- 1State Key Laboratory of Safety and Control for Chemicals, SINOPEC Research Institute of Safety Engineering Co., Ltd., Qingdao, China
- 2State Key Laboratory of Heavy Oil Processing, China University of Petroleum (East China), Qingdao, China
A series of alkali metal (Li, Na, and K)-modified Pd catalysts and Pd/Al2O3 were prepared and used to remove oxygen in a propylene flow with hydrogen’s existence. The results showed that the alkali metals could enhance the performance of the Pd catalysts and the effect followed the order of K > Na > Li. X-Ray diffraction (XRD), N2-physisorption, transmission electron microscopy (TEM), hydrogen temperature programmed reduction (H2-TPR), and X-ray photoelectron spectroscopy (XPS) were carried out to investigate the alkali metal-modified Pd catalysts and the promotional effect mechanism was explained. The results showed that alkali metal modification increased the electron density of Pd atoms to induce the negatively charged Pd species, which could enhance the adsorption of oxygen while weakening the adsorption of propylene, and then enhance the performance of the modified catalysts for oxygen removal from unsaturated hydrocarbon. The Pd-K/A catalyst performed the best on both oxygen removal and propylene hydrogenation inhibition.
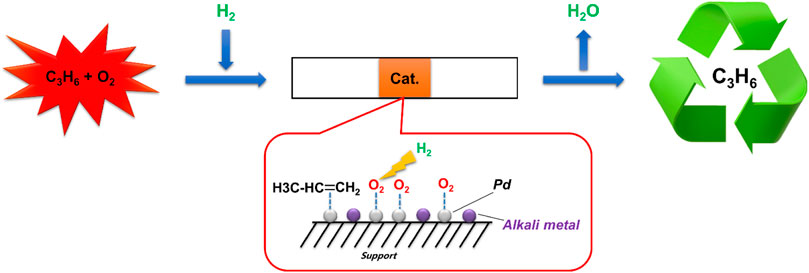
GRAPHICAL ABSTRACT | To recycle the propylene safely from a gas mixture consisting of propylene and oxygen, alkali metal-modified Pd catalysts were used to remove oxygen from the gas with the help of hydrogen.
Introduction
Propylene oxide (PO) is an important petrochemical product with a great market in the chemical industry. Hydrogen-peroxide-to-propylene-oxide technology (HPPO) for the production of propylene oxide has great advantages in environmental protection, which has attracted great attention in recent years (Climent et al., 2011; Lin et al., 2016; Li et al., 2022). The tail gas of the HPPO process contains large amount of oxygen produced by hydrogen peroxide decomposition, which makes the tail gas too dangerous to be separated and recycled (Movileanu et al., 2012; Razus et al., 2009; Razus et al., 2007). Some methods have been reported for solving this problem by removing the oxygen via catalytic reactions. Joaquim Henrique Teles et al. reported a catalyst consisting of LiCl and TiO2, which could catalyze the oxidative dehydrogenation reaction between propane and oxygen in the HPPO exhaust at 585°C to remove oxygen (Teles et al., 2004). Lv et al. reported a Mn-contained adsorbent for oxygen removal, ethylene or propylene with little oxygen could be purified at 200°C by the adsorbent (Lv et al., 2005). Schindler et al. developed a Pt-Sn/α-Al2O3 catalyst, by whose effect oxygen and hydrogen could react to produce water at a temperature between 250–300°C, while a small amount of propylene might react with hydrogen (Schindler et al., 2011). Although there have been many technologies for oxygen removal in gas mixtures, most of the technologies were used for inert gas purification (Kim et al., 2022). In addition, disadvantages like high reaction temperature, frequent regeneration, or low performance (or selectivity) of the oxygen-removal reaction restricted the application of these technologies to the removal of oxygen in the tail gas of the HPPO process. In the HPPO tail gas oxygen-removal reaction, the reaction between oxygen and hydrogen should be promoted, while propylene hydrogenation should be inhibited. Among several catalysts, the Pd catalyst usually showed good performance in hydrogen oxidation and selective hydrogenation. Although Pd is a good metal for catalytic reaction, the selectivity is not perfect. To improve its selectivity, people use many methods. The adsorption and desorption abilities to the olefine of Pd are important; Pd-containing bimetallic catalysts were usually used to solve the problem (Ravanchi et al., 2018; Shi et al., 2022). Pd/Al2O3-Mg and Pd/CaCO3 catalysts were reported having good selectivity in the olefine purification reaction because of the weaker Lewis acid centers on the surface (Cordoba et al., 2019). It indicates that alkali earth metals can weaken the acid centers in Pd catalysts, which helps to improve the selectivity of the catalysts. In hydrogen catalytic purification area, Pd has been widely used too. Pd has a very strong affinity for O2 and H2, the dissociative adsorption of H2 and O2 molecules on the surface of Pd catalysts occurs with little or no activation energy barrier (Adams and Chen, 2011). Pd-substituted Co3O4-ZrO2 was used for catalytic hydrogen combustion, and Pd improved H2 and O2 activation, which reduced the temperature of the reaction; the activation energy of the Pd catalyst was decreased by more than 2-fold (Singh et al., 2017). If the advantage of the Pd catalyst on hydrogen oxidation and selective hydrogenation can be combined, it should be a good choice for oxygen removal from unsaturated hydrocarbon (Kim et al., 2022).
In this work, a Pd catalyst was prepared, and it was used to remove oxygen (>2 vol %) in a propylene (>90 vol %) flow with hydrogen’s existence; the oxygen removal efficiency (ORE) and propane content in the product were investigated. Furthermore, alkali metals (Li, Na, and K) were used to modify the Pd catalyst; several characterizations were carried out to study the influence of the alkali metals on Pd species in the catalysts. It was found that oxygen could be removed from the propylene flow by Pd catalysts, alkali metals could enhance the performance of the Pd catalyst, and the effect followed the order of K > Na > Li. The characterization results explained the mechanism of the influence of alkali metals to the Pd catalyst.
Experimental section
Catalyst preparation
Pd/Al2O3 and alkali metal-modified Pd/Al2O3 catalysts were prepared by wetness impregnation. Aqueous solutions of PdCl2 (Shanghai Aladdin Biochemical Technology Co., Ltd.) and alkali metal nitrate of Li, Na, or K (Sinopharm Chemical Reagent Beijing Co., Ltd.) were added to Al2O3 (Sinopec Catalyst Co., Ltd.) support. After impregnation, the samples were dried at 100°C for 10 h and then calcined at 500°C for 4 h. The obtained catalysts were denoted as Pd/A, Pd-Li/A, Pd-Na/A, and Pd-K/A. The content of Pd was 0.3 wt %, and alkali metals were added with the same alkali metal/Pd mole ratio.
Catalyst characterization
Powder X-ray diffraction (XRD) was carried out on a Rigaku Ultima IV (Cu-Kα, λ = 0.154 nm, 3 KW). The textual characterization was obtained by N2-physisorption on a Micromeritics ASAP 2020 at 77 K; the data were processed by using the Brunauer–Emmett–Teller (BET) method. Hydrogen temperature-programmed reduction (H2-TPR) was carried out using a Micromeritics Autochem 2950 with 10 % H2/Ar. X-ray photoelectron spectroscopy (XPS) was carried out on a VG ESCALAB MK II spectrometer with an Al-Kα (1,486.6 eV) photon source. The morphology of the catalysts was carried out on a JEOL JEM-2100UHR transmission electron microscopy (TEM).
Catalyst test
Hydrocarbon oxygen removal tests were carried out on a fixed-bed reaction system. 1 ml catalyst was filled in a stainless steel reactor (I.D. = 6 mm). Before the test, the catalysts were pretreated in a H2 flow (15 sccm) at 150°C for 2 h. The tests were performed at 0.5 MPa and a LHSV 4,000 h−1. A gas mixture was injected into the reactor. The reaction products were separated in a separator, then the gas was analyzed using gas chromatography (GC, Agilent 7890B) with a flame ionization detector (FID) and two thermal conductivity detectors (TCD). The oxygen-removal performance was presented by oxygen removal efficiency (ORE), which was defined by the following formula:
Results and discussions
Oxygen-removal performance
In order to investigate the oxygen-removal performance of Pd/A and Pd-M/A (M = Li, Na or K) with the existence of hydrogen, a gas mixture of propylene (94 vol %), oxygen (2 vol %), and hydrogen (4 vol %) was introduced into the reactor after the catalysts were reduced. Figure 1A shows the oxygen removal efficiency (ORE) of different catalysts. The Pd/A catalyst had an ORE lower than 40 % at 80°C and achieved 94 % at 265°C. After the catalyst was modified by alkali metals, the performance of the Pd catalyst was enhanced remarkably. The Pd-Li/A had a similar performance with Pa/A between 80 and 265°C. Pd-Na/A and Pd-K/A performed better than Pd/A at the same temperature, and they achieved a 100% conversion at 265°C. Hydrogen also reacted with propylene while it reacted with oxygen, the byproduct was propane. Figure 1B shows propane content in the product gas. All catalysts had a similar trend between 80 and 265°C. Pd/A and Pd-Li/A had the same performance, while Pd-Na/A and Pd-K/A produced less propane in the reaction at the same temperature. According to the reaction results, the modification by alkali metals enhanced the performance of the Pd catalyst for the reaction of hydrogen and oxygen, while the reaction of hydrogen and propylene was restrained. The promotion effects seemed dependent on the chemical nature of the alkali metals, the order was K > Na > Li.
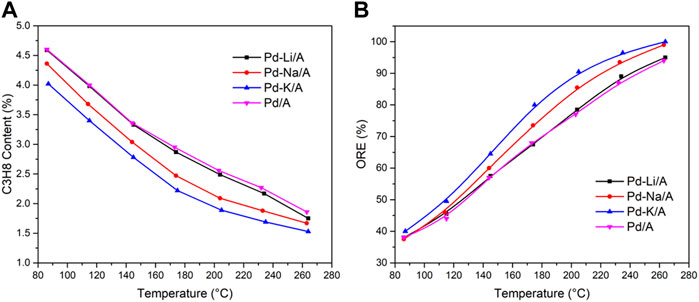
FIGURE 1. Oxygen removal efficiency (A) and content of propane in the products (B) for different catalysts.
Structural feature studies
The XRD patterns of Al2O3, Pd/A, and alkali metal-modified Pd catalysts are shown in Figure 2. The pattern of Al2O3 indicated that it was γ-Al2O3. In the patterns of Pd/A and alkali metal-modified Pd catalysts, there was no observable peak that belonged to Pd or other species, which indicated that Pd and alkali metals were highly dispersed on the supports (Peralta-Robledo et al., 2020). The pore structure information of Al2O3 support, Pd/A, and alkali metal-modified Pd catalysts are listed in Table 1. Compared with Al2O3 support, the surface area of Pd/A and Pd-M/A (M = Li, Na, K) decreased a little bit, which might be ascribed to the metal species covering the surface of the support. Figure 3 shows the TEM image of Pd/A and alkali metal-modified Pd catalysts. As shown in the images, Pd species were dispersed on the surface of the catalysts, no big block was observed. The particle size of Pd species on the surface of catalysts was statistically analyzed. The results showed that the particle size distribution of the catalysts was between 2.0 and 3.5 nm. The average particle size of Pd/A was 3.14 nm, while it was 2.67, 2.56, and 2.78 nm, respectively, over the Pd-Li/A, Pd-Na/A, and Pd-K/A. It indicated that the alkali metal modification helped the Pd species to form smaller particles, which improved the distribution of the Pd species on the surface of the catalysts.
H2-TPR studies
To study the reducibility of the Pd/A and alkali metal (Li, Na, and K)-modified catalysts, the hydrogen temperature-programmed reduction was carried out. Figure 4 shows the H2-TPR profiles of the catalysts, the differences of the profiles of the catalysts are obvious. Pd/A and Pd-Li/A presented negative peaks at 68°C, and Li-modified Pd catalysts presented small positive peaks at around 63°C; otherwise, Pd-Na/A and Pd-K/A presented big positive peaks at 76 and 72°C, respectively. The positive peaks of the profiles could be ascribed to the hydrogen consumption of the reduction of Pd oxide, while the negative peaks are ascribed to the hydrogen generation of the decomposition of the β-PdH formed during the reduction at lower temperature (Batista et al., 2001). Pd oxide is easily reduced by H2 at room temperature; Pd/A showed only a negative peak, which ascribed to the β-PdH decomposition. Positive peaks appeared in the profiles of alkali-modified Pd catalysts, although there was still a sharp negative peak in the profile of Pd-Li/A. The positive peak could be ascribed to the interaction between Pd and Li, but the interaction was too weak to affect the Pd species much. The positive peaks in the profiles of Pd-Na/A and Pd-K/A were obvious, but the negative peak disappeared. In addition to the small positive peak at 63°C, a big peak appeared at a higher temperature, which responded to the reduction of Pd oxide. These phenomena indicated that there was an interaction between the Pd species and alkali metals, which affected the reducibility of Pd oxide and the formation of β-PdH on the surface of Pd species. The intensity of the interaction followed the order of K > Na > Li. According to the rule, Pd-K/A had the least β-PdH which responded to hydrogenation of propylene, which could well coincide with the reaction results.
XPS studies
XPS was carried out to investigate the electron structure information of Pd species on the surface of the catalysts and the effect of alkali modification. The spectra of Pd 3d for Pd/A and alkali metal (Li, Na, and K)-modified Pd catalysts are displayed in Figure 5. In the curve of Pd/A, the peak at 336.9 eV could be ascribed to PdO, which was due to the oxidation in the production process (Batista et al., 2001; Li et al., 2016). With the addition of alkali metals, the peak of PdO shifted to a lower binding energy. The top of the peaks appeared at 336.7, 336.6, and 336.4 eV over Pd-Li/A, Pd-Na/A, and Pd-K/A, respectively. The results indicated that alkali metals played the role of the electron donor, who had interaction with the Pd species in the modified catalysts. The decrease of the binding energy indicated that the addition of alkali metals increased the electron density of Pd species, and the electron donation effect became stronger with the chemical nature of the alkali metals, following the order of K > Na > Li (Liotta et al., 1996a; Li et al., 2016). The binding energy of Pd-Li/A decreased the least among the modified catalysts, it could be attributed such that Li gives out the least electron for the strongest electronegativity among the alkali metals, and the interaction between Pd and Li could be weaker than other alkali metals. Pd species were the main active sites of the reaction between hydrogen and oxygen or hydrogen and propylene; the negatively charged Pd species should perform differently. Adsorption of reactant molecules on the active sites is an important step during the reaction, and the electron density of Pd species could affect the adsorption to change the reaction performance (Liotta et al., 1996b). Adsorption of propylene on the active site by π bond should be decreased for the electron density increase of the negatively charged Pd species. On the other hand, the negatively charged Pd species could enhance the adsorption of oxygen because of the electron transfer from Pd species to the anti-bonding π* orbital of oxygen molecules (Huang and Leung, 2011; Matveev et al., 2015; Xu et al., 2019). This change may promote the reaction between hydrogen and oxygen, while the reaction between hydrogen and propylene would be decreased. For the difference of interaction between Pd species and alkali metals, the activity of the modified Pd-based catalysts should follow the order of Pd-K/A > Pd-Na/A > Pd-Li/A, the results were consistent with the performance of the oxygen removal test.
Stability studies
The aforementioned comparative experiments and characterizations showed that Pd-K/A was the best item among the studied catalysts; then, a long-term experiment was carried out to investigate the stability of the Pd-K/A catalyst. The reaction was performed on a fixed-bed reactor in which 1 ml Pd-K/A catalyst was loaded. A gas mixture consisting of propylene (94 vol %), oxygen (2 vol %), and hydrogen (4 vol %) was used as model feed. After the reaction ran for 150 h, steam (about 2 mol %) was introduced into the reactor to investigate the influence of water to the oxygen removal reaction. The test was performed for 200 h at the conditions of T = 240°C, p = 0.5 MPa, and a LHSV = 4,000 h−1. When the temperature of the catalyst stabilized at 240°C, the gas product was analyzed by using gas chromatography every 2 h.
Figure 6 shows the ORE and the C3H8 content in the gas product during the long-term experiment. In the initial stage, the ORE of the reaction was above 97% at the first 24 h, and the content of C3H8 in the product was about 1.68%. The reason for the perfect performance was that the fresh Pd-K/A catalyst had a relatively high activity at the beginning, and the reaction of H2 with O2 and H2 with propylene were all promoted. After the initial stage, the activity of the Pd-K/A catalyst became stable; the ORE remained at 95–97%, while the content of C3H8 in the product remained at 1.55–1.65%. When the steam was introduced into the reaction at 150 h, the ORE showed a downward trend, the content of C3H8 in the product went down too, which indicated that the activity of the Pd-K/A catalyst was affected. In the oxygen-removal process, the product of the reaction between H2 and O2 is H2O. It means that there was always water in the reaction system. In Figure 6, the ORE of the reaction before 150 h remained above 95 % stably, but the ORE began going down while the additional water (2 % steam) was introduced into the reactor. It indicated that water could influence the activity of the Pd-K/A catalyst; water molecules might adsorb on the surface of the catalyst to cover the active site, leading to the activity falling. According the result of the stability experiment, the quantity of the water involving the reaction was important. Although water was continuously generated during the reaction, a small amount of water could not affect the activity of the Pd-based catalyst. When the additional water was introduced in the system, the amount of water molecules around the active sites increased, which resulted in the formation of a water film that covered the active site (Kim et al., 2022).
Conclusion
Pd and alkali metal-modified Pd catalysts could remove oxygen in the propylene flow with hydrogen existence by catalytic reaction between oxygen and hydrogen, and the reaction between propylene and hydrogen could be inhibited to a certain extent. The addition of alkali metals could facilitate the reducibility of Pd species and inhibit the formation of β-PdH which was responsible for propylene hydrogenation. The electron density of the Pd atom was increased by the metal–metal interaction to form negatively charged Pd species which adsorbed oxygen molecules easier than propylene molecules. The improvement of alkali metals depended on the chemical nature of the alkali metals, and the order was K > Na > Li. The characterization results of Pd-K/A indicated that the strongest interaction between Pd and K contributed to its highest ORE and selectivity. The stability test showed that the Pd-K/A catalyst could perform well for a long time; the ORE could remain at 95–97% at 240°C. Water could influence the activity of the catalyst, but the quantity of water was important. Water formed during the reaction could not affect the catalyst, but additional water might weaken the activity of the catalyst for the water molecules adsorbed on the surface covering the active site of the catalyst.
Data availability statement
The original contributions presented in the study are included in the article/Supplementary Material; further inquiries can be directed to the corresponding authors.
Author contributions
JcZ: Investigation, data curation, and writing—original draft. JJ: Resources. SW: Data curation. JgZ: Resources. CZ: Data curation. NS: Data curation. WL: Data curation. BS: Resources and writing—review and editing WX: Resources and supervision ZY: Resources and supervision YP: Resources and writing—review and editing.
Funding
The present work was supported by Funding of Applied Research Project for Postdoctoral Researchers in Qingdao.
Conflict of interest
JcZ, JJ, SW, JgZ, CZ, NS, WL, BS, WX, and ZY were employed by the Company SINOPEC Research Institute of Safety Engineering Co., Ltd.
The remaining author declares that the research was conducted in the absence of any commercial or financial relationships that could be construed as a potential conflict of interest.
Publisher’s note
All claims expressed in this article are solely those of the authors and do not necessarily represent those of their affiliated organizations, or those of the publisher, the editors, and the reviewers. Any product that may be evaluated in this article, or claim that may be made by its manufacturer, is not guaranteed or endorsed by the publisher.
References
Adams, B. D., and Chen, A. (2011). The role of palladium in a hydrogen economy. Mat. TodayKidlingt. 14 (6), 282–289. doi:10.1016/s1369-7021(11)70143-2
Batista, J., Pintar, A., Mandrino, D., Jenko, M., and Martin, V. (2001). XPS and TPR examinations of γ-alumina-supported Pd-Cu catalysts. Appl. Catal. A General 206 (1), 113–124. doi:10.1016/s0926-860x(00)00589-5
Climent, M. J., Corma, A., and Iborra, S. (2011). Heterogeneous catalysts for the one-pot synthesis of chemicals and fine chemicals. Chem. Rev. 111 (2), 1072–1133. doi:10.1021/cr1002084
Cordoba, M., Coloma-Pascual, F., Quiroga, M. E., and Lederhos, C. R. (2019). Olefin purification and selective hydrogenation of alkynes with low loaded Pd nanoparticle catalysts. Ind. Eng. Chem. Res. 58 (37), 17182–17194. doi:10.1021/acs.iecr.9b02081
Huang, H., and Leung, D. Y. C. (2011). Complete oxidation of formaldehyde at room temperature using TiO2 supported metallic Pd nanoparticles. ACS Catal. 1 (4), 348–354. doi:10.1021/cs200023p
Kim, T., Song, Y., Kang, J., Kim, S. K., and Kim, S. (2022). A review of recent advances in hydrogen purification for selective removal of oxygen: Deoxo catalysts and reactor systems. Int. J. Hydrogen Energy 47 (59), 24817–24834. doi:10.1016/j.ijhydene.2022.05.221
Li, W., Wu, G., Hu, W., Dang, J., Wang, C., Weng, X., et al. (2022). Direct propylene epoxidation with molecular oxygen over cobalt-containing zeolites. J. Am. Chem. Soc. 144 (9), 4260–4268. doi:10.1021/jacs.2c00792
Li, Y., Zhang, C., He, H., Zhang, J., and Chen, M. (2016). Influence of alkali metals on Pd/TiO2 catalysts for catalytic oxidation of formaldehyde at room temperature. Catal. Sci. Technol. 6 (7), 2289–2295. doi:10.1039/c5cy01521a
Lin, M., Xia, C., Zhu, B., Li, H., and Shu, X. (2016). Green and efficient epoxidation of propylene with hydrogen peroxide (HPPO process) catalyzed by hollow TS-1 zeolite: A 1.0kt/a pilot-scale study. Chem. Eng. J. 295, 370–375. doi:10.1016/j.cej.2016.02.072
Liotta, L. F., Deganello, G., Delichere, P., Leclercq, C., and Martin, G. A. (1996a). Localization of alkali metal ions in sodium-promoted palladium catalysts as studied by low energy ion scattering and transmission electron microscopy. J. Catal. 164 (2), 334–340. doi:10.1006/jcat.1996.0389
Liotta, L. F., Martin, G. A., and Deganello, G. (1996b). The influence of alkali metal ions in the chemisorption of CO and CO2on supported palladium catalysts: A fourier transform infrared spectroscopic study. J. Catal. 164 (2), 322–333. doi:10.1006/jcat.1996.0388
Lv, S., Qin, Y., Huang, F., and Wu, X. (2005). Manganese deoxidier and its preparation method and application. CN. Patent No. 200510116710.1. Beijing: China National Intellectual Property Administration.
Matveev, A. V., Kaichev, V. V., Saraev, A. A., Gorodetskii, V. V., Knop-Gericke, A., Bukhtiyarov, V. I., et al. (2015). Oxidation of propylene over Pd(551): Temperature hysteresis induced by carbon deposition and oxygen adsorption. Catal. Today 244, 29–35. doi:10.1016/j.cattod.2014.08.023
Movileanu, C., Gosa, V., and Razus, D. (2012). Explosion of gaseous ethylene–air mixtures in closed cylindrical vessels with central ignition. J. Hazard. Mat. 235-236, 108–115. doi:10.1016/j.jhazmat.2012.07.028
Peralta-Robledo, R. E., Santolalla-Vargas, C. E., Sanchez-Minero, F., Santes, V., Flores-Valle, S. O., and Elizalde Solis, O. (2020). Effect of the formulation of Pd/γ-Al2O3+Pd/H-ZSM-5 catalysts prepared by mechanical mixing for the thermal and catalytic hydrotreating of castor oil. Catal. Today 346, 81–86. doi:10.1016/j.cattod.2019.02.063
Ravanchi, M. T., Sahebdelfar, S., and Komeili, S. (2018). Acetylene selective hydrogenation: A technical review on catalytic aspects. Rev. Chem. Eng. 34 (2), 215–237. doi:10.1515/revce-2016-0036
Razus, D., Brinzea, V., Mitu, M., and Oancea, D. (2009). Explosion characteristics of LPG–air mixtures in closed vessels. J. Hazard. Mat. 165 (1), 1248–1252. doi:10.1016/j.jhazmat.2008.10.082
Razus, D., Movileanua, C., and Oancea, D. (2007). The rate of pressure rise of gaseous propylene–air explosions in spherical and cylindrical enclosures. J. Hazard. Mat. 139 (1), 1–8. doi:10.1016/j.jhazmat.2006.05.103
Schindler, G. P., Walsdorff, C., Koerner, R., and Goebbel, H. G. (2011). Process for producing propylene oxide. U.S. Patent No. 7863468. (Washington, DC: U.S. Patent and Trademark Office).
Shi, Y., Zhou, Y., Lou, Y., Chen, Z., Xiong, H., and Zhu, Y. (2022). Homogeneity of supported single-atom active sites boosting the selective catalytic transformations. Adv. Sci. 9, 2201520. doi:10.1002/advs.202201520
Singh, S. A., Vishwanath, K., and Madras, G. (2017). Role of hydrogen and oxygen activation over Pt and Pd-doped composites for catalytic hydrogen combustion. ACS Appl. Mat. Interfaces 9 (23), 19380–19388. doi:10.1021/acsami.6b08019
Teles, J. H., Rehfinger, A., Bassler, P., Rieber, N., Hefner, W., Wenzel, A., et al. (2004). Working up a mixture comprising alkene and oxygen. oxygen. U.S. Patent No. 6712942. (Washington, DC: U.S. Patent and Trademark Office).
Keywords: Pd catalyst, alkali metal modification, β-PDH, oxygen removal, propylene purification
Citation: Zhao J, Jiang J, Wen S, Zhang J, Zhang C, Sheng N, Liang W, Sun B, Xu W, Yang Z and Pan Y (2022) Research on alkali metal-modified Pd catalyst for oxygen removal from propylene. Front. Chem. 10:987556. doi: 10.3389/fchem.2022.987556
Received: 06 July 2022; Accepted: 25 August 2022;
Published: 16 September 2022.
Edited by:
Guigao Liu, Nanjing University of Science and Technology, ChinaCopyright © 2022 Zhao, Jiang, Wen, Zhang, Zhang, Sheng, Liang, Sun, Xu, Yang and Pan. This is an open-access article distributed under the terms of the Creative Commons Attribution License (CC BY). The use, distribution or reproduction in other forums is permitted, provided the original author(s) and the copyright owner(s) are credited and that the original publication in this journal is cited, in accordance with accepted academic practice. No use, distribution or reproduction is permitted which does not comply with these terms.
*Correspondence: Jie Jiang, SmlhbmdqLnFkYXlAc2lub3BlYy5jb20=; Wei Xu, WHV3LnFkYXlAc2lub3BlYy5jb20=