- Key Laboratory of Natural Medicines of the Changbai Mountain, Affifiliated Ministry of Education, College of Pharmacy, Yanbian University, Jilin, China
A series of quillaic acid derivatives with different substituents on the 28-carboxyl group were designed and synthesized. Five human cancer cell lines (HCT116, BEL7402, HepG2, SW620, and MCF-7) were evaluated for their antitumor activity in vitro. Some of the tested derivatives showed improved antiproliferative activity compared to the lead compound, quillaic acid. Among them, compound E (IC50 = 2.46 ± 0.44 μM) showed the strongest antiproliferative activity against HCT116 cells; compared with quillaic acid (IC50 > 10 μM), its efficacy against HCT116 cancer cells was approximately 4-fold higher than that of quillaic acid. Compound E also induces cell cycle arrest and apoptosis by modulating NF-κB and MAPK pathways. Therefore, the development of compound E is certainly valuable for anti-tumor applications.
1 Introduction
Malignant tumors, also known as cancers, have become a disease that threatens human health (Bray et al., 2018). Drug therapy remains an effective and important method for the clinical treatment of cancer. One of the main obstacles to the pharmacological properties of compounds with effective anti-tumor activity is their toxicity to normal cells. Therefore, it is of great importance to develop new anti-cancer drugs that are less toxic to normal cells (Shen et al., 2019).
In recent years, the discovery of natural plant-derived antitumor drugs has attracted significant attention from medicinal chemists. All approved therapeutic drugs from 1981 to 2019 (39 years) and corresponding diseases from 1946 to 2019, a total of 1,881 drugs were reported. Among them, 71 (3.8%) were natural products, 14 (0.8%) were natural products’ “Botanical” (in general these have been approved), and 356 (18.9%) were from semi-synthetic modifications of natural products (Newman and Cragg 2020). Natural products remain the best source of effective drugs for treating human diseases.
Saponaria officinalis L. (Caryophyllaceae), commonly known as soap weed, is native to Europe and Asia, and is grown as a horticultural plant worldwide. Quillaic acid (Figure 1A), which is isolated from this plant, is a pentacyclic triterpenoid (Lu et al., 2015).
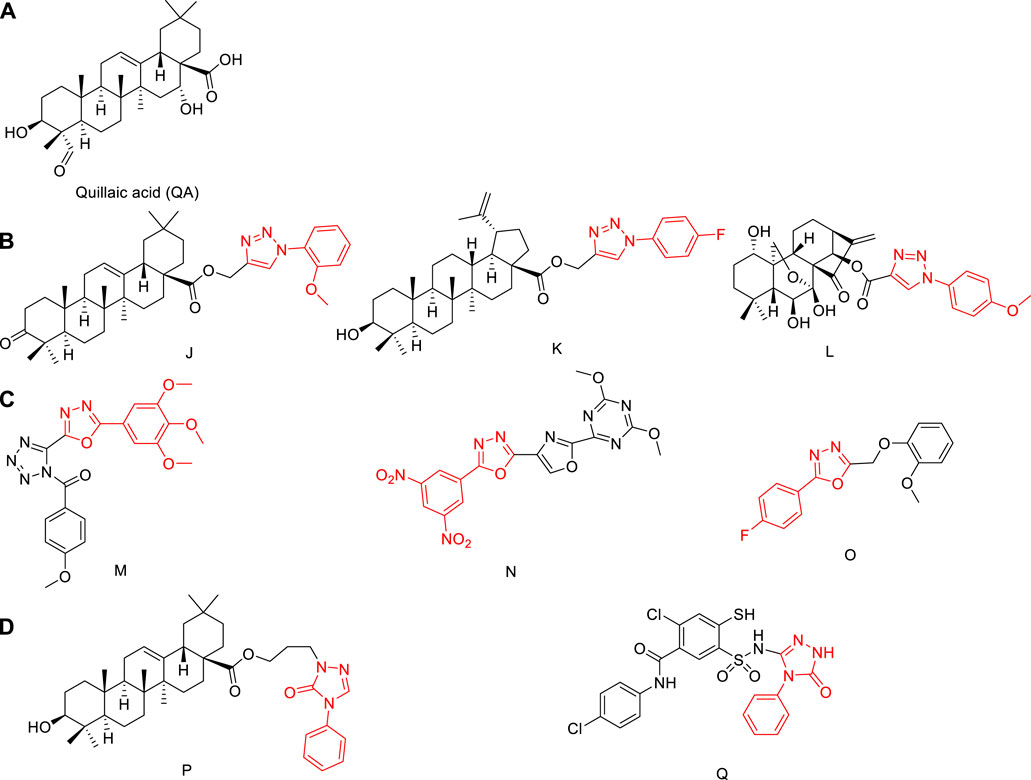
FIGURE 1. (A) Structures of quillaic acid. (B) Structures of natural-product derivatives containing phenyl-1,2,3-triazole; (C) Structures of compounds containing phenyl-1,3,4-oxadiazole; (D) Structures of compounds containing 1,2,4-triazol-3-one.
Quillaic acid has a variety of biological activities, including anti-inflammatory (Rodriguez-Diaz et al., 2011), immune-stimulatory (Moses et al., 2014), anti-viral (Boke Sarikahya et al., 2021), and cytotoxic (Guzman et al., 2020). Quillaic acid is a well-known cytotoxic triterpenoid that has demonstrated apoptosis-inducing ability in diverse cells (Yazici et al., 2021).
Phenyl-1,2,3-triazole has a high dipole moment and can form hydrogen bonds with drug targets, which is conducive to the binding of the compound to the target (Bock et al., 2007), Triazole is an important heterocyclic structural unit, used in anticonvulsant effect (Liu et al., 2017; Huang et al., 2018; Shao et al., 2018; Zhang G. R. et al., 2018), antibacterial (Yan et al., 2021; Zhang et al., 2021), anti-inflammatory (Pang et al., 2020; Ma et al., 2022; Zhang et al., 2022), anti-cancer (Liu et al., 2021), and anti-toxoplasmic (Zhang H. B. et al., 2018; Shang et al., 2021) applications, among others.
A biological allele of amides and esters, 1,3,4-oxadiazole can affect the pharmacokinetic properties of a drug by increasing its lipophilicity, thereby improving the ability of the drug to diffuse across a membrane (Bajaj et al., 2015). One of four isomers of oxadiazole, 1,3,4-oxadiazole also has antibacterial (Wang et al., 2021), antitumor (Zhao et al., 2021), and other biological properties.
Fragments of phenyl-1,2,3-triazole or phenyl-1,3,4-oxadiazole molecules are sometimes combined with natural products to improve their anti-tumor activities (Chi et al., 2017; Shen et al., 2019). The synthetic strategies of compounds J, K, L, M, N, and O in Figure 1B and Figure 1C have attracted increasing attention (Rashid et al., 2013; Khan et al., 2016; Lakshmithendral et al., 2019; Shen et al., 2019; Kotla et al., 2020; Balaraju et al., 2021). Among them, compounds J and K were modified at the carboxyl position and linked to phenyl-1,2,3-triazole with an ester bond, and the antitumor activity was significantly enhanced. Compound L is also linked by an ester bond, and also obtains better antitumor activity. Therefore, combining a phenyl-1,2,3-triazole group or phenyl-1,3,4-oxadiazole with quillaic acid through an ester bond is an effective strategy to enhance the antitumor activity of quillaic acid.
3H-1,2,4-triazol-3-one also has a wide range of applications in anti-fungal (Sheng et al., 2011) and anti-inflammatory (Wei et al., 2018) fields. Compounds P and Q in Figure 1D showed significant anti-tumor activity (Chi et al., 2017; Zhang H. J. et al., 2018). Therefore, combining 3H-1,2,4-triazol-3-one with quillaic acid through an ester bond enhanced the antitumor activity of quillaic acid.
In this study, quillaic acid was selected as the lead compound, and phenyl-1,2,3-triazole, phenyl-1,3,4-oxadiazole, and 3H-1,2,4-triazol-3-one were introduced into the C-28 carboxyl group of quillaic acid. Thereby, we designed and synthesized different phenyl-1,2,3-triazoles (A1–10), phenyl-1,3,4-oxadiazoles (B1–B6), 3H-1,2,4-triazol-3-ones (C1–C6).
Compounds D and E were designed and synthesized according to an alternative design with an isoxazole or tetrazole ring because of their similar physicochemical and biological properties to the 1,3,4-oxadiazole and 1,2,4-triazole-3-one rings, respectively (Figure 2). Subsequently, all 24 new compounds were screened against five different cancer cell lines. The toxicity of the compounds in normal human liver L02 cells was also tested.
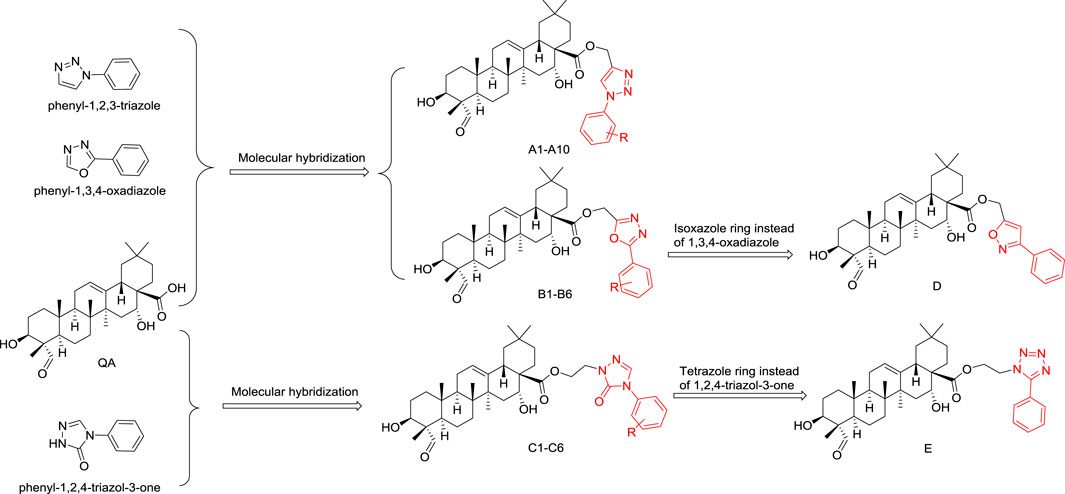
FIGURE 2. Designing quillaic-acid derivatives containing a nitrogen-containing heterocycle to achieve potential anti-proliferative activity.
2 Results and discussion
2.1 Chemistry
The synthesis of the intermediates is shown in Scheme 1. Compounds 1a–1j were obtained by diazotization and azide reactions of various anilines (Zhao et al., 2019). Then, a click reaction between intermediates 1a–1j and propynol gave compounds 2a–2j (Luan et al., 2019). Compounds 2a–2j were mixed with thionyl chloride in CH2Cl2 at room temperature to give compounds 3a–3j (Ghosh et al., 2012).
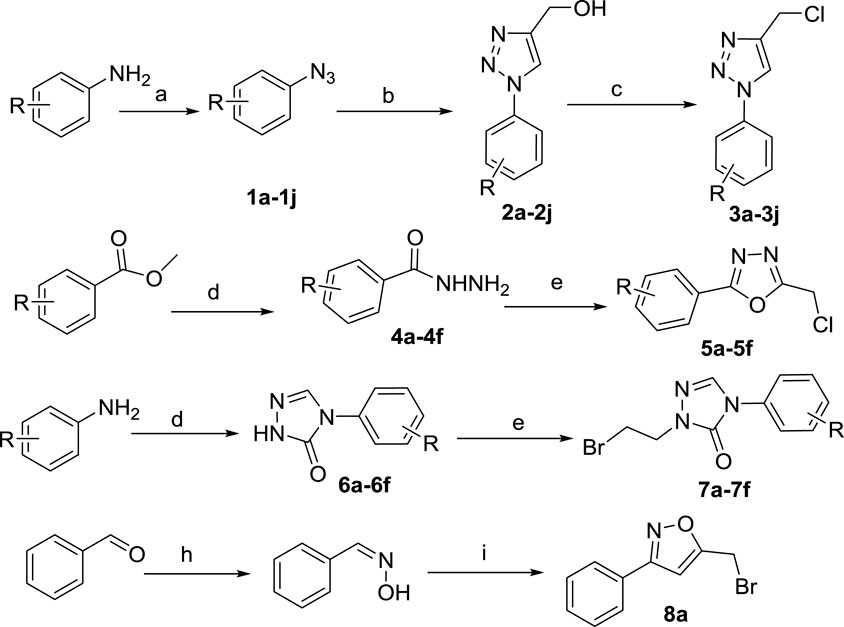
SCHEME 1. Reagents and conditions: (A) (i) HCl, NaNO2, H2O, 0–5°C, 30 min; (ii) NaN3, H2O, 0–5°C, 2–4 h; (B) propynol, Na ascorbate, CuSO4•5H2O, H2O, t-BuOH/H2O, 25°C, overnight; (C) thionyl chloride, CH2Cl2, r. t., overnight; (D) NH2-NH2•H2O, EtOH, reflux, overnight; (E) chloroacetic acid, POCl3, reflux, 4 h; (F) (I) methyl hydrazinocarboxylate, triethyl orthoformate, EtOH, reflux, 48 h; (ii) NaOCH3, EtOH, reflux, 48 h; (G) 1,2-dibromoethane, DMF, K2CO3, 60°C, 4–6 h; (H) NH2-OH•HCl, K2CO3, CH3OH:H2O = 1:1, r. t., overnight; (I) NaClO, CH2Cl2, H2O, 0°C, 8 h.
The substituted methyl benzoate reacted with hydrazine hydrate to obtain 4a–4f, which were then closed with chloroacetic acid under the action of phosphorus oxychloride to obtain 5a–5f (Sheng et al., 2011). Aniline reacted with methyl carbazate and triethyl orthoformate; it was then catalyzed by sodium methoxide to obtain 6a-6f, which reacted with dibromoethane to obtain the intermediates 7a-7f (Wei et al., 2017). Benzaldehyde reacts with hydroxylamine hydrochloride and then reacts with sodium hypochlorite solution to obtain 8a (Liu et al., 2010).
The general path to synthesize the target quillaic acid analogues A1–A10, B1–B6, C1–C6, D, and E is shown in Scheme 2. Quillaic acid was used as the raw material, and K2CO3 as the catalyst, to react with different intermediates (3a-3j, 5a-5f, 7a-7f, 8a) in anhydrous DMF at 60°C to obtain the target compounds A1–A10, B1–B6, C1–C6, and D (Chi et al., 2017; Wei et al., 2018). Quillaic acid first reacted with dibromoethane and then reacted with 5-phenyl-1H-tetrazole with K2CO3, a KI catalyst, at 60°C in anhydrous DMF to obtain the target compound E (Zhang et al., 2019). All synthesized compounds were characterized by 1H-NMR, 13C-NMR, and high-resolution mass spectrometry.
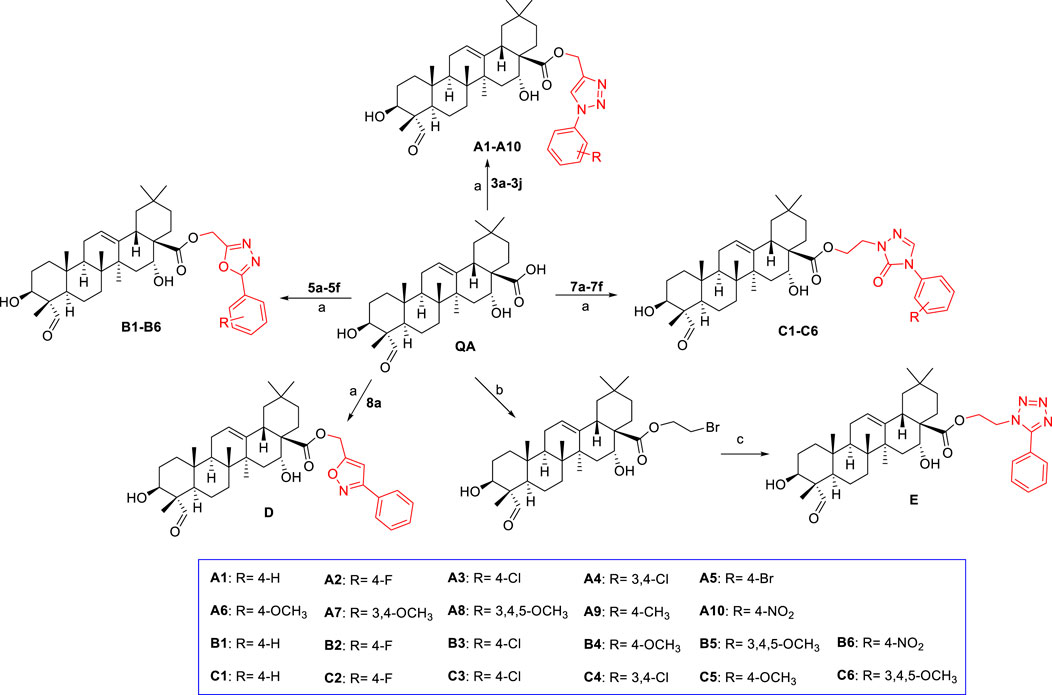
SCHEME 2. Reagents and conditions: (A) DMF, K2CO3, 60°C, 4–6 h; (B) 1,2-dibromoethane, DMF, K2CO3, KI, 60°C, 4–6 h; (C) 5-phenyl-1H-tetrazole, DMF, K2CO3, KI, 60°C, 8 h.
2.2 Biological evaluation
2.2.1 In vitro anti-proliferative activity and SAR study
The anti-proliferative activities against five human cancer cell lines, including HCT116 (human colorectal cancer cell line), MCF-7 (human breast cancer cell line), SW620 (human colorectal cancer cell line), BEL7402 (human hepatocellular carcinoma cell line), and HepG2 (human hepatocellular carcinoma cell line) were established for all the target compounds using the MTT assay. The IC50 values (concentration required to inhibit tumor cell proliferation by 50%) are listed in Table 1.
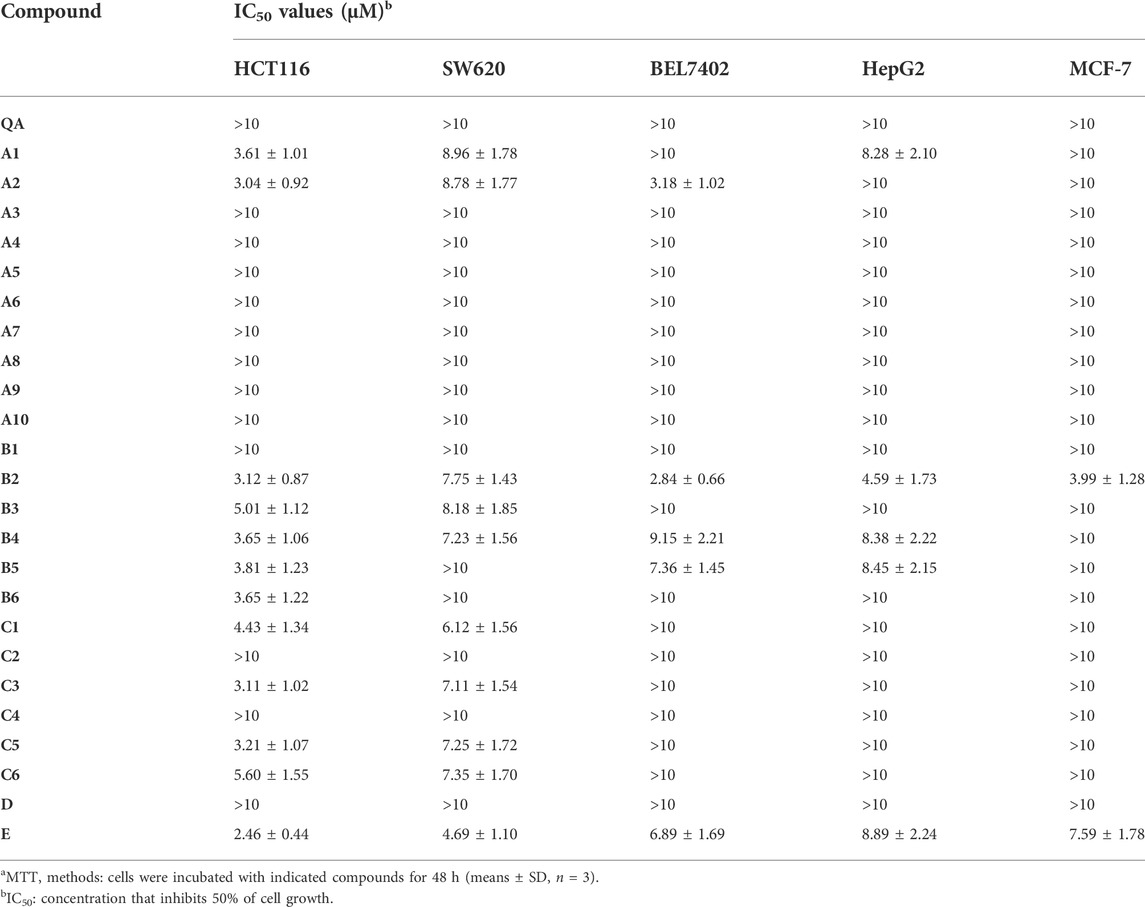
TABLE 1. Antiproliferative efficacy of quillaic acid derivatives of compounds A1- A10, B1-B6, C1-C6, D, and E in three human cancer cell linesa.
The IC50 of quillaic acid against five types of cancer cells was greater than 10 μM. Quillaic acid derivatives A1 and A2 containing different phenyl 1,2,3-triazoles exhibited stronger anti-proliferative activities against all five selected cancer cell lines than quillaic acid. Among them, compound A2 with 4-fluorophenyl 1,2,3-triazole was the most potent compound in the series, having an IC50 value of 3.04 μM in the HCT116 cell line.
Compounds B2–B5, with different phenyl-1,3,4-oxadiazoles, were slightly more potent against HCT116, SW620, MCF-7, HepG2, and BEL7402 cells than quillaic acid. Among them, compound B2 (with 4-fluorophenyl-1,2,3-triazole) was the most potent compound in this series with an IC50 value of 3.12 μM against the HCT116 cell line and 2.84 μM against the BEL7402 cell line. In order to explore the anti-proliferative activity of the phenyl-1,2,3-triazole group, we designed and synthesized compound D by replacing it with isoxazole. Unfortunately, compound D had no strong activity against the five types of cancer cells. The order of activity was 4-F > 4-NO2 > 3,4,5-OCH3 > 4-OCH3 > 4-Cl.
Compounds C1, C3, C5, and C6, with different phenyl-1,2,4-triazol-3-ones, were slightly more potent against HCT116 and SW620 cells than quillaic acid. Compound C3 (with 4-chlorophenyl-1,2,4-triazol-3-one) was the most potent compound in this series with an IC50 value of 3.11 μM against the HCT116 cell line. The order of activity was 4-Cl > 4-OCH3 > 4-H > 3,4,5-OCH3. In order to explore the importance of the phenyl-1,2,4-triazol-3-one group for anti-proliferative activity, we designed and synthesized compound E by replacing it with tetrazole. Fortunately, compound E had strong activity against five types of cancer cells. Compound E had the strongest activity against HCT116 cells, with an IC50 of 2.46 μM, followed by SW620 with an IC50 of 4.69 μM.
2.2.2 Selective inhibition of cancer-cell growth by compounds B2, and E in vitro
The lack of selectivity between normal and cancer cells is one of the main limitations of antitumor drugs (Dy and Adjei, 2013). Therefore, we evaluated the cytotoxicity of compounds B2, and E in normal cell lines L02 (human normal liver cells) to determine the selectivity index (ratio of cytotoxicity in L02 cells compared to that in cancer cells). As shown in Table 2, compound E exhibited a 4.06-fold higher selectivity for HCT116 cells than for normal L02 cells; compound E not only exhibited the strongest anti-proliferative activity against HCT116 cells, but selectively inhibited tumor cells. Therefore, this compound was chosen for further biological studies.

TABLE 2. In vitro cytotoxic of compounds B2 and F against normal cell line (L02)a.
2.2.3 Compound E inhibited HCT116 cell colony formation
The colony-forming cell assay was used to determine the ability of cells to proliferate and differentiate into colonies and thereby to investigate the anti-proliferative efficacy of compound E (Lei et al., 2015). As shown in Figure 3, exposure of HCT116 cells to compound E significantly decreased the number and size of colonies in a concentration-dependent manner.
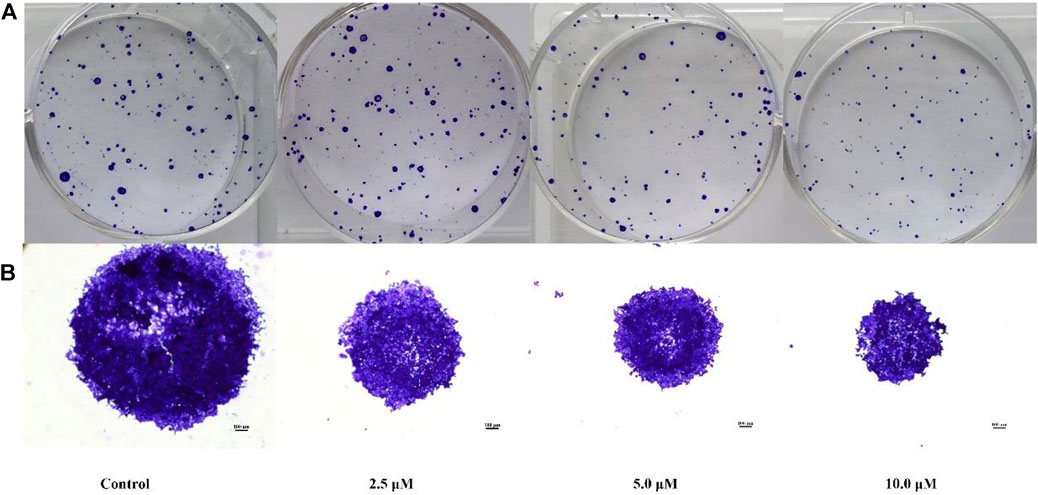
FIGURE 3. Colony formation of HCT116 cells inhibited by compound E. (A) HCT116 cells were incubated with varying concentrations of E (0, 2.5, 5, 10 μM) and stained with crystal violet. (B) The micrographic differences between the colonies. Images were taken of stained single colonies observed under a microscope.
2.2.4 Compound E induced cell-cycle arrest
To determine the effect of compound E on cell-cycle progression, flow cytometry analysis was performed on HCT116 cells treated with different concentrations (10 and 30 µM) of compound E. A dose-dependent G1 phase-transition arrest was observed in HCT116 cells treated with E, as shown in Figure 4. Treatment of HCT116 cells with compound E at a concentration of 30 μM resulted in 81.2% of cells arrested in the G1 phase, compared with 53.9% in the untreated group.
2.2.5 Compound E induced morphological changes in HCT116 cells by haematoxylin and eosin staining
Induction of apoptosis is the mechanism of action of many anti-proliferative drugs. When cells undergo apoptosis, they generally also undergo morphological changes (Liu et al., 2009). Therefore, we used H&E staining to assess morphological changes in the cells. As shown in Figure 5, HCT116 cells incubated with E for 24 h displayed significant apoptotic morphological changes.
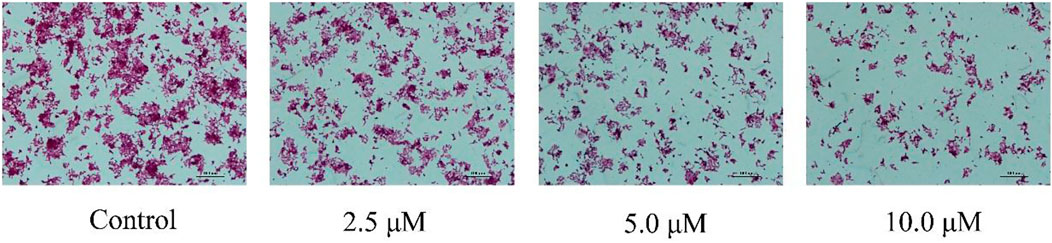
FIGURE 5. Cell morphological alterations and nuclear changes associated with HCT116 cells after incubation with varying concentrations E (0, 2.5, 5, 10 μM) for 24 h were assessed by staining with H&E and visualized by microscopy.
2.2.6 Compound E induced cancer-cell apoptosis
To confirm that E induces apoptosis in HCT116 cells, HCT116 cells were treated with different concentrations (10 and 30 µM) of compound E. After treatment with compound E, the apoptosis rate of HCT116 cells increased from 5.28% (control) to 14.76%, as shown in Figure 6. This result indicated that compound E induced apoptosis.
2.2.7 Western-blot analysis of the expression of Bax, and Bcl-2
The Bcl-2 protein family (including the anti-apoptotic protein Bcl-2 and the pro-apoptotic protein Bax) is a group of key apoptotic proteins that play an important role in the regulation of apoptosis (Han et al., 2013; Zhang and Saghatelian, 2013; Zheng et al., 2021). HCT116 cells were treated with different concentrations (2.5, 5, and 10 μM) of E to detect the expression of Bax and Bcl-2 proteins. The addition of 10 µM of compound E significantly increased the expression of Bax and decreased the level of Bcl-2, as shown in Figure 7.
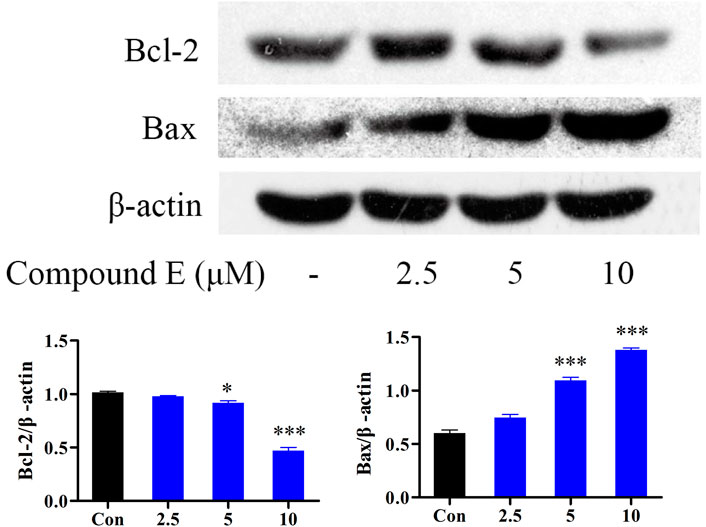
FIGURE 7. Effect of compound E on the levels of Bax, and Bcl-2 in HCT116 cells. Cells were pretreated with the various concentrations of compound E (2.5, 5 and 10 μM) for 24 h. The expression of Bax, and Bcl-2 were analyzed by Western blot. ***p < 0.001, **p < 0.01, *p < 0.05 vs. control group. The error bars represent the mean ± SD for three independent experiments.
2.2.8 Effect of compound E on NF-κB signaling pathway in HCT116 cells
The NF-κB pathway plays an important role in a variety of cellular functions, including cell growth, apoptosis, and tumorigenesis (Hwang et al., 2012). Interfering with NF-κB signaling can inhibit tumor cell proliferation and metastasis (Yenmis et al., 2021). Therefore, NF-κB inhibitors are considered as potential anticancer drugs (Wang et al., 2017). The results showed that compound E inhibited the phosphorylation and degradation of IκB (Figure 8), but had no inhibitory effect on NF-κB p65.
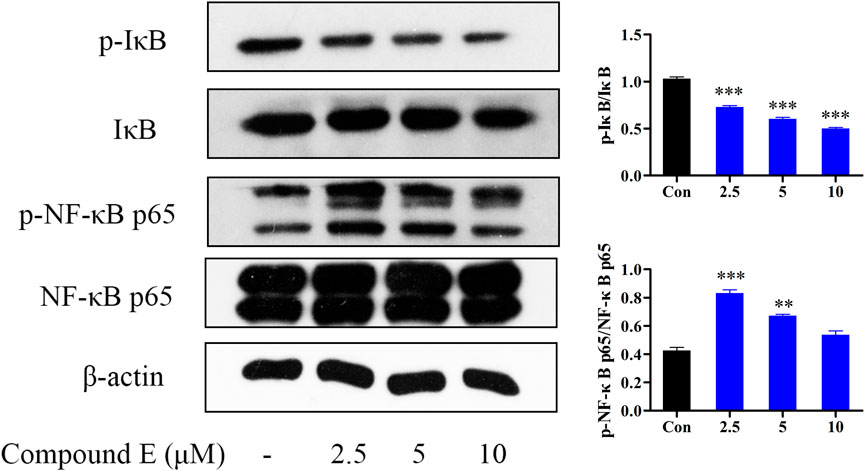
FIGURE 8. Effect of compound E on NF-κB signaling pathway in HCT116 cells. Cells were pretreated with the various concentrations of compound E (2.5, 5 and 10 μM) for 24 h. The expression of phospho-NF-κB p65, NF-κB p65, phospho-IκB, IκB were analyzed by Western blot. ***p < 0.001, **p < 0.01, *p < 0.05 vs. control group. The error bars represent the mean ± SD for three independent experiments.
2.2.9 Effect of compound E on MAPK signaling pathway in HCT116 cells
Extracellular signal-regulated kinases are the most closely related MAPK. MAPK, a family of serine/threonine protein kinases, is also an important transmitter of signaling from the cell surface to the nucleus, and it is involved in the regulation of many physiological processes, including apoptosis (Bian et al., 2021). The MAPK pathway has been implicated in regulating tumor angiogenesis, proliferation, metastasis and invasion (Ko et al., 2017). MAPK family is mainly composed of ERK, p38 and JNK subfamily. The results showed that compound E down-regulated the phosphorylation levels of ERK, JNK and p38 at 10 μM (Figure 9).
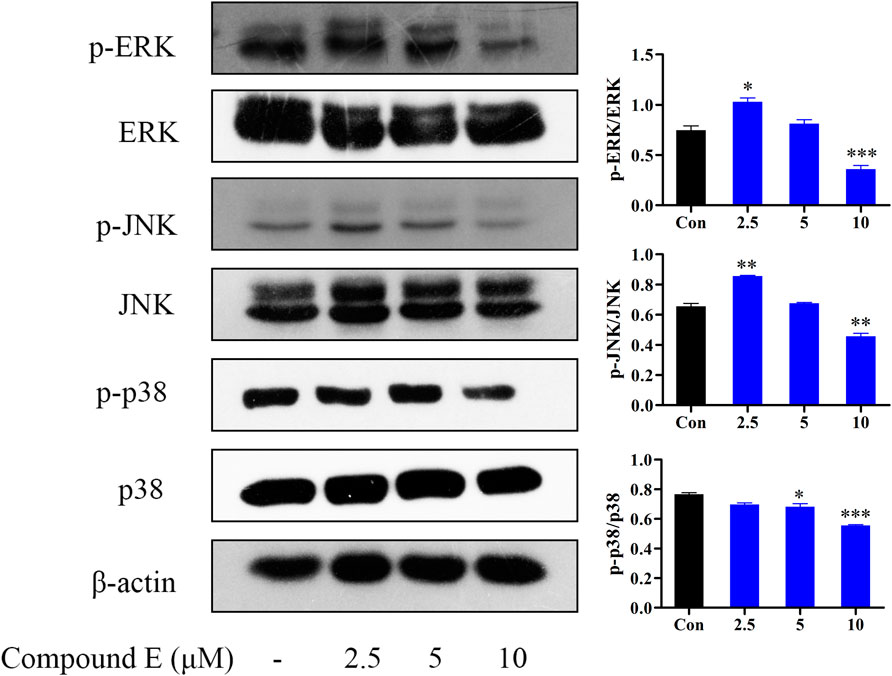
FIGURE 9. Effect of compound E on NF-κB signaling pathway in HCT116 cells. Cells were pretreated with the various concentrations of compound E (2.5, 5 and 10 μM) for 24 h. The expression of phospho-ERK, ERK, phospho-JNK, JNK, phospho-p38, p38 were analyzed by Western blot. ***p < 0.001, **p < 0.01, *p < 0.05 vs. control group. The error bars represent the mean ± SD for three independent experiments.
3 Conclusion
In summary, a new type of saponin derivative was designed, synthesized, and tested for its antitumor activity. The in vitro cell-growth inhibition test showed that compound E had the highest anti-proliferative activity against HCT116 cells (IC50 = 2.46 μM). The results of flow cytometry showed that compound E induced cell apoptosis and blocked cells in the G1 phase. Compound E induced HCT116 cell apoptosis by increasing the expression of Bax and decreasing the level of Bcl-2. Compound E also exerted its antitumor activity through NF-κB signaling pathway and MAPK signaling pathway. Therefore, compound E may be a potential anti-cancer drug and has value for further research.
4 Experimental section
4.1 Chemistry
All reagents and solvents were purchased from commercial sources and used as received without further purification. Reactions were monitored by thin-layer chromatography (TLC) in silica gel, and the TLC plates were visualised by exposure to ultraviolet light (254 and 365 nm). Compounds were purified using flash column chromatography over silica gel (200–300 mesh). Melting points were determined in open capillary tubes and are uncorrected. 1H-NMR and 13C-NMR spectra were measured on Bruker AV-300 and AV-500 spectrometer, with TMS as internal standard. Mass spectra were obtained on an HP1100LC (Agilent Technologies, USA).
4.2 General procedure for synthesis of compounds A1-A10, B1-B6, C1-C6, and D
A 25 ml round bottomed flask was charged with quillaic acid (50 mg, 0.10 mmol), intermediates 3a-3j, 5a-5f, 7a-7f, and 8a (0.12 mmol), K2CO3 (41.4 mg, 0.30 mmol) and DMF (5 ml). This reaction mixture was stirred vigorously at 60°C for 6 h and poured into the crushed ice. The mixture was extracted with ethyl acetate and the organic phase was washed with saturated sodium bicarbonate solution and brine, and dried over Na2SO4. The evaporation of the solvents gave the crude products, which were purified by silica gel column to afford compounds A1-A10, B1-B6, C1-C6, and D.
4.2.1 (1-phenyl-1H-1,2,3-triazol-4-yl)methyl (4aR,5R,6aS,6bR,8aR,9S,10S,12aR,12bR,14bS)-9-formyl-5,10-dihydroxy-2,2,6a,6b,9,12a-hexamethyl-1,3,4,5,6,6a,6b,7,8,8a,9,10,11,12,12a,12b,13,14b-octadecahydropicene-4a (2H)-carboxylate (A1)
White solid; Yield: 79%; mp: 186–188°C; Purity: 95.72%; 1H-NMR (300 MHz, CDCl3) δ: 9.40 (s, 1H, -CHO), 8.02 (s, 1H, triazole-H), 7.75–7.71 (m, 2H, Ar-H), 7.58–7.53 (m, 2H, Ar-H), 7.50–7.45 (m, 1H, Ar-H), 5.40 (s, 1H, C12-H), 5.26 (dd, J1 = 18 Hz, J2 = 12 Hz, 2H, -COO-CH2-), 4.58 (s, 1H, C16-H), 3.78 (d, J = 9 Hz, 1H, C3-H), 3.08 (dd, J1 = 15 Hz, J2 = 6 Hz, 1H, C18-H), 2.18 (t, J = 15 Hz, 1H), 1.94–1.84 (m, 3H), 1.78 (d, J = 6 Hz, 3H), 1.73–1.56 (m, 7H), 1.49–1.26 (m, 9H), 1.24–1.10 (m, 3H), 1.04 (s, 3H), 0.98 (s, 3H), 0.92 (s, 3H), 0.83 (s, 3H), 0.46 (s, 3H). 13C-NMR (75 MHz, CDCl3) δ: 207.09, 176.71, 142.81, 136.85, 129.81, 128.96, 122.63, 122.49, 120.48, 74.66, 71.82, 57.64, 55.21, 48.65, 48.16, 46.53, 46.38, 41.39, 40.68, 39.76, 38.07, 35.92, 35.47, 32.78, 32.26, 31.01, 30.43, 26.87, 26.12, 24.52, 23.21, 20.66, 16.78, 15.56, 8.94. ESI-HRMS calcd for C39H54N3O5+ ([M + H]+): 644.40580; found: 644.40446.
4.2.2 (1-(4-fluorophenyl)-1H-1,2,3-triazol-4-yl)methyl (4aR,5R,6aS,6bR,8aR,9S,10S,12aR,12bR,14bS)-9-formyl-5,10-dihydroxy-2,2,6a,6b,9,12a-hexamethyl-1,3,4,5,6,6a,6b,7,8,8a,9,10,11,12,12a,12b,13,14b-octadecahydropicene-4a (2H)-carboxylate (A2)
White solid; Yield: 67%; mp: 171–172°C; Purity: 94.65%; 1H-NMR (300 MHz, CDCl3) δ: 9.40 (s, 1H, -CHO), 7.98 (s, 1H, triazole-H), 7.73–7.69 (m, 2H, Ar-H), 7.24 (d, J = 9 Hz, 2H, Ar-H), 5.39 (s, 1H, C12-H), 5.24 (t, J = 15 Hz, 2H, -COO-CH2-), 4.57 (s, 1H, C16-H), 3.78 (d, J = 9 Hz, 1H, C3-H), 3.06 (d, J = 12 Hz, 1H, C18-H), 2.18 (t, J = 15 Hz, 1H), 1.87 (t, J = 15 Hz, 3H), 1.77 (s, 1H), 1.66 (s, 6H), 1.49 (t, J = 12 Hz, 2H), 1.36 (s, 3H), 1.28 (s, 2H), 1.20–1.14 (m, 3H), 1.11 (t, J = 3 Hz, 1H), 1.05 (s, 3H), 0.97 (s, 3H), 0.91 (s, 3H), 0.89 (s, 1H), 0.87 (s, 2H), 0.85 (s, 3H), 0.46 (s, 3H). 13C-NMR (75 MHz, CDCl3) δ: 207.50, 176.09, 162.09 (d, J = 244.5 Hz), 143.74, 143.47, 133.50, 123.90, 122.66 (d, J = 9.0 Hz), 121.91, 117.14 (d, J = 23.25 Hz), 86.44, 73.09, 57.23, 55.51, 48.20, 46.69, 41.37, 37.51, 35.68, 35.40, 34.96, 33.25, 31.66, 30.63, 26.81, 24.58, 16.71, 15.51, 15.12, 13.72, 9.24. ESI-HRMS calcd for C39H52N3O5F+ ([M + H]+): 662.39638; found: 662.39465.
4.2.3 (1-(4-chlorophenyl)-1H-1,2,3-triazol-4-yl)methyl (4aR,5R,6aS,6bR,8aR,9S,10S,12aR,12bR,14bS)-9-formyl-5,10-dihydroxy-2,2,6a,6b,9,12a-hexamethyl-1,3,4,5,6,6a,6b,7,8,8a,9,10,11,12,12a,12b,13,14b-octadecahydropicene-4a (2H)-carboxylate (A3)
White solid; Yield: 67%; mp: 193–194°C; 1H-NMR (300 MHz, DMSO-d6) δ: 9.20 (s, 1H, -CHO), 8.81 (s, 1H, triazole-H), 7.95 (d, J = 9 Hz, 2H, Ar-H), 7.67 (d, J = 9 Hz, 2H, Ar-H), 5.17 (d, J = 9 Hz, 3H), 4.85 (s, 1H), 4.63 (s, 1H), 4.34 (s, 1H), 3.65 (s, 1H), 2.91 (d, J = 18 Hz, 1H), 2.21 (t, J = 12 Hz, 1H), 2.04 (d, J = 6 Hz, 1H), 1.85 (t, J = 15 Hz, 3H), 1.66–1.51 (m, 6H), 1.40 (t, J = 12 Hz, 3H), 1.28 (s, 3H), 1.19–1.06 (m, 5H), 0.98 (s, 2H), 0.90 (s, 4H), 0.83 (s, 3H), 0.65 (s, 3H), 0.21 (s, 3H). 13C-NMR (75 MHz, DMSO-d6)) δ: 207.50, 176.08, 143.74, 143.60, 135.77, 133.43, 130.28, 123.85, 122.00, 73.09, 70.84, 57.13, 55.51, 48.17, 46.69, 46.30, 41.35, 35.67, 35.41, 34.91, 33.25, 30.62, 26.80, 24.57, 23.24, 20.51, 16.70, 15.49, 9.23. ESI-HRMS calcd for C39H53N3O5Cl+ ([M + H]+): 678.36683; found: 678.36523.
4.2.4 (1-(3,4-dichlorophenyl)-1H-1,2,3-triazol-4-yl)methyl (4aR,5R,6aS,6bR,8aR,9S,10S,12aR,12bR,14bS)-9-formyl-5,10-dihydroxy-2,2,6a,6b,9,12a-hexamethyl-1,3,4,5,6,6a,6b,7,8,8a,9,10,11,12,12a,12b,13,14b-octadecahydropicene-4a (2H)-carboxylate (A4)
White solid; Yield: 65%; mp: 205–206°C; 1H-NMR (300 MHz, DMSO-d6) δ: 9.20 (s, 1H, -CHO), 8.91 (s, 1H, triazole-H), 8.26 (s, 1H, Ar-H), 7.99 (d, J = 9 Hz, 1H, Ar-H), 7.89 (d, J = 9 Hz, 1H, Ar-H), 5.16 (t, J = 9 Hz, 3H), 4.85 (d, J = 3 Hz, 1H), 4.63 (d, J = 6 Hz, 1H), 3.62 (s, 1H), 2.91 (d, J = 9 Hz, 1H), 2.21 (t, J = 15 Hz, 1H), 1.90–1.74 (m, 3H), 1.67–1.46 (m, 7H), 1.35–1.27 (m, 6H), 1.14 (t, J = 15 Hz, 4H), 0.91 (m, 7H), 0.82 (d, J = 6 Hz, 2H), 0.65 (s, 3H), 0.63 (s, 3H). 13C-NMR (75 MHz, DMSO-d6)) δ: 207.48, 176.04, 143.76, 136.49, 132.88, 132.28, 131.48, 124.24, 122.04, 121.91, 120.33, 73.07, 70.82, 57.11, 55.50, 48.15, 46.66, 46.27, 41.31, 38.03, 35.66, 35.42, 34.90, 33.25, 32.14, 31.68, 30.62, 26.77, 26.37, 24.57, 23.17, 20.50, 16.70, 15.42, 9.20. ESI-HRMS calcd for C39H52N3O5Cl2+ ([M + H]+): 712.32785; found: 712.32629.
4.2.5 (1-(4-bromophenyl)-1H-1,2,3-triazol-4-yl)methyl (4aR,5R,6aS,6bR,8aR,9S,10S,12aR,12bR,14bS)-9-formyl-5,10-dihydroxy-2,2,6a,6b,9,12a-hexamethyl-1,3,4,5,6,6a,6b,7,8,8a,9,10,11,12,12a,12b,13,14b-octadecahydropicene-4a (2H)-carboxylate (A5)
White solid; Yield: 56%; mp: 190–192°C; 1H-NMR (300 MHz, DMSO-d6) δ: 9.20 (s, 1H, -CHO), 8.82 (d, J = 3 Hz, 1H, triazole-H), 7.89 (d, J = 6 Hz, 2H, Ar-H), 7.80 (dd, J1 = 9 Hz, J2 = 3 Hz, 2H, Ar-H), 5.24–5.09 (m, 3H), 4.84 (s, 1H), 4.64 (s, 1H), 4.34 (s, 1H), 3.66 (t, J = 15 Hz, 1H), 2.91 (t, J = 15 Hz, 1H), 2.21 (t, J = 12 Hz, 1H), 2.06–1.47 (m, 10H), 1.40–1.23 (m, 7H), 1.19–1.06 (m, 4H), 0.98–0.83 (m, 12H), 0.76 (s, 1H), 0.62 (d, J = 6 Hz, 3H). 13C-NMR (75 MHz, DMSO-d6) δ: 207.50, 176.07, 143.74, 143.62, 136.18, 133.21, 123.83, 122.23, 121.91, 121.77, 86.44, 73.08, 70.83, 57.19, 55.51, 48.17, 46.69, 46.30, 41.35, 37.49, 35.67, 35.43, 34.96, 33.25, 32.16, 31.66, 30.63, 27.72, 26.79, 26.40, 24.57, 23.18, 20.53, 16.70, 15.48, 15.09, 13.72, 9.25. ESI-HRMS calcd for C39H53N3O5Br+ ([M + H]+): 722.31631; found: 722.31482.
4.2.6 (1-(4-methoxyphenyl)-1H-1,2,3-triazol-4-yl)methyl (4aR,5R,6aS,6bR,8aR,9S,10S,12aR,12bR,14bS)-9-formyl-5,10-dihydroxy-2,2,6a,6b,9,12a-hexamethyl-1,3,4,5,6,6a,6b,7,8,8a,9,10,11,12,12a,12b,13,14b-octadecahydropicene-4a (2H)-carboxylate (A6)
White solid; Yield: 59%; mp: 192–193°C; 1H-NMR (300 MHz, DMSO-d6) δ: 9.21 (s, 1H, -CHO), 8.65 (s, 1H, triazole-H), 7.79 (d, J = 9 Hz, 2H, Ar-H), 7.13 (d, J = 9 Hz, 2H, Ar-H), 5.18–5.07 (m, 3H), 4.84 (d, J = 6 Hz, 1H), 4.65 (d, J = 3 Hz, 1H), 4.34 (s, 1H), 3.82 (s, 3H, -OCH3), 3.63 (s, 1H), 2.91 (d, J = 18 Hz, 1H), 2.22 (t, J = 12 Hz, 1H), 1.95–1.47 (m, 10H), 1.41–1.05 (m, 10H), 0.99–0.82 (m, 13H), 0.69 (s, 4H). 13C-NMR (75 MHz, DMSO-d6)) δ: 207.52, 176.09, 159.73, 143.79, 143.19, 130.40, 123.60, 122.00, 121.90, 115.28, 73.10, 70.83, 57.32, 56.03, 55.52, 48.18, 46.70, 46.33, 41.37, 38.08, 35.69, 34.94, 33.25, 32.17, 31.65, 30.63, 26.82, 26.39, 24.57, 23.20, 20.56, 16.75, 15.57, 9.25. ESI-HRMS calcd for C40H56N3O6+ ([M + H]+): 674.41636; found: 674.41486.
4.2.7 (1-(3,4-dimethoxyphenyl)-1H-1,2,3-triazol-4-yl)methyl (4aR,5R,6aS,6bR,8aR,9S,10S,12aR,12bR,14bS)-9-formyl-5,10-dihydroxy-2,2,6a,6b,9,12a-hexamethyl-1,3,4,5,6,6a,6b,7,8,8a,9,10,11,12,12a,12b,13,14b-octadecahydropicene-4a (2H)-carboxylate (A7)
White solid; Yield: 71%; mp: 176–178°C; 1H-NMR (300 MHz, CDCl3) δ: 9.38 (s, 1H, -CHO), 7.96 (s, 1H, triazole-H), 7.33 (s, 1H, Ar-H), 7.15 (d, J = 6 Hz, 1H, Ar-H), 6.96 (d, J = 9 Hz, 1H, Ar-H), 5.39 (s, 1H, C12-H), 5.28–5.18 (m, 2H, -COO-CH2-), 4.57 (m, 1H, C16-H), 3.97 (s, 3H, -OCH3), 3.95 (s, 3H, -OCH3), 3.77 (t, J = 6 Hz, 1H, C3-H), 3.06 (d, J = 9 Hz, 1H, C18-H), 2.18 (t, J = 15 Hz, 2H), 1.93–1.85 (m, 3H), 1.75 (d, J = 12 Hz, 4H), 1.70–1.63 (m, 4H), 1.46 (d, J = 12 Hz, 1H), 1.36 (s, 3H), 1.27 (s, 2H), 1.03 (s, 3H), 0.96 (s, 3H), 0.89 (d, J = 6 Hz, 5H), 0.85 (d, J = 6 Hz, 5H), 0.47 (s, 3H). 13C-NMR (75 MHz, CDCl3) δ: 207.04, 176.73, 149.82, 149.52, 143.23, 142.83, 130.41, 122.59, 122.46, 112.33, 111.17, 104.76, 74.64, 71.80, 57.60, 56.26, 55.19, 48.64, 48.13, 46.52, 46.37, 41.39, 40.66, 39.76, 38.05, 35.92, 35.44, 32.76, 32.24, 30.94, 30.42, 26.85, 26.09, 24.50, 23.21, 20.67, 16.75, 15.59, 8.90. ESI-HRMS calcd for C41H58N3O7+ ([M + H]+): 704.42693; found: 704.42505.
4.2.8 (1-(3,4,5-trimethoxyphenyl)-1H-1,2,3-triazol-4-yl)methyl (4aR,5R,6aS,6bR,8aR,9S,10S,12aR,12bR,14bS)-9-formyl-5,10-dihydroxy 2,2,6a,6b,9,12a-hexamethyl-1,3,4,5,6,6a,6b,7,8,8a,9,10,11,12,12a,12b,13,14b octadecahydropicene-4a (2H)-carboxylate (A8)
White solid; Yield: 70%; mp: 170–171°C; 1H-NMR (300 MHz, CDCl3) δ: 9.38 (s, 1H, -CHO), 7.99 (s, 1H, triazole-H), 6.93 (s, 2H, Ar-H), 5.39 (s, 1H, C12-H), 5.23 (dd, J1 = 18 Hz, J2 = 12 Hz, 2H, -COO-CH2-), 4.57 (s, 1H, C16-H), 3.94 (s, 6H, -OCH3), 3.89 (s, 3H, -OCH3), 3.77 (d, J = 6 Hz, 1H, C3-H), 3.05 (d, J = 6 Hz, 1H, C18-H), 2.18 (t, J = 15 Hz, 2H), 2.05 (s, 1H), 1.90 (d, J = 18 Hz, 2H), 1.77–1.62 (m, 9H), 1.46 (d, J = 12 Hz, 1H), 1.36 (s, 3H), 1.27–1.09 (m, 7H), 1.03 (s, 3H), 0.96 (s, 3H), 0.90 (s, 3H), 0.84 (s, 3H), 0.46 (s, 3H). 13C-NMR (75 MHz, CDCl3) δ: 207.01, 176.77, 153.96, 143.31, 142.86, 138.43, 132.66, 122.67, 122.44, 98.23, 74.64, 71.80, 61.08, 57.54, 56.48, 55.17, 48.63, 48.11, 46.50, 46.36, 41.40, 40.66, 39.75, 38.04, 35.92, 35.45, 35.41, 32.75, 32.24, 30.98, 30.42, 26.85, 26.08, 24.46, 23.21, 20.67, 16.72, 15.59, 8.90. ESI-HRMS calcd for C40H56N3O6+ ([M + H]+): 674.41636; found: 674.41357.
4.2.9 (1-(p-tolyl)-1H-1,2,3-triazol-4-yl)methyl (4aR,5R,6aS,6bR,8aR,9S,10S,12aR,12bR,14bS)-9-formyl-5,10-dihydroxy-2,2,6a,6b,9,12a-hexamethyl-1,3,4,5,6,6a,6b,7,8,8a,9,10,11,12,12a,12b,13,14b-octadecahydropicene-4a (2H)-carboxylate (A9)
White solid; Yield: 56%; mp: 204–206°C; 1H-NMR (300 MHz, DMSO-d6) δ: 9.20 (s, 1H, -CHO), 8.70 (s, 1H, triazole-H), 7.76 (d, J = 6 Hz, 2H, Ar-H), 7.39 (d, J = 6 Hz, 2H, Ar-H), 5.18–5.07 (m, 3H), 4.85 (s, 1H), 4.65 (s, 1H), 4.34 (s, 1H), 3.63 (s, 1H), 2.91 (d, J = 12 Hz, 1H), 2.37 (s, 3H), 2.22 (t, J = 12 Hz, 1H), 1.90–1.67 (m, 5H), 1.62–1.47 (m, 5H), 1.41–1.28 (m, 6H), 1.20–1.05 (m, 5H), 0.98–0.90 (m, 6H), 0.82 (s, 6H), 0.67 (s, 4H). 13C-NMR (75 MHz, DMSO-d6) δ: 207.50, 176.08, 143.79, 143.30, 138.75, 134.75, 130.61, 123.56, 121.90, 120.26, 73.10, 70.83, 57.29, 55.52, 48.18, 46.70, 46.33, 41.36, 35.69, 35.42, 34.92, 33.24, 32.17, 31.66, 30.62, 26.82, 26.40, 24.58, 23.20, 21.01, 20.52, 16.74, 15.53, 9.24. ESI-HRMS calcd for C40H56N3O5+ ([M + H]+): 658.42145; found: 658.41998.
4.2.10 (1-(4-nitrophenyl)-1H-1,2,3-triazol-4-yl)methyl (4aR,5R,6aS,6bR,8aR,9S,10S,12aR,12bR,14bS)-9-formyl-5,10-dihydroxy-2,2,6a,6b,9,12a-hexamethyl-1,3,4,5,6,6a,6b,7,8,8a,9,10,11,12,12a,12b,13,14b-octadecahydropicene-4a (2H)-carboxylate (A10)
Yellow solid; Yield: 59%; mp: 193–194°C; 1H-NMR (300 MHz, DMSO-d6) δ: 9.19 (s, 1H, -CHO), 9.03 (s, 1H, triazole-H), 8.47 (d, J = 9 Hz, 2H, Ar-H), 8.25 (d, J = 9 Hz, 2H, Ar-H), 5.17 (s, 3H), 4.85 (s, 1H), 4.63 (d, J = 6 Hz, 1H), 4.34 (s, 1H), 3.62 (s, 1H), 2.91 (d, J = 12 Hz, 1H), 2.37 (s, 3H), 2.22 (t, J = 15 Hz, 1H), 1.91–1.65 (m, 5H), 1.56–1.40 (m, 6H), 1.36–1.23 (m, 5H), 1.18–1.04 (m, 5H), 0.97–0.91 (m, 6H), 0.81 (d, J = 15 Hz, 6H), 0.61 (s, 4H). 13C-NMR (75 MHz, DMSO-d6)) δ: 207.49, 176.08, 147.17, 144.08, 143.71, 141.24, 126.03, 124.35, 121.94, 120.98, 73.08, 70.81, 57.08, 55.49, 48.18, 46.64, 46.27, 41.34, 38.03, 35.66, 35.40, 34.95, 33.25, 32.13, 31.65, 30.63, 26.80, 26.35, 24.57, 23.18, 20.55, 16.69, 15.46, 9.17. ESI-HRMS calcd for C39H53N4O7+ ([M + H]+): 689.39088; found: 689.38971.
4.2.11 (5-phenyl-1,3,4-oxadiazol-2-yl)methyl (4aR,5R,6aS,6bR,8aR,9S,10S,12aR,12bR,14bS)-9-formyl-5,10-dihydroxy-2,2,6a,6b,9,12a-hexamethyl-1,3,4,5,6,6a,6b,7,8,8a,9,10,11,12,12a,12b,13,14b-octadecahydropicene-4a (2H)-carboxylate (B1)
White solid; Yield: 71%; mp: 130–132; 1H-NMR (300 MHz, CDCl3) δ: 9.41 (s, 1H, -CHO), 8.06 (d, J = 6 Hz, 2H, Ar-H), 7.59–7.51 (m, 3H), 5.41 (s, 1H, C12-H), 5.34–5.26 (m, 2H, -COO-CH2-), 4.59 (s, 1H, C16-H), 3.78 (d, J = 6 Hz, 1H, C3-H), 3.09 (d, J = 6 Hz, 1H, C18-H), 2.20 (t, J = 15 Hz, 1H), 1.95–1.80 (m, 6H), 1.68 (d, J = 18 Hz, 7H), 1.51 (s, 2H), 1.38 (s, 3H), 1.26 (t, J = 12 Hz, 3H), 1.03 (d, J = 15 Hz, 6H), 0.90 (d, J = 18 Hz, 7H), 0.65 (s, 3H). 13C-NMR (75 MHz, CDCl3) δ: 207.07, 175.72, 165.63, 161.66, 142.47, 132.17, 129.14, 127.02, 123.39, 122.79, 76.61, 74.64, 71.84, 55.34, 55.19, 49.02, 48.19, 46.58, 46.29, 41.41, 40.70, 39.80, 38.07, 35.93, 35.54, 35.37, 32.73, 32.24, 30.72, 30.39, 26.94, 26.11, 24.57, 23.26, 20.68, 16.94, 15.60, 8.92. ESI-HRMS calcd for C39H53N2O6+ ([M + H]+): 645.38981; found: 645.38849.
4.2.12 (5-(4-fluorophenyl)-1,3,4-oxadiazol-2-yl)methyl (4aR,5R,6aS,6bR,8aR,9S,10S,12aR,12bR,14bS)-9-formyl-5,10-dihydroxy-2,2,6a,6b,9,12a-hexamethyl-1,3,4,5,6,6a,6b,7,8,8a,9,10,11,12,12a,12b,13,14b-octadecahydropicene-4a (2H)-carboxylate (B2)
White solid; Yield: 72%; mp: 122–124°C; Purity: 98.40%; 1H-NMR (300 MHz, CDCl3) δ: 9.42 (s, 1H, -CHO), 8.07 (t, J = 6 Hz, 2H, Ar-H), 7.23 (t, J = 9 Hz, 2H, Ar-H), 5.41 (s, 1H, C12-H), 5.31 (s, 2H, -COO-CH2-), 4.71–4.59 (m, 1H, C16-H), 3.80 (s, 1H, C3-H), 3.09 (d, J = 15 Hz, 1H, C18-H), 2.20 (t, J = 12 Hz, 1H), 1.95–1.80 (m, 5H), 1.78–1.61 (m, 8H), 1.52–1.39 (m, 6H), 1.34–1.23 (m, 4H), 1.07 (s, 4H), 0.99 (s, 3H), 0.93 (s, 3H), 0.89 (s, 3H), 0.66 (s, 3H). 13C-NMR (75 MHz, CDCl3) δ: 207.07, 175.74, 165.43 (d, J = 92.2 Hz), 164.02, 161.71, 142.48, 129.40, 129.33, 122.80, 119.74, 116.64, 116.46, 74.63, 71.88, 55.31, 55.18, 49.06, 48.23, 46.58, 46.28, 41.44, 40.74, 39.82, 38.09, 35.96, 35.56, 35.36, 32.72, 32.27, 30.68, 30.39, 26.96, 26.12, 24.59, 23.26, 20.68, 16.96, 15.61, 8.94. ESI-HRMS calcd for C39H52N2O6F+ ([M + H]+): 663.38039; found: 663.37909.
4.2.13 (5-(4-chlorophenyl)-1,3,4-oxadiazol-2-yl)methyl (4aR,5R,6aS,6bR,8aR,9S,10S,12aR,12bR,14bS)-9-formyl-5,10-dihydroxy-2,2,6a,6b,9,12a-hexamethyl-1,3,4,5,6,6a,6b,7,8,8a,9,10,11,12,12a,12b,13,14b-octadecahydropicene-4a (2H)-carboxylate (B3)
White solid; Yield: 65%; mp: 132–134°C; Purity: 98.98%; 1H-NMR (300 MHz, CDCl3) δ: 9.41 (s, 1H, -CHO), 8.00 (d, J = 9 Hz, 2H, Ar-H), 7.52 (d, J = 9 Hz, 2H, Ar-H), 5.40 (s, 1H, C12-H), 5.31 (t, J = 15 Hz, 2H, -COO-CH2-), 4.59 (s, 1H, C16-H), 3.78 (t, J = 6 Hz, 1H, C3-H), 3.07 (dd, J1 = 12 Hz, J2 = 3 Hz, 1H, C18-H), 2.19 (t, J = 15 Hz, 1H), 1.95–1.84 (m, 4H), 1.78 (s, 1H), 1.70 (d, J = 9 Hz, 6H), 1.57–1.47 (m, 3H), 1.38 (s, 3H), 1.26 (t, J = 15 Hz, 3H), 1.17–1.11 (m, 2H), 1.06 (s, 3H), 0.98 (s, 3H), 0.93 (s, 3H), 0.88 (s, 3H), 0.64 (s, 3H). 13C-NMR (75 MHz, CDCl3) δ: 207.09, 175.74, 164.81, 161.84, 142.45, 138.54, 129.56, 128.29, 122.79, 121.85, 74.60, 71.85, 55.30, 55.18, 49.04, 48.20, 46.56, 46.26, 41.42, 40.71, 39.80, 38.07, 35.93, 35.54, 35.34, 32.72, 32.25, 30.68, 30.39, 26.94, 26.10, 24.58, 23.25, 20.67, 16.96, 15.60, 8.94. ESI-HRMS calcd for C39H52N2O6Cl+ ([M + H]+): 679.35084; found: 679.34991.
4.2.14 (5-(4-methoxyphenyl)-1,3,4-oxadiazol-2-yl)methyl (4aR,5R,6aS,6bR,8aR,9S,10S,12aR,12bR,14bS)-9-formyl-5,10-dihydroxy-2,2,6a,6b,9,12a-hexamethyl-1,3,4,5,6,6a,6b,7,8,8a,9,10,11,12,12a,12b,13,14b-octadecahydropicene-4a (2H)-carboxylate (B4)
White solid; Yield: 65%; mp: 126–128°C; Purity: 97.88%; 1H-NMR (300 MHz, CDCl3) δ: 9.42 (s, 1H, -CHO), 7.99 (d, J = 9 Hz, 2H, Ar-H), 7.03 (d, J = 9 Hz, 2H, Ar-H), 5.41 (t, J = 6 Hz, 1H, C12-H), 5.31 (t, J = 6 Hz, 2H, -COO-CH2-), 4.60 (s, 1H, C16-H), 3.91 (s, 3H, -OCH3), 3.80 (s, 1H, C3-H), 3.08 (d, J = 12 Hz, 1H, C18-H), 2.19 (t, J = 12 Hz, 1H), 1.96–1.84 (m, 5H), 1.80 (t, J = 6 Hz, 1H), 1.71–1.62 (m, 7H), 1.52–1.46 (m, 3H), 1.38 (s, 4H), 1.34–1.22 (m, 4H), 1.06 (s, 4H), 0.99 (s, 3H), 0.93 (s, 3H), 0.89 (s, 3H), 0.66 (s, 3H). 13C-NMR (75 MHz, CDCl3) δ: 207.09, 175.75, 165.60, 162.67, 161.16, 142.51, 128.84, 122.78, 115.86, 114.57, 74.61, 71.85, 55.53, 55.37, 55.21, 49.04, 48.19, 46.60, 46.30, 41.42, 40.71, 39.81, 38.09, 35.95, 35.54, 35.37, 32.73, 32.25, 30.68, 30.39, 26.95, 26.11, 24.60, 23.26, 20.69, 16.96, 15.62, 8.92. ESI-HRMS calcd for C40H55N2O7+ ([M + H]+): 675.40038; found: 675.39911.
4.2.15 (5-(3,4,5-trimethoxyphenyl)-1,3,4-oxadiazol-2-yl)methyl (4aR,5R,6aS,6bR,8aR,9S,10S,12aR,12bR,14bS)-9-formyl-5,10-dihydroxy-2,2,6a,6b,9,12a-hexamethyl-1,3,4,5,6,6a,6b,7,8,8a,9,10,11,12,12a,12b,13,14b-octadecahydropicene-4a (2H)-carboxylate (B5)
White solid; Yield: 66%; mp: 136–138°C; Purity: 97.48%; 1H-NMR (300 MHz, CDCl3) δ: 9.40 (s, 1H, -CHO), 5.40 (s, 1H, C12-H), 5.31 (s, 2H, -COO-CH2-), 4.59 (s, 1H, C16-H), 3.95 (s, 6H, -OCH3), 3.93 (s, 3H, -OCH3), 3.78 (d, J = 9 Hz, 1H, C3-H), 3.08 (dd, J1 = 15 Hz, J2 = 3 Hz, 1H, C18-H), 2.20 (t, J = 15 Hz, 1H), 1.95–1.78 (m, 6H), 1.68 (s, 7H), 1.51 (t, J = 12 Hz, 2H), 1.38 (s, 3H), 1.25 (t, t, J = 9 Hz, 3H), 1.17–1.11 (m, 2H), 1.05 (s, 3H), 0.99 (s, 3H), 0.90 (d, J = 18 Hz, 7H), 0.63 (s, 3H). 13C-NMR (75 MHz, CDCl3) δ: 207.06, 175.76, 165.50, 161.54, 153.73, 142.47, 141.50, 122.78, 118.39, 104.34, 74.58, 71.84, 61.05, 56.42, 55.30, 55.17, 49.05, 48.17, 46.56, 46.22, 41.43, 40.78, 39.79, 38.07, 35.91, 35.55, 35.34, 32.72, 32.24, 30.61, 30.38, 26.95, 26.09, 24.63, 23.27, 20.68, 16.94, 15.58, 8.93. ESI-HRMS calcd for C42H59N2O9+ ([M + H]+): 735.42151; found: 735.42041.
4.2.16 (5-(4-nitrophenyl)-1,3,4-oxadiazol-2-yl)methyl (4aR,5R,6aS,6bR,8aR,9S,10S,12aR,12bR,14bS)-9-formyl-5,10-dihydroxy-2,2,6a,6b,9,12a-hexamethyl-1,3,4,5,6,6a,6b,7,8,8a,9,10,11,12,12a,12b,13,14b-octadecahydropicene-4a (2H)-carboxylate (B6)
White solid; Yield: 66%; mp: 140–142°C; Purity: 98.55%; 1H-NMR (300 MHz, CDCl3) δ: 9.41 (s, 1H, -CHO), 8.41 (d, J = 9 Hz, 2H, Ar-H), 8.26 (d, J = 9 Hz, 2H, Ar-H), 5.41 (s, 1H, C12-H), 5.34 (s, 2H, -COO-CH2-), 4.59 (s, 1H, C16-H), 3.79 (d, J = 6 Hz, 1H, C3-H), 3.08 (dd, J1 = 15 Hz, J2 = 6 Hz, 1H, C18-H), 2.20 (t, J = 12 Hz, 1H), 1.96 (s, 1H), 1.89–1.84 (m, 3H), 1.72–1.66 (m, 6H), 1.51 (t, J = 6 Hz, 2H), 1.39 (s, 3H), 1.27 (d, J = 12 Hz, 4H), 1.18–1.11 (m, 2H), 1.06 (s, 3H), 0.99 (s, 4H), 0.93–0.87 (m, 7H), 0.66 (s, 3H). 13C-NMR (75 MHz, CDCl3) δ: 207.07, 175.75, 163.80, 162.75, 149.82, 142.44, 128.84, 127.99, 124.46, 122.81, 74.58, 71.87, 55.27, 55.17, 49.07, 48.18, 46.54, 46.24, 41.45, 40.75, 39.82, 38.07, 35.94, 35.56, 35.32, 32.71, 32.26, 30.68, 30.38, 26.95, 26.10, 24.58, 23.27, 20.67, 16.97, 15.63, 8.96. ESI-HRMS calcd for C39H52N3O8+ ([M + H]+): 690.37489; found: 690.37347.
4.2.17 2-(5-oxo-4-phenyl-4,5-dihydro-1H-1,2,4-triazol-1-yl)ethyl (4aR,5R,6aS,6bR,8aR,9S,10S,12aR,12bR,14bS)-9-formyl-5,10-dihydroxy-2,2,6a,6b,9,12a-hexamethyl-1,3,4,5,6,6a,6b,7,8,8a,9,10,11,12,12a,12b,13,14b-octadecahydropicene-4a (2H)-carboxylate (C1)
White solid; Yield: 70%; mp: 174–176°C; Purity: 99.00%; 1H-NMR (300 MHz, CDCl3) δ: 9.41 (s, 1H, -CHO), 7.72 (s, 1H, triazole-H), 7.58–7.48 (m, 4H, Ar-H), 7.39 (d, J = 9 Hz, 1H, Ar-H), 5.42 (s, 1H, C12-H), 4.49–4.42 (m, 2H, -COO-CH2-), 4.33–4.25 (m, 1H, C16-H), 4.13 (t, J = 6 Hz, 2H, -N-CH2-), 3.78 (t, J = 6 Hz, 1H, C3-H), 3.06 (dd, J1 = 15 Hz, J2 = 6 Hz, 1H, C18-H), 2.13–2.04 (m, 2H), 1.92–1.88 (m, 2H), 1.78–1.60 (m, 9H), 1.52–1.44 (m, 3H), 1.33–1.24 (m, 6H), 1.18–1.07 (s, 6H), 0.93 (d, J = 12 Hz, 10H), 0.74 (s, 3H). 13C-NMR (75 MHz, CDCl3) δ: 207.05, 176.62, 151.87, 142.47, 133.94, 133.75, 129.71, 127.73, 122.46, 121.74, 73.93, 71.82, 61.82, 55.23, 49.41, 48.22, 46.79, 46.00, 44.47, 41.49, 40.97, 40.00, 38.13, 35.95, 35.45, 34.98, 32.66, 32.21, 30.20, 29.13, 27.06, 26.12, 25.34, 23.27, 20.70, 17.15, 15.81, 8.91. ESI-HRMS calcd for C40H56N3O6+ ([M + H]+): 674.41636; found: 674.41486.
4.2.18 2-(4-(4-fluorophenyl)-5-oxo-4,5-dihydro-1H-1,2,4-triazol-1-yl)ethyl (4aR,5R,6aS,6bR,8aR,9S,10S,12aR,12bR,14bS)-9-formyl-5,10-dihydroxy-2,2,6a,6b,9,12a-hexamethyl-1,3,4,5,6,6a,6b,7,8,8a,9,10,11,12,12a,12b,13,14b-octadecahydropicene-4a (2H)-carboxylate (C2)
White solid; Yield: 74%; mp: 176–178°C; 1H-NMR (300 MHz, CDCl3) δ: 9.39 (s, 1H, -CHO), 7.69 (d, J = 3 Hz, 1H, triazole-H), 7.52 (dd, J1 = 9 Hz, J2 = 3 Hz, 2H, Ar-H), 7.19 (t, J = 6 Hz, 2H, Ar-H), 5.41 (s, 1H, C12-H), 4.46–4.40 (m, 2H, -COO-CH2-), 4.31–4.24 (m, 1H, C16-H), 4.11 (t, J = 6 Hz, 2H, -N-CH2-), 3.78 (d, J = 6 Hz, 1H, C3-H), 3.04 (d, J = 12 Hz, 1H, C18-H), 2.20–2.03 (m, 2H), 1.90 (d, J = 6 Hz, 2H), 1.81–1.63 (m, 10H), 1.49 d, J = 9 Hz, 2H), 1.37–1.27 (m, 6H), 1.13 (t, J = 6 Hz, 2H), 1.06 (s, 3H), 0.95 (d, J = 3 Hz, 6H), 0.90 (s, 3H), 0.84 (s, 1H), 0.73 (s, 3H). 13C-NMR (75 MHz, CDCl3) δ: 207.11, 176.62, 161.65 (d, J = 246 Hz), 151.90, 142.46, 133.94, 129.72, 123.96 (d, J = 8.25 Hz), 122.45, 116.68 (d, J = 23.25 Hz), 73.90, 71.81, 61.77, 55.26, 49.40, 48.19, 46.77, 45.97, 44.52, 41.49, 40.96, 40.00, 38.13, 35.94, 35.42, 34.96, 32.65, 32.21, 30.20, 29.10, 27.05, 26.10, 25.33, 23.27, 20.70, 17.15, 15.81, 8.92. ESI-HRMS calcd for C40H55N3O6F+ ([M + H]+): 692.40694; found: 692.40527.
4.2.19 2-(4-(4-chlorophenyl)-5-oxo-4,5-dihydro-1H-1,2,4-triazol-1-yl)ethyl (4aR,5R,6aS,6bR,8aR,9S,10S,12aR,12bR,14bS)-9-formyl-5,10-dihydroxy-2,2,6a,6b,9,12a-hexamethyl-1,3,4,5,6,6a,6b,7,8,8a,9,10,11,12,12a,12b,13,14b-octadecahydropicene-4a (2H)-carboxylate (C3)
White solid; Yield: 65%; mp: 166–168°C; Purity: 97.39%; 1H-NMR (300 MHz, CDCl3) δ: 9.39 (s, 1H, -CHO), 7.71 (s, 1H, triazole-H), 7.53 (d, J = 9 Hz, 2H, Ar-H), 7.47 (d, J = 9 Hz, 2H, Ar-H), 5.40 (s, 1H, C12-H), 4.44–4.39 (m, 2H, -COO-CH2-), 4.31–4.24 (m, 1H, C16-H), 4.11 (t, J = 6 Hz, 2H, -N-CH2-), 3.78 (d, J = 9 Hz, 1H, C3-H), 3.03 (dd, J1 = 15 Hz, J2 = 3 Hz, 1H, C18-H), 2.18–2.03 (m, 2H), 1.91–1.87 (m, 2H), 1.76–1.67 (m, 9H), 1.48 (t, J = 9 Hz, 2H), 1.40–1.22 (m, 7H), 1.13 (t, J = 6 Hz, 3H), 1.06 (s, 3H), 0.94 (s, 6H), 0.86 (s, 1H), 0.90 (s, 3H), 0.72 (s, 3H). 13C-NMR (75 MHz, CDCl3) δ: 207.11, 176.62, 151.68, 142.45, 133.53, 133.45, 132.26, 129.87, 122.87, 122.45, 73.91, 71.81, 61.74, 55.24, 49.40, 48.21, 46.76, 45.98, 44.54, 41.48, 40.95, 39.99, 38.13, 35.94, 35.41, 34.96, 32.65, 32.20, 30.20, 29.11, 27.05, 26.10, 25.33, 23.27, 20.70, 17.13, 15.81, 8.93. ESI-HRMS calcd for C40H55N3O6Cl+ ([M + H]+): 708.37739; found: 708.37628.
4.2.20 2-(4-(3,4-dichlorophenyl)-5-oxo-4,5-dihydro-1H-1,2,4-triazol-1-yl)ethyl (4aR,5R,6aS,6bR,8aR,9S,10S,12aR,12bR,14bS)-9-formyl-5,10-dihydroxy-2,2,6a,6b,9,12a-hexamethyl-1,3,4,5,6,6a,6b,7,8,8a,9,10,11,12,12a,12b,13,14b-octadecahydropicene-4a (2H)-carboxylate (C4)
White solid; Yield: 55%; mp: 187–189°C; 1H-NMR (300 MHz, CDCl3) δ: 9.41 (s, 1H, -CHO), 7.77 (s, 1H, triazole-H), 7.73 (s, 1H, Ar-H), 7.58 (d, J = 9 Hz, 1H, Ar-H), 7.48 (d, J = 9 Hz, 1H, Ar-H), 5.39 (s, 1H, C12-H), 4.41 (s, 2H, -COO-CH2-), 4.30 (s, 1H, C16-H), 4.11 (s, 2H, -N-CH2-), 3.78 (s, 1H, C3-H), 3.04 (d, J = 12 Hz, 1H, C18-H), 2.08 (t, J = 12 Hz, 2H), 1.85 (d, J = 12 Hz, 3H), 1.69 (d, J = 12 Hz, 8H), 1.49 (t, J = 12 Hz, 3H), 1.30 (t, J = 18 Hz, 6H), 1.07 (s, 6H), 0.93 (d, J = 12 Hz, 9H), 0.72 (s, 3H). 13C-NMR (75 MHz, CDCl3) δ: 207.05, 176.58, 151.38, 142.45, 133.83, 133.01, 131.78, 131.36, 123.27, 122.45, 120.48, 74.01, 71.84, 61.66, 55.20, 49.36, 48.25, 46.74, 45.99, 44.64, 41.48, 40.93, 39.99, 38.12, 35.96, 35.44, 34.99, 32.65, 32.22, 30.22, 29.22, 27.06, 26.11, 25.28, 23.28, 20.70, 17.13, 15.81, 8.93. ESI-HRMS calcd for C40H54N3O6Cl2+ ([M + H]+): 742.33842; found: 742.33636.
4.2.21 2-(4-(4-methoxyphenyl)-5-oxo-4,5-dihydro-1H-1,2,4-triazol-1-yl)ethyl (4aR,5R,6aS,6bR,8aR,9S,10S,12aR,12bR,14bS)-9-formyl-5,10-dihydroxy-2,2,6a,6b,9,12a-hexamethyl-1,3,4,5,6,6a,6b,7,8,8a,9,10,11,12,12a,12b,13,14b-octadecahydropicene-4a (2H)-carboxylate (C5)
White solid; Yield: 58%; mp: 186–188°C; Purity: 98.33%; 1H-NMR (300 MHz, CDCl3) δ: 9.40 (s, 1H, -CHO), 7.64 (s, 1H, triazole-H), 7.43 (d, J = 9 Hz, 2H, Ar-H), 7.00 (d, J = 9 Hz, 2H, Ar-H), 5.42 (s, 1H, C12-H), 4.47–4.40 (m, 2H, -COO-CH2-), 4.31–4.24 (m, 1H, C16-H), 4.11 (t, J = 2 Hz, 2H, -N-CH2-), 3.86 (s, 3H, -OCH3), 3.79 (d, J = 9 Hz, 1H, C3-H), 3.05 (dd, J1 = 12 Hz, J2 = 3 Hz, 1H, C18-H), 2.20–2.03 (m, 2H), 1.92–1.82 (m, 3H), 1.75–1.63 (m, 10H), 1.49 (t, J = 9 Hz, 2H), 1.38–1.24 (m, 6H), 1.16–1.09 (m, 2H), 1.06 (s, 3H), 0.95 (s, 6H), 0.91 (S, 3H), 0.74 (s, 3H). 13C-NMR (75 MHz, CDCl3) δ: 207.05, 176.63, 159.05, 152.17, 142.44, 134.42, 126.55, 123.81, 122.46, 114.84, 73.86, 71.82, 61.87, 55.60, 55.24, 49.46, 48.23, 46.80, 45.98, 44.47, 41.50, 41.01, 40.02, 38.13, 35.96, 35.45, 34.95, 32.65, 32.21, 30.19, 28.99, 27.07, 26.12, 25.41, 23.28, 20.72, 17.16, 15.82, 8.91. ESI-HRMS calcd for C41H58N3O7+ ([M + H]+): 704.42693; found: 704.42523.
4.2.22 2-(5-oxo-4-(3,4,5-trimethoxyphenyl)-4,5-dihydro-1H-1,2,4-triazol-1-yl)ethyl (4aR,5R,6aS,6bR,8aR,9S,10S,12aR,12bR,14bS)-9-formyl-5,10-dihydroxy-2,2,6a,6b,9,12a-hexamethyl-1,3,4,5,6,6a,6b,7,8,8a,9,10,11,12,12a,12b,13,14b-octadecahydropicene-4a (2H)-carboxylate (C6)
White solid; Yield: 67%; mp: 174–176°C; Purity: 98.72%; 1H-NMR (300 MHz, CDCl3) δ: 9.40 (s, 1H, -CHO), 7.68 (s, 1H, triazole-H), 6.75 (s, 2H, Ar-H), 5.42 (s, 1H, C12-H), 4.45–4.38 (m, 2H, -COO-CH2-), 4.33–4.26 (m, 1H, C16-H), 4.11 (t, J = 6 Hz, 2H, -N-CH2-), 3.90 (s, 6H, -OCH3), 3.87 (s, 3H, -OCH3), 3.78 (dd, J1 = 9 Hz, J2 = 6 Hz, 1H, C3-H), 3.06 (d, J = 6 Hz, 1H, C18-H), 2.07 (t, J = 12 Hz, 1H), 1.91–1.82 (m, 4H), 1.77–1.64 (m, 10H), 1.50 (t, J = 9 Hz, 3H), 1.38 (d, J = 6 Hz, 1H), 1.32 (s, 3H), 1.27 (s, 1H), 1.12 (t, J = 6 Hz, 2H), 1.06 (s, 3H), 0.95 (s, 6H), 0.88 (d, J = 6 Hz, 4H), 0.75 (s, 3H). 13C-NMR (75 MHz, CDCl3) δ: 207.04, 176.60, 153.88, 151.99, 142.38, 134.24, 129.33, 122.49, 100.11, 73.85, 71.84, 61.81, 60.99, 56.40, 55.22, 49.50, 48.23, 46.80, 44.44, 41.52, 41.03, 40.04, 38.13, 35.96, 35.45, 34.94, 32.64, 32.24, 30.18, 28.91, 27.09, 26.10, 25.46, 23.29, 20.72, 17.20, 15.82, 8.93. ESI-HRMS calcd for C43H62N3O9+ ([M + H]+): 764.44806; found: 764.44617.
4.2.23 (3-phenylisoxazol-5-yl)methyl (4aR,5R,6aS,6bR,8aR,9S,10S,12aR,12bR,14bS)-9-formyl-5,10-dihydroxy-2,2,6a,6b,9,12a-hexamethyl-1,3,4,5,6,6a,6b,7,8,8a,9,10,11,12,12a,12b,13,14b-octadecahydropicene-4a (2H)-carboxylate (D)
White solid; Yield: 66%; mp: 176–178°C; 1H-NMR (300 MHz, CDCl3) δ: 9.41 (s, 1H, -CHO), 7.81 (t, J = 6 Hz, 2H, Ar-H), 7.49 (d, J = 3 Hz, 3H, Ar-H), 6.60 (s, 1H, isoxazole-H), 5.42 (s, 1H, C12-H), 5.19 (s, 2H, -COO-CH2-), 4.59 (s, 1H, C16-H), 3.80 (s, 1H, C3-H), 3.09 (d, J = 15 Hz, 1H, C18-H), 2.20 (t, J = 15 Hz, 1H), 1.93–1.67 (m, 9H), 1.59 (s, 3H), 1.53–1.39 (m, 7H), 1.33–1.18 (m, 4H), 1.15–0.88 (m, 14H), 0.62 (s, 3H). 13C-NMR (75 MHz, CDCl3) δ: 207.07, 176.02, 167.09, 162.49, 142.56, 130.21, 128.97, 128.65, 126.79, 122.79, 102.36, 74.77, 71.83, 56.36, 55.19, 48.78, 48.20, 46.58, 46.31, 41.41, 40.74, 39.82, 38.08, 35.94, 35.53, 35.41, 32.76, 32.30, 30.94, 30.43, 26.91, 26.11, 24.48, 23.27, 20.67, 17.00, 15.57, 8.90. ESI-HRMS calcd for C40H54NO6+ ([M + H]+): 644.39456; found: 644.39355.
4.3 General procedure for synthesis of compounds E
Stir a mixture of quillaic acid (50 mg, 0.10 mmol), 2 equivalents of K2CO3, catalytic amount KI, and 2 equivalents of 1,2-dibromoethane in DMF at 60°C. The reaction was monitored by TLC. After quenching the reaction with cold water, extracting with dichloromethane, collecting the organic phase and evaporating to dryness to obtain a crude product. The crude product was added K2CO3, KI and 5-phenyl-1H-tetrazole in the DMF solution, and reacted at 60°C for 8 h. After the reaction was quenched with cold water, extracted with dichloromethane, the organic phase was collected and evaporated to dryness, and purified by silica gel column chromatography to obtain the target compound E.
4.3.1 2-(5-phenyl-1H-tetrazol-1-yl)ethyl (4aR,5R,6aS,6bR,8aR,9S,10S,12aR,12bR,14bS)-9-formyl-5,10-dihydroxy-2,2,6a,6b,9,12a-hexamethyl-1,3,4,5,6,6a,6b,7,8,8a,9,10,11,12,12a,12b,13,14b-octadecahydropicene-4a (2H)-carboxylate (E)
White solid; Yield: 32%; mp: 196–198°C; Purity: 98.23%; 1H-NMR (300 MHz, CDCl3) δ: 9.38 (s, 1H, -CHO), 8.17 (t, J = 3 Hz, 2H, Ar-H), 7.51 (t, J = 3 Hz, 3H, Ar-H), 5.27 (t, J = 3 Hz, 1H, C12-H), 4.96–4.89 (m, 2H, -COO-CH2-), 4.71–4.65 (s, 1H, C16-H), 4.52–4.41 (m, 2H, -N-CH2-), 3.76 (d, J = 9 Hz, 1H, C3-H), 2.95 (d, J = 12 Hz, 1H, C18-H), 2.12 (t, J = 12 Hz, 1H), 1.84–1.73 (m, 6H), 1.65–1.59 (m, 5H), 1.53–1.15 (m, 11H), 1.04 (s, 5H), 0.89 (t, J = 12 Hz, 9H), 0.85 (s, 3H), 0.54 (s, 3H). 13C-NMR (75 MHz, CDCl3) δ: 206.97, 176.20, 165.48, 142.46, 130.53, 128.95, 127.15, 126.86, 122.63, 74.52, 71.80, 61.67, 55.16, 51.80, 48.73, 48.16, 46.55, 46.17, 41.27, 40.45, 39.71, 38.02, 35.90, 35.38, 35.33, 32.71, 32.03, 30.77, 30.34, 26.89, 26.09, 24.49, 23.16, 20.64, 16.75, 15.60, 8.86. ESI-HRMS calcd for C39H55N4O5+ ([M + H]+): 659.41670; found: 659.41547.
4.4 Cell lines and cell culture
The propidium iodide (PI) and Annexin V-FITC apoptosis detection kit were purchased from Invitrogen (Eugene, OR, United States). 3-(4,5-Dimethylthiazol-2-yl)-2,5-diphenyl-2H-tetrazolium bromide (MTT) was purchased from Sigma-Aldrich Co. (St. Louis, MO, United States). All human cell lines were used in this study, colorectal cancer cell HCT116, colorectal cancer cell SW620, breast cancer cell MCF-7, liver hepatocellular carcinoma cell BEL7402, liver hepatocellular carcinoma HepG2 and normal liver cell L02 were initially purchased from American Type Culture Collection (ATCC, Manassas, VA, United States). RPMI-1640 media, Dulbecco’s modified Eagle’s medium (DMEM), and foetal bovine serum (FBS) were provided from Gibco Company (Grand Island, NY, United States). Cell line MCF-7 and HepG2 were cultivated in DMEM containing 10% (v/v) heat-inactivated FBS, 100 units/ml penicillin and 100 mg/ml streptomycin (Grand Island, NY, United States). Cell lines HCT116, BEL7402, SW620, and L02 were cultivated in RPMI 1640 medium containing 10% (v/v) heat-inactivated FBS, 100 units/ml penicillin, and 100 mg/ml streptomycin. The cells were incubated at 37°C under a 5% CO2 and 90% relative humidity (RH) atmosphere.
4.5 In vitro anticancer activity (MTT assay)
Stock solutions were prepared by diluting target compounds in DMSO at concentrations (1.25, 2.5, 5, and 10 mM). The stock solution was then diluted (1.25, 2.5, 5, and 10 μM) with culture medium, and the concentration of DMSO was controlled not to exceed 0.1%.
Place the cells in a 96-well plate at an appropriate density to ensure exponential growth (1 × 104–1.2×104 cells per well) throughout the experiment, and then allow the cells to adhere for 24 h. The cells were then treated with continuous concentrations of each compound (1.25, 2.5, 5, and 10 μM) for 48 h. After 48 h of incubation, 15 μl of MTT solution was added to each well, and the final concentration was 2 mg/ml. Then incubate for another 4 h. After incubation, remove the MTT solution and add 150 ml of DMSO to each well for staining. Shake vigorously for 10 min at room temperature to ensure complete dissolution. The optical density (OD) was read on a microplate reader (ELx 800, BioTek, Highland Park, Winooski, VT, United States) with a wavelength of 492 nm, and then the data was analyzed.
4.6 Analysis for cell cycle by flow cytometry
Inoculate HCT116 cells in a 6-well plate (5.0 × 105 cells per well) and incubate at 37°C for 24 h. The exponentially growing cells were then incubated with compound E at 10 and 30 μM. After 24 h, untreated cells (control) or cells treated with compound E were centrifuged at 1,000 rpm for 15 min, and then fixed in 70% ethanol at 20°C for at least 24 h. The cells were then resuspended in phosphate buffered saline (PBS) containing 0.1 mg/ml RNase A and 5 mg/ml PI. The DNA content of cells used for cell cycle distribution analysis was measured by flow cytometry, and analyzed using FACSalibur flow cytometer and Cell Quest software (Becton-Dickinson, Franklin Lakes, NJ, United States).
4.7 Colony formation assay
Exponentially growing HCT116 cells (600 cells per well) were plated on 6-well plates with Roswell Park Memorial Institute (RPMI) 1,640 medium containing 10% fetal bovine serum (FBS). After incubating for 12 h, replace with fresh medium. Then, the cells were treated with different concentrations of compound E (2.5, 5 and 10 μM) dissolved in DMSO. Some cells only use DMSO as a negative control. Then incubate for another 7 days. Finally, the cells were fixed with 95% ethanol for 25 min, stained with 0.1% crystal violet for 15 min at room temperature, and then washed with PBS until the colonies were completely removed.
4.8 Apoptosis analysis
Apoptosis detection kit (Invitrogen, Eugene, OR, United State) was used to detect cell apoptosis. Culture the cells in a 6-well plate (5.0 × 105 cells per well) and incubate at 37°C for 24 h. The exponentially growing cells were then incubated with compound E at 10 and 30 μM. After 24 h of incubation, the cells were collected, washed twice with PBS, and washed with 1× binding buffer at room temperature for 30 min in the dark. A FACSCalibur flow cytometer with Cell Quest software (Bectone Dickinson, Franklin Lakes, NJ, United State) was used to count apoptotic cells.
4.9 Western blot analysis
HCT116 cells were cultured with different concentrations of compound E for 24 h. Then, the floating cells were collected and washed twice with ice-cold PBS. Add cold RIPA buffer. After the cells were lysed on ice for 20 min, the lysate was centrifuged at 12,000 rpm for 15 min at 4°C. The BCA method determines the protein concentration. Separated by SDS/PAGE electrophoresis and transferred to a nitrocellulose filter membrane for Western blot analysis. The membrane was blocked with PBS containing 5% skim milk at room temperature for 2 h. Then incubate the membrane with primary antibodies against Bax, Bcl-2, p-IκB, IκB, p-NF-κB p65, NF-κB p65, p-ERK, ERK, p-JNK, JNK, p-p38, and p38 protein and β-actin at 4°C with gentle rotation overnight. Next, incubate the membrane with the fluorescent secondary antibody for 2 h. The protein is detected by electrochemiluminescence (Bio-Rad, CA, United State).
Data availability statement
The original contributions presented in the study are included in the article/Supplementary Material, further inquiries can be directed to the corresponding authors.
Author contributions
Conceptualization and review methodologies—XH and DW. Original draft writing—XH and HD. Editing—Z-CH and G-HY. Figure creation—HD, L-JJ, and C-FE. Revision—J-LL, Q-KS and Z-SQ. All authors have read and agreed to the published version of the manuscript.
Funding
This work was supported by the National Natural Science Foundation of China (Nos. 81960626, 82160741,82160666, and 82060628), Doctoral Research Startup Fundation of Yanbian University (No. ydbq202215) and Jilin Provincial Education Department of China (No. JJKH 20191156KJ).
Acknowledgments
The authors gratefully acknowledge the National Natural Science Foundation of China.
Conflict of interest
The authors declare that the research was conducted in the absence of any commercial or financial relationships that could be construed as a potential conflict of interest.
Publisher’s note
All claims expressed in this article are solely those of the authors and do not necessarily represent those of their affiliated organizations, or those of the publisher, the editors and the reviewers. Any product that may be evaluated in this article, or claim that may be made by its manufacturer, is not guaranteed or endorsed by the publisher.
Supplementary material
The Supplementary Material for this article can be found online at: https://www.frontiersin.org/articles/10.3389/fchem.2022.951713/full#supplementary-material
References
Bajaj, S., Asati, V., Singh, J., and Roy, P. P. (2015). 1, 3, 4-Oxadiazoles: An emerging scaffold to target growth factors, enzymes and kinases as anticancer agents. Eur. J. Med. Chem. 97, 124–141. doi:10.1016/j.ejmech.2015.04.051
Balaraju, V., Kalyani, S., Sridhar, G., and Laxminarayana, E. (2021). Design, synthesis and biological assessment of 1, 3, 4-oxadiazole incorporated oxazole-triazine derivatives as anticancer agents. Chem. Data Collect. 33, 100695. doi:10.1016/j.cdc.2021.100695
Bian, M., Zhen, D., Shen, Q. K., Du, H. H., Ma, Q. Q., and Quan, Z. S. (2021). Structurally modified glycyrrhetinic acid derivatives as anti-inflammatory agents. Bioorg. Chem. 107, 104598. doi:10.1016/j.bioorg.2020.104598
Bock, V. D., Speijer, D., Hiemstra, H., and Van Maarseveen, J. H. (2007). 1, 2, 3-triazoles as peptide bond isosteres: Synthesis and biological evaluation of cyclotetrapeptide mimics. Org. Biomol. Chem. 5, 971–975. doi:10.1039/b616751a
Boke Sarikahya, N., Goren, A. C., Sumer Okkali, G., Coven, F. O., Orman, B., Kirci, D., et al. (2021). Chemical composition and biological activities of propolis samples from different geographical regions of Turkey. Phytochem. Lett. 44, 129–136. doi:10.1016/j.phytol.2021.06.008
Bray, F., Ferlay, J., Soerjomataram, I., Siegel, R. L., Torre, L. A., and Jemal, A. (2018). Global cancer statistics 2018: GLOBOCAN estimates of incidence and mortality worldwide for 36 cancers in 185 countries. CA A Cancer J. Clin. 68, 394–424. doi:10.3322/caac.21492
Chi, K. Q., Wei, Z. Y., Wang, K. S., Wu, J., Chen, W. Q., Jin, X. J., et al. (2017). Design, synthesis, and evaluation of novel ursolic acid derivatives as HIF-1α inhibitors with anticancer potential. Bioorg. Chem. 75, 157–169. doi:10.1016/j.bioorg.2017.09.013
Dy, G. K., and Adjei, A. A. (2013). Understanding, recognizing, and managing toxicities of targeted anticancer therapies. CA. A Cancer J. Clin. 63, 249–279. doi:10.3322/caac.21184
Ghosh, K., Sarkar, A. R., and Chattopadhyay, A. P. (2012). Anthracene-labeled 1, 2, 3-triazole-linked bispyridinium amide for selective sensing of H2PO4- by fluorescence and gel formation. Eur. J. Org. Chem. 2012, 1311–1317. doi:10.1002/ejoc.201101240
Guzman, L., Villalon, K., Marchant, M. J., Tarnok, M. E., Cardenas, P., Aquea, G., et al. (2020). In vitro evaluation and molecular docking of QS-21 and quillaic acid from Quillaja saponaria Molina as gastric cancer agents. Sci. Rep. 10, 10534. doi:10.1038/s41598-020-67442-3
Han, Z. X., Wang, H. M., Jiang, G., Du, X. P., Gao, X. Y., and Pei, D. S. (2013). Overcoming paclitaxel resistance in lung cancer cells via dual inhibition of stathmin and bcl-2. Cancer biother. Radiopharm. 28, 398–405. doi:10.1089/cbr.2012.1323
Huang, X., Chen, T., Han, R. B., and Piao, F. Y. (2018). Synthesis and Anticonvulsant Activity of 3-(alkylamino, alkoxy)-1, 3, 4, 5-Tetrahydro-2H-benzo[b]azepine-2-one Derivatives. CNS Neurol. Disord. Drug Targets 17, 448–457. doi:10.2174/1871527317666180704101332
Hwang, S. G., Park, J., Park, J. Y., Park, C. H., Lee, K. H., Cho, J. W., et al. (2012). Anti-cancer activity of a novel small molecule compound that simultaneously activates p53 and inhibits NF-κB signaling. PLoS One 7, e44259. doi:10.1371/journal.pone.0044259
Khan, I., Guru, S. K., Rath, S. K., Chinthakindi, P. K., Singh, B., Koul, S., et al. (2016). A novel triazole derivative of betulinic acid induces extrinsic and intrinsic apoptosis in human leukemia HL-60 cells. Eur. J. Med. Chem. 108, 104–116. doi:10.1016/j.ejmech.2015.11.018
Ko, J. H., Lee, J. H., Jung, S. H., Lee, S. G., Chinnathambi, A., Alharbi, S. A., et al. (2017). 2, 5-Dihydroxyacetophenone induces apoptosis of multiple myeloma cells by regulating the MAPK activation pathway. Molecules 22, 1157. doi:10.3390/molecules22071157
Kotla, R., Murugulla, A. C., Ruddarraju, R., Rao, M. V. B., Aparna, P., and Donthabakthuni, S. (2020). Synthesis and biological evaluation of 1, 3, 4-oxadiazole fused tetrazole amide derivatives as anticancer agents. Chem. Data Collect. 30, 100548. doi:10.1016/j.cdc.2020.100548
Lakshmithendral, K., Saravanan, K., Elancheran, R., Archana, K., Manikandan, N., Arjun, H. A., et al. (2019). Design, synthesis and biological evaluation of 2-(phenoxymethyl)-5-phenyl-1, 3, 4-oxadiazole derivatives as anti-breast cancer agents. Eur. J. Med. Chem. 168, 1–10. doi:10.1016/j.ejmech.2019.02.033
Lei, K. F., Wu, Z. M., and Huang, C. H. (2015). Impedimetric quantification of the formation process and the chemosensitivity of cancer cell colonies suspended in 3D environment. Biosens. Bioelectron. X. 74, 878–885. doi:10.1016/j.bios.2015.07.060
Liu, H. J., Huang, X., Shen, Q. K., Deng, H., Li, Z., and Quan, Z. S. (2021). Design, synthesis, and anticancer activity evaluation of hybrids of azoles and barbituric acids. Iran. J. Pharm. Res. 20, 144–155. doi:10.22037/ijpr.2020.113547.14363
Liu, X. J., Zhang, H. J., and Quan, Z. S. (2017). Synthesis and evaluation of the anticonvulsant activities of 2, 3-dihydrophthalazine-1, 4-dione derivatives. Med. Chem. Res. 26, 1935–1946. doi:10.1007/s00044-017-1896-8
Liu, Y., Li, J., Xu, H., Zhang, Y., Liu, Y., and Liu, X. (2009). Mitochondria-mediated tumstatin peptide-induced HepG2 cell apoptosis. Int. J. Mol. Med. 24, 653–659. doi:10.3892/ijmm_00000276
Liu, Y. X., Cui, Z. P., Liu, B., Cai, B. L., Li, Y. H., and Wang, Q. M. (2010). Design, synthesis, and herbicidal activities of novel 2-cyanoacrylates containing isoxazole moieties. J. Agric. Food Chem. 58, 2685–2689. doi:10.1021/jf902541w
Lu, Y., Van, D., Deibert, L., Bishop, G., and Balsevich, J. (2015). Antiproliferative quillaic acid and gypsogenin saponins from Saponaria officinalis L. roots. Phytochemistry 113, 108–120. doi:10.1016/j.phytochem.2014.11.021
Luan, T., Jin, C., Jin, C. M., Gong, G. H., and Quan, Z. S. (2019). Synthesis and biological evaluation of ursolic acid derivatives bearing triazole moieties as potential anti-Toxoplasma gondii agents. J. Enzyme Inhib. Med. Chem. 34, 761–772. doi:10.1080/14756366.2019.1584622
Ma, Q., Bian, M., Gong, G., Bai, C., Liu, C., Wei, C., et al. (2022). Synthesis and evaluation of bakuchiol derivatives as potent anti-inflammatory agents in vitro and in vivo. J. Nat. Prod. (Gorakhpur). 85, 15–24. doi:10.1021/acs.jnatprod.1c00377
Moses, T., Papadopoulou, K. K., and Osbourn, A. (2014). Metabolic and functional diversity of saponins, biosynthetic intermediates and semi-synthetic derivatives. Crit. Rev. Biochem. Mol. Biol. 49, 439–462. doi:10.3109/10409238.2014.953628
Newman, D. J., and Cragg, G. M. (2020). Natural products as sources of new drugs over the nearly four decades from 01/1981 to 09/2019. J. Nat. Prod. 83, 770–803. doi:10.1021/acs.jnatprod.9b01285
Pang, L., Liu, C. Y., Gong, G. H., and Quan, Z. S. (2020). Synthesis, in vitro and in vivo biological evaluation of novel lappaconitine derivatives as potential anti-inflammatory agents. Acta Pharm. Sin. B 10, 628–645. doi:10.1016/j.apsb.2019.09.002
Rashid, S., Dar, B. A., Majeed, R., Hamid, A., and Bhat, B. A. (2013). Synthesis and biological evaluation of ursolic acid-triazolyl derivatives as potential anti-cancer agents. Eur. J. Med. Chem. 66, 238–245. doi:10.1016/j.ejmech.2013.05.029
Rodriguez-Diaz, M., Delporte, C., Cartagena, C., Cassels, B. K., Gonzalez, P., Silva, X., et al. (2011). Topical anti-inflammatory activity of quillaic acid from Quillaja saponaria Mol. and some derivatives. J. Pharm. Pharmacol. 63, 718–724. doi:10.1111/j.2042-7158.2011.01263.x
Shang, F. F., Wang, M. Y., Ai, J. P., Shen, Q. K., Guo, H. Y., Jin, C. M., et al. (2021). Synthesis and evaluation of mycophenolic acid derivatives as potential anti-Toxoplasma gondii agents. Med. Chem. Res. 30, 2228–2239. doi:10.1007/s00044-021-02803-9
Shao, Y. P., Han, R. B., Wu, H. F., and Piao, F. Y. (2018). Synthesis and anticonvulsant activity of some novel 7-(benzylamino)-1H-benzo[b] [1, 4]diazepine-2, 4(3H, 5H)-dione derivatives. Med. Chem. Res. 27, 642–652. doi:10.1007/s00044-017-2089-1
Shen, Q. K., Deng, H., Wang, S. B., Tian, Y. S., and Quan, Z. S. (2019). Synthesis, and evaluation of in vitro and in vivo anticancer activity of 14-substituted oridonin analogs: A novel and potent cell cycle arrest and apoptosis inducer through the p53-MDM2 pathway. Eur. J. Med. Chem. 173, 15–31. doi:10.1016/j.ejmech.2019.04.005
Sheng, C., Che, X., Wang, W., Wang, S., Cao, Y., Miao, Z., et al. (2011). Design and synthesis of novel triazole antifungal derivatives by structure-based bioisosterism. Eur. J. Med. Chem. 46, 5276–5282. doi:10.1016/j.ejmech.2011.03.019
Wang, S., Chen, J., Shi, J., Wang, Z., Hu, D., and Song, B. (2021). Novel cinnamic acid derivatives containing the 1, 3, 4-oxadiazole moiety: Design, synthesis, antibacterial activities, and mechanisms. J. Agric. Food Chem. 69, 11804–11815. doi:10.1021/acs.jafc.1c03087
Wang, Z., Li, M. Y., Mi, C., Wang, K. S., Ma, J., and Jin, X. (2017). Mollugin has an anti-cancer therapeutic effect by inhibiting TNF-α-induced NF-κB activation. Int. J. Mol. Sci. 18, 1619. doi:10.3390/ijms18081619
Wei, Z. Y., Chi, K. Q., Wang, K. S., Wu, J., Liu, L. P., and Piao, H. R. (2018). Design, synthesis, evaluation, and molecular docking of ursolic acid derivatives containing a nitrogen heterocycle as anti-inflammatory agents. Bioorg. Med. Chem. Lett. 28, 1797–1803. doi:10.1016/j.bmcl.2018.04.021
Wei, Z. Y., Cui, B. R., Cui, X., Wu, Y. L., Fu, Y., Liu, L. P., et al. (2017). Design, synthesis, and negative inotropic evaluation of 4-phenyl-1H-1, 2, 4-triazol-5(4H)-one derivatives containing triazole or piperazine moieties. Chem. Biol. Drug Des. 89, 47–60. doi:10.1111/cbdd.12828
Yan, G. F., Ying, H. L., Yang, L., Fang, L. Y., Xuan, Y. Y., Jin, C. H., et al. (2021). Synthesis and antimicrobial activity evaluation of imidazole-fused imidazo[2, 1-b] [1, 3, 4]thiadiazole analogues. ChemMedChem 16, 2354–2365. doi:10.1002/cmdc.202100122
Yazici, B. N., Ersoy, E., Eroglu, O. E., Yazici, B. N., Boga, M., Boran, T., et al. (2021). Cytotoxic and apoptotic effects of Hypericum androsaemum on prostate adenocarcinoma (PC-3) and hepatocellular carcinoma (Hep G2) cell lines with identification of secondary metabolites by LC-HRMS. Turk. J. Chem. 45, 1621–1638. doi:10.3906/kim-2104-17
Yenmis, G., Yaprak Sarac, E., Besli, N., Soydas, T., Tastan, C., Dilek Kancagi, D., et al. (2021). Anti-cancer effect of metformin on the metastasis and invasion of primary breast cancer cells through mediating NF-kB activity. Acta Histochem. 123, 151709. doi:10.1016/j.acthis.2021.151709
Zhang, G. R., Ren, Y., Yin, X. M., and Quan, Z. S. (2018). Synthesis and evaluation of the anticonvulsant activities of new 5-substitued-[1, 2, 4]triazolo[4, 3-a]quinoxalin-4(5H)-one derivatives. Lett. Drug Des. Discov. 15, 406–413. doi:10.2174/1570180814666170619094408
Zhang, H. B., Shen, Q. K., Wang, H., Jin, C. M., Jin, C. M., and Quan, Z. S. (2018). Synthesis and evaluation of novel arctigenin derivatives as potential anti-Toxoplasma gondii agents. Eur. J. Med. Chem. 158, 414–427. doi:10.1016/j.ejmech.2018.08.087
Zhang, H. J., Zhang, G. R., Piao, H. R., and Quan, Z. S. (2018). Synthesis and characterisation of celastrol derivatives as potential anticancer agents. J. Enzyme Inhib. Med. Chem. 33, 190–198. doi:10.1080/14756366.2017.1404590
Zhang, L. H., Zhang, Z. H., Li, M. Y., Wei, Z. Y., Jin, X. J., and Piao, H. R. (2019). Synthesis and evaluation of the HIF-1α inhibitory activities of novel ursolic acid tetrazole derivatives. Bioorg. Med. Chem. Lett. 29, 1440–1445. doi:10.1016/j.bmcl.2019.04.028
Zhang, T., and Saghatelian, A. (2013). Emerging roles of lipids in BCL-2 family-regulated apoptosis. Biochimica Biophysica Acta - Mol. Cell Biol. Lipids 1831, 1542–1554. doi:10.1016/j.bbalip.2013.03.001
Zhang, T. Y., Li, C. S., Cui, M. Y., Bai, X. Q., Chen, J. H., Song, Z. W., et al. (2021). Design, synthesis and evaluation of dihydrotriazine derivatives-bearing 5-aryloxypyrazole moieties as antibacterial agents. Mol. Divers. 25, 861–876. doi:10.1007/s11030-020-10071-9
Zhang, T. Y., Li, C. S., Li, P., Bai, X. Q., Guo, S. Y., Jin, Y., et al. (2022). Synthesis and evaluation of ursolic acid-based 1, 2, 4-triazolo[1, 5-a]pyrimidines derivatives as anti-inflammatory agents. Mol. Divers. 26, 27–38. doi:10.1007/s11030-020-10154-7
Zhao, J. W., Guo, J. W., Huang, M. J., You, Y. Z., Wu, Z. H., Liu, H. M., et al. (2019). Design, synthesis and biological evaluation of new steroidal β-triazoly enones as potent antiproliferative agents. Steroids 150, 108431. doi:10.1016/j.steroids.2019.108431
Zhao, T., Yang, Y., Yang, J., Cui, Y., Cao, Z., Zuo, D., et al. (2021). Harmine-inspired design and synthesis of benzo[d]imidazo[2, 1-b]thiazole derivatives bearing 1, 3, 4-oxadiazole moiety as potential tumor suppressors. Bioorg. Med. Chem. 46, 116367. doi:10.1016/j.bmc.2021.116367
Keywords: quillaic acid, Western blot, antitumor, cell-cycle arrest, apoptosis
Citation: Huang X, Zhang C-H, Deng H, Wu D, Guo H-Y, Lee JJ, Chen F-E, Shen Q-K, Jin L-L and Quan Z-S (2022) Synthesis and evaluation of anticancer activity of quillaic acid derivatives: A cell cycle arrest and apoptosis inducer through NF-κB and MAPK pathways. Front. Chem. 10:951713. doi: 10.3389/fchem.2022.951713
Received: 24 May 2022; Accepted: 16 August 2022;
Published: 07 September 2022.
Edited by:
Marc Poirot, Centre de Recherche en Cancérologie de Toulouse (INSERM), FranceCopyright © 2022 Huang, Zhang, Deng, Wu, Guo, Lee, Chen, Shen, Jin and Quan. This is an open-access article distributed under the terms of the Creative Commons Attribution License (CC BY). The use, distribution or reproduction in other forums is permitted, provided the original author(s) and the copyright owner(s) are credited and that the original publication in this journal is cited, in accordance with accepted academic practice. No use, distribution or reproduction is permitted which does not comply with these terms.
*Correspondence: Li-Li Jin, amlubGlsaUB5YnUuZWR1LmNu; Zhe-Shan Quan, enNxdWFuQHlidS5lZHUuY24=
†These authors have contributed equally to this work