- 1College of Pharmacy, Anhui University of Chinese Medicine, Hefei, China
- 2Anhui Province Key Laboratory of Chinese Medicinal Formula, Hefei, Anhui, China
A novel series of benzenesulfonamide derivatives that selectively act on the AT2 receptor have been designed and synthesized. The binding affinity and functional activity were evaluated by radio-ligand binding analysis and cell neurite outgrowth assay, respectively. The compounds 8d, 8h, 8i, 8j, 8l, and 9h exhibited moderate selectivity and affinity for the AT2 receptor. Among them, 8j exhibited agonist activity and 8l displayed similar selectivity to the AT2 receptor with PD123,319. Molecular docking was carried out to analyze the binding mode and binding site between the compound and the AT2 receptor to provide a reference for further development.
1 Introduction
Benzenesulfonamide derivatives have a variety of biological activities, such as being anti-microbial and anti-tumor, protecting against cardiovascular disease, offering resistance to diabetes, and so on. The AT2 receptor, which is one of the subtypes of Angiotensin II receptor, is rarely expressed in normal tissue. In certain pathological conditions, the expression of the AT2 receptor was significantly up-regulated, such as myocardial infarction, vascular injury, cerebral ischemia, and so on (Carey, 2005; Juillerat-Jeanneret, 2020). A growing number of studies have suggested that AT2 receptor antagonists could alleviate peripheral neuropathic pain by blocking neuronal excitability (Hein et al., 1995).
C21/M024 is the first selective non-peptide AT2 receptor agonist (Wan et al., 2004). It was observed that a migration of the methylene imidazole substituent resulted in the compound with AT2 receptor antagonist activity (Murugaiah et al., 2012; Wallinder et al., 2019) (Figure 1). To develop an AT2 receptor antagonist with novel scaffold, a series of heterocyclic substituted benzenesulfonamide derivatives were designed based on the principle of scaffold hopping and bioelectronic isosteric (as shown in Figure 2). The binding affinity and functional activity were evaluated by the recognized assay.
2 Results and discussion
2.1 Chemistry
The boric acid intermediates 4a-4c were obtained according to the synthetic route shown in Scheme 1 (Liu et al., 2013). The triazole and pyrazole was substituted to produce 5a and 5b, respectively. Then, the target compounds (8a-8l and 9a-9h) were synthesized by Suzuki coupling, amino deprotection, and esterification (as shown in Scheme 2).
2.2 Biological evaluation
2.2.1 Binding assay
The target compounds were evaluated for AT1 receptor binding affinity by displacement of [125I]-Ang II from the AT1 receptor in rat liver membranes. The radio-iodinated AT2 receptor selective ligand [125I]-CGP42112 was used to study AT2 receptor binding affinity in HEK-293 cells (HEK293-hAT2R) (Dudley et al., 1990; Whitebread et al., 1991). All of above methods were widely accepted and used to evaluate the binding activity of AT1 and AT2 receptors. PD-123,319 is often used as a tool compound in the analysis of AT2 receptor antagonists (Blankley et al., 1991). The results are summarized in Table 1.
2.2.2 Function evaluation assay
The compounds (i.e., 8d, 8h, 9h, 8i, 8j, 8k, and 8l) with higher-affinity and which were structurally diverse were selected for further function evaluation by neurite outgrowth assay with NG108-15 cells, which is accepted as an AT2 receptor function assay (Buisson et al., 1992; Gasparo, 1996). The ratio of cell neurite outgrowth was used to evaluate, and the details are shown in the Supplementary Material.
Ang II can induce the outgrowth of cell neurite at a ratio of nearly 20% (shown in Figure 3A). When co-incubated with AT2 receptor antagonist PD-123,319, the outgrowth of Ang II induce ratio were decreased. This means the outgrowth of cell neurite might be induced by the AT2 receptor. As shown in Figures 3B–D, the outgrowth of the cell neurite was significantly less than 20% when incubated with the evaluated compounds alone, which means that the compounds inhibit the Ang II effect. The cell neurite outgrowth was slightly increased when the cells were inhibited by PD-123,319, which means that the inhibition of Ang II was induced by the AT2 receptor. Accordingly, 8i, 8k, 8d, 8l, 8h, and 9h had antagonistic activity against the AT2 receptor. The cell outgrowth ability of 8j were blocked by PD-123,319, which means that 8j exerted AT2 receptor agonistic activity (shown in Figure 3A). These results were found to be significant according to a two-way analysis of variance (ANOVA).
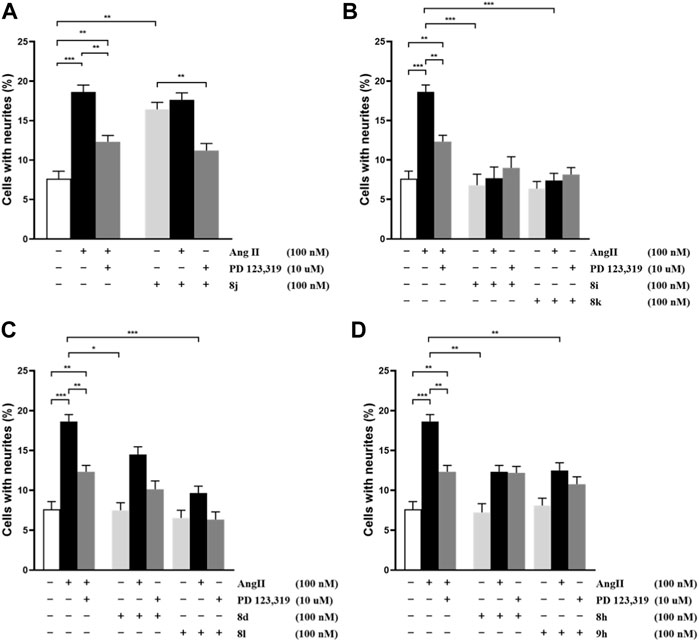
FIGURE 3. Effect of compounds 8j, 8i, 8k, 8d, 8l, 8h, and 9h on neurite outgrowth in NG108-15 cells. The number of cells with neurites was expressed as the percentage of the total number in the micrographs (at least 400 cells per well plate according to the experimental requirements). The results are significant according to two-way ANOVA: ***, p < 0.001; **, p < 0.01; *, p < 0.05; no significant difference is not shown.
2.3 Molecular docking studies
The binding affinity with the AT2 receptor and the functional activity of selected compounds (i.e., 8j, 8i, 8k, 8d, 8l, 8h, and 9h) are summarized in Table 2. The pyrazole group may provide an opportunity for binding to the AT1 receptor. Minor changes of the sulfonamide substituents of ligands not only affect the selectivity between compounds and receptors but also affect the functional activity of compounds.
To better acquire comprehension of the relationship of structure modification and affinity and activity, and to elucidate the key binding sites where compounds retain antagonistic activity, molecular docking studies were implemented by utilizing SYBYL software (Porrello et al., 2009; Sallander et al., 2016; Zhang et al., 2017; Connolly et al., 2019). As shown in Figures 4B,D, the disappearance of hydrogen bond interaction between sulfonamide side chain and receptor protein not only reduced the affinity between the compound and the AT2 receptor by 100 times but also changed the functional activity from antagonist to agonist. As shown in Figures 4A,D, when the isopropoxy side chain of the sulfonamide group of 8l is replaced with n-butyl (compound 8i), the n-butyl of the sulfonamide group will competitively extend to the hydrophobic pocket.
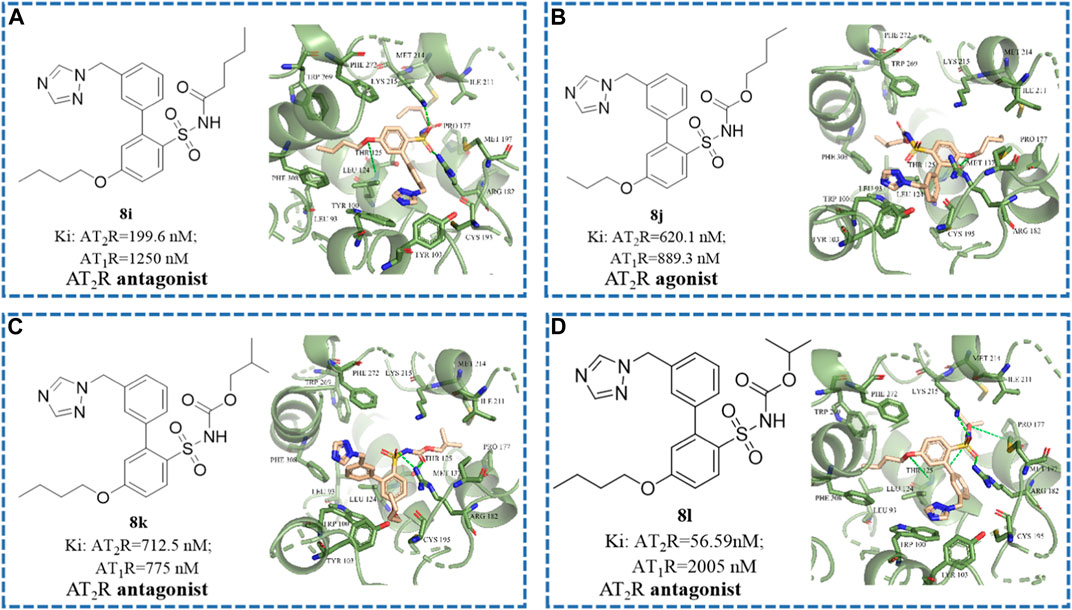
FIGURE 4. The structural formula and docking results of compounds (A) 8i, (B) 8j, (C) 8k, and (D) 8l. The green dotted line indicates hydrogen bond interaction.
We speculated that the Thr 125, Lys 215, Arg 182, and Met 137 residues of the AT2 receptor may be the key binding sites for binding affinity. The additional hydrogen bond formed by residues Lys 215, Arg 182, and Pro 177 with the alkyl chain on the sulfonamide group may be the key binding sites for the antagonistic activity of the compound.
3 Discussion
PD-123,319 is the classical AT2 receptor antagonist. Why then did we design a novel series compound with a benzenesulfonamide structure? As shown in Figure 5, an AT2 receptor antagonist with the tetrahydroisoquinoline structure, especially EMA401, has been reported to exhibit analgesic efficacy in animal models, and finished the phase II clinical trial for neuropathic pain with satisfactory results. However, more recent trials were discontinued due to drug toxicity after most patients had completed enrollment (Smith et al., 2013; Rice et al., 2014; Smith and Muralidharan, 2015; Smith et al., 2016), which indicated that EMA401 was ineffective. Therefore, we speculated that the tetrahydroisoquinoline structure may have had some “off-target” effects or pharmacokinetic problems. Consequently, we focused on the benzenesulfonamide scaffold.
As shown in Figure 6, both 8l and PD-123,319 can bind to the receptor protein through hydrophobic bonds with amino acid residues, such as Thr 125, Lys 215, Met 128, Tyr 103, and Trp 100, and occupy the cavity of the receptor protein. Furthermore, 81 (Ki: AT2R = 56.59 nM) and PD-123,319 [Ki: AT2R = 34 nM (Blankley et al., 1991)] have almost familiar binding affinity to the AT2 receptor. Therefore, the formation of these hydrophobic bonds is considered to be an important molecular basis for compounds to maintain high affinity for AT2 receptors.
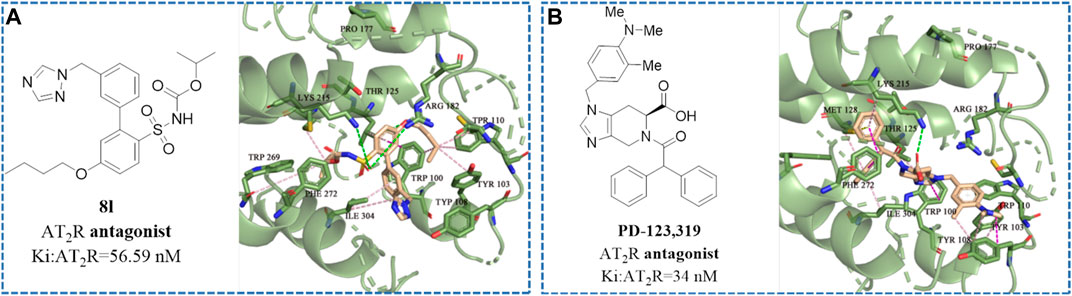
FIGURE 6. The structural formula and docking results of (A) compound 8l and (B) PD-123,319 (Blankley et al., 1991) (Ki: AT2R = 34 nM). The green dotted line indicates hydrogen bond interaction. The purple dotted line indicates hydrophobic interaction.
4 Conclusion
A series of novel AT2 receptor selective compounds with benzenesulfonamide scaffolds have been synthesized and evaluated. Among them, 8j with agonist activity and compounds 8i, 8k, 8d, 8l, and 8h exhibited moderate higher selectivity and antagonist activity. The structure activity relationship and the key binding site of the AT2 receptor were discussed. These results may provide potential compounds and references for further development.
5 Experimental section
5.1 Chemistry
Starting material and solvents were purchased from commercial sources. Reactions were monitored by thin-layer chromatography (TLC) using precoated silica gel plates (silica gel GF/UV 254), and spots were visualized under UV light (254 nm). Melting points (uncorrected) were determined on a Mel-TEMP II melting point apparatus and are uncorrected. 1H NMR and 13C NMR spectra were recorded with a Bruker Avance 300 MHz spectrometer at 300 K, using TMS as an internal standard. MS spectra or high-resolution mass spectra (HRMS) were recorded on a Shimadzu GC-MS 2050 (ESI) or an Agilent 1946AMSD (ESI) Mass Spectrum. MS spectra or high-resolution mass Column chromatography was performed with silica gel (200e300 mesh). Chemical shifts were reported on the d scale and J values were given in Hz. The synthesis of intermediates 4a-4c is shown in the Supplementary Material.
5.1.1 General procedure A: Synthesis of intermediates (5a-5b)
+To a 250 ml round-bottom flask was added potassium carbonate (4.98 g, 36.01 mmol) dissolved in acetone (100 ml). The base was activated at room temperature by stirring for 10 min. Afterward, 3-bromobenzyl bromide (6 g, 24.01 mmol), 1,2,4-triazole or pyrazole (36.01 mmol), and potassium iodide (797.03 mg, 4.80 mmol) were added to a round-bottom flask and stirred 15 h at 55°C. The reaction was diluted with water and ethyl acetate. The layers were separated, and the organic layer was washed twice with saturated NaCl solution and dried with Na2SO4. The organic layer was concentrated to give 5a (Yellow oil) or 5b (Yellow oil).
5.1.2 General procedure B: Synthesis of intermediates (6a-6f)
Compounds 5a (2.89 g, 12.13 mmol), 4a (3.80 g, 12.13 mmol), Pd(OAc)2 (54.48 mg, 242.64 umol), PPh3 (254.57 mg, 970.57 umol), toluene (30 ml), ethanol (20 ml), and NaOH (1.6 M, 15 ml) were added to a 250 ml round-bottom flask and stirred for 5 h at 90°C. The reaction was extracted with ethyl acetate. The organic layers were combined, washed with saturated NaCl solution, dried over MgSO4, and concentrated in vacuo to give crude product. The residue was purified on silica gel with PE/EA (5:1) as eluent to get 6a. Yellow oil (3.20 g, 61.83%).
Compounds 6b-6f were prepared by the same synthetic method as 6a.
5.1.3 General procedure C: Synthesis of intermediates (7a-7f)
To a 100 ml round-bottom flask was added 6a (1.80 g, 4.22 mmol). Then, trifluoroacetic acid (6.92 ml, 92.83 mmol) was added slowly to the solution in an ice bath and the mixture was stirred under N2 atmosphere for 12 h at room temperature. The reaction mixture was evaporated and most TFA was removed, leaving oil which was dissolved in ethyl acetate and washed with water and saturated NaCl solution. The crude product was achieved after drying over anhydrous Na2SO4 and evaporating under reduced pressure. Using silica gel column chromatography (PE/EA as eluent), the residue was purified to obtain 7a. White solid (1.0 g, 63.97%). 1H NMR (300 MHz, Chloroform-d) δ 8.20 (s, 1H, triazole-H), 8.05 (d, J = 8.1 Hz, 1H, triazole-H), 7.94 (s, 1H, Ar-H), 7.50 (d, J = 8.3 Hz, 3H, Ar-H), 7.34 (d, J = 6.4 Hz, 1H, Ar-H), 7.30 (d, J = 8.2 Hz, 1H, Ar-H), 7.13 (d, J = 1.4 Hz, 1H, Ar-H), 5.41 (s, 2H, -CH2), 4.74 (s, 2H, -NH2), 2.58 (d, J = 7.2 Hz, 2H, -CH2), 1.94 (m, 1H, -CH), 0.96 (d, J = 6.6 Hz, 6H, -CH3). 13C NMR (75 MHz, CDCl3) δ 152.25, 146.72, 140.18, 139.24, 138.27, 134.43, 132.71, 129.69, 129.52, 129.01, 128.57, 127.84, 53.30, 44.96, 30.07, 22.34. HR-MS: [M + H]+: 371.1542 C19H22N4O2S require 371.1542.
Compounds 7b-7f were prepared by the same synthetic method as 7a.
5.1.4 General procedure D: Synthesis of target compounds (8a-8l, 9a-9h)
N-((3'-((1H-1,2,4-triazol-1-yl)methyl)-5-isobutyl-[1,1′-biphenyl]-2-yl)sulfonyl)pentanamide (8a).
Compound 7a (200 mg, 539.85 umol) was dissolved in DCM (5 ml), followed by triethylamine (375.20 mmL, 2.70 mmol) and valeryl chloride (127.63 mmL, 1.08 mmol) added on an ice bath. The reaction mixture was stirred for 4 h at room temperature under N2 atmosphere. The reaction was extracted with ethyl acetate. The organic layers were combined, washed with saturated NaCl solution, dried over MgSO4, and concentrated in vacuo. The crude product was purified by silica gel column chromatography using CH2Cl2/MeOH as an eluent to give 8a. White solid (146 mg, 59.49%). m.p. 138.4–139.7°C. 1H NMR (300 MHz, Chloroform-d) δ 9.88 (s, 1H, -NH), 8.26 (d, J = 8.2 Hz, 1H, Ar-H), 8.22 (s, 1H, triazole-H), 7.96 (s, 1H, triazole-H), 7.63 (s, 1H, Ar-H), 7.49-7.27 (m, 4H, Ar-H), 7.15 (s, 1H, Ar-H), 5.34 (s, 2H, -CH2), 2.59 (d, J = 7.0 Hz, 2H, -CH2), 1.97 (t, J = 6.9 Hz, 3H, -CH2, -CH), 1.46 (m, 2H, -CH2), 1.31-1.20 (m, 2H, -CH2), 0.96 (d, J = 6.5 Hz, 6H, -CH3), 0.85 (t, J = 7.2 Hz, 3H, -CH3). 13C NMR (75 MHz, CDCl3) δ 171.15, 147.97, 140.02, 139.50, 134.96, 133.63, 132.71, 130.93, 130.32, 129.59, 128.99, 128.93, 127.79, 53.50, 45.09, 35.46, 30.02, 26.04, 22.39, 22.08, 13.72. ESI-MS: [M + H]+: 455.2107 C24H31N4O3S require 455.2111.
((3'-((1H-1,2,4-triazol-1-yl)methyl)-5-isobutyl-[1,1′-biphenyl]-2-yl)sulfonyl)carbamae (8b).
Compound 8b was prepared as described for 8a, replacing valeryl chloride with butyl chloroformate. Yield: 125.60 mg (49.44%). m.p. 148.1-149.3°C; 1H NMR (400 MHz, DMSO-d6) δ 11.47 (s, 1H, -NH), 8.60 (s, 1H, triazole-H), 8.00 (s, 1H, triazole-H), 7.97 (d, J = 8.2 Hz, 1H, Ar-H), 7.41 (dd, J = 14.5, 7.8 Hz, 2H, Ar-H), 7.29 (d, J = 7.7 Hz, 1H, Ar-H), 7.23 (d, J = 7.9 Hz, 2H, Ar-H), 7.12-7.09 (m, 1H, Ar-H), 5.46 (s, 2H, -CH2), 3.93 (t, J = 6.5 Hz, 2H, -CH2), 2.56 (d, J = 7.1 Hz, 2H, -CH2), 1.89 (m, 1H, -CH), 1.39 (m, 2H, -CH2), 1.15 (m, 2H, -CH2), 0.88 (d, J = 6.6 Hz, 6H, -CH3), 0.81 (t, J = 7.4 Hz, 3H, -CH3). 13C NMR (101 MHz, DMSO) δ 152.11, 151.32, 147.46, 144.78, 140.50, 139.58, 135.93, 135.43, 133.49, 130.17, 128.94, 128.85, 128.66, 128.41, 127.62, 65.88, 52.60, 44.31, 30.49, 29.87, 22.54, 18.73, 13.89. ESI-MS: [M + H]+: 471.2055 C24H31N4O4S require 471.2061.
Isobutyl((3'-((1H-1,2,4-triazol-1-yl)methyl)-5-isobutyl-[1,1′-biphenyl]-2-yl)sulfonyl)carbamate (8c).
Compound 8c was prepared as described for 8a, replacing valeryl chloride with isobutyl chloroformate. Yield: 111.00 mg (45.23%). M.p. 145.3-146.2°C; 1H NMR (300 MHz, Chloroform-d) δ 9.62 (s, 1H, -NH), 8.24 (d, J = 8.2 Hz, 1H, triazole-H), 8.17 (s, 1H, triazole-H), 7.95 (s, 1H, Ar-H), 7.56 (s, 1H, Ar-H), 7.47-7.28 (m, 4H, Ar-H), 7.16 (d, J = 1.4 Hz, 1H, Ar-H), 5.31 (s, 2H, -CH2), 3.83 (d, J = 6.5 Hz, 2H, -CH2), 2.60 (d, J = 7.1 Hz, 2H, -CH2), 1.96 (m, 1H, -CH), 1.81 (m, 1H, -CH), 0.97 (d, J = 6.6 Hz, 6H, -CH3), 0.81 (d, J = 6.7 Hz, 6H, -CH3). 13C NMR (75 MHz, Chloroform-d) δ 152.03, 150.85, 147.93, 143.22, 139.99, 139.79, 134.89, 133.64, 132.71, 130.96, 130.03, 129.38, 128.93, 128.70, 127.74, 72.58, 53.43, 45.07, 30.05, 27.61, 22.36, 18.76. ESI-MS: [M + H]+: 471.2043 C24H31N4O4S require 471.2061.
Isopropyl((3’-((1H-1,2,4-triazol-1-yl)methyl)-5-isobutyl-[1,1′-biphenyl]-2-yl)sulfonyl)carbamate (8d).
`Compound 8d was prepared as described for 8a, replacing valeryl chloride with isopropyl chloroformate. Yield: 145.00 mg (57.08%). m.p. 150.9-151.7°C; 1H NMR (400 MHz, DMSO-d6) δ 11.34 (s, 1H, -NH), 8.60 (s, 1H, triazole-H), 7.98 (d, J = 9.2 Hz, 2H, triazole-H, Ar-H), 7.47-7.37 (m, 2H, Ar-H), 7.29 (d, J = 7.8 Hz, 1H, Ar-H), 7.26∼7.20 (m, 2H, Ar-H), 7.12 (d, J = 15.9 Hz, 1H, Ar-H), 5.46 (s, 2H, -CH2), 4.69 (m, 1H, -CH), 2.56 (d, J = 7.1 Hz, 2H, -CH2), 1.89 (m, 1H, -CH), 1.05 (d, J = 6.2 Hz, 6H, -CH3), 0.88 (d, J = 6.6 Hz, 6H, -CH3). 13C NMR (101 MHz, DMSO-d6) δ 152.08, 150.74, 147.39, 144.78, 140.47, 139.53, 135.84, 135.55, 133.45, 130.30, 129.00, 128.97, 128.76, 128.65, 127.62, 70.13, 52.64, 44.31, 29.87, 22.50, 21.83. ESI-MS: [M + H]+: 457.1886 C23H29N4O4S require 457.1904.
N-((3'-((1H-1,2,4-triazol-1-yl)methyl)-5-butyl-[1,1′-biphenyl]-2-yl)sulfonyl)pentanamide (8e).
Yield: 153.00 mg (43.29%). m.p. 141.8–142.4°C; 1H NMR (400 MHz, DMSO-d6) δ 11.44 (s, 1H, -NH), 8.63 (s, 1H, triazole-H), 8.02-7.97 (m, 2H, triazole-H, Ar-H), 7.45 (m, 1H, Ar-H), 7.40 (d, J = 7.6 Hz, 1H, Ar-H), 7.28 (m, 3H, Ar-H), 7.11 (d, J = 1.6 Hz, 1H, Ar-H), 5.46 (s, 2H, -CH2), 2.73-2.64 (m, 2H, -CH2), 1.98 (t, J = 7.4 Hz, 2H, -CH2), 1.64-1.53 (m, 2H, -CH2), 1.38-1.32 (m, 2H, -CH2), 1.30 (m, 2H, -CH2), 1.17 (m, 2H, -CH2), 0.90 (t, J = 7.3 Hz, 3H, -CH3), 0.81 (t, J = 7.3 Hz, 3H, -CH3). 13C NMR (101 MHz, DMSO) δ 171.74, 152.16, 148.64, 144.78, 140.55, 139.68, 136.01, 135.39, 132.77, 130.64, 129.15, 129.02, 128.61, 128.14, 127.66, 52.57, 35.17, 34.82, 33.01, 26.29, 22.28, 21.96, 14.18, 14.08. ESI-MS: [M + H]+: 455.2186 C24H31N4O3S require 455.2111.
Butyl((3'-((1H-1,2,4-triazol-1-yl)methyl)-5-butyl-[1,1′-biphenyl]-2-yl)sulfonyl)carbamate (8f).
Yield: 116.00 mg (50.34%). m.p. 150.1-151.4°C; 1H NMR (400 MHz, DMSO-d6) δ 11.46 (s, 1H, -NH), 8.60 (s, 1H, triazole-H), 8.03∼7.99 (m, 1H, triazole-H), 7.97 (dd, J = 8.3, 1.6 Hz, 1H, Ar-H), 7.46 (d, J = 8.3 Hz, 1H, Ar-H), 7.41 (t, J = 7.5 Hz, 1H, Ar-H), 7.29 (d, J = 7.7 Hz, 1H, Ar-H), 7.23 (d, J = 7.6 Hz, 2H, Ar-H), 7.14 (s, 1H, Ar-H), 5.46 (s, 2H, -CH2), 3.94 (t, J = 6.4 Hz, 2H, -CH2), 2.68 (t, J = 7.6 Hz, 2H, -CH2), 1.59 (m, 2H, -CH2), 1.45-1.37 (m, 2H, -CH2), 1.36-1.28 (m, 2H, -CH2), 1.16 (m, 2H, -CH2), 0.93∼0.87 (m, 3H, CH3), 0.86∼0.76 (m, 3H, -CH3). 13C NMR (101 MHz, DMSO) δ 152.10, 151.36, 148.65, 144.77, 140.65, 139.60, 135.88, 135.39, 132.84, 130.32, 128.95, 128.93, 128.63, 128.17, 127.61, 65.85, 52.62, 34.80, 33.03, 30.50, 22.25, 18.73, 14.18, 13.89. ESI-MS: [M + H]+: 472.2199 C24H31N4O4S require 471.2061.
Isobutyl((3'-((1H-1,2,4-triazol-1-yl)methyl)-5-butyl-[1,1′-biphenyl]-2-yl)sulfonyl)carbamate (8g).
Yield: 119.00 mg (55.79%). m.p. 168.4–169.1°C; 1H NMR (300 MHz, Chloroform-d) δ 8.24 (d, J = 8.2 Hz, 1H, Ar-H), 8.16 (s, 1H, triazole-H), 7.95 (s, 1H, -NH), 7.54 (s, 1H, triazole-H), 7.40 (d, J = 7.4 Hz, 2H, Ar-H), 7.33 (d, J = 13.0 Hz, 2H, Ar-H), 7.24 (d, J = 7.5 Hz, 1H, Ar-H), 7.18 (d, J = 1.6 Hz, 1H, Ar-H), 5.30 (s, 2H, -CH2), 3.83 (d, J = 6.5 Hz, 2H, -CH2), 2.81-2.66 (m, 2H, -CH2), 1.82 (m, 1H, -CH), 1.74-1.60 (m, 2H, -CH2), 1.42 (m, 2H, -CH2), 0.98 (t, J = 7.3 Hz, 3H, -CH3), 0.81 (d, J = 6.7 Hz, 6H, -CH3). 13C NMR (75 MHz, Chloroform-d) δ 152.05, 150.86, 149.12, 143.23, 140.14, 139.81, 134.77, 133.66, 132.08, 131.09, 129.97, 129.37, 128.89, 128.02, 127.69, 72.57, 53.41, 35.44, 33.06, 27.61, 22.38, 18.74, 13.89. ESI-MS: [M + H]+: 471.2061 C24H31N4O4S require 471.2061.
Isopropyl((3'-((1H-1,2,4-triazol-1-yl)methyl)-5-butyl-[1,1′-biphenyl]-2-yl)sulfonyl)carbamate (8h).
Yield: 149.00 mg (59.51%). m.p. 167.7–168.3°C; 1H NMR (300 MHz, Chloroform-d) δ 8.23 (d, J = 8.2 Hz, 1H, Ar-H), 8.14 (s, 1H, triazole-H), 7.93 (s, 1H, -NH), 7.51 (s, 1H, triazole-H), 7.40 (dd, J = 7.8, 2.6 Hz, 2H, Ar-H), 7.35 (s, 1H, Ar-H), 7.32 (d, J = 4.7 Hz, 1H, Ar-H), 7.23 (d, J = 7.4 Hz, 1H, Ar-H), 7.17 (d, J = 1.5 Hz, 1H, Ar-H), 5.31 (s, 2H, -CH2), 4.86 (m, 1H, -CH), 2.81-2.69 (m, 2H, -CH2), 1.67 (m, 2H, -CH2), 1.48-1.36 (m, 2H, -CH2), 1.15 (d, J = 6.3 Hz, 6H, -CH3), 0.97 (t, J = 7.3 Hz, 3H, -CH3). 13C NMR (75 MHz, Chloroform-d) δ 152.04, 150.28, 149.07, 143.23, 140.16, 139.78, 134.82, 133.71, 132.08, 131.19, 129.89, 129.43, 128.81, 127.92, 127.64, 70.74, 53.38, 35.44, 33.06, 22.37, 21.66, 13.89. ESI-MS: [M + H]+: 457.1910 C23H29N4O4S require 457.1904.
N-((3'-((1H-1,2,4-triazol-1-yl)methyl)-5-butoxy-[1,1′-biphenyl]-2-yl)sulfonyl)pentanamide (8i).
Yield: 124.00 mg (50.94%). m.p. 167.4–168.8°C; 1H NMR (300 MHz, Chloroform-d) δ 9.72 (s, 1H, -NH), 8.30 (d, J = 9.0 Hz, 1H, triazole-H), 8.21 (s, 1H, triazole-H), 7.96 (s, 1H, Ar-H), 7.61 (s, 1H, Ar-H), 7.43 (t, J = 7.6 Hz, 1H, Ar-H), 7.34 (d, J = 7.7 Hz, 1H, Ar-H), 7.30 (d, J = 4.3 Hz, 1H, Ar-H), 7.05 (dd, J = 9.0, 2.6 Hz, 1H, Ar-H), 6.84 (d, J = 2.6 Hz, 1H, Ar-H), 5.34 (s, 2H, -CH2), 4.07 (t, J = 6.4 Hz, 2H, -CH2), 2.01 (t, J = 7.4 Hz, 2H, -CH2), 1.83 (m, 2H, -CH2), 1.52 (m, 4H, -CH2), 1.28 (m, 2H, -CH2), 1.02 (t, J = 7.4 Hz, 3H, -CH3), 0.86 (t, J = 7.3 Hz, 3H, -CH3). 13C NMR (75 MHz, Chloroform-d) δ 171.08, 162.61, 152.18, 143.48, 141.91, 139.82, 133.63, 129.43, 128.98, 128.94, 127.92, 118.22, 113.06, 93.76, 68.30, 53.50, 35.51, 31.03, 26.07, 22.11, 19.16, 13.80, 13.74. ESI-MS: [M + H]+: 471.2044 C24H31N4O4S require 471.2061.
Butyl((3'-((1H-1,2,4-triazol-1-yl)methyl)-5-butoxy-[1,1′-biphenyl]-2-yl)sulfonyl)carbamate (8j).
Yield: 118.00 mg (51.63%). m.p. 158.9–160.4°C; 1H NMR (300 MHz, Chloroform-d) δ 9.46 (s, 1H, -NH), 8.26 (d, J = 8.9 Hz, 1H, triazole-H), 8.15 (s, 1H, triazole-H), 7.94 (s, 1H, Ar-H), 7.51 (s, 1H, Ar-H), 7.41 (t, J = 7.5 Hz, 1H, Ar-H), 7.34 (m, 1H, Ar-H), 7.31 (s, 1H, Ar-H), 7.04 (dd, J = 9.0, 2.6 Hz, 1H, Ar-H), 6.85 (d, J = 2.6 Hz, 1H, Ar-H), 5.31 (s, 2H, -CH2), 4.07 (td, J = 6.5, 2.6 Hz, 4H, -CH2), 1.89-1.77 (m, 2H, -CH2), 1.53 (m, 4H, -CH2), 1.26 (dd, J = 15.3, 7.6 Hz, 2H, -CH2), 1.02 (t, J = 7.4 Hz, 3H, -CH3), 0.90 (t, J = 7.3 Hz, 3H, -CH3). 13C NMR (75 MHz, Chloroform-d) δ 162.58, 152.04, 151.50, 150.92, 143.23, 142.43, 139.62, 133.67, 129.71, 129.23, 128.81, 127.80, 121.66, 118.11, 112.95, 68.32, 66.40, 53.39, 31.04, 30.49, 19.16, 18.81, 13.80, 13.61. ESI-MS: [M + H]+: 487.1998 C24H31N4O5S require 487.2010.
Isobutyl((3'-((1H-1,2,4-triazol-1-yl)methyl)-5-butoxy-[1,1′-biphenyl]-2-yl)sulfonyl)carbamate (8k).
Yield: 109.00 mg (42.38%). m.p. 172.3–173.1°C; 1H NMR (300 MHz, Chloroform-d) δ 9.48 (s, 1H, -NH), 8.27 (d, J = 8.9 Hz, 1H, triazole-H), 8.15 (s, 1H, triazole-H), 7.95 (s, 1H, Ar-H), 7.53 (s, 1H, Ar-H), 7.41 (t, J = 7.5 Hz, 1H, Ar-H), 7.34 (d, J = 7.7 Hz, 1H, Ar-H), 7.31 (s, 1H, Ar-H), 7.04 (dd, J = 9.0, 2.6 Hz, 1H, Ar-H), 6.85 (d, J = 2.5 Hz, 1H, Ar-H), 5.30 (s, 2H, -CH2), 4.07 (t, J = 6.4 Hz, 2H, -CH2), 3.84 (d, J = 6.6 Hz, 2H, -CH2), 1.88-1.77 (m, 3H, -CH2, -CH), 1.53 (m, 2H, -CH2), 1.02 (t, J = 7.4 Hz, 3H, -CH3), 0.84 (d, J = 6.7 Hz, 6H, -CH3). 13C NMR (75 MHz, Chloroform-d) δ 162.58, 152.13, 150.93, 143.22, 142.40, 139.63, 133.66, 133.62, 129.81, 129.23, 128.90, 128.84, 127.82, 118.13, 112.97, 72.51, 68.32, 53.39, 31.02, 27.64, 19.15, 18.78, 13.79. ESI-MS: [M + H]+: 487.1995 C24H31N4O5S require 487.2010.
Isopropyl((3'-((1H-1,2,4-triazol-1-yl)methyl)-5-butoxy-[1,1′-biphenyl]-2-yl)sulfonyl)carbamate (8l).
Yield: 163.00 mg (61.82%). m.p. 166.7-168.1°C; 1H NMR (300 MHz, Chloroform-d) δ 9.26 (s, 1H, -NH), 8.25 (d, J = 8.9 Hz, 1H, triazole-H), 8.14 (s, 1H, triazole-H), 7.94 (s, 1H, Ar-H), 7.50 (s, 1H, Ar-H), 7.39 (d, J = 7.5 Hz, 1H, Ar-H), 7.33 (d, J = 13.1 Hz, 2H, Ar-H), 7.03 (dd, J = 9.0, 2.5 Hz, 1H, Ar-H), 6.84 (d, J = 2.5 Hz, 1H, Ar-H), 5.30 (s, 2H, -CH2), 4.87 (m, 1H, -CH), 4.07 (t, J = 6.4 Hz, 2H, -CH2), 1.82 (m, 2H, -CH2), 1.53 (m, 2H, -CH2), 1.18 (d, J = 6.2 Hz, 6H, -CH3), 1.01 (t, J = 7.4 Hz, 3H, -CH3). 13C NMR (75 MHz, Chloroform-d) δ 162.53, 152.04, 150.32, 143.20, 142.38, 139.57, 133.68, 129.72, 129.26, 128.88, 128.83, 127.79, 118.09, 116.27, 112.91, 70.70, 68.31, 53.39, 31.03, 21.72, 19.15, 13.80. ESI-MS: [M + H]+: 473.1846 C23H29N4O5S require 473.1853.
N-((3'-((1H-pyrazol-1-yl)methyl)-5-isobutyl-[1,1′-biphenyl]-2-yl)sulfonyl)pentanamide (9a).
Yield: 121.00 mg (47.96%). m.p. 125.8-126.7°C; 1H NMR (400 MHz, DMSO-d6) δ 11.46 (s, 1H, -NH), 8.00 (d, J = 8.2 Hz, 1H, Ar-H), 7.82 (d, J = 2.1 Hz, 1H, pyrazole-H), 7.48 (d, J = 1.5 Hz, 1H, Ar-H), 7.40 (m, 2H, Ar-H), 7.26-7.17 (m, 3H, Ar-H, pyrazole-H), 7.07 (d, J = 1.6 Hz, 1H, Ar-H), 6.28 (t, J = 2.0 Hz, 1H, pyrazole-H), 5.39 (s, 2H, -CH2), 2.55 (d, J = 7.1 Hz, 2H, -CH2), 1.99 (t, J = 7.4 Hz, 2H, -CH2), 1.88 (m, 1H, -CH), 1.39-1.29 (m, 2H, -CH2), 1.17 (m, 2H, -CH2), 0.88 (d, J = 6.6 Hz, 6H, -CH3), 0.81 (t, J = 7.3 Hz, 3H, -CH3). 13C NMR (101 MHz, DMSO-d6) δ 171.71, 147.38, 140.54, 139.51, 139.43, 137.50, 135.46, 133.37, 130.70, 130.52, 128.75, 128.72, 128.67, 128.48, 127.25, 105.93, 55.08, 44.34, 35.17, 29.84, 26.29, 22.55, 21.95, 14.06. ESI-MS: [M + H]+: 454.2142 C25H31N3O3S require 454.2159.
Butyl((3'-((1H-pyrazol-1-yl)methyl)-5-isobutyl-[1,1′-biphenyl]-2-yl)sulfonyl)carbamate (9b).
Yield: 120.00 mg (48.39%). m.p. 129.1-131.4°C; 1H NMR (400 MHz, DMSO-d6) δ 11.47 (s, 1H, -NH), 7.97 (d, J = 8.2 Hz, 1H, Ar-H), 7.80 (d, J = 1.8 Hz, 1H, pyrazole-H), 7.47 (d, J = 1.6 Hz, 1H, Ar-H), 7.42 (m, 1H, Ar-H), 7.37 (t, J = 7.8 Hz, 1H, Ar-H), 7.22-7.16 (m, 3H, Ar-H, pyrazole-H), 7.09 (d, J = 1.6 Hz, 1H, Ar-H), 6.27 (t, J = 2.0 Hz, 1H, pyrazole-H), 5.38 (s, 2H, -CH2), 3.93 (t, J = 6.5 Hz, 2H, -CH2), 2.56 (d, J = 7.1 Hz, 2H, -CH2), 1.89 (m, 1H, -CH2), 1.39 (m, 2H, -CH2), 1.15 (m, 2H, -CH2), 0.88 (d, J = 6.6 Hz, 6H, -CH3), 0.81 (t, J = 7.4 Hz, 3H, -CH3). 13C NMR (101 MHz, DMSO-d6) δ 151.35, 147.37, 140.67, 139.45, 139.35, 137.44, 135.50, 133.47, 130.71, 130.64, 130.21, 128.74, 128.56, 128.46, 127.17, 105.91, 65.85, 55.12, 44.34, 30.52, 29.86, 22.52, 18.74, 13.87. ESI-MS: [M + H]+: 470.2107 C25H31N3O4S require 470.2108.
Isobutyl((3'-((1H-pyrazol-1-yl)methyl)-5-isobutyl-[1,1′-biphenyl]-2-yl)sulfonyl)carbamate (9c).
Yield: 114.00 mg (45.27%). m.p. 149.6-151.3°C; 1H NMR (400 MHz, DMSO-d6) δ 11.47 (s, 1H, -NH), 7.98 (d, J = 8.2 Hz, 1H, Ar-H), 7.82-7.77 (m, 1H, pyrazole-H), 7.51-7.45 (m, 1H, Ar-H), 7.44-7.36 (m, 2H, Ar-H), 7.23-7.17 (m, 3H, pyrazole-H, Ar-H), 7.09 (s, 1H, Ar-H), 6.27 (t, J = 2.1 Hz, 1H, pyrazole-H), 5.38 (s, 2H, -CH2), 3.73 (d, J = 6.4 Hz, 2H, -CH2), 2.55 (d, J = 7.1 Hz, 2H, -CH2), 1.88 (m, 1H, -CH), 1.70 (m, 1H, -CH), 0.88 (d, J = 6.5 Hz, 6H, -CH3), 0.73 (d, J = 6.7 Hz, 6H, -CH3). 13C NMR (101 MHz, DMSO-d6) δ 151.37, 147.35, 140.63, 139.46, 139.36, 137.45, 135.59, 133.50, 130.72, 130.07, 128.79, 128.56, 128.53, 128.49, 127.16, 105.92, 71.92, 55.11, 44.31, 29.86, 27.65, 22.50, 18.96. ESI-MS: [M + H]+: 470.2104 C25H31N3O4S require 470.2108.
Isopropyl((3'-((1H-pyrazol-1-yl)methyl)-5-isobutyl-[1,1′-biphenyl]-2-yl)sulfonyl)carbamate (9d).
Yield: 132.00 mg (51.27%). m.p. 146.6-147.5°C; 1H NMR (400 MHz, DMSO-d6) δ 11.35 (s, 1H, -NH), 7.98 (d, J = 8.2 Hz, 1H, Ar-H), 7.80 (d, J = 2.1 Hz, 1H, pyrazole-H), 7.47 (d, J = 1.4 Hz, 1H, Ar-H), 7.42 (dd, J = 8.3, 1.4 Hz, 1H, Ar-H), 7.37 (t, J = 7.8 Hz, 1H, Ar-H), 7.23-7.16 (m, 3H, pyrazole-H, Ar-H), 7.09 (d, J = 1.5 Hz, 1H, Ar-H), 6.27 (t, J = 2.0 Hz, 1H, pyrazole-H), 5.38 (s, 2H, -CH2), 4.70 (t, J = 6.2 Hz, 1H, -CH), 2.56 (d, J = 7.1 Hz, 2H, -CH2), 1.88 (m, 1H, -CH), 1.06 (d, J = 6.2 Hz, 6H, -CH3), 0.88 (d, J = 6.6 Hz, 6H, -CH3). 13C NMR (101 MHz, DMSO-d6) δ 150.74, 147.35, 140.63, 139.39, 139.36, 137.42, 135.55, 133.46, 130.73, 130.32, 128.70, 128.60, 128.57, 128.48, 127.18, 105.93, 70.12, 55.13, 44.32, 29.87, 22.50, 21.85. ESI-MS: [M + H]+: 456.1945 C24H29N3O4S require 456.1952.
N- ((3'-((1H-pyrazol-1-yl)methyl)-5-butyl-[1,1′-biphenyl]-2-yl)sulfonyl)pentanamide (9e).
Yield: 149.00 mg (56.19%). m.p. 140.1-141.3°C; 1H NMR (300 MHz, DMSO-d6) δ 11.53 (s, 1H, -NH), 8.02 (d, J = 8.2 Hz, 1H, Ar-H), 7.88-7.84 (m, 1H, pyrazole-H), 7.53-7.49 (m, 1H, Ar-H), 7.47 (dd, J = 8.3, 1.4 Hz, 1H, Ar-H), 7.41 (t, J = 7.9 Hz, 1H, Ar-H), 7.29-7.20 (m, 3H, pyrazole-H, Ar-H), 7.15-7.10 (m, 1H, Ar-H), 6.30 (t, J = 2.0 Hz, 1H, pyrazole-H), 5.42 (s, 2H, -CH2), 2.70 (t, J = 7.7 Hz, 2H, -CH2), 2.02 (t, J = 7.3 Hz, 2H, -CH2), 1.60 (m, 2H, -CH2), 1.37 (m, 2H, -CH2), 1.32 (dd, J = 7.2, 3.3 Hz, 2H, -CH2), 1.18 (m, 2H, -CH2), 0.92 (t, J = 7.3 Hz, 3H, -CH3), 0.83 (t, J = 7.2 Hz, 3H, -CH3). 13C NMR (75 MHz, DMSO-d6) δ 171.78, 148.63, 140.67, 139.51, 139.46, 137.53, 135.37, 133.46, 132.76, 130.74, 128.74, 128.66, 128.50, 128.10, 127.27, 105.95, 55.06, 35.14, 34.81, 33.03, 26.27, 22.30, 21.98, 14.21, 14.11. ESI-MS: [M + H]+: 454.2144 C25H31N3O3S require 454.2159.
Butyl((3'-((1H-pyrazol-1-yl)methyl)-5-butyl-[1,1′-biphenyl]-2-yl)sulfonyl)carbamate (9f).
Yield: 134.00 mg (50.75%). m.p. 142.5-143.1°C; 1H NMR (300 MHz, DMSO-d6) δ 11.54 (s, 1H, -NH), 7.99 (d, J = 8.2 Hz, 1H, Ar-H), 7.83 (d, J = 2.1 Hz, 1H, pyrazole-H), 7.53-7.44 (m, 2H, Ar-H), 7.43-7.35 (m, 1H, Ar-H), 7.22 (d, J = 8.4 Hz, 3H, pyrazole-H, Ar-H), 7.14 (d, J = 1.3 Hz, 1H, Ar-H), 6.30 (t, J = 2.0 Hz, 1H, pyrazole-H), 5.40 (s, 2H, -CH2), 3.95 (t, J = 6.5 Hz, 2H, -CH2), 2.70 (t, J = 7.6 Hz, 2H, -CH2), 1.60 (m, 2H, -CH2), 1.43 (dd, J = 14.1, 7.4 Hz, 2H, -CH2), 1.37-1.30 (m, 2H, -CH2), 1.17 (m, 2H, -CH2), 0.92 (t, J = 7.3 Hz, 3H, -CH3), 0.83 (t, J = 7.3 Hz, 3H, -CH3). 13C NMR (75 MHz, DMSO-d6) δ 151.59, 148.58, 147.22, 140.78, 139.48, 139.39, 137.45, 135.46, 132.85, 130.76, 130.37, 130.14, 128.92, 128.55, 127.17, 105.94, 65.81, 55.09, 34.80, 33.07, 30.52, 22.26, 18.75, 14.21, 13.93. ESI-MS: [M + H]+: 470.2103 C25H31N3O4S require 470.2108.
Isobutyl((3'-((1H-pyrazol-1-yl)methyl)-5-butyl-[1,1′-biphenyl]-2-yl)sulfonyl)carbamate (9g).`
Yield: 107.00 mg (39.27%). m.p. 149.3-151.2°C; 1H NMR(400 MHz, DMSO-d6) δ 11.47 (s, 1H, -NH), 7.97 (d, J = 8.2 Hz, 1H, Ar-H), 7.84-7.76 (m, 1H, pyrazole-H), 7.45 (d, J = 11.4 Hz, 2H, Ar-H), 7.41-7.31 (m, 1H, Ar-H), 7.20 (d, J = 6.9 Hz, 3H, Ar-H, pyrazole-H), 7.11 (d, J = 9.5 Hz, 1H, Ar-H), 6.27 (s, 1H, pyrazole-H), 5.38 (s, 2H, -CH2), 3.73 (d, J = 6.4 Hz, 2H, CH2), 2.67 (t, J = 7.5 Hz, 2H, -CH2), 1.71 (m, 1H, -CH), 1.58 (m, 2H, -CH2), 1.33 (dd, J = 14.6, 7.2 Hz, 2H, -CH2), 0.89 (t, J = 7.3 Hz, 3H, -CH3), 0.74 (d, J = 6.7 Hz, 6H, -CH3). 13C NMR (101 MHz, DMSO-d6) δ 151.35, 148.62, 140.80, 139.45, 139.36, 137.42, 135.44, 132.87, 130.72, 130.26, 128.57, 128.51, 128.49, 128.15, 127.18, 105.92, 71.93, 55.11, 34.78, 33.02, 27.65, 22.19, 18.94, 14.17. ESI-MS: [M + H]+: 470.2093 C25H31N3O4S require 470.2108.
Isopropyl((3'-((1H-pyrazol-1-yl)methyl)-5-butyl-[1,1′-biphenyl]-2-yl)sulfonyl)carbamate (9h).
Yield: 114.00 mg (45.19%). m.p. 169.8-171.3°C; 1H NMR(400 MHz, DMSO-d6) δ 11.36 (s, 1H, -NH), 7.97 (d, J = 8.2 Hz, 1H, Ar-H), 7.80 (d, J = 2.1 Hz, 1H, pyrazole-H), 7.49-7.43 (m, 2H, Ar-H), 7.40-7.34 (m, 1H, Ar-H), 7.23-7.16 (m, 3H, Ar-H, pyrazole-H), 7.12 (d, J = 1.6 Hz, 1H, Ar-H), 6.27 (t, J = 2.0 Hz, 1H, pyrazole-H), 5.38 (s, 2H, -CH2), 4.70 (m, 1H, -CH), 2.73-2.64 (m, 2H, -CH2), 1.58 (m, 2H, -CH2), 1.37-1.29 (m, 2H, -CH2), 1.06 (d, J = 6.3 Hz, 6H, -CH3), 0.89 (t, J = 7.3 Hz, 3H, -CH3). 13C NMR (101 MHz, DMSO-d6) δ 150.77, 148.60, 140.78, 139.39, 139.36, 137.39, 135.45, 132.83, 130.72, 130.46, 128.62, 128.56, 128.48, 128.06, 127.19, 105.93, 70.11, 55.13, 34.78, 33.02, 22.19, 21.86, 14.16. ESI-MS: [M + H]+: 456.1937 C24H29N3O4S require 456.1952.
5.2 Radioligand binding assay
Rat liver membranes were prepared according to the method of Dudley et al (Dudley et al., 1990). After HEK-293 cells were transfected with the AT2 receptor, a lysis buffer was used to separate the cell membrane of HEK-293 cells (Grieger et al., 2016), using 27-G Resuspend. The lysis solution was mixed and centrifuged at 12,000 g for 10 min at 4°C. Details are shown in the Supplementary Material.
5.3 In vitro morphological effects studies
NG108-15 cells (China Center for Type Culture Collection CCTCC) were used to study the in vitro morphological effects. In their undifferentiated state, neuroblastoma × glioma hybrid NG108-15 cells havea rounded shape and divide actively. The cells were cultured from passage 18–25 in Dulbecco’s modified Eagle’s medium (DMEM, Gibco BRL, Thermo Fisher Technology (China) Co., Ltd, China) with 10% fetal bovine serum (FBS, Gibco), HAT supplement and 50 mg L−1 gentamycin at 37°C in 75 cm2 Nunclon Delta flasks in a humidified atmosphere of 95% air and 5% CO2, as previously described (Buisson et al., 1992). Subcultures were performed at subconfluency. Under these conditions, cells express mainly the AT2 receptor subtype (Whitebread et al., 1991). Cells were treated during 3 days, once a day (first treatment 24 h after plating), and micrographs were taken on the fourth day. The details of experimental grouping and administration method are shown in the Supplementary Material.
5.4 Molecular docking simulation
The AT2 receptor protein (PDB ID: 5UNF) was obtained from the RSCB protein bank database, the water molecules of theprotein were removed, the endogenous ligands were extracted, the C and D chains were selected, and a series of treatments such as energy optimization, hydrogenation, and side chain repair were carried out on the protein. Using SYBYL-2.1.1, the 2D structure of docking ligand is drawn by software and the energy is optimized. Using the docking suite module in SYBYL, the active pocket is automatically generated based on the endogenous ligand. The processed protein and ligand molecules are semi-flexibly docked in SFXC mode, and the endogenous ligand 8 ES is used as the template molecule to evaluate the similarity between the docked ligand and the endogenous ligand. Combined with the scoring functions, such as Crash, Ploar, and Similarity, the docking results are comprehensively analyzed to obtain the total score and the conformation of the compound with the highest score is taken as the docking result. Finally, the analysis results module in SYBYL is used to judge the action mode of compounds and receptors, and the sequence viewer module is used to determine the key amino acid residues.
5.5 Statistical analysis
Biological results are reported as means ± SE. Statistical analysis was performed by using one-way analysis of variance. p value of less than 0.05 was considered to be statistically significant.
Data availability statement
The original contributions presented in the study are included in the article/Supplementary Material; further inquiries can be directed to the corresponding author.
Ethics statement
The animal study was reviewed and approved by Ethics Committee of Anhui University of Chinese Medicine.
Author contributions
All authors listed have made a substantial, direct, and intellectual contribution to the work and approved it for publication.
Funding
This study was supported by grants from the Anhui Natural Science Foundation (1908085MH269).
Conflict of interest
The authors declare that the research was conducted in the absence of any commercial or financial relationships that could be construed as a potential conflict of interest.
Publisher’s note
All claims expressed in this article are solely those of the authors and do not necessarily represent those of their affiliated organizations, or those of the publisher, the editors, and the reviewers. Any product that may be evaluated in this article, or claim that may be made by its manufacturer, is not guaranteed or endorsed by the publisher.
Supplementary material
The Supplementary Material for this article can be found online at: https://www.frontiersin.org/articles/10.3389/fchem.2022.984717/full#supplementary-material
References
Blankley, C. J., Hodges, J. C., Klutchko, S. R., Himmelsbach, R. J., Chucholowski, A., Connolly, C. J., et al. (1991). Synthesis and structure-activity relationships of a novel series of non-peptide angiotensin II receptor binding inhibitors specific for the AT2 subtype. J. Med. Chem. 34 (11), 3248–3260. doi:10.1021/jm00115a014
Buisson, B., Bottari, S. P., Gasparo, M. D., Gallo-PayetN. , , and Payet, M. D. (1992). The angiotensin AT2 receptor modulates T-type calcium current in non-differentiated NG108-15 cells. FEBS Lett. 309 (2), 161–164. doi:10.1016/0014-5793(92)81086-2
Carey, Robert M. (2005). Update on the role of the AT2 receptor. Curr. Opin. Nephrol. Hypertens. 14, 67–71. doi:10.1097/00041552-200501000-00011
Connolly, A., Holleran, B. J., Simard, E., Baillargeon, J. P., Lavigne, P., and Leduc, R. (2019). Interplay between intracellular loop 1 and helix VIII of the angiotensin II type 2 receptor controls its activation. Biochem. Pharmacol. 168, 330–338. doi:10.1016/j.bcp.2019.07.018
Dudley, D. T., Panek, R. L., Major, T. C., Lu, G. H., Bruns, R. F., Klinkefus, B. A., et al. (1990). Subclasses of angiotensin II binding sites and their functional significance. Mol. Pharmacol. 38 (3), 370–377.
Gasparo, D. M. (1996). Angiotensin II induction of neurite outgrowth by AT2 receptors in NG108-15 cells. effect counteracted by the AT1 receptors [J]. J. Biol. Chem. 271 (37), 22729.
Grieger, J. C., Soltys, S. M., and Samulski, R. J. (2016). Production of recombinant adeno-associated virus vectors using suspension HEK293 cells and continuous harvest of vector from the culture media for GMP FIX and FLT1 clinical vector. Mol. Ther. 24 (2), 287–297. doi:10.1038/mt.2015.187
Hein, L., Barsh, G. S., Pratt, R. E., Dzau, V. J., and Kobilka, B. K. (1995). Behavioural and cardiovascular effects of disrupting the angiotensin II type-2 receptor gene in mice. Nature 377, 744–747. doi:10.1038/377744a0
Juillerat-Jeanneret, L. (2020). The other angiotensin II receptor: AT2R as a therapeutic target. J. Med. Chem. 63 (5), 1978–1995. doi:10.1021/acs.jmedchem.9b01780
Liu, J., Liu, Q., Yang, X., Xu, S., Zhang, H., Bai, R., et al. (2013). Design, synthesis, and biological evaluation of 1, 2, 4-triazole bearing 5-substituted biphenyl-2-sulfonamide derivatives as potential antihypertensive candidates. Bioorg. Med. Chem. 21, 7742–7751. doi:10.1016/j.bmc.2013.10.017
Murugaiah, A. M., Wu, X., Wallinder, C., Mahalingam, A. K., Wan, Y., Skold, C., et al. (2012). From the first selective non-peptide AT2 receptor agonist to structurally related antagonists. J. Med. Chem. 55 (5), 2265–2278. doi:10.1021/jm2015099
Porrello, E. R., Delbridge, L. M. D., and Thomas, W. G. (2009). The angiotensin II type 2 (AT2) receptor: an enigmatic seven transmembrane receptor. Front. Biosci. 14 (3), 958–972. doi:10.2741/3289
Rice, A. S. C., Dworkin, R. H., McCarthy, T. D., Anand, P., Bountra, C., McCloud, P. I., et al. (2014). EMA401, an orally administered highly selective angiotensin II type 2 receptor antagonist, as a novel treatment for postherpetic neuralgia: a randomised, double-blind, placebo-controlled phase 2 clinical trial. Lancet 383 (9929), 1637–1647. doi:10.1016/s0140-6736(13)62337-5
Sallander, J., Wallinder, C., Hallberga, A., Aqvist, J., and Gutierrez-de-Teran, H. (2016). Structural determinants of subtype selectivity and functional activity of angiotensin II receptors. Bioorg. Med. Chem. Lett. 26, 1355–1359. doi:10.1016/j.bmcl.2015.10.084
Smith, M. T., Anand, P., and Rice, A. S. C. (2016). Selective small molecule angiotensin II type 2 receptor antagonists for neuropathic pain: preclinical and clinical studies [J]. Pain 157, S33–S41. doi:10.1097/j.pain.0000000000000369
Smith, M. T., and Muralidharan, A. (2015). Targeting angiotensin II type 2 receptor pathways to treat neuropathic pain and inflammatory pain. Expert Opin. Ther. Targets 19 (1), 25–35. doi:10.1517/14728222.2014.957673
Smith, M. T., Wyse, B. D., and Edwards, S. R. (2013). Small molecule angiotensin II type 2 receptor (AT2R) antagonists as novel analgesics for neuropathic pain: comparative pharmacokinetics, radioligand binding, and efficacy in rats [J]. Pain Med. 14 (5), 692–705. doi:10.1111/pme.12063
Wallinder, C., Sköld, C., Sundholm, S., Guimond, M. O., Yahiaoui, S., Lindeberg, G., et al. (2019). High affinity rigidified AT2 receptor ligands with indane scaffolds. Medchemcomm 10 (12), 2146–2160. doi:10.1039/c9md00402e
Wan, Y., Wallinder, C., Plouffe, B., Beaudry, H., Mahalingam, A. K., Wu, X., et al. (2004). Design, synthesis, and biological evaluation of the first selective nonpeptide AT2 receptor agonist. J. Med. Chem. 47, 5995–6008. doi:10.1021/jm049715t
Whitebread, S. E., Taylor, V., Bottari, S. P., Kamber, B., and de Gasparo, M. (1991). Radioiodinated cgp 42111A: a novel high affinity and highly selective ligand for the characterization of angiotensin AT2 receptors. Biochem. Biophys. Res. Commun. 181 (3), 1365–1371. doi:10.1016/0006-291x(91)92089-3
Keywords: benzenesulfonamide derivatives, AT2 receptor, antagonist, selective, drug design
Citation: Wang D, Zhao W, Zhang Z, Zhang Y, Li J and Huang W (2022) Design, synthesis and biological evaluation of novel biphenylsulfonamide derivatives as selective AT2 receptor antagonists. Front. Chem. 10:984717. doi: 10.3389/fchem.2022.984717
Received: 02 July 2022; Accepted: 11 July 2022;
Published: 26 August 2022.
Edited by:
Kun Xu, Beijing University of Technology, ChinaReviewed by:
Dahong Li, Shenyang Pharmaceutical University, ChinaJichao Chen, Nanjing University of Chinese Medicine, China
Copyright © 2022 Wang, Zhao, Zhang, Zhang, Li and Huang. This is an open-access article distributed under the terms of the Creative Commons Attribution License (CC BY). The use, distribution or reproduction in other forums is permitted, provided the original author(s) and the copyright owner(s) are credited and that the original publication in this journal is cited, in accordance with accepted academic practice. No use, distribution or reproduction is permitted which does not comply with these terms.
*Correspondence: Yanchun Zhang, eWN6aGFuZzIwMTdAMTYzLmNvbQ==
†These authors have contributed equally to this work