- 1School of Materials and Chemical Engineering, Henan University of Urban Construction, Pingdingshan, China
- 2Henan Province Key Laboratory of Water Pollution Control and Rehabilitation Technology, Henan University of Urban Construction, Pingdingshan, China
- 3Key Laboratory of Advanced Functional Materials, Ministry of Education, Faculty of Materials and Manufacturing, Beijing University of Technology, Beijing, China
Graphitic carbon nitride (g-C3N4) photocatalysis for water splitting is harvested as a fascinating way for addressing the global energy crisis. At present, numerous research subjects have been achieved to design and develop g-C3N4 photocatalysis, and the photocatalytic system still suffers from low efficiency that is far from practical applications. Here, there is an inspiring review on the latest progress of the doping strategies to modify g-C3N4 for enhancing the efficiency of photocatalytic water splitting, including non-metal doping, metal doping, and molecular doping. Finally, the review concludes a summary and highlights some perspectives on the challenges and future research of g-C3N4 photocatalysts.
Introduction
The global energy demands and environmental crisis have stimulated tremendous research on the exploration of green and renewable energy due to awareness of energy conservation and environmental protection (Miao et al., 2022). Since titanium dioxide (TiO2) was discovered as the photoanode for photoelectrochemical (PEC) water splitting(Fujishima and Honda, 1972), semiconductor-based photocatalysis for solar hydrogen production has seen an upsurge in global interests (Wang and Wang, 2019; Zada et al., 2020; Qi et al., 2021; Wei et al., 2021). However, it is still very challenging to achieve high solar-to-hydrogen (STH) conversion efficiency toward practical applications. To make high utilization of solar energy, the exploration of visible-light-active photocatalysts is highly desirable. In 2009, Wang et al. developed the pioneering work on g-C3N4 for visible-light–driven photocatalytic water splitting(Wang et al., 2009), and g-C3N4-based photocatalysis has drawn considerable attention in the last decade (Xiao et al., 2021; Xing et al., 2021; Chen et al., 2022a) (Figures 1A–E). For unification in this study, we will consider the two kinds of materials with triazine (C3N3) unit or tri-s-triazine (C6N7) unit (Figure 1A) to name as g-C3N4. g-C3N4 affords a lamellar structure consisting of C and N atoms which is similar to graphene and can be traced back to the original form of “melon” found by Berzelius and Liebig in 1834 (Liebig, 1834). Unlike TiO2, g-C3N4 affords a narrow bandgap of 2.7 eV (Figure 1C) with the valance band (VB) position at +1.6 eV and conduction band (CB) position at −1.1 eV vs. normal hydrogen electrode (NHE) (Wang et al., 2009) (Figure 1E). This enables g-C3N4 to drive photocatalytic reaction using visible light.
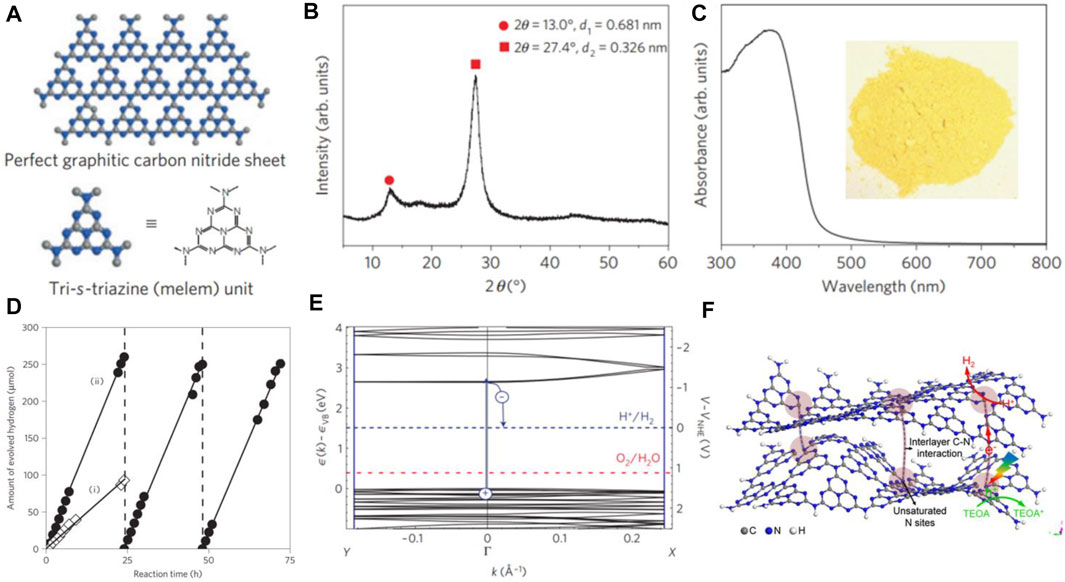
FIGURE 1. (A) Schematic diagram of a perfect g-C3N4 sheet constructed from melem units, (B) experimental XRD pattern of g-C3N4, (C) ultraviolet–visible diffuse reflectance spectrum of the g-C3N4. Inset: Photograph of g-C3N4, (D) typical time course of H2 production from water containing 10 vol% triethanolamine as an electron donor under visible light (of wavelength longer than 420 nm) by (i) unmodified g-C3N4 and (ii) 3.0 wt% Pt-deposited g-C3N4 photocatalyst, (E) density-functional-theory band structure for polymeric melon calculated along with the chain (Γ–X direction) and perpendicular to the chain (Y–Γ direction). The position of the reduction level for H+ to H2 is indicated by the dashed blue line, and the oxidation potential of H2O to O2 is indicated by the red dashed line just above the valence band. Copyright 2009, Springer Nature; (F) illustration of the charge transfer in g-C3N4 nanosheets under visible light. Copyright 2017, Elsevier.
Generally speaking, the g-C3N4 photocatalyst possesses all the following abilities: stability, non-toxicity, abundant source, visible-light-responsive absorption, and easy to control and modify. As a fantastic visible-light–driven photocatalyst, its remarkable property has been conducted on widely photocatalytic applications, such as H2 evolution from water (Zhao et al., 2017a; Yu et al., 2021; Zhao et al., 2022), O2 evolution (Yang et al., 2019; Zhao et al., 2019; Xu et al., 2021), overall water splitting (Chen et al., 2019; Bhagat and Dashora, 2021; Wu et al., 2021), photodegradation of pollutants (Xiong et al., 2016; Duan et al., 2019; Jing et al., 2021), CO2 reduction (Jiang et al., 2018; Yang et al., 2020a; Cheng et al., 2020), organic synthesis (Devthade et al., 2018; Camussi et al., 2019; Lima et al., 2019), and photoelectrocatalysis (Karimi-Nazarabad et al., 2019; Wu et al., 2020; Huo et al., 2021). However, the three crucial issues of light absorption efficiency, charge separation and transfer efficiency, and surface reaction efficiency still restrict the development of high-performance g-C3N4 photocatalysis, which is fairly difficult to achieve by pristine g-C3N4.
Although the g-C3N4-based photocatalysis has been fully discussed in many recent review articles(Li et al., 2020a; Li et al., 2021a; Chen et al., 2021; Xing et al., 2021; Zhu et al., 2021; Chen et al., 2022b), a relative focus review about doping strategies to modify g-C3N4 for improving the efficiency of water splitting is still lacking. Compared with other effective strategies for modification of g-C3N4, like heterojunction construction, defect introduction, and nanostructure controlling, the modification process of g-C3N4 can be comparatively simplified by element doping to tune the band gap, which considerably broadens the light absorption and accelerates the electron-hole pair separation (Li et al., 2020b). This work overviews the recent advances of g-C3N4 materials focusing on efficient photocatalytic water splitting in doping strategies for modifying carbon nitride including non-metal doping, metal doping, and molecular doping. This review also aims to present a general summarization in boosting the g-C3N4 photocatalyst to seek new inspiration for material science.
Basic Properties of Carbon Nitride for Solar Water Splitting
Under irradiation, the electrons of the g-C3N4 photocatalyst can be excited from the VB to CB by absorbing the photons with the energy (hν) higher than the bandgap energy, wherein holes are left in the VB (Luo et al., 2016). The large parts of the photoexcited charge carriers will combine rapidly, and only a small part of photogenerated electrons and holes can be transferred to the surface of g-C3N4 to involve the reaction. Then, the water molecules can be reduced with the photoexcited electrons for H2 evolution and oxidized by the photoexcited holes for O2 generation during the photocatalytic reaction. For H2 evolution, the CB potential of the photocatalyst should be more negative than the H2 reduction potential, while the VB potential should be more positive than the water oxidation potential for the O2 evolution from water. The g-C3N4 photocatalyst possesses a CB of −1.1 eV and a VB of 1.6 eV is fit for splitting water to H2 and O2.
So far, visible-light photoadsorption, high chemical stability, appropriate CB and VB potentials, and strong photocatalytic activity make g-C3N4 be the most widely focused in photocatalytic water splitting. Nevertheless, the efficiency of water splitting by g-C3N4 is still low, mainly due to limited photoadsorption in the visible-light region, limited ability of electron transport along or across the g-C3N4 sheets (Figure 1F), and recombination of photoexcited electron-hole pairs.
In a word, due to dramatic development of g-C3N4 photocatalysts in the STH conversion field, a review focusing on the photocatalytic water splitting is still necessary to provide researchers a state-of-art progress in this dynamic research field. This review presents a brief discussion of the current doping research, accompanied with the challenges and future direction of g-C3N4 photocatalysts for photocatalytic applications.
Doping Strategies for Modifying Carbon Nitride
To develop advanced g-C3N4 photocatalysts, a doping strategy is considered an appealing way to modulate physicochemical properties such as band structure tailoring and light adsorption improving, which, therefore, enhance the performance of photocatalyst (Patnaik et al., 2021). Based on the arrangements of doping elements, the classification of doping can be divided into non-metal doping, metal doping, and molecular doping.
Non-Metal Doping
The fabrication of non-metal doped carbon nitride is effective in modulating the electronic structure of g-C3N4 by distorting the π-conjugated orbital. Boron-doped g-C3N4 was prepared by microwave heating for hydrogen evolution, and boric acid was used as a doping source combined with melamine and urea for thermal condensation (Chen et al., 2018). g-C3N4 nanosheets can be in situ modified by boron atoms to improve the photoadsorption, hinder the annihilation of charge carriers, and prolong the lifetime of photogenerated electrons. Combining g-C3N4 with strongly electronegative dopants such as fluorine to form an F-doped material not only raised the valence band but also affected the thermodynamic driving force for H2 reduction (Zhu et al., 2017). Fluorinated (F) carbon nitride solids were also reported with excellent visible-light photocatalytic activity (Shevlin and Guo, 2016). Fluorination not only provided a modified texture but also enabled effective adjustment of the electronic band structure, which was demonstrated by improved activities. To further explore the structural distortion-dependent photoreactivity, it is more desirable to exploit co-doping that may be a good attempt to further improve the photocatalytic activity of g-C3N4 through the synergistic effects of the two dopants. For instance, B/F co-doped g-C3N4 was fabricated by polymerizing urea and ionic liquids ([Bmim][BF4]), which was used as the texture modifier and dopant source (Lin and Wang, 2014). This research leads in a new one-pot fabrication of B and F co-doped g-C3N4.
In addition to F, other halogen elements we cannot ignore are chloride (Cl), bromine (Br), and iodine (I) in the doping area. Br-doped g-C3N4 was also successfully synthesized by using ionic liquid as the Br source and soft-template for photoredox water splitting (Zhao et al., 2017b). The Br-doping tuned light absorption and band structure without destroying the major construction of the g-C3N4 polymer. Similarly, I doped g-C3N4 materials also lead to positive effects that enlarged the specific surface area, enhanced optical absorption, narrowed the bandgap, and accelerated the photoinduced charge carrier transfer rate, leading to an increasing H2 evolution rate (Iqbal et al., 2020).
In addition, introducing other non-metal elements are also effective strategies in promoting the photocatalytic property (Deng et al., 2019; Li et al., 2021b; Li et al., 2021c; Liu et al., 2021; Yan et al., 2022). For instance, carbon (C) self-doped g-C3N4 was prepared via a combined method of melamine-cyanuric acid complex supramolecular pre-assembly and solvothermal pre-treatment (Li et al., 2021b). The H2 evolution rate for optimized g-C3N4 was 18 times higher than that of bulk g-C3N4, and the enhanced performance derives from the extended optical absorption, accelerated photoactivated charge carrier separation, and transfer efficiency. Unique oxygen-doped g-C3N4 materials were synthesized, which realized the synergetic control of the electronic structure and morphology and possessed the advantages of enlarged surface area, increased exposed active edges, and improved separation efficiency (Yan et al., 2022). Some other high-performance non-metal doped materials including phosphorus-doped g-C3N4(Yang et al., 2018; Lin et al., 2020; Zhao et al., 2020) and sulfur-doped g-C3N4 (Li et al., 2019a; Yang et al., 2020b; Long et al., 2020) have also been developed. For instance, Yang et al. reported a flower-like P-doped g-C3N4 which was prepared using phosphoric acid and cyanuric acid-melamine complex, which served as the P source and the precursor of g-C3N4, respectively, (Yang et al., 2018). The as-prepared P-doped g-C3N4 showed a high visible-light photocatalytic H2 evolution rate of 256.4 μmol h−1 50 mg−1 and almost 24-folds higher than those of the pristine g-C3N4. A sulfur (S) doped-g-C3N4 nanosheet with terminal-methylate was presented with a tunable bandgap (Li et al., 2019a). The VB near the Fermi level was split due to S atoms into methylated melon units, which generated a new empty mid-gap electronic state and improved the light-responsive property up to 700 nm. Furthermore, the photocatalytic activity restricted by intralayered hydrogen bonds should also be considered. Yang et al. reported an S-doped g-C3N4 through poly-condensation and the mixture of dicyandiamide and thioacetamide, resulting in greatly enhanced visible-light-response ability and n → π* electron transition. The substituting of sp2-hybridized N with S atoms contributed to break intralayered hydrogen bonds, which resulted in photocatalytic H2 production (Yang et al., 2020b) (Figures 2A,B).
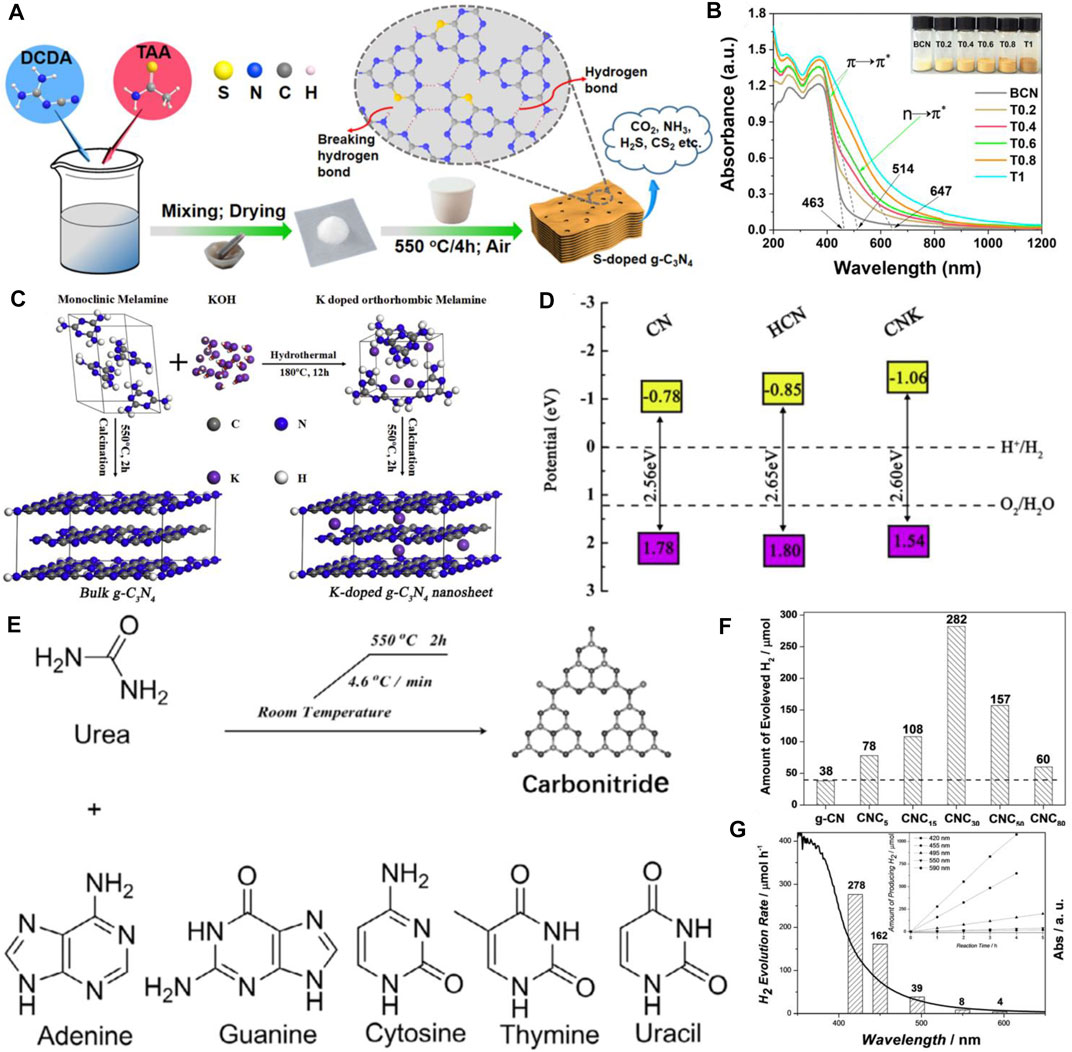
FIGURE 2. (A) Schematic illustration for the formation of multi-layered cake-like porous g-C3N4 with broken hydrogen bonds by S-doping, (B) UV–visible diffuse reflectance spectra of samples. Copyright 2020, Elsevier; (C) Schematic illustration of the KOH-assisted hydrothermal-reformed melamine strategy for achieving simultaneous K-doping and exfoliation of g-C3N4, (D) band structure alignments for the CN, HCN, and CNK sample. Copyright 2018, Elsevier; (E) Chemical production of polymeric carbon nitride semiconductors from nucleobases and urea, (F) Effect of cytosine amount on the HER, (G) wavelength dependence of the HER with CNC30 loaded with 3 wt% Pt. Inset: time-dependence of the HER with CNC30 at different irradiation wavelengths. Copyright 2017 John Wiley and Sons, Inc.
Metal Doping
Due to abundant orbital electrons, the electron structure and optical property of g-C3N4 can also be affected by metal element doping, leading to focusing on the metal doping method. The cobalt (Co)-doped g-C3N4 nanosheet was investigated to provide more separation centers by forming Co–N bond, which can celebrate charge transfer and enhance photocatalytic performance (Yang et al., 2021). Moreover, potassium-modified g-C3N4 (K-g-C3N4) nanosheets were synthesized (Sun et al., 2019) (Figures 2C,D). Photocatalytic H2 production tests under visible light irradiation showed high photocatalytic activities of K-g-C3N4 nanosheets (up to about 13-folds higher than that of original g-C3N4) as well as an apparent quantum efficiency (AQE) of 6.98% at 420 nm. In addition, lanthanum (La) and Co co-doped g-C3N4 was prepared by the wet impregnation method (Tasleem and Tahir, 2021). The H2 evolution by La/Co co-doped-g-C3N4 showed the highest H2 production of 250 μmol g−1h−1 among the samples, which was 2.5, 1.35, and 1.25 times higher than that of original g-C3N4, La-g-C3N4, and Co-g-C3N4, respectively. The enhanced activity can be contributed to the celebrated charge separation, which was originally the electron trapping capability of La and Co.
Molecular Doping
Heteroatom doping as discussed above is often used to modulate the atomic and band structure of g-C3N4 to promote light harvesting and celebrate electron-hole separation and transfer. Especially, integrating another structure-matching aromatic structure with g-C3N4 is a unique method to tune the intrinsic features (Yang et al., 2017; Li et al., 2018; Li and Zhang, 2018; Li et al., 2019b; Li et al., 2019c; Liu et al., 2019; Li et al., 2020c; Jiang et al., 2021; Zhou et al., 2021). The thermal co-polymerization of the aromatic comonomers and precursors of g-C3N4 can narrow the band gaps of g-C3N4, which extends the visible light absorption edge to enhance the utilization of sunlight. For instance, Liu et al. designed in-plane benzene-ring doped g-C3N4 nanosheets by copolymerizing urea and 4, 4′-sulfonyldiphenol. It exhibited dramatic H2 generation efficiency with a PHE rate of 12.3 mmol h−1 g−1, which was almost 12-folds higher than that of intrinsic g-C3N4 and the AQE of 17.7% at 420 nm (Jiang et al., 2021). Moreover, copolymerization of urea and naphthoic acid has been conducted to construct an aromatic ring–doped g-C3N4, which was an effective strategy to extend the π-conjugated system for visible light absorption and elevate the efficiency of charge transfer (Li et al., 2020c). In addition, Yang et al. enriched the construction of g-C3N4 by using nucleobases (adenine, guanine, cytosine, thymine, and uracil) and urea to energize the production of the charge carrier with light irradiation, which inducted photoredox reactions for stable H2 evolution (Zhou et al., 2021) (Figures 2E–G).
Conclusion and Outlook
This review presents a promising visible-light–driven photocatalyst, g-C3N4, benefiting from its unique microstructure, resistance against acids, and bases and fantastic band structure. Nevertheless, the pristine g-C3N4 suffers from some shortcomings, including limited photoadsorption capability and fast recombination of photoexcited electron-hole pairs, which largely restricts practical applications. The present review depicts a focus review on the doping strategies to design efficient g-C3N4 in the use of photocatalytic water splitting. In summary, doping can introduce the impurity levels in the band gap region to create a new band edge potential, which can extend the spectral response region with decreased band gap. In addition, the hetero dopants get settled either in the lattice or insert in the interlayers of g-C3N4, inducing the formation of hybridized orbitals. The hybridization between g-C3N4 and dopant orbital remarkably affects the charge transportation, life time of charge carriers, and the photocatalytic performance of g-C3N4. In short, doping is a feasible and effective strategy to regulate photo-absorbance, redox potentials, and transfer of photo-induced charge carriers and one of the attractive strategies to tune the physicochemical properties of g-C3N4.
To date, doping provides an innovative approach to promote the efficiency of g-C3N4 photocatalyst. However, some issues like nonuniform doping, formation of surface trapping center, or low oxidizing and reducing capability resulting from narrowing the bandgap still existed, while the mechanisms in this field are at the primary stage and further systematic investigations are still needed because of the relatively low visible-light photocatalytic efficiency, which is far from the requirements of practical applications. Some issues that must be resolved for doped g-C3N4 photocatalyst involve the fact that 1) the doping mechanisms of enhanced photocatalytic property is not clear. For example, many explanations of doping technology still stay at the stage of “the enhanced photocatalytic activity is contributed to the doping method” with no discussion about mechanism and essential meaning of element doping. 2) It is still challenging to bring forth new ideas on doping methods, and finding the right balance of lower redox ability and higher photocatalytic activity is highly desired. To overcome the challenges, lots of attempts are still needed. The heteroatom-doping assisted with theoretical simulation calculation can be a feasible method to analyze the doping effect. Especially, it is significant to develop a crystalline g-C3N4 (CCN) doped by metals or non-metals, which improves the charge separation, increases the reactive facet exposing, and shows dramatic photocatalytic water splitting performance. Furthermore, a broad range of heterostructures, including quantum dots/g-C3N4 junction, polymer/g-C3N4 junction, semiconductor/g-C3N4 junction, cocatalyst modification of single atoms and defects engineering, as well as nanostructure and crystalline control, should also be considered for improved photocatalysis to increase the photoabsorption, accelerating the charge separation and transfer, elongating the charge carrier lifetime, and boosting the photocatalytic water splitting. Focusing on the perspective of green and renew energy, it is no doubt that g-C3N4-based photocatalyst will draw more attention on the research of water splitting in the future.
Author Contributions
YY conceived the structure of the manuscript and wrote the manuscript. WN, LD, and YM collected materials and data. JW and KX revised the manuscript.
Funding
This work is financially supported by the Natural Science Youth Foundation of Henan Province (202300410032), Key scientific research projects of colleges and universities of the Henan Provincial Department of Education (21A150010), and the Foundation for University Key Teacher by the Henan University of Urban Construction (YCJQNGGJS202109).
Conflict of Interest
The authors declare that the research was conducted in the absence of any commercial or financial relationships that could be construed as a potential conflict of interest.
Publisher’s Note
All claims expressed in this article are solely those of the authors and do not necessarily represent those of their affiliated organizations, or those of the publisher, the editors, and the reviewers. Any product that may be evaluated in this article, or claim that may be made by its manufacturer, is not guaranteed or endorsed by the publisher.
References
Bhagat, B. R., and Dashora, A. (2021). Understanding the Synergistic Effect of Co-loading and B-Doping in G-C3N4 for Enhanced Photocatalytic Activity for Overall Solar Water Splitting. Carbon 178, 666–677. doi:10.1016/j.carbon.2021.03.049
Camussi, I., Mannucci, B., Speltini, A., Profumo, A., Milanese, C., Malavasi, L., et al. (2019). g-C3N4 - Singlet Oxygen Made Easy for Organic Synthesis: Scope and Limitations. ACS Sustain. Chem. Eng. 7 (9), 8176–8182. doi:10.1021/acssuschemeng.8b06164
Chen, F., Wu, C., Zheng, G., Qu, L., and Han, Q. (2022). Few-layer Carbon Nitride Photocatalysts for Solar Fuels and Chemicals: Current Status and Prospects. Chin. J. Catal. 43, 1216–1229. doi:10.1016/s1872-2067(21)63985-2
Chen, J., Li, K., Li, X., Fan, J., and Lv, K. (2021). Preparation and Modification of Crystalline Carbon Nitride. Chin. J. Inorg. Chem. 37 (10), 1713–1726.
Chen, P., Xing, P., Chen, Z., Lin, H., and He, Y. (2018). Rapid and Energy-Efficient Preparation of Boron Doped G-C3N4 with Excellent Performance in Photocatalytic H2-Evolution. Int. J. Hydrogen Energy 43, 19984–19989. doi:10.1016/j.ijhydene.2018.09.078
Chen, X., Shi, R., Chen, Q., Zhang, Z., Jiang, W., Zhu, Y., et al. (2019). Three-dimensional Porous G-C3N4 for Highly Efficient Photocatalytic Overall Water Splitting. Nano Energy 59, 644–650. doi:10.1016/j.nanoen.2019.03.010
Chen, X., Wang, J., Chai, Y., Zhang, Z., and Zhu, Y. (2022). Efficient Photocatalytic Overall Water Splitting Induced by the Giant Internal Electric Field of a G-C3N4/rGO/PDIP Z-Scheme Heterojunction. Adv. Mat. 33, 2007479.
Cheng, L., Yin, H., Cai, C., Fan, J., and Xiang, Q. (2020). Single Ni Atoms Anchored on Porous Few‐Layer g‐C3N4 for Photocatalytic CO2 Reduction: The Role of Edge Confinement. Small 16, 2002411. doi:10.1002/smll.202002411
Deng, P., Gan, M., Zhang, X., Li, Z., and Hou, Y. (2019). Non-noble-metal Ni Nanoparticles Modified N-Doped G-C3N4 for Efficient Photocatalytic Hydrogen Evolution. Int. J. Hydrogen Energy 44, 30084–30092. doi:10.1016/j.ijhydene.2019.09.129
Devthade, V., Kamble, G., Ghugal, S. G., Chikhalia, K. H., and Umare, S. S. (2018). Visible Light-Driven Biginelli Reaction over Mesoporous G-C3N4 Lewis-Base Catalyst. ChemistrySelect 3, 4009–4014. doi:10.1002/slct.201800591
Duan, Y., Li, X., Lv, K., Zhao, L., and Liu, Y. (2019). Flower-like G-C3N4 Assembly from Holy Nanosheets with Nitrogen Vacancies for Efficient NO Abatement. Appl. Surf. Sci. 492, 166–176. doi:10.1016/j.apsusc.2019.06.125
Fujishima, A., and Honda, K. (1972). Electrochemical Photolysis of Water at a Semiconductor Electrode. Nature 238, 37–38. doi:10.1038/238037a0
Huo, Y., Zhang, L., Wang, S., and Wang, X. (2021). Polyoxometalate@g-C3N4 Nanocomposite for Enhancing Visible Light Photoelectrocatalytic Performance. Chemosphere 279, 130559. doi:10.1016/j.chemosphere.2021.130559
Iqbal, W., Yang, B., Zhao, X., Rauf, M., Mohamed, I. M. A., Zhang, J., et al. (2020). Facile One-Pot Synthesis of Mesoporous G-C3N4 Nanosheets with Simultaneous Iodine Doping and N-Vacancies for Efficient Visible-Light-Driven H2 Evolution Performance. Catal. Sci. Technol. 10, 549–559. doi:10.1039/c9cy02111f
Jiang, R., Lu, G., Liu, J., Wu, D., Yan, Z., and Wang, Y. (2021). Incorporation of π-conjugated Molecules as Electron Donors in G-C3N4 Enhances Photocatalytic H2-Production. Renew. Energy 164, 531–540. doi:10.1016/j.renene.2020.09.040
Jiang, Z., Wan, W., Li, H., Yuan, S., Zhao, H., and Wong, P. K. (2018). A Hierarchical Z-Scheme α-Fe2 O3/g-C3N4 Hybrid for Enhanced Photocatalytic CO2 Reduction. Adv. Mat. 30, 1706108. doi:10.1002/adma.201706108
Jing, L., Wang, D., He, M., Xu, Y., Xie, M., Song, Y., et al. (2021). An Efficient Broad Spectrum-Driven Carbon and Oxygen Co-doped G-C3N4 for the Photodegradation of Endocrine Disrupting: Mechanism, Degradation Pathway, DFT Calculation and Toluene Selective Oxidation. J. Hazard. Mater. 401, 123309. doi:10.1016/j.jhazmat.2020.123309
Karimi-Nazarabad, M., Goharshadi, E. K., and Mahdizadeh, S. J. (2019). Efficient Photoelectrocatalytic Water Oxidation by Palladium Doped G-C3N4 Electrodeposited Thin Film. J. Phys. Chem. C 123 (43), 26106–26115. doi:10.1021/acs.jpcc.9b07755
Li, J., Liu, X., Che, H., Liu, C., and Li, C. (2021). Facile Construction of O-Doped Crystalline/Non-crystalline G-C3N44 Embedded Nano-Homojunction for Efficiently Photocatalytic H2 Evolution. Carbon 172, 602–612. doi:10.1016/j.carbon.2020.10.051
Li, K., and Zhang, W. D. (2018). Creating Graphitic Carbon Nitride Based Donor-π-Acceptor-π-Donor Structured Catalysts for Highly Photocatalytic Hydrogen Evolution. Small 14, e1703599. doi:10.1002/smll.201703599
Li, K., Sun, M., and Zhang, W.-D. (2018). Polycyclic Aromatic Compounds-Modified Graphitic Carbon Nitride for Efficient Visible-Light-Driven Hydrogen Evolution. Carbon 134, 134–144. doi:10.1016/j.carbon.2018.03.089
Li, K., Zhang, M., Ou, X., Li, R., Li, Q., Fan, J., et al. (2021). Strategies for the Fabrication of 2D Carbon Nitride Nanosheets. Acta Phys. -Chim. Sin. 37 (8), 2008010.
Li, L., Zhang, J., Zhang, Q., Wang, X., and Dai, W.-L. (2021). Superior Sponge-like Carbon Self-Doping Graphitic Carbon Nitride Nanosheets Derived from Supramolecular Pre-assembly of a Melamine-Cyanuric Acid Complex for Photocatalytic H2 Evolution. Nanotechnology 32, 155604. doi:10.1088/1361-6528/abd6d1
Li, S., Peng, Y., Hu, C., and Chen, Z. (2020). Self-assembled Synthesis of Benzene-Ring-Grafted G-C3N4 Nanotubes for Enhanced Photocatalytic H2 Evolution. Appl. Catal. B Environ. 279, 119401. doi:10.1016/j.apcatb.2020.119401
Li, X., Hu, Z., Li, Q., Lei, M., Fan, J., Carabineiro, S. A. C., et al. (2020). Three in One: Atomically Dispersed Na Boosting the Photoreactivity of Carbon Nitride towards NO Oxidation. Chem. Commun. 56, 14195–14198. doi:10.1039/d0cc05948j
Li, Y., Gu, M., Zhang, X., Fan, J., Lv, K., Carabineiro, S. A. C., et al. (2020). 2D G-C3N4 for Advancement of Photo-Generated Carrier Dynamics: Status and Challenges. Mater. Today 41, 270–303. doi:10.1016/j.mattod.2020.09.004
Li, Y., Wang, S., Chang, W., Zhang, L., Wu, Z., Song, S., et al. (2019). Preparation and Enhanced Photocatalytic Performance of Sulfur Doped Terminal-Methylated G-C3N4 Nanosheets with Extended Visible-Light Response. J. Mat. Chem. A 7, 20640–20648. doi:10.1039/c9ta07014a
Li, Z., Yang, Q., Chen, C., Zhang, Z., and Fang, X. (2019). Enhanced Photocatalytic Performance of Polymeric C3N4 Doped with Theobromine Composed of an Imidazole Ring and a Pyrimidine Ring. Chin. J. Catal. 40, 875–885. doi:10.1016/s1872-2067(19)63337-1
Li, Z., Zhou, S., Yang, Q., Zhang, Z., and Fang, X. (2019). Insight into the Enhanced Hydrogen Evolution Activity of 2,4-Diaminopyrimidine-Doped Graphitic Carbon Nitride Photocatalysts. J. Phys. Chem. C 123, 2228–2237. doi:10.1021/acs.jpcc.8b10252
Liebig, J. V. (1834). About Some Nitrogen Compounds. Ann. Pharm. 10, 10. doi:10.1002/jlac.18340100103
Lima, M. J., Sampaio, M. J., Silva, C. G., Silva, A. M. T., and Faria, J. L. (2019). Magnetically Recoverable Fe3O4/g-C3N4 Composite for Photocatalytic Production of Benzaldehyde under UV-LED Radiation. Catal. Today 328, 293–299. doi:10.1016/j.cattod.2018.11.018
Lin, Q., Li, Z., Lin, T., Li, B., Liao, X., Yu, H., et al. (2020). Controlled Preparation of P-Doped G-C3N4 Nanosheets for Efficient Photocatalytic Hydrogen Production. Chin. J. Chem. Eng. Chem. Eng. 28, 2677–2688. doi:10.1016/j.cjche.2020.06.037
Lin, Z., and Wang, X. (2014). Ionic Liquid Promoted Synthesis of Conjugated Carbon Nitride Photocatalysts from Urea. ChemSusChem 7, 1547–1550. doi:10.1002/cssc.201400016
Liu, E., Lin, X., Hong, Y., Yang, L., Luo, B., Shi, W., et al. (2021). Rational Copolymerization Strategy Engineered C Self-Doped G-C3N4 for Efficient and Robust Solar Photocatalytic H2 Evolution. Renew. Energy 178, 757–765. doi:10.1016/j.renene.2021.06.066
Liu, J., Yu, Y., Qi, R., Cao, C., Liu, X., Zheng, Y., et al. (2019). Enhanced Electron Separation on In-Plane Benzene-Ring Doped G-C3N4 Nanosheets for Visible Light Photocatalytic Hydrogen Evolution. Appl. Catal. B Environ. 244, 459–464. doi:10.1016/j.apcatb.2018.11.070
Long, D., Wang, L., Cai, H., Rao, X., and Zhang, Y. (2020). Sulfur Doped Carbon-Rich G-C3N4 for Enhanced Photocatalytic H2 Evolution: Morphology and Crystallinity Effect. Catal. Lett. 150, 2487–2496. doi:10.1007/s10562-020-03156-5
Luo, B., Liu, G., and Wang, L. (2016). Recent Advances in 2D Materials for Photocatalysis. Nanoscale 8, 6904–6920. doi:10.1039/c6nr00546b
Miao, Y., Zhao, Y., Zhang, S., Shi, R., and Zhang, T. (2022). Strain Engineering: A Boosting Strategy for Photocatalysis. Adv. Mater., 2200868. doi:10.1002/adma.202200868
Patnaik, S., Sahoo, D. P., and Parida, K. (2021). Recent Advances in Anion Doped G-C3N4 Photocatalysts: A Review. Carbon 172, 682–711. doi:10.1016/j.carbon.2020.10.073
Qi, M.-Y., Conte, M., Anpo, M., Tang, Z.-R., and Xu, Y.-J. (2021). Cooperative Coupling of Oxidative Organic Synthesis and Hydrogen Production over Semiconductor-Based Photocatalysts. Chem. Rev. 121 (21), 13051–13085. doi:10.1021/acs.chemrev.1c00197
Shevlin, S. A., and Guo, Z. X. (2016). Anionic Dopants for Improved Optical Absorption and Enhanced Photocatalytic Hydrogen Production in Graphitic Carbon Nitride. Chem. Mat. 28, 7250–7256. doi:10.1021/acs.chemmater.6b02002
Sun, S., Li, J., Cui, J., Gou, X., Yang, Q., Jiang, Y., et al. (2019). Simultaneously Engineering K-Doping and Exfoliation into Graphitic Carbon Nitride (G-C3N44) for Enhanced Photocatalytic Hydrogen Production. Int. J. Hydrogen Energy 44, 778–787. doi:10.1016/j.ijhydene.2018.11.019
Tasleem, S., and Tahir, M. (2021). Synergistically Improved Charge Separation in Bimetallic Co-la Modified 3D G-C3N4 for Enhanced Photocatalytic H2 Production under UV-Visible Light. Int. J. Hydrogen Energy 46, 20995–21012. doi:10.1016/j.ijhydene.2021.03.235
Wang, S., and Wang, L. (2019). Recent Progress of Tungsten- and Molybdenum-Based Semiconductor Materials for Solar-Hydrogen Production. Tungsten 1, 19–45. doi:10.1007/s42864-019-00006-9
Wang, X., Maeda, K., Thomas, A., Takanabe, K., Xin, G., Carlsson, J. M., et al. (2009). A Metal-free Polymeric Photocatalyst for Hydrogen Production from Water under Visible Light. Nat. Mater 8, 76–80. doi:10.1038/nmat2317
Wei, L., Zeng, D., Xie, Z., Zeng, Q., Zheng, H., Fujita, T., et al. (2021). NiO Nanosheets Coupled with CdS Nanorods as 2D/1D Heterojunction for Improved Photocatalytic Hydrogen Evolution. Front. Chem. 9, 655583. doi:10.3389/fchem.2021.655583
Wu, C., Xue, S., Qin, Z., Nazari, M., Yang, G., Yue, S., et al. (2021). Making G-C3N4 Ultra-thin Nanosheets Active for Photocatalytic Overall Water Splitting. Appl. Catal. B Environ. 282, 119557. doi:10.1016/j.apcatb.2020.119557
Wu, F., Ma, Y., and Hu, Y. H. (2020). Near Infrared Light-Driven Photoelectrocatalytic Water Splitting over P-Doped G-C3N4. ACS Appl. Energy Mat. 3 (11), 11223–11230. doi:10.1021/acsaem.0c02148
Xiao, M., Jiao, Y., Luo, B., Wang, S., Chen, P., Lyu, M., et al. (2021). Understanding the Roles of Carbon in Carbon/g-C3N4 Based Photocatalysts for H2 Evolution. Nano Res.. doi:10.1007/s12274-021-3897-7
Xing, Y., Wang, X., Hao, S., Zhang, X., Wang, X., Ma, W., et al. (2021). Recent Advances in the Improvement of G-C3N4 Based Photocatalytic Materials. Chin. Chem. Lett. 32, 13–20. doi:10.1016/j.cclet.2020.11.011
Xiong, T., Cen, W., Zhang, Y., and Dong, F. (2016). Bridging the G-C3N4 Interlayers for Enhanced Photocatalysis. ACS Catal. 6 (4), 2462–2472. doi:10.1021/acscatal.5b02922
Xu, J., Gao, Q., Wang, Z., and Zhu, Y. (2021). An All-Organic 0D/2D Supramolecular Porphyrin/g-C3n4 Heterojunction Assembled via π-π Interaction for Efficient Visible Photocatalytic Oxidation. Appl. Catal. B Environ. 291, 120059. doi:10.1016/j.apcatb.2021.120059
Yan, J., Liu, J., Sun, Y., Ding, D., Wang, C., Sun, L., et al. (2022). Exfoliation-induced O-Doped G-C3N4 Nanosheets with Improved Photoreactivity towards RhB Degradation and H2 Evolution. Inorg. Chem. Front. 9, 1423–1433. doi:10.1039/d1qi01625c
Yang, C., Tan, Q., Li, Q., Zhou, J., Fan, J., Li, B., et al. (2020). 2D/2D Ti3C2 MXene/g-C3n4 Nanosheets Heterojunction for High Efficient CO2 Reduction Photocatalyst: Dual Effects of Urea. Appl. Catal. B Environ. 268, 118738. doi:10.1016/j.apcatb.2020.118738
Yang, C., Wang, B., Zhang, L., Yin, L., and Wang, X. (2017). Synthesis of Layered Carbonitrides from Biotic Molecules for Photoredox Transformations. Angew. Chem. 129, 6727–6731. doi:10.1002/ange.201702213
Yang, C., Zhang, S., Huang, Y., Lv, K., Fang, S., Wu, X., et al. (2020). Sharply Increasing the Visible Photoreactivity of G-C3n4 by Breaking the Intralayered Hydrogen Bonds. Appl. Surf. Sci. 505, 144654. doi:10.1016/j.apsusc.2019.144654
Yang, H., Zhou, Y., Wang, Y., Hu, S., Wang, B., Liao, Q., et al. (2018). Three-dimensional Flower-like Phosphorus-Doped G-C3n4 with a High Surface Area for Visible-Light Photocatalytic Hydrogen Evolution. J. Mat. Chem. A 6, 16485–16494. doi:10.1039/c8ta05723k
Yang, X., Tian, L., Zhao, X., Tang, H., Liu, Q., and Li, G. (2019). Interfacial Optimization of G-C3n4-Based Z-Scheme Heterojunction toward Synergistic Enhancement of Solar-Driven Photocatalytic Oxygen Evolution. Appl. Catal. B Environ. 244, 240–249. doi:10.1016/j.apcatb.2018.11.056
Yang, X., Tian, Z., Chen, Y., Huang, H., Hu, J., and Wen, B. (2021). In Situ synthesis of 2D Ultrathin Cobalt Doped G-C3n4 Nanosheets Enhances Photocatalytic Performance by Accelerating Charge Transfer. J. Alloys Compd. 859, 157754. doi:10.1016/j.jallcom.2020.157754
Yu, H., Ma, H., Wu, X., Wang, X., Fan, J., and Yu, J. (2021). One‐Step Realization of Crystallization and Cyano‐Group Generation for g‐C3 N4 Photocatalysts with Improved H2 Production. Sol. RRL 5, 2000372. doi:10.1002/solr.202000372
Zada, A., Khan, M., Qureshi, M. N., Liu, S.-y., and Wang, R. (2020). Accelerating Photocatalytic Hydrogen Production and Pollutant Degradation by Functionalizing G-C3n4 with SnO2. Front. Chem. 7, 941. doi:10.3389/fchem.2019.00941
Zhao, B., Gao, D., Liu, Y., Fan, J., and Yu, H. (2022). Cyano Group-Enriched Crystalline Graphitic Carbon Nitride Photocatalyst: Ethyl Acetate-Induced Improved Ordered Structure and Efficient Hydrogen-Evolution Activity. J. Colloid Interface Sci. 608, 1268–1277. doi:10.1016/j.jcis.2021.10.108
Zhao, D., Chen, J., Dong, C.-L., Zhou, W., Huang, Y.-C., Mao, S. S., et al. (2017). Interlayer Interaction in Ultrathin Nanosheets of Graphitic Carbon Nitride for Efficient Photocatalytic Hydrogen Evolution. J. Catal. 352, 491–497. doi:10.1016/j.jcat.2017.06.020
Zhao, D., Dong, C. L., Wang, B., Chen, C., Huang, Y. C., Diao, Z., et al. (2019). Synergy of Dopants and Defects in Graphitic Carbon Nitride with Exceptionally Modulated Band Structures for Efficient Photocatalytic Oxygen Evolution. Adv. Mat. 31, 1903545. doi:10.1002/adma.201903545
Zhao, S., Zhang, Y., Wang, Y., Zhou, Y., Qiu, K., Zhang, C., et al. (2017). Ionic Liquid-Assisted Synthesis of Br-Modified G-C3 N4 Semiconductors with High Surface Area and Highly Porous Structure for Photoredox Water Splitting. J. Power Sources 370, 106–113. doi:10.1016/j.jpowsour.2017.10.023
Zhao, Z., Xie, C., Cui, H., Wang, Q., Shu, Z., Zhou, J., et al. (2020). Scalable One-Pot Synthesis of Phosphorus-Doped G-C3N4 Nanosheets for Enhanced Visible-Light Photocatalytic Hydrogen Evolution. Diam. Relat. Mater. 104, 107734. doi:10.1016/j.diamond.2020.107734
Zhou, L., Hu, K., Dong, T., Wang, Q., Huang, H., Lu, C., et al. (2021). Carbon Ring and Molecular Scaffold Co-doped G-C3N4 Heterostructural Nanosheets for Highly Efficient Hydrogen Evolution. Mater. Res. Bull. 144, 111482. doi:10.1016/j.materresbull.2021.111482
Zhu, B., Cheng, B., Fan, J., Ho, W., and Yu, J. (2021). G‐C3N4 ‐Based 2D/2D Composite Heterojunction Photocatalyst. Small Struct. 2, 2100086. doi:10.1002/sstr.202100086
Keywords: doping, G-C3N4, photoadsorption, band structure, water splitting
Citation: Yang Y, Niu W, Dang L, Mao Y, Wu J and Xu K (2022) Recent Progress in Doped g-C3N4 Photocatalyst for Solar Water Splitting: A Review. Front. Chem. 10:955065. doi: 10.3389/fchem.2022.955065
Received: 28 May 2022; Accepted: 09 June 2022;
Published: 13 July 2022.
Edited by:
Kangle Lv, South-Central University for Nationalities, ChinaCopyright © 2022 Yang, Niu, Dang, Mao, Wu and Xu. This is an open-access article distributed under the terms of the Creative Commons Attribution License (CC BY). The use, distribution or reproduction in other forums is permitted, provided the original author(s) and the copyright owner(s) are credited and that the original publication in this journal is cited, in accordance with accepted academic practice. No use, distribution or reproduction is permitted which does not comply with these terms.
*Correspondence: Yilong Yang, eWx5YW5nQGhuY2ouZWR1LmNu; Kaidong Xu, MzAwMTA5MDhAaG5jai5lZHUuY24=