, As, and Cu levels in rainfed soils where maize was cultivated, on the whole, are higher than those in paddy soils where rice was cultivated (Figure 2), suggesting that the accumulation effect depends not only on the crop’s physiological properties but also on mobility and availability of metals in soils, it does not appear to be entirely associated with the total element concentrations in the soils. Some studies found that leafy vegetables can generally accumulate Pb and Cd to a higher extent than non-leafy vegetables (Zhuang et al., 2009; Nabulo et al., 2010; Chang et al., 2014; Gebeyehu and Bayissa, 2020). Cd is usually considered a highly mobile heavy metal in regard to moving from soil-to-plant and is of primary concern in soil and food contamination, particularly in rice cropping systems (Kim et al., 2015; Zhao et al., 2015). A high average BAF for Cd in rice correspond to a lower mean content of total Cd in paddy soils (Figures 2, 3). Gu et al. (2018) investigated the BAF of rice for Cd, Cu, Pb, and Zn, the results indicated that Cd and Zn showed stronger bio-accumulation and mobility capability. These findings demonstrate that Cd accumulation in rice is mainly influenced by its availability, rather than total amount in soils, which support the conclusions of many previous studies (Du et al., 2013; Jing et al., 2020).
4.2 Health risk assessment
An important aspect in assessing risk to human health from potentially harmful chemicals in food is the knowledge of the dietary intake of such substances. Based on average concentration of metals in the edible part of each food crops and the respective consumption rate (Zhu et al., 2000), EDIs of As, Cd, and Cu by consumption of rice and corn grains for the local adults were 0.44, 0.24, and 21.52 μg kg−1 d−1, and for the local children were 0.55, 0.31, and 38.02 μg kg−1 d−1, respectively (Table 3). These EDIs are far below those in the mining-affected areas (Zhuang et al., 2009; Du et al., 2013), but exceed the oral reference dose (RfD) recommended by USEPA (2013) and ATSDR (2013). Analogously, EDIs of Hg and Pb for the local children exceed also the RfD limits, but not for adults (Table 3). The EDIs through consumption of rice were significantly higher than that of maize due to rice as staple crop of local inhabitant. Thus, adverse health effects induced by ingesting contaminated food crops arise largely from rice consumption. Moreover, the local children intakes of metals by consumption of contaminated food crops was about 1.25–2.8 times higher than those of the local adults due to children consumption 1.3 times more food than adults relative to their body weight (Table 3). A similar phenomenon was also reported by Kim et al. (2013), they found that the mean intakes of Cd at ages 1–2 were the highest in different age groups of Korea through the intake of various agricultural products grown in greenhouse. Ding et al. (2018) investigated trace elements in soils and selected agricultural plants in the Tongling mining area of China, their findings revealed that EDI of the trace elements, except Cd, were generally below the maximum tolerable daily intake. These estimates were also consistent with the long-term dietary intake assessment on other contaminants performed by the FAO/WHO (FAO/WHO, 2005). Therefore, children as a susceptible group have a higher health risk through consuming the same contaminated foodstuff than adults.
The estimation of target hazard quotient (THQ) offers an indication of the risk level due to pollutant exposure (USEPA, 2017; Shaheen et al., 2016; Miranzadeh Mahabadi et al., 2020). Estimated THQs of As, Hg, Pb, Zn, and Cu through consumption of rice and maize were below one for adults, while this value for Cd approached one. Concerning children, THQs of As, Hg, Pb, and Zn were also less than one, but for Cd and Cu were beyond one, suggesting that Cd and Cu exposure through daily intakes of rice and corn grains locally produced have posed a severe health risk to the local residents (Figure 4), in agreement with the conclusion of Jin et al. (2009). Although the ingested dose of heavy metals from cereals is not equal to the absorbed pollutant dose in reality due to a fraction of intake heavy metals being excreted (Balkhair and Ashraf, 2016; Yaradua et al., 2020), if considering dietary intakes from the locally produced non-cereal foods consumption such as vegetables, meat, eggs, and milk, THQ of metals, especially Cd, is certainly higher and the health risk even more severe. Consequently, effective mitigation measures are necessary to cure Cd-contaminated soils and to reduce the metal transferring from soil to crops in this region.
5 Conclusion
The soils from a heavily industrialized and intensively cultivated area in the Chengdu Plain have been experiencing a considerable accumulation of heavy metals comparison with the background values. These enrichments are not entirely reflected to crops metals burdens due to difference in bioavailability and/or mobility among metals and/or in physiological properties between crops. Health risk identified by the estimated daily intake (EDI) and target hazard quotients (THQ) suggest that potential health risk to the local inhabitant is mainly from Cd exposure, resulting from rice consumption. Mitigation strategies to curtail Cd-contaminated soils and crops Cd burdens need careful tailoring to meet the needs of health and safety in this region in the future.
Data availability statement
The original contributions presented in the study are included in the article/Supplementary Material; further inquiries can be directed to the corresponding author.
Author contributions
QL: Investigation, Visualization, Writing-original draft. XL: Methodology, Investigation, Formal analysis, LH: Conceptualization, Supervision, Writing-review and editing.
Funding
This work was financially supported by the Creative Research Groups Support Program of Guizhou Education Department [KY (2017) 049], the Guizhou Province Key Laboratory of Ecological Protection and Restoration of Typical Plateau Wetlands [(2020) 2002], and the Youth Growth S&T Personnel Foundation of the Guizhou Education Department [KY (2017) 278].
Conflict of interest
The authors declare that the research was conducted in the absence of any commercial or financial relationships that could be construed as a potential conflict of interest.
Publisher’s note
All claims expressed in this article are solely those of the authors and do not necessarily represent those of their affiliated organizations, or those of the publisher, the editors, and the reviewers. Any product that may be evaluated in this article, or claim that may be made by its manufacturer, is not guaranteed or endorsed by the publisher.
References
Abuzaid, A. S., and Fadl, M. E. (2018). Mapping potential risks of long-term wastewater irrigation in alluvial soils, Egypt[J]. Arabian J. Geosciences 11 (15), 1–12. doi:10.1007/s12517-018-3780-3
Afonne, O. J., and Ifediba, E. C. (2020). Heavy metals risks in plant foods – need to step up precautionary measures. Curr. Opin. Toxicol. 22, 1–6. doi:10.1016/j.cotox.2019.12.006
Ahmad, W., Alharthy, R. D., Zubair, M., Ahmed, M., Hameed, A., and Rafique, S. (2021). Toxic and heavy metals contamination assessment in soil and water to evaluate human health risk. Sci. Rep. 11 (1), 17006–17012. doi:10.1038/s41598-021-94616-4
Alengebawy, A., Abdelkhalek, S. T., Qureshi, S. R., and Wang, M. Q. (2021). Heavy metals and pesticides toxicity in agricultural soil and plants: Ecological risks and human health implications. Toxics 9 (3), 42. doi:10.3390/toxics9030042
Ali, H., Khan, E., and Ilahi, I. (2019). Environmental Chemistry and ecotoxicology of hazardous heavy metals: Environmental persistence, toxicity, and bioaccumulation. J. Chem. 2019, 1–14. doi:10.1155/2019/6730305
Álvarez-Ayuso, E., Abad-Valle, P., Murciego, A., and Villar-Alonso, P. (2016). Arsenic distribution in soils and rye plants of a cropland located in an abandoned mining area[J]. Sci. Total Environ. 542, 238–246. doi:10.1016/j.scitotenv.2015.10.054
ATSDR (2013). “Toxicological profile for uranium,” in Agency for toxic Substances and Disease Registry (A TSDR) toxicological profiles (Atlanta (GA): Agency for Toxic Substances and Disease Registry US).
Balkhair, K. S., and Ashraf, M. A. (2016). Field accumulation risks of heavy metals in soil and vegetable crop irrigated with sewage water in Western region of Saudi Arabia. Saudi J. Biol. Sci. 23 (1), S32–S44. doi:10.1016/j.sjbs.2015.09.023
Bello, S., Nasiru, R., Garba, N. N., and Adeyemo, D. (2019). Carcinogenic and non-carcinogenic health risk assessment of heavy metals exposure from Shanono and Bagwai artisanal gold mines, Kano state, Nigeria. Sci. Afr. 6, e00197. doi:10.1016/j.sciaf.2019.e00197
Bhatti, S. S., Kumar, V., Sambyal, V., Singh, J., and Nagpal, A. K. (2018). Comparative analysis of tissue compartmentalized heavy metal uptake by common forage crop: A field experiment. Catena 160, 185–193. doi:10.1016/j.catena.2017.09.015
Briffa, J., Sinagra, E., and Blundell, R. (2020). Heavy metal pollution in the environment and their toxicological effects on humans. Heliyon 6 (9), e04691. doi:10.1016/j.heliyon.2020.e04691
CalEPA (California Environmental Protection Agency) (2013). Technical support document for noncancer RELs, Appendix D.1. Sacramento, CA: Office of Environmental Health Hazard Assessment.
Chang, C. Y., Yu, H. Y., Chen, J. J., Li, F. B., Zhang, H. H., and Liu, C. P. (2014). Accumulation of heavy metals in leaf vegetables from agricultural soils and associated potential health risks in the Pearl River Delta, South China. Environ. Monit. Assess. 186 (3), 1547–1560. doi:10.1007/s10661-013-3472-0
Chen, D., Guo, H., Li, R., Li, L., Pan, G., Chang, A., et al. (2016). Low uptake affinity cultivars with biochar to tackle Cd-tainted rice — a field study over four rice seasons in hunan, China. Sci. total Environ. 541, 1489–1498. doi:10.1016/j.scitotenv.2015.10.052
Chen, L., Zhou, S., Shi, Y., Wang, C., Li, B., Li, Y., et al. (2018). Heavy metals in food crops, soil, and water in the Lihe River Watershed of the Taihu Region and their potential health risks when ingested. Sci. total Environ. 615, 141–149. doi:10.1016/j.scitotenv.2017.09.230
Christou, A., Eliadou, E., Michael, C., Hapeshi, E., and Fatta-Kassinos, D. (2014). Assessment of long-term wastewater irrigation impacts on the soil geochemical properties and the bioaccumulation of heavy metals to the agricultural products. Environ. Monit. Assess. 186 (8), 4857–4870. doi:10.1007/s10661-014-3743-4
Deng, S., Yu, J., Wang, Y., Xie, S., Ran, Z., and Wei, W. (2019). Distribution, transfer, and time-dependent variation of Cd in soil-rice system: A case study in the Chengdu Plain, southwest China. Soil Tillage Res. 195, 104367. doi:10.1016/j.still.2019.104367
Ding, Z., Li, Y., Sun, Q., and Zhang, H. (2018). Trace elements in soils and selected agricultural plants in the Tongling mining area of China. Int. J. Environ. Res. Public Health. 15 (2), 202. doi:10.3390/ijerph15020202
Du, Y., Hu, X. F., Wu, X. H., Shu, Y., Jiang, Y., and Yan, X. J. (2013). Affects of mining activities on Cd pollution to the paddy soils and rice grain in Hunan province, Central South China. Environ. Monit. Assess. 185 (12), 9843–9856. doi:10.1007/s10661-013-3296-y
Elbana, T. A., Ramadan, M. A., Gaber, H. M., Bahnassy, M. H., Kishk, F. M., and Selim, H. (2013). Heavy metals accumulation and spatial distribution in long term wastewater irrigated soils. J. Environ. Chem. Eng. 1 (4), 925–933. doi:10.1016/j.jece.2013.08.005
FAO/WHO (2005). Food safety risk analysis. Part I. An overview and framework manual. Rome: Food and Agriculture Organization of the United Nations and World Health Organization, 448. http://www.fao.org/3/a0822e/a0822e.
Gebeyehu, H. R., and Bayissa, L. D. (2020). Levels of heavy metals in soil and vegetables and associated health risks in Mojo area, Ethiopia. PloS one 15 (1), e0227883. doi:10.1371/journal.pone.0227883
Gu, Q., Yang, Z., Yu, T., Yang, Q., Hou, Q., and Zhang, Q. (2018). From soil to rice—a typical study of transfer and bioaccumulation of heavy metals in China. Acta Agric. Scand. B Soil Plant Sci. 68 (7), 631–642. doi:10.1080/09064710.2018.1455218
Guénet, H., Davranche, M., Vantelon, D., Bouhnik-Le Coz, M., Jardé, E., Dorcet, V., et al. (2017). Highlighting the wide variability in arsenic speciation in wetlands: A new insight into the control of the behavior of arsenic[J]. Geochimica Cosmochimica Acta 203, 284–302. doi:10.1016/j.gca.2017.01.013
Hasan, A. B., Reza, A. H. M., Kabir, S., Siddique, M. A. B., Ahsan, M. A., and Akbor, M. A. (2020). Accumulation and distribution of heavy metals in soil and food crops around the ship breaking area in southern Bangladesh and associated health risk assessment. SN Appl. Sci. 2 (2), 1–18. doi:10.1007/s42452-019-1933-y
Huang, Y., Chen, Q., Deng, M., Japenga, J., Li, T., Yang, X., et al. (2018). Heavy metal pollution and health risk assessment of agricultural soils in a typical peri-urban area in southeast China. J. Environ. Manag. 207, 159–168. doi:10.1016/j.jenvman.2017.10.072
Ishtiaq, M., Jehan, N., Khan, S. A., Muhammad, S., Saddique, U., Iftikhar, B., et al. (2018). Potential harmful elements in coal dust and human health risk assessment near the mining areas in Cherat, Pakistan. Environ. Sci. Pollut. Res. 25 (15), 14666–14673. doi:10.1007/s11356-018-1655-5
Jin, L. X., Hou, Q. Y., Bao, Y. H., Xu, Z., Li, Z. H., Yang, Z. F., et al. (2009). Assessment of ecological security and human health risk in cadmium-contaminated farmland areas in Deyang (in Chinese with English abstract)[J]. Mod. Geol. 22 (6), 984–989. doi:10.3969/j.issn.1000-8527.2008.06.014
Jin, L. X., Hou, Q. Y., Yang, Z. F., Bao, Y. H., Li, Z. H., Xu, Z., et al. (2008). Health risk assessment of heavy metals in farmland ecosystems in Deyang, Sichuan (in Chinese with English abstract) [J]. Front. Geosciences 15 (5), 47–56.
Jing, F., Chen, C., Chen, X., Liu, W., Wen, X., Hu, S., et al. (2020). Effects of wheat straw derived biochar on cadmium availability in a paddy soil and its accumulation in rice. Environ. Pollut. 257, 113592. doi:10.1016/j.envpol.2019.113592
Kaninga, B., Chishala, B. H., Maseka, K. K., Sakala, G. M., Young, S. D., Lark, R. M., et al. (2020). Do soil amendments used to improve agricultural productivity have consequences for soils contaminated with heavy metals? Heliyon 6 (11), e05502. doi:10.1016/j.heliyon.2020.e05502
Khan, S., Cao, Q., Zheng, Y. M., Huang, Y., and Zhu, Y. (2008). Health risks of heavy metals in contaminated soils and food crops irrigated with wastewater in Beijing, China. Environ. Pollut. 152 (3), 686–692. doi:10.1016/j.envpol.2007.06.056
Kim, R. Y., Yoon, J. K., Kim, T. S., Yang, J. E., and Owens, G. (2015). Bioavailability of heavy metals in soils: Definitions and practical implementation—a critical review. Environ. Geochem. Health 37 (6), 1041–1061. doi:10.1007/s10653-015-9695-y
Kim, W. I., Ji-Ho, L., Anitha, K., and Doo-Ho, K. (2013). Dietary exposure estimates of trace elements in selected agricultural products grown in greenhouse and associated health risks in Korean population. J. Agric. Chem. Environ. 2 (3), 35–41. doi:10.4236/jacen.2013.23006
Li, Z., Ma, Z., van der Kuijp, T. J., Yuan, Z., and Huang, L. (2014). A review of soil heavy metal pollution from mines in China: Pollution and health risk assessment. Sci. total Environ. 468, 843–853. doi:10.1016/j.scitotenv.2013.08.090
Lien, K. W., Pan, M. H., and Ling, M. P. (2021). Levels of heavy metal cadmium in rice (Oryza sativa L.) produced in Taiwan and probabilistic risk assessment for the Taiwanese population. Environ. Sci. Pollut. Res. 28 (22), 28381–28390. doi:10.1007/s11356-020-11902-w
Liu, H. Y., Xie, Z. R., Chen, D. Y., Zhou, X. M., Feng, X. M., and Guo, K. Y. (2004). Preliminary assessment of soil environmental quality in Chengdu (in Chinese with English abstract) [J]. J. Environ. Sci. 24 (2), 297–303.
Loutfy, N., Fuerhacker, M., Tundo, P., Raccanelli, S., El Dien, A., and Ahmed, M. T. (2006). Dietary intake of dioxins and dioxin-like PCBs, due to the consumption of dairy products, fish/seafood and meat from Ismailia city, Egypt. Sci. total Environ. 370 (1), 1–8. doi:10.1016/j.scitotenv.2006.05.012
Loutfy, N., Mentler, A., Shoeab, M., Ahmed, M. T., and Füerhacker, M. (2012). Analysis and exposure assessment of some heavy metals in foodstuffs from Ismailia city, Egypt. Toxicol. Environ. Chem. 94 (1), 78–90. doi:10.1080/02772248.2011.638445
Masindi, V., and Muedi, K. L. (2018). Environmental contamination by heavy metals[J]. Heavy met. 10, 115–132.
Meng, W., Wang, Z., Hu, B., Li, H., and Goodman, R. C. (2016). Heavy metals in soil and plants after long-term sewage irrigation at tianjin China: A case study assessment. Agric. water Manag. 171, 153–161. doi:10.1016/j.agwat.2016.03.013
Ministry of Ecology and Environment of the PRC (2018). Soil environmental quality Risk control standard for soil contamination of agricultural land (GB 15618-2018) (in Chinese) [S]. Beijing: China Environmental Science Press.
Ministry of Health of the PRC (2017). National food safety standard maximum Levels of pollutants in foods (GB 2762-2017) (in Chinese) [S].
Miranzadeh Mahabadi, H., Ramroudi, M., Asgharipour, M. R., Rahmani, H. R., and Afyuni, M. (2020). Assessment of heavy metals contamination and the risk of target hazard quotient in some vegetables in Isfahan[J]. Pollution 6 (1), 69–78. doi:10.22059/POLL.2019.285113.645
Nabulo, G., Young, S. D., and Black, C. R. (2010). Assessing risk to human health from tropical leafy vegetables grown on contaminated urban soils. Sci. Total Environ. 408 (22), 5338–5351. doi:10.1016/j.scitotenv.2010.06.034
Ouyang, W., Jiao, W., Li, X., Giubilato, E., and Critto, A. (2016). Long-term agricultural non-point source pollution loading dynamics and correlation with outlet sediment geochemistry. J. Hydrology 540, 379–385. doi:10.1016/j.jhydrol.2016.06.043
Pigna, M., Caporale, A. G., Cavalca, L., Sommella, A., and Violante, A. (2015). Arsenic in the soil environment: Mobility and phytoavailability. Environ. Eng. Sci. 32 (7), 551–563. doi:10.1089/ees.2015.0018
Qin, G., Niu, Z., Yu, J., Li, Z., Ma, J., and Xiang, P. (2021). Soil heavy metal pollution and food safety in China: Effects, sources and removing technology. Chemosphere 267, 129205. doi:10.1016/j.chemosphere.2020.129205
Qin, Y. S., Yu, H., Feng, W. Q., Wang, Z. Y., and Tu, S. H. (2013). Heavy metal content and potential ecological risk assessment in paddy soil in northern Chengdu Plain (in Chinese with English abstract) [J]. Chin. J. Ecol. 33 (19), 6335–6344.
Ren, L., Qin, B., Tang, W., Ma, Z., and Hu, Y. (2006). Analysis on the sources of mercury in soils in the chengdu basin, china (in Chinese with Englishabstract) [J]. Chin. J. Geochem. 25, 10–10. doi:10.1007/BF02839750
Reza, S. K., Baruah, U., Singh, S. K., and Das, T. H. (2015). Geostatistical and multivariate analysis of soil heavy metal contamination near coal mining area, Northeastern India. Environ. Earth Sci. 73 (9), 5425–5433. doi:10.1007/s12665-014-3797-1
Saha, N., Mollah, M. Z. I., Alam, M. F., and Safiur Rahman, M. (2016). Seasonal investigation of heavy metals in marine fishes captured from the Bay of Bengal and the implications for human health risk assessment. Food control. 70, 110–118. doi:10.1016/j.foodcont.2016.05.040
Sall, M. L., Diaw, A. K. D., Gningue-Sall, D., Efremova Aaron, S., and Aaron, J. J. (2020). Toxic heavy metals: Impact on the environment and human health, and treatment with conducting organic polymers, a review. Environ. Sci. Pollut. Res. 27 (24), 29927–29942. doi:10.1007/s11356-020-09354-3
Semenov, D. O., Fatjejev, A. I., Smirnova, K. B., Shemet, A. M., Lykova, O. A., Tyutyunnyk, N. V., et al. (2019). Geochemical and anthropogenic factors of variability of heavy metals content in the soils and crops of Ukraine at the example of copper[J]. Environ. Monit. Assess. 191 (8), 1–9. doi:10.1007/s10661-019-7622-x
SEPA (2018). Soil environmental quality Risk control standard for soil contamination of agricultural land (GB15618-2018)[S] (Beijing: China Environmental Science Press).
Setia, R., Dhaliwal, S. S., Singh, R., Kumar, V., Taneja, S., Kukal, S. S., et al. (2021). Phytoavailability and human risk assessment of heavy metals in soils and food crops around Sutlej river, India. Chemosphere 263, 128321. doi:10.1016/j.chemosphere.2020.128321
Shaheen, N., Ahmed, M., Islam, M., Habibullah-Al-Mamun, M., Tukun, A. B., Islam, S., et al. (2016). Health risk assessment of trace elements via dietary intake of ‘non-piscine protein source’ foodstuffs (meat, milk and egg) in Bangladesh. Environ. Sci. Pollut. Res. 23 (8), 7794–7806. doi:10.1007/s11356-015-6013-2
Sharma, P., Kumar, S., and Pandey, A. (2021). Bioremediated techniques for remediation of metal pollutants using metagenomics approaches: A review. J. Environ. Chem. Eng. 9 (4), 105684. doi:10.1016/j.jece.2021.105684
Singh, S. P., Tack, F. M., and Verloo, M. G. (1998). Heavy metal fractionation and extractability in dredged sediment derived surface soils[J. Water Air Soil Pollut. 102 (3), 313–328. doi:10.1023/a:1004916632457
Sodango, T. H., Li, X., Sha, J., and Bao, Z. (2018). Review of the spatial distribution, source and extent of heavy metal pollution of soil in China: Impacts and mitigation approaches. J. health Pollut. 8 (17), 53–70. doi:10.5696/2156-9614-8.17.53
Soil Survey Staff (1996). Soil survey laboratory methods manual. Soil Survey Investigations Report, No. 42. Ver. 3.0. Lincoln, NE: USDA-NRCS.
Tang, W. C., Zhang, X. Z., He, Y. S., and Qin, B. (2007). Distribution characteristics and origion of Cd, Pb and Zn in surface soil in the plain area of the Chengdu Basin (in Chinese with English abstract) [J]. Geochem. 36 (1), 89–97. doi:10.3321/j.issn:0379-1726.2007.01.010
Tapia-Gatica, J., González-Miranda, I., Salgado, E., Bravo, M. A., Tessini, C., Dovletyarova, E. A., et al. (2020). Advanced determination of the spatial gradient of human health risk and ecological risk from exposure to As, Cu, Pb, and Zn in soils near the Ventanas Industrial Complex (Puchuncaví, Chile). Environ. Pollut. 258, 113488. doi:10.1016/j.envpol.2019.113488
Timothy, N., and Tagui Williams, E. (2019). Environmental pollution by heavy metal: An overview. Int. J. Environ. Chem. 3 (2), 72–82. doi:10.11648/j.ijec.20190302.14
Udom, G. J., Obilor, O. F., Ise, U. P., Nkechi, O., Udoh, A., Aturamu, A., et al. (2022). Heavy metal content of a polyherbal drug: Any exposure-associated public health risks?[J]. J. Health Sci. Surveillance Syst. 10 (2), 189–196. doi:10.30476/JHSSS.2021.93527.1457
USEPA (2008). Child-Specific Exposure factors handbook (2008, Final Report). Available at: http://cfpub.epa.gov/ncea/cfm/recordisplay.cfm?deid=199243 (Accessed July 2, 2022).
USEPA (2012). EPA region III risk-based concentration (RBC) table, region III. Philadelphia, Pennsylvania: Environmental Protection Agency.
USEPA (2013). Regional Screening Levels (RSLs)-Generic Tables. Available at: https://www.epa.gov/risk/regional-screening-levels-rsls-generic-tables (Accessed July 2, 2022).
USEPA (2017). Mid atlantic risk assessment, regional screening levels (RSLs) - generic tables. Available at: http://www.epa.gov/region9/superfund/prg (Accessed May, 2017).
Violante, A., Cozzolino, V., Perelomov, L., Caporale, A. G., and Pigna, M. (2010). Mobility and bioavailability of heavy metals and metalloids in soil environments[J]. J. soil Sci. plant Nutr. 10 (3), 268–292. doi:10.4067/S0718-95162010000100005
Wang, G., Zhang, S., Xiao, L., Zhong, Q., Li, L., Xu, G., et al. (2017). Heavy metals in soils from a typical industrial area in sichuan, China: Spatial distribution, source identification, and ecological risk assessment. Environ. Sci. Pollut. Res. 24 (20), 16618–16630. doi:10.1007/s11356-017-9288-7
Wang, M., Chen, W., and Peng, C. (2016). Risk assessment of Cd polluted paddy soils in the industrial and township areas in Hunan, Southern China. Chemosphere 144, 346–351. doi:10.1016/j.chemosphere.2015.09.001
Wang, X., Bai, J., Wang, J., Le, S., Wang, M., and Zhao, Y. (2019). Variations in cadmium accumulation and distribution among different oilseed rape cultivars in Chengdu Plain in China. Environ. Sci. Pollut. Res. 26 (4), 3415–3427. doi:10.1007/s11356-018-3857-2
Wang, X., Yu, D., Li, C., Pan, Y., Pan, J., Wang, X., et al. (2016). Characteristics of variations in the organic carbon fractions in paddy soils. Soil Sci. Soc. Am. J. 80 (4), 983–991. doi:10.2136/sssaj2016.03.0063
Wang, Y., Duan, X., and Wang, L. (2020). Spatial distribution and source analysis of heavy metals in soils influenced by industrial enterprise distribution: Case study in Jiangsu Province. Sci. Total Environ. 710, 134953. doi:10.1016/j.scitotenv.2019.134953
Wu, X., Cobbina, S. J., Mao, G., Xu, H., Zhang, Z., and Yang, L. (2016). A review of toxicity and mechanisms of individual and mixtures of heavy metals in the environment. Environ. Sci. Pollut. Res. 23 (9), 8244–8259. doi:10.1007/s11356-016-6333-x
Yang, Y., Christakos, G., Guo, M., Xiao, L., and Huang, W. (2017). Space-time quantitative source apportionment of soil heavy metal concentration increments. Environ. Pollut. 223, 560–566. doi:10.1016/j.envpol.2017.01.058
Yao, T. S. (1987). Study on the background values of eleven chemical elements in alluvial paddy soils and grain crops (wheat, rice) in the agricultural economic natural area of the Western Sichuan Plain [J]. J. Sichuan Normal Univ. Nat. Sci. Ed. (Res. Album Backgr. Value Elem. Soil Grain), 1–67. (in Chinese with English abstract).
Yaradua, A. I., Alhassan, A. J., Nasir, A., Matazu, S. S., Usman, A., Idi, A., et al. (2020). Human health risk assessment of heavy metals in onion bulbs cultivated in katsina state, north west Nigeria. Archives Curr. Res. Int. 20 (2), 30–39. doi:10.9734/acri/2020/v20i230175
Yuswir, N. S., Praveena, S. M., Aris, A. Z., Ismail, S. N. S., and Hashim, Z. (2015). Health risk assessment of heavy metal in urban surface soil (klang district, Malaysia). Bull. Environ. Contam. Toxicol. 95 (1), 80–89. doi:10.1007/s00128-015-1544-2
Zahedifar, M., Dehghani, S., Moosavi, A. A., and Gavili, E. (2017). Temporal variation of total and DTPA-extractable heavy metal contents as influenced by sewage sludge and perlite in a calcareous soil. Archives Agron. Soil Sci. 63 (1), 136–149. doi:10.1080/03650340.2016.1193164
Zhang, J., Gao, Y., Yang, N., Dai, E., Yang, M., Wang, Z., et al. (2021). Ecological risk and source analysis of soil heavy metals pollution in the river irrigation area from Baoji, China. Plos one 16 (8), e0253294. doi:10.1371/journal.pone.0253294
Zhao, F. J., Ma, Y., Zhu, Y. G., Tang, Z., and McGrath, S. P. (2015). Soil contamination in China: Current status and mitigation strategies. Environ. Sci. Technol. 49 (2), 750–759. doi:10.1021/es5047099
Zheng, S., Wang, Q., Yuan, Y., and Sun, W. (2020). Human health risk assessment of heavy metals in soil and food crops in the Pearl River Delta urban agglomeration of China. Food Chem. 316, 126213. doi:10.1016/j.foodchem.2020.126213
Zhu, H. D., Wang, J. X., and Chen, R. S. (2000). Study on element concentration and dietary intake in foods among Chinese residents (in Chinese with English abstract) [J]. Chin. J. Radiat. Med. Prot. 20 (6), 378–384. doi:10.3760/cma.j.issn.0254-5098.2000.06.002
Zhuang, P., McBride, M. B., Xia, H., Li, N., and Li, Z. (2009). Health risk from heavy metals via consumption of food crops in the vicinity of Dabaoshan mine, South China. Sci. total Environ. 407 (5), 1551–1561. doi:10.1016/j.scitotenv.2008.10.061
Keywords: heavy metals, food crops, health risk, daily intake, Chengdu Plain
Citation: Liu Q, Li X and He L (2022) Health risk assessment of heavy metals in soils and food crops from a coexist area of heavily industrialized and intensively cropping in the Chengdu Plain, Sichuan, China. Front. Chem. 10:988587. doi: 10.3389/fchem.2022.988587
Received: 07 July 2022; Accepted: 26 July 2022;
Published: 01 September 2022.
Edited by:
Hu Li, Guizhou University, ChinaReviewed by:
Wei Wang, Hunan Institute of Science and Technology, ChinaDongmei Qi, Zhejiang University, China
Yun Yang, Hanjiang Normal University, China
Copyright © 2022 Liu, Li and He. This is an open-access article distributed under the terms of the Creative Commons Attribution License (CC BY). The use, distribution or reproduction in other forums is permitted, provided the original author(s) and the copyright owner(s) are credited and that the original publication in this journal is cited, in accordance with accepted academic practice. No use, distribution or reproduction is permitted which does not comply with these terms.
*Correspondence: Lei He, aGVsZWkwMzA0QGdtYWlsLmNvbQ==