- State Key Laboratory Breeding Base of Green Pesticide and Agricultural Bioengineering, Key Laboratory of Green Pesticide and Agricultural Bioengineering, Ministry of Education, Guizhou University, Guiyang, China
Pyridaben is an acaricide widely used around the world to control phytophagous mites, white flies, aphids, and thrips. It is highly toxic to nontarget organisms such as predatory mites, bees, and fishes. Therefore, the occurrence and removal of pyridaben in food and the environment are worthy of concern. This mini-review focuses on pyridaben residue levels in crops, aquatic systems, and soils, as well as the green synthesis and removal of pyridaben. During the period of 2010–2022, pyridaben was reported in monitoring studies on fruits, vegetables, herbs, bee products, aquatic systems, and soils. Vegetable and agricultural soil samples exhibited the highest detection rates and residue levels. One-pot synthesis offers a green chemistry and sustainable alternative for the synthesis of pyridaben. Among traditional home treatments, peeling is the most effective way to remove pyridaben from crops. Magnetic solid-phase extraction technology has emerged as a powerful tool for the adsorption and separation of pyridaben. Photocatalytic methods using TiO2 as a catalyst were developed as advanced oxidation processes for the degradation of pyridaben in aqueous solutions. Current gaps in pyridaben removal were proposed to provide future development directions for minimizing the exposure risk of pyridaben residues to human and nontarget organisms.
Introduction
Pyridaben (2-tert-butyl-5-(4-tert-butylbenzylthio)-4-chloropyridazin-3(2H)-one) is an acaricide containing pyridazinone moiety. It was developed by Nissan Chemical Industries Ltd. in 1980s (Hirata et al., 1995). Over the past decades, pyridaben has become one of the most widely used acaricides (Rand and Clark, 2000; Boulaid et al., 2005; Kim et al., 2015; Chen et al., 2018; Malhat et al., 2021). As many as 408 pyridaben preparations were registered in China by 2022 (Chinese Pesticide Information Network, 2022). A questionnaire in Shaanxi and Shanxi in China showed that pyridaben was one of the most frequently used pesticide in apple orchard, with a used frequency of 86.0% (Shi et al., 2020). As a nonsystematic broad-spectrum acaricide, pyridaben is mainly applied in orchards, crop fields (in particular vegetables), tea plantations, and gardens to control phytophagous mites, white flies, aphids, and thrips (Igarashi and Sakamoto, 1994; Rand and Clark, 2000; Kim et al., 2015). Pyridaben is a powerful inhibitor of glutamate-dependent mitochondrial respiration. Specifically, it inhibits the activity of NADH: ubiquinone oxidoreductase (complex I) and thus inhibits electron transport in insect systems (Hollingworth et al., 1994; Latli et al., 1998).
Pesticides are potentially hazardous to humans and ecosystems. The effects of exposure to pesticides and their transformation products on agricultural commodities consumers and nontarget organisms have been a matter of concern in recent years. In the classification of pesticides by hazard of the World Health Organization, pyridaben is classified as a moderately hazardous (Class II) pesticide (World Health Organization, 2020). The selective inhibition of complex I by pyridaben may constitute part of the pathogenic mechanism of Parkinson’s disease (Gomez et al., 2007). Exposure to pyridaben can induce embryonic loss in early pregnancy in pigs (Bae et al., 2021). Pyridaben is highly toxic to aquatic organisms, such as shrimp (Mysidopsis bahia), bluegill sunfish (Lepornis macrochirus), rainbow trout (Oncorhynchus mykiss), and zebrafish (Danio rerio) (Rand and Clark, 2000; European Food Safety Authority, 2010; Ma et al., 2021a). For nontarget arthropods such as honey bees and predatory mites, the hazard quotient values of pyridaben were significantly above the trigger values (European Food Safety Authority, 2010). The results of previous studies have demonstrated that pyridaben had no selectivity to pest mites which are harmful to crops and their natural enemies, predatory mites, which are important for biological control (Hardman et al., 2003; Cheng et al., 2021). The application of pyridaben caused the highest mortality rates on eggs, larvae, and adults of Euseius scutalis (Athias-Henriot), and negatively affected the fecundity of adult females among the frequently used acaricides (Döker and Kazak, 2020). Similarly, pyridaben was reported to exhibit high toxicity to phytoseiidae mites such as Galendromus occidentailis (Nesbitt), Neoseiulus womersleyi (Schicha), Phytoseiulus persimilis (Athias-Henriot), and Neoseiulus cucumeris (Oudemans) (Alston and Thomson 2004; Park et al., 2011; Cheng et al., 2021). The use of pyridaben should be avoided in integrated pest management programs. Considering the risks of pyridaben to humans and nontarget organisms, its occurrence and removal are worthy of concern.
Xu et al. (2016) have summarized the discovery, synthesis, analysis, pesticide resistance, toxicological mechanisms, and environmental compatibility of pyridaben. European Food Safety Authority has presented overviews on the properties, residues, environmental fate and behavior, and ecotoxicology of pyridaben, and outlined the risk assessment of pyridaben and its metabolites for environmental compartments (European Food Safety Authority, 2010, European Food Safety Authority et al., 2017). The presence and removing methods of pyridaben have not been comprehensively summarized to date. This mini-review focuses on the occurrence of pyridaben which was reported during the period of 2010–2022, as well as the green synthesis and removal of pyridaben.
Green synthesis of pyridaben
To date, four major routes for the synthesis of pyridaben have been reported (Figure 1A) (Shogo et al., 1995; Xu et al., 2016; Dang et al., 2020). Route I which involves a key furanone intermediate 3 has no practical value due to its low yield. In route II and other similar synthetic routes, the use of NaSH or NaS2 inevitably results in the formation of irritant and toxic gas H2S, which poses risks to operators and environments. Route III is the thioetherification between dichloropyridazinone 5 and p-(tert-butyl)benzyl mercaptan 2. For the synthesis of 2, thiourea 8 is an alternative of thiolation reagent NaSH. The reaction between 5, 7, and 8 can be performed in one pot, which is more convenient and efficient than stepwise reactions. Route III is a green synthetic route because it gives pyridaben in high yield and avoids the generation of toxic gas or undesired sulfide by-products. Route IV uses α-oxo ketene dithioacetal 10 instead of p-tert-butyl benzyl mercaptan 2 to condense with 5. This route avoids the use of NaSH, but high boiling point solvents such as N,N-dimethylformamide or dimethyl sulfoxide is required for the synthesis of 10.
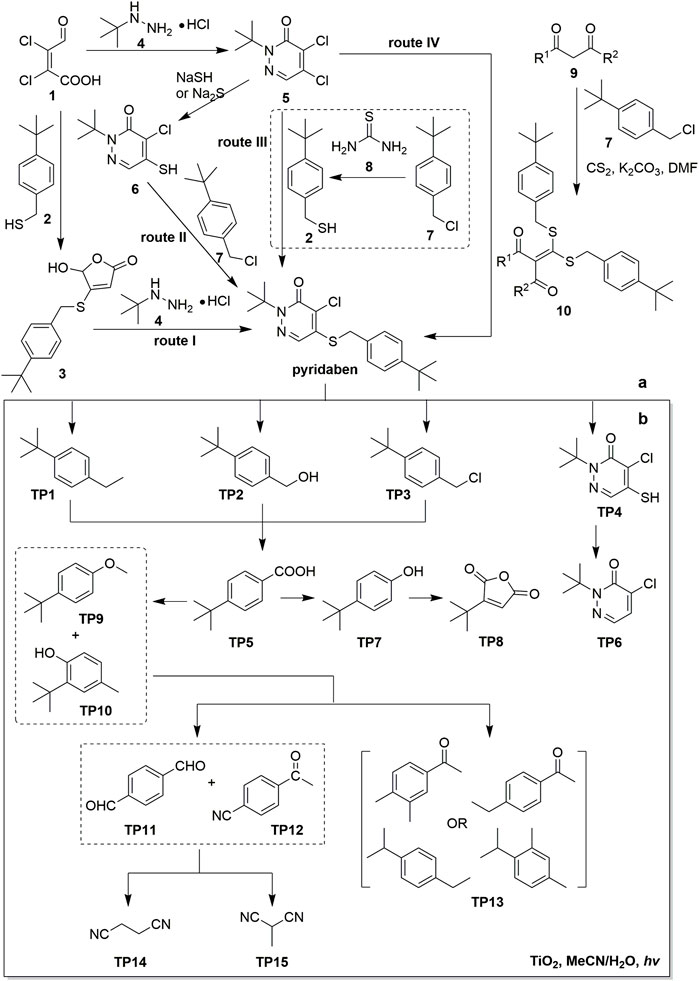
FIGURE 1. Synthesis and degradation of pyridaben. (A) Four major synthetic routes of pyridaben; (B) Degradation pathways of pyridaben in photocatalytic systems.
Occurrence of pyridaben
Pesticide residues in crops and the surrounding environment are inevitable after application. The issue of pesticide residues has aroused public awareness and government concerns. According to the criteria presented by Andreu and Picό (2012), pyridaben is susceptible to accumulating in biota due to its low water solubility (0.022 mg/L at 20°C) and high octanol-water partition coefficient (log Kow = 6.37 at 23°C) (European Food Safety Authority, 2010). As a result, pyridaben and its transformation products have the potential to accumulate in human bodies and ecosystems.
Pyridaben levels on agricultural products
Pyridaben is widely applied on fruit trees and vegetables to control red spider mites (Tetranychus cinnbarinus) and flea beetles (Phyllotreta spp). The results of the 6th Chinese total diet study indicated that pyridaben was the most frequently detected acaricides with a detection rate of 11.8% (34/288) (Xin et al., 2020). Among the 12 kinds of dietary samples, pyridaben was mainly detected in vegetable and fruit samples, with the maximum concentration of 49.55 μg/kg. A three-year (2013–2015) monitoring survey of pesticide residues on peaches in China found pyridaben residues in 10.6% of samples (Li et al., 2018). The residue values ranged from 0.01 to 0.28 mg/kg, which was lower than the maximum residual level (MRL) value of European Union (0.3 mg/kg). In another study of five local fruit cultivars in Shanghai, 1 out of 40 peach samples was positive for pyridaben, with a residue value of 7.8 μg/kg (Zhang et al., 2021). Pesticide residues in 2922 mandarin, tangerine, and orange samples from China were evaluated during the period of 2013–2018 (Li et al., 2020). Pyridaben was detected in 7.2% samples, and the residue levels were in the range of 0.005–0.63 mg/kg, which were lower than the MRL of China (2 mg/kg). The cumulative chronic dietary intakes did not pose health risks for Chinese general population and children. Ping et al. (2022) analyzed the residues of 24 pesticides in 293 greenhouse vegetable samples grown under modern urban agriculture from Beijing. Pyridaben was detected in pakchoi and tomato samples, with detection rates of 18.8% and 2%, respectively. The concentration levels of pyridaben residue in pakchoi ranged from 130.0 to 3400.2 μg/kg, which were the highest among the residues of 24 investigated pesticides; they accounted for 42.7% to the estimated daily intake. Fortunately, the hazard index was far lower than 1, which indicated low dietary risk. In another study, 19,786 vegetable samples collected from Xinjiang Uygur Autonomous Region of China during the period of 2010–2014 were analyzed (Wu et al., 2017). Pyridaben was found in 645 samples, 14 of which exceeded the MRL values set by the Chinese government. The residues of pyridaben contributed to 7.0% of the hazard indices through consuming vegetables.
In 91 snow fungus samples collected from Fujian Province, pyridaben was one of the highest frequently detected pesticide, with a detection rate of 9.9% and residues in the range of 0.011–0.046 mg/kg (Yao et al., 2020). In 151 honeysuckle samples collected from different planting bases in China, pyridaben was detected in 42.19% samples in 2017 and 45.98% samples in 2018, with residues in the range of 0.001–0.075 mg/kg and 0.001–0.080 mg/kg, respectively. The short-term, long-term, and the cumulative risks of adults to pesticide in honeysuckle were all negligible (Wu et al., 2021).
Pyridaben exhibited high toxicity to bees (LD50 = 0.535 μg/bee for acute oral toxicity and LD50 = 0.024 μg/bee for acute contact toxicity) (European Food Safety Authority, 2010; Bahreini et al., 2020). As one of the most lipophilic pesticides (log Kow = 6.37), pyridaben are easily accumulated in wax given the lipophilic nature of beeswax (Wilmart et al., 2021). In the survey from spring 2016 to autumn 2017, pyridaben was detected in 2.1% pollen samples (4/189) and 9.7% beebread samples (22/226) collected from five major beekeeping areas in China (Tong et al., 2018). The detection rate in pollen was smaller than that in beebread, but the residues in pollen were much higher than that in beebread. The mean values of pyridaben residue in pollen and beebread were 37.4 and 10.4 ng/g, respectively. In another investigation during spring of 2016 to four different types of bee wax samples collected from apiaries in Belgian, 1 out 182 of brood comb wax samples was positive for pyridaben, with a residue value equal to 0.01 mg/kg (Agrebi et al., 2020).
Pyridaben levels in environmental matrices
At the beginning of autumn, pyridaben is often used to prevent European red mite in apple orchards in China. The surface soil samples collected from an apple orchard of China in August 2017 were analyzed (Shi et al., 2020). It is stated that 100% of soil samples were positive for pyridaben, with concentrations ranging from 1.6 to 47.2 ng/g dw. In a study of the orchards and vegetable field in southern China, pyridaben was detected in collected agricultural soils with a detection rate of about 6% (Pan et al., 2019). In the soil monitoring project in five districts of southern Jordan during 2016 and 2017, pyridaben was found with high frequency and high concentration (12%, up to 5,820 μg/kg) (Kailani et al., 2021). Pyridaben is characterized by low mobility in soil and high concentration levels in soil due to it low solubility in water. (Sabzevari and Hofman, 2022). In turn, its penetration to ground water and surface water is limited. The detection frequency of pyridaben was relatively low in sediment-river-water systems, sediment-seawater system, and surface water from tropical river basins in China (Zheng et al., 2016; Tan et al., 2021; Wang et al., 2022). A three-year monitoring program of Abou Ali River located in the north of Lebanon showed that the detection frequency of pyridaben in surface water and groundwater were 18% and 20%, respectively, with a maximum concentration of 0.49 μg/L (Jabali et al., 2020).
Removal of pyridaben residues
Pyridaben residues in agricultural commodities and environmental matrices would lead to hazardous effects on human and nontarget organisms. Various methods are employed to remove pyridaben for minimizing the risk of human and ecosystems exposure to it.
Household processing
Household processing is the most common method to reduce the risk of pesticides for agricultural commodities consumers. The effects of food processing on pesticide residue levels can be evaluated by the processing factor (PF) calculated by Eq. 1 (Food and Agriculture Organization and World Health Organization, 2006):
Table 1 summarizes the effect of various household processing, including washing, blanching/boiling, frying/drying, peeling, and juicing on pyridaben residues.
Washing
In most cases, washing is the first step in household treatment for food to remove dust and pesticide on the surface. Data reported in the previous studies about the effect of washing on pyridaben residues are contradictory. Washing can substantially reduce residue levels of pyridaben in hot pepper fruits and green bean pods, with PF of 0.46–0.57 and 0.32, respectively (Aguilera et al., 2014; Kim et al., 2015). On the contrary, the application of an intensive washing cannot reduce the residue levels of pyridaben in tomatoes, apples, and cowpeas (Boulaid et al., 2005; Han et al., 2014; Huan et al., 2015). In the processing of apricots, pyridaben residues were reduced with a loss of 19.5% (Cámara et al., 2020). The efficiency of washing of a pesticide is dependent on many factors, such as the washing procedure and physicochemical properties of the pesticide and the crop epidermis. As a nonsystemic pesticide, pyridaben cannot be transported easily into the inner part of crops and thus mainly remains on the surface. It has been demonstrated that no correlation exists between water solubility of pesticides and pesticide removal by washing (Christensen et al., 2003; Chung, 2018). The decisive factor is the amount of pyridaben dissolved in the waxy layer, which is related to the nature of cuticle. In most cases, pyridaben is strongly retained by waxes of fruits and vegetables due to its high liposolubility. Therefore, washing is relatively inefficient in removing pyridaben residues.
Blanching/boiling
Blanching or boiling is a traditional method of treating vegetables to make them edible. The efficiency of blanching/boiling is affected by various factors such as cooking time, temperature, moisture loss, and pesticide degradation. Kim et al. (2015) found that blanching after washing was the most efficient method to reduce the level of pyridaben residues in pepper fruit. Compared with washing, blanching improved the removal of pyridaben by 27.6%–29.5%. The increase in the reduction ratio can be attributed by the squeezing step in blanching, the purpose of which was to remove excess water. In another study of green bean pod treatment, the PF for pyridaben was 0.67 (Aguilera et al., 2014). As reported by European Food Safety Authority et al. (2017), pyridaben is stable under the treatment of pasteurization, baking/brewing/boiling, and sterilization. The degradation or hydrolysis of pyridaben appears to not occur during the boiling process. Meanwhile, pyridaben has low volatility (1.18 × 10–6 mmHg, 20°C). The concentration effect was observed for the treatment of cowpea and tomato due to water loss, with PF of 1.6–1.11 and 2.1, respectively (Boulaid et al., 2005; Huan et al., 2015).
Frying/drying
Frying/drying food with oven or frying pan is a traditional method of food treatment. On the one hand, the pesticide residues may decrease due to thermal degradation. On the other hand, pesticide residues can be concentrated as a result of dehydration. For example, frying and stir-frying were effective to reduce pyridaben residues on cowpeas with PF = 0–0.14 (Huan et al., 2015). Compared with boiling, the temperature of frying and stir-frying was higher and the residues of pyridaben were readily transferred from cowpea into oil due to its high liposolubility. Consistently, the residue level of pyridaben in fried oil increased as frying time progressed. The results of another study indicated that frying can reduce the pyridaben residues in hot pepper fruit, but the effect was inconsiderable compared with that of washing even with increased temperature and prolonged time (Kim et al., 2015). On the contrary, drying significantly increased pyridaben residues in kiwifruits and honeysuckles (Wang et al., 2020; Xiao et al., 2022).
Peeling
Peeling is commonly the first step in treatment of fruits and certain vegetables. Pyridaben primarily deposits on the peel of the edible part of crops due to its nonsystemic characteristic. In general, peeling is the most efficient method to reduce pyridaben residues. For example, peeling significantly affected the removal of pyridaben from kiwifruits and tomatoes, with PF of 0.15 and 0.3, respectively (Boulaid et al., 2005; Wang et al., 2020). Similarly, peeling and coring significantly affected the reduction of pyridaben on apples (PF = 0.12) (Han et al., 2014). The cuticular wax of apples served as a transport barrier.
Juicing
Juicing is one of the most common household processes in fruit treatment. The removal efficiency of juicing is determined by the sort of fruit. After peeling, pyridaben residues in the flesh of kiwifruit were concentrated by juicing due to evaporation of water (Wang et al., 2020). Nevertheless, the pyridaben residues in apple can be effectively removed by juicing, with a PF of 0.22, which was attributed to the removal of apple pomace in the juicing process of apple (Han et al., 2014).
Magnetic solid–phase extraction
In the recent decades, magnetic solid–phase extraction (MSPE) has emerged as an effective tool for enrichment and separation of pesticide residues (Yu et al., 2022; Zhao et al., 2022). After adsorption, the separation of adsorbent and sample solutions can be easily achieved by applying an external magnetic field. MSPE technology combines the high efficiency of solid-phase extraction and the convenience of magnetic separation. Fe3O4/MoS2@G nanocomposite was prepared via a co-mixing solvothermal method and employed for the MSPE of pyridaben in an emulsified aqueous solution using dichloromethane as an extractant (Ma et al., 2021b). As an amphiphilic nanocomposite, Fe3O4/MoS2@G consists of hydrophilic and hydrophobic moieties. The π-π stacking interaction between Fe3O4/MoS2@G and the π electronic system of pyridaben was responsible for the effective adsorption. The extraction recovery for pyridaben was greater than 87% after reusing for 9 consecutive cycles. Zhao et al. (2022) reported a metal–organic framework material as the absorbent of pyridaben. In this study, NiO/Co@C magnetic nanocomposites were assembled as a MSPE adsorbent. NiO/Co@C contained a hollow porous structure, resulting in high pyridaben adsorption performance, which was up to 51.3 mg/g. The extraction recovery loss of pyridaben was less than 10% after 9 cycles.
Advanced oxidation processes
Advanced oxidation processes (AOPs) were also employed as a powerful way to degrade pesticides (Zeshan et al., 2022). The catalyzed efficiency of TiO2 particles in acetonitrile/water solutions with irradiation of medium pressure Mercury lamp were investigated (Zhu et al., 2004; Zhu et al., 2005). The degradation kinetics followed the Langmuir-Hinshelwood model with kinetic constant k = 4.3 × 10–5 mol/L min and equilibrium adsorption constant K = 3.1 × 103 L/mol (Zhu et al., 2004). The degradation rate of pyridaben increased with the increase of catalyst TiO2, nevertheless, an overdose of TiO2 would retard the photocatalytic reaction (Zhu et al., 2005). Direct photolysis dominated the photodegradation of pyridaben at 300 < λ ≤ 360 nm, while photocatalysis degradation by TiO2 was dominant at λ ≥ 360 nm. In TiO2 aqueous dispersions, cationic surfactant cetyltrimethyl ammonium bromide can help pyridaben coadsorb on TiO2 particles surface and facilitate the photocatalytic degradation (Zhu et al., 2007). A low dose of H2O2 can also improve the photocatalytic rate by removing surface-trapped electrons and increasing the efficiency of hole utilization on TiO2 particles, as well as providing sufficient oxygen for photocatalytic degradation. TP1–TP15 were identified as photolytic products using gas chromatography tandem mass spectrometry (Figure 1B) (Zhu et al., 2004; Zhu et al., 2005). The primary reaction intermediates TP1–TP4 underwent subsequent oxidation and rearrangement steps, which led to the formation of various short-chain compounds. Mechanism studies suggested that the key step in the photodegradation of pyridaben was the cleavage of C–S bond between benzyl and pyridazinone group.
Conclusion and perspectives
This mini-review presents the levels of pyridaben in agricultural products, water and soil reported between 2010 and 2022. The removal methods of pyridaben are also summarized, including household processing, MSPE, and AOPs technologies. The one-pot reaction of dichloropyridazinone, p-tert-butylbenzyl chloride, and thiourea is a green synthesis method of pyridaben. As an acaricide used worldwide, pyridaben has been reported in monitoring studies on fruits, vegetables, herbs, and bee products. In general, vegetable samples exhibit the highest detection rates and residue levels. Pyridaben has low solubility in water and easily remains in soil. It exhibits high concentration levels and detection rates in agricultural soil samples. Peeling is the most effective household method for removing pyridaben residues, while washing, blanching/boiling, frying/drying, and juicing show limited removal efficiencies and even show concentration effects. Notably, some vegetables, such as leafy vegetables, do not have peelable outer layers. Therefore, effective vegetables processing methods need to be developed. MSPE and AOPs technologies have been developed for the removal of pyridaben. These methods are effective in treating pyridaben in aqueous solutions. However, effective soil treatment methods have not been developed so far. This gap deserves further concern given the tendency of pyridaben to remain in soil.
Author contributions
LC and MP organized and prepared this manuscript; DH supervised and contributed to reviewing the manuscript.
Funding
This work was funded by the National Natural Science Foundation of China under Grant (21897002) and (31972290).
Conflict of interest
The authors declare that the research was conducted in the absence of any commercial or financial relationships that could be construed as a potential conflict of interest.
Publisher’s note
All claims expressed in this article are solely those of the authors and do not necessarily represent those of their affiliated organizations, or those of the publisher, the editors and the reviewers. Any product that may be evaluated in this article, or claim that may be made by its manufacturer, is not guaranteed or endorsed by the publisher.
References
Agrebi, N. E., Traynor, K., Wilmart, O., Tosi, S., Leinartz, L., Danneels, E., et al. (2020). Pesticide and veterinary drug residues in belgian beeswax: occurrence, toxicity, and risk to honey bees. Sci. Total Environ. 745, 141036. doi:10.1016/j.scitotenv.2020.141036
Aguilera, A., Valverde, A., Camacho, F., Boulaid, M., and García-Fuentes, L. (2014). Household processing factors of acrinathrin, fipronil, kresoxim-methyl and pyridaben residues in green beans. Food control. 35, 146–152. doi:10.1016/j.foodcont.2013.06.038
Alston, D. G., and Thomson, S. V. (2004). Effects of fungicide residues on the survival, fecundity, and predation of the mites tetranychus urticae (Acari: Tetranychidae) and Galendromus occidentalis (Acari: Phytoseiidae). J. Econ. Entomol. 97, 950–956. doi:10.1603/0022-0493(2004)097
Andreu, V., and Picό, Y. (2012). Determination of currently used pesticide in biota. Anal. Bioanal. Chem. 404, 2659–2681. doi:10.1007/s00216-012-6331-x
Bae, H., You, S., Lim, W., and Song, G. (2021). Pyridaben leads to inhibition of cell growth and induction of cell death through intracellular mechanisms in early pregnancy. Pestic. Biochem. Physiol. 171, 104733. doi:10.1016/j.pestbp.2020.104733
Bahreini, R., Nasr, M., Docherty, C., Herdt, O., Muirhead, S., Feindel, D., et al. (2020). Evaluation of potential miticide toxicity to varroa destructor and honey bees, Apis mellifera, under laboratory conditions. Sci. Rep. 10, 21529. doi:10.1038/s41598-020-78561-2
Boulaid, M., Aguilera, A., Camacho, F., Soussi, M., and Valverde, A. (2005). Effect of household processing and unit-to-unit variability of pyrifenox, pyridaben, and tralomethrin residues in tomatoes. J. Agric. Food Chem. 53, 4054–4058. doi:10.1021/jf040455y
Cámara, M. A., Cermeño, S., Martínez, G., and Oliva, J. (2020). Removal residues of pesticides in apricot, peach and orange processed and dietary exposure assessment. Food Chem. x. 325, 126936. doi:10.1016/j.foodchem.2020.126936
Chen, X. X., Liu, X. Z., Dong, B. Z., and Hu, J. Y. (2018). Simultaneous determination of pyridaben, dinotefuran, DN and UF in eggplant ecosystem under open-field conditions: is dsipation behaviour and residue distribution. Chemosphere 195, 245–251. doi:10.1016/j.chemosphere.2017.12.011
Cheng, S. H., Lin, R. H., You, Y., Lin, T., Zeng, Z. H., Yu, C. H., et al. (2021). Comparative sensitivity of neoseiulus cucumeris and its prey tetranychus cinnabarinus, after exposed to nineteen pesticides. Ecotoxicol. Environ. Saf. 217, 112234. doi:10.1016/j.ecoenv.2021.112234
Christensen, H. B., Granby, K., and Rabolle, M. (2003). Processing factors and variability of pyrimethanil, fenhexamid and tolylfluanid in strawberries. Food Addit. Contam. 20, 728–741. doi:10.1080/0265203031000138286
Chung, S. W. C. (2018). How effective are common household preparations on removing pesticide residues from fruit and vegetables? a review. J. Sci. Food Agric. 98, 2857–2870. doi:10.1002/jsfa.8821
Chinese Pesticide Information Network (2022). Industry data. Available at: http://www.chinapesticide.org.cn/hysj/index.jhtml (Accessed May 20, 2022).
Dang, M. M., Liu, X. P., Huang, L., Li, J. M., Ren, Y. G., Long, C. Y., et al. (2020). Synthesis and biological activities of pyridaben. Fine Chem. Intermed. 50 (9–11), 37. doi:10.19342/j.cnki.issn.1009-9212.2020.04.003
Döker, İ., and Kazak, C. (2020). Toxicity and risk assessment of acaricides on the predatory mite, Euseius scutalis (Athias-Henriot) (Acari: Phytoseiidae) under laboratory conditions. Chemosphere 261, 127760. doi:10.1016/j.chemosphere.2020.127760
European Food Safety Authority (2010). Conclusion on the peer review of the pesticide risk assessment of the active substance pyridaben. EFSA J. 8, 1632. doi:10.2903/j.efsa.2010.1632
European Food Safety Authority, Brancato, A., Brocca, D., De Lentdecker, C., Ferreira, L., Greco, L., et al. (2017). Review of the existing maximum residue levels for pyridaben according to article 12 of regulation (EC) No 396/2005. EFSA J. 15, e05054. doi:10.2903/j.efsa.2017.5054
Food and Agriculture Organization and World Health Organization (2006). Updating the principles and methods of risk assessment: MRLs for pesticides and veterinary drugs. Rome.
Gomez, C., Bandez, M. J., and Navarro, A. (2007). Pesticides and impairment of mitochondrial function in relation with the parkinsonian syndrome. Front. Biosci. 12, 1079. doi:10.2741/2128
Han, Y. T., Dong, F. S., Xu, J., Liu, X. G., Li, Y. B., Kong, Z. Q., et al. (2014). Residue change of pyridaben in apple samples during apple cider processing. Food control. 37, 240–244. doi:10.1016/j.foodcont.2013.09.053
Hardman, J. M., Franklin, J. L., Moreau, D. L., and Bostanian, N. J. (2003). An index for selective toxicity of miticides to phytophagous mites and their predators based on orchard trials. Pest Manag. Sci. 59, 1321–1332. doi:10.1002/ps.769
Hirata, K., Kawamura, Y., Kudo, M., and Igarashi, H. (1995). Development of a new acaricide, pyridaben. J. Pestic. Sci. 20, 177–179. doi:10.1584/jpestics.20.177
Hollingworth, R. M., Ahammadsahib, K. I., Gadelhak, G., and McLaughlin, J. L. (1994). New inhibitors of complex i of the mitochondrial electron transport chain with activity as pesticides. Biochem. Soc. Trans. 22, 230–233. doi:10.1042/bst0220230
Huan, Z. B., Xu, Z., Jiang, W., Chen, Z. Q., and Luo, J. H. (2015). Effect of Chinese traditional cooking on eight pesticides residue during cowpea processing. Food Chem. x. 170, 118–122. doi:10.1016/j.foodchem.2014.08.052
Igarashi, H., and Sakamoto, S. (1994). Summary of toxicity studies with pyridaben. J. Pestic. Sci. 19, S243–S251. doi:10.1584/jpestics.19.4_S243
Jabali, Y., Millet, M., and El-Hoz, M. (2020). Spatio-temporal distribution and ecological risk assessment of pesticides in the water resources of abou ali river, northern lebanon. Environ. Sci. Pollut. Res. 27, 17997–18012. doi:10.1007/s11356-020-08089-5
Kailani, M. H., Al-Antary, T. M., and Alawi, M. A. (2021). Monitoring of pesticides residues in soil samples from the southern districts of jordan in 2016/2017. Toxin Rev. 40, 198–214. doi:10.1080/15569543.2019.1580747
Kim, S. -W., El-Aty, A. M. A., Rahman, M. M., Choi, J. -H., Choi, O.-J., Rhee, G. -S., et al. (2015). Detection of pyridaben residue levels in hot pepper fruit and leave by liquid chromatography-tandem mass spectrometry: effect of household processes. Biomed. Chromatogr. 29, 990–917. doi:10.1002/bmc.3383
Latli, B., Morrmoto, H., Williams, P. G., and Casida, J. E. (1998). Photoaffinity radioligand for NADH: ubiquinone oxidoreductase: [S-C3H2] (trifluoromethyl) diazirinyl-pyridaben. J. Label. Compd. Radiopharm. 41, 191–199. doi:10.1002/(SICI)1099-1344(199803)41:3<191:AID-JLCR74>3.0
Li, Z. X., Nie, J. Y., Yan, Z., Cheng, Y., Lan, F., Huang, Y. N., et al. (2018). A monitoring survey and dietary risk assessment for pesticide residues on peaches in China. Regul. Toxicol. Pharmacol. 97, 152–162. doi:10.1016/j.yrtph.2018.06.007
Li, Z. X., Zhang, Y. H., Zhao, Q. Y., Wang, C. Q., Cui, Y. L., Li, J., et al. (2020). Occurrence, temporal variation, quality and safety assessment of pesticide residues on citrus fruits in China. Chemosphere 258, 127381. doi:10.1016/j.chemosphere.2020.127381
Ma, J. Z., Huang, Y., Jiang, P., Liu, Z., Luo, Q., Zhong, K. Y., et al. (2021a). Pyridaben induced cardiotoxicity during the looping stages of zebrafish (Danio rerio) embryos. Aquat. Toxicol. 237, 105870. doi:10.1016/j.aquatox.2021.105870
Ma, X. C., Ma, Z. Q., Zhao, M. X., Wang, Y. H., Peng, Y., Guo, X., et al. (2021b). Facile synthesis of magnetic molybdenum disulfide@graphene nanocomposite with amphiphilic properties and its application in solid-phase extraction for a wide polarity of insecticides in wolfberry samples. Anal. Methods 13, 672–684. doi:10.1039/d0ay01939a
Malhat, F., Saber, E., Anagnostopoulos, C., and Shokr, S. A. (2021). Dissipation behavior and dietary risk assessment of pyridaben in open field strawberries and cucumber under Egyptian cultivation conditions. Environ. Sci. Pollut. Res. 28, 60122–60129. doi:10.1007/s11356-021-14752-2
Pan, L. X., Feng, X. X., Cao, M., Zhang, S. W., Huang, Y. F., Xu, T. H., et al. (2019). Determination and distribution of pesticides and antibiotics in agricultural soils from northern China. RSC Adv. 9, 15686–15693. doi:10.1039/c9ra00783k
Park, J. -J., Kim, M., Lee, J. -H., Shin, K. -I., Lee, S. E., Kim, J. -G., et al. (2011). Sublethal effects of fenpyroximate and pyridaben on two predatory mite species, Neoseiulus womersleyi and Phytoseiulus persimilis (Acari, Phytoseiidae). Exp. Appl. Acarol. 54, 243–259. doi:10.1007/s10493-011-9435-7
Ping, H., Wang, B. H., Li, C., Li, Y., Ha, X. J., Jia, W. S., et al. (2022). Potential health risk of pesticide residues in greenhouse vegetables under modern urban agriculture: a case study in beijing, china. J. Food Compost. Anal. 105, 104222. doi:10.1016/j.jfca.2021.104222
Rand, G. M., and Clark, J. R. (2000). Hazard/risk assessment of pyridaben: i. aquatic toxicity and environmental chemistry. Ecotoxicology 9, 157–168. doi:10.1023/A:1008934502348
Sabzevari, S., and Hofman, J. (2022). A world wide review of currently used pesticides’ monitoring in agricultural soils. Sci. Total Environ. 812, 152344. doi:10.1016/j.scitotenv.2021.152344
Shi, R. G., Yuan, L., Chen, M. L., Zheng, X. Q., Liu, X. W., Zhao, Y. J., et al. (2020). Detection of frequently used pesticides in apple orchard soil in China by high resolution mass spectrometry. Pol. J. Environ. Stud. 29, 1341–1350. doi:10.15244/pjoes/108509
Shogo, M., Kazuyoshi, A., Masuo, Y., and Shuichi, I. (1995). Antitumor agent, novel 3(2H)-pyridazinone derivatives and their preparation. European Patent No 066,523. MunichGerman: European Patent Office.
Tan, H. D., Zhang, H. J., Wu, C. Y., Wang, C. M., and Li, Q. F. (2021). Pesticides in surface waters of tropical river basins draining areas with rice–vegetable rotations in Hainan, china: occurrence, relation to environmental factors, and risk assessment. Environ. Pollut. 283, 117100. doi:10.1016/j.envpol.2021.117100
Tong, Z., Duan, J. S., Wu, Y. C., Liu, Q. Q., He, Q. B., Shi, Y. H., et al. (2018). A survey of multiple pesticide residues in pollen and beebread collected in china. Sci. Total Environ. 640–641, 1578–1586. doi:10.1016/j.scitotenv.2018.04.424
Wang, L., Zheng, M. G., Xu, H. Y., Hua, Y., Liu, A. F., Li, Y., et al. (2022). Fate and ecological risks of current-use pesticides in seawater and sediment of the yellow sea and east china sea. Environ. Res. 207, 112673. doi:10.1016/j.envres.2021.112673
Wang, N., Zhao, S., Long, X. F., Gong, J., Sui, C. L., Zhang, Y. P., et al. (2020). Determination, risk assessment and processing factors for pyridaben in field-incurred kiwifruit samples. J. Environ. Sci. Health Part B 55, 613–619. doi:10.1080/03601234.2020.1753458
World Health Organization (2020). The WHO recommended classification of pesticides by hazard and guidelines to classification 2019.
Wilmart, O., Legrève, A., Scippo, M. -L., Reybroeck, W., Urbain, B., Graff, D. C., et al. (2021). Honey bee exposure scenarios to selected residues through contaminated beeswax. Sci. Total Environ. 772, 145533. doi:10.1016/j.scitotenv.2021.145533
Wu, L. P., Zhou, X. L., Zhao, D. Y., Feng, T., Zhou, J., Sun, T., et al. (2017). Seasonal variation and exposure risk assessment of pesticide residues in vegetables from xinjiang uygur autonomous region of china during 2010–2014. J. Food Compost. Anal. 58, 1–9. doi:10.1016/j.jfca.2016.12.025
Wu, P. L., Wang, P. S., Gu, M. Y., Xue, J., and Wu, X. L. (2021). Human health risk assessment of pesticide residues in honeysuckle samples from different planting bases in China. Sci. Total Environ. 759, 142747. doi:10.1016/j.scitotenv.2020.142747
Xiao, O. L., Li, M. M., Chen, D. Y., Chen, J. Y., Gandara-Simal, J., Dai, X. F., et al. (2022). The dissipation, processing factors, metabolites, and risk assessment of pesticides in honeysuckle from field to table. J. Hazard. Mat. 431, 128519. doi:10.1016/j.jhazmat.2022.128519
Xin, S. K., Zhao, X. X., Lyu, B., Chen, D. W., Li, J. G., Zhao, Y. F., et al. (2020). Occurrences and dietary exposure analysis for acaricides in the 6th chinese total diet study. J. Hyg. Res. 49, 397–402. (in Chinese). doi:10.19813/j.cnki.weishengyanjiu.2020.03.009
Xu, Y., Zhuang, Z. X., Guo, W. T., Cui, R. R., Fan, J. Y., and Liu, Y. (2016). Research status and prospects of pyridaben. Shandong Chem. Indus 45, 32–38. doi:10.19319/j.cnki.issn.1008-021x.2016.24.014
Yao, Q. H., Yan, S. A., Chen, H. Z., Li, J., and Lin, Q. (2020). Dietary risk assessment of pesticide residues on Tremella fuciformis berk (snow fungus) from fujian province of china. Food Addit. Contam. Part A 37, 1289–1299. doi:10.1080/19440049.2020.1766119
Yu, X., Zhong, T., Zhang, Y. J., Zhao, X. H., Xiao, Y., Wang, L., et al. (2022). Design, preparation, and application of magnetic nanoparticles for food safety analysis: a review of recent advances. J. Agric. Food Chem. 70, 46–62. doi:10.1021/acs.jafc.1c03675
Zeshan, M., Bhatti, I. A., Mohsin, M., Iqbal, M., Amjed, N., Nisar, J., et al. (2022). Remediation of pesticides using TiO2 based photocatalytic strategies: a review. Chemosphere 300, 134525. doi:10.1016/j.chemosphere.2022.134525
Zhang, Y. D., Si, W. S., Chen, L., Shen, G. Q., Bai, B., Zhou, C. Y., et al. (2021). Determination and dietary risk assessment of 284 pesticide residues in local fruit cultivars in shanghai, china. Sci. Rep. 22, 9681. doi:10.1038/s41598-021-89204-5
Zhao, J. L., Huang, P. F., Wang, X. M., Yang, J., Zhou, Z., Du, X. Z., et al. (2022). Efficient adsorption removal of organic nitrogen pesticides: insight into a new hollow NiO/Co@C magnetic nanocomposites derived from metal–organic framework. Sep. Purif. Technol. 287, 120608. doi:10.1016/j.seppur.2022.120608
Zheng, S., Chen, B., Qiu, X. Y., Chen, M., Ma, Z. Y., Yu, X. G., et al. (2016). Distribution and risk assessment of 82 pesticides in jiulong river and estuary in south china. Chemosphere 144, 1177–1192. doi:10.1016/j.chemosphere.2015.09.050
Zhu, X. L., Feng, X. G., Yuan, C. W., Cao, X. M., and Li, J. H. (2004). Photocatalytic degradation of pesticide pyridaben in suspension of TiO2: identification of intermediates and degradation pathways. J. Mol. Catal. A Chem. 214, 293–300. doi:10.1016/j.molcata.2004.01.001
Zhu, X. L., Yuan, C. W., Bao, Y. C., Yang, J. H., and Wu, Y. Z. (2005). Photocatalytic degradation of pesticide pyridaben on TiO2 particles. J. Mol. Catal. A Chem. 229, 95–105. doi:10.1016/j.molcata.2004.11.010
Keywords: pyridaben, green synthesis, detection rate, household processing, magnetic solid-phase extraction, advanced oxidation processes
Citation: Chen L, Pan M and Hu D (2022) An overview on the green synthesis and removal methods of pyridaben. Front. Chem. 10:975491. doi: 10.3389/fchem.2022.975491
Received: 22 June 2022; Accepted: 27 June 2022;
Published: 14 July 2022.
Edited by:
Huanzhen Ni, Lilly Research Laboratories, United StatesCopyright © 2022 Chen, Pan and Hu. This is an open-access article distributed under the terms of the Creative Commons Attribution License (CC BY). The use, distribution or reproduction in other forums is permitted, provided the original author(s) and the copyright owner(s) are credited and that the original publication in this journal is cited, in accordance with accepted academic practice. No use, distribution or reproduction is permitted which does not comply with these terms.
*Correspondence: Deyu Hu, gzu_dyhu@126.com
†These authors have contributed equally to this work and share first authorship