- 1College of Materials Science and Engineering, Chongqing University, Chongqing, China
- 2College of Engineering and Technology, Southwest University, Chongqing, China
Transition metal dichalcogenides (TMDs) have been widely explored for their excellent gas sensing properties, especially high sensitivity and stability at room temperature. MoTe2 exhibits good sensitivity and selectivity to some nitrogen-containing gases (i.e., NO2, NH3) and has received extensive attention in gas sensing. In addition, increasingly complex production environments place demands on high-quality gas sensors. Therefore, worldwide efforts are devoted to designing and manufacturing MoTe2-based gas sensors with faster response and recovery speed. This paper summarizes the research progress of MoTe2-based gas sensing, focuses on the practical measures to improve the response and recovery speed of MoTe2-based sensors, and discusses the mechanism. This provides guidance for exploring higher performance MoTe2 sensors.
Introduction
Presently, the detection of toxic and harmful gases has received extensive attention in many fields, such as industrial production, environmental regulation and medical diagnosis (Balendhran et al., 2013). In recent years, gas sensors based on semiconducting metal oxides and conductive polymers have developed rapidly, showing good gas sensing properties in many applications (Wetchakun et al., 2011), (Wang et al., 2020). It is worth noting that such sensors require high operating temperatures (usually higher than 200°C), which brings a series of unavoidable problems, such as high energy consumption and processing difficulties (Zhu and Zeng, 2017). Therefore, it is urgent to solve the problem of high operating temperature faced by gas sensors.
Semiconductor two-dimensional (2D) transition metal dichalcogenides (TMDs) have large specific surface areas and excellent surface energy levels (Rani et al., 2019), where the specific surface area represents the total area per unit mass of the material. The larger the specific surface area, the larger the contact surface provided under the same mass condition, which helps to improve the sensor’s response (Kim et al., 2020). Among them, molybdenum ditelluride (MoTe2) is a new member in 2D TMDs, which has longer bonds, lower binding energy, and a smaller band gap (about 1.0 eV) by comparison (Song et al., 2016). Long bonds lead to lower binding energy, which reduces the resistance of gas adsorption on the material’s surface, which is beneficial to improve the response speed of the sensor. It also makes the desorption more rapid, helping to shorten the recovery time of the sensor. A narrower band gap can effectively reduce the bulk resistance of the material and facilitate the transition of conduction band electrons, thereby improving sensitivity. These excellent properties make it promising as a new gas sensing material. Some experiments have demonstrated the feasibility of MoTe2 in gas sensing.
For example, Feng et al. (2017) prepared a field effect transistor (FET) based on MoTe2. Experiments found that after the material was rapidly annealed at 300°C, the polarity of the MoTe2 field effect transistor changed from n-type to p-type, with an apparent Schottky barrier. The authors used a p-type MoTe2 FET to detect the concentration of NO2 and exhibited a large response (140%) to 100ppb NO2 under optimal conditions of zero gate bias with excellent reversibility at 25 °C.The authors attribute the improved sensitivity to the modulation of the Schottky barrier of the p-type MoTe2 FET, fully demonstrating the potential of 2D MoTe2.
Shackery et al. (2018) employed a micromechanical lift-off method to fabricate back-to-back diodes (SDs) with unique structures, mainly by connecting MoTe2-based Schottky diodes in series. It was found that MoTe2 Schottky diodes exhibited better performance than MoTe2 field effect transistors in terms of response speed and recovery time when exposed to the same concentration of NO2 and NH3 atmospheres, at 70ppb NO2, the response time is only 15 s. The author believes that the gas is physically adsorbed on the surface of the material based on the van der Waals force, and the charge transfer is not sustainable due to the weak van der Waals force. When the external environment changes, the charge transfer also changes, which explains the fast recovery speed of MoTe2 SD. It is also confirmed that the two-dimensional SD based on MoTe2 has good application prospects in gas sensing.
Although MoTe2 has made some progress, similar to other low-dimensional semiconductor transition metal dichalcogenides (TMDs), the completed work has found that gas sensors based on MoTe2 also exhibit slower recovery performance (Balendhran et al., 2013). This obviously limits the further application of such sensors. At the same time, some toxic and harmful gases are harmful to our health even at low concentrations (Lee et al., 2022), so it is imperative to solve the reversibility problem of MoTe2 gas sensors so that they can detect gases quickly, sensitively and selectively (Zheng et al., 2021). In recent years, technicians have explored various methods to improve the gas-sensing properties of MoTe2 with fast adsorption/desorption kinetics, such as surface modification of the material, application of gate bias (Feng et al., 2017), have achieved ideal gas sensing performance, but these methods also face a choice between performance and cost. Therefore, we believe it is of great significance to explore a method that can significantly ameliorate the adsorption/recovery speed of the MoTe2 gas sensor while ensuring the material has good sensitivity and selectivity. In this review, we focus on the two most practical and feasible methods for MoTe2 gas-sensing materials in the current research, namely UV-light activation and additive doping, and also introduce the related performance improvement mechanism.
Research status of MoTe2-based gas sensors
UV light activation
As described above, MoTe2 has a narrow band gap, only about 1.0 eV, and this characteristic makes the photodetection range detectable by TMDs become wider, extending from the visible light to the near-infrared range. Recent experiments have found that MoTe2-based photodetectors also exhibit good responses over a wider spectral range (Su et al., 2019), which inspired us to consider the illumination factor to solve the MoTe2 recovery problem.
Ultraviolet light has gotten extensive attention in gas sensing as excitation energy. When the sensor is exposed to UV light with energy higher than the MoTe2 band gap (about 1.0 eV), electrons in the valence band are excited to migrate to the conduction band, forming electron-hole pairs with holes in MoTe2 (Zhou et al., 2018). Under the action of the electric field, electrons diffuse into MoTe2 while holes migrate to the surface. At this time, the adsorbed oxygen on the surface of MoTe2 will react with these holes (Kozawa et al., 2014), resulting in desorption. The remaining electrons in the MoTe2 channel reduce the depletion layer, increasing the gas sensor’s current (Kang et al., 2019). When the gas sensor comes into contact with the analyte, the resistance changes of the sensor due to the gas adsorption and desorption process will also be amplified under ultraviolet light. Thus, the high response and fast recovery of the MoTe2-based gas sensor are achieved. We summarize some information on the gas sensing performance of MoTe2-based gas sensors under UV light conditions, and the specific details are shown in Table 1. The sensor response is defined as (G—G0)/G0 × 100%, where G0 and G are the channel conductances before and 5 min after analyte exposure, respectively.
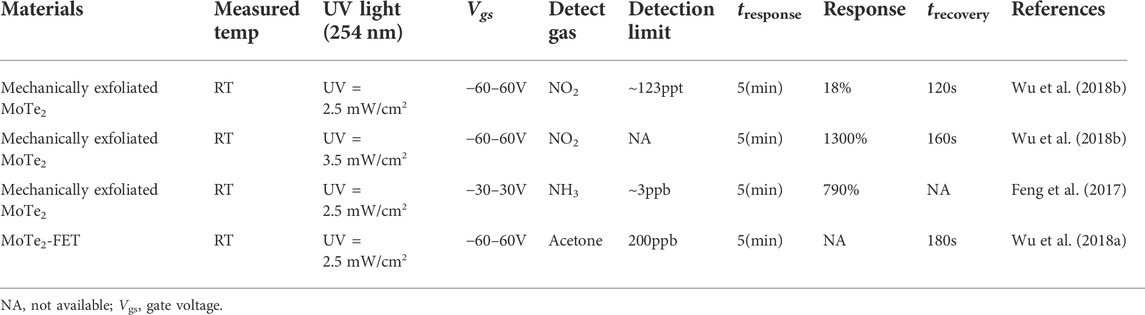
TABLE 1. Summary of gas sensing properties of MoTe2-based gas sensors activated by ultraviolet light.
Wu et al. (2018b) fabricated a sensor based on p-type MoTe2 (Figure 1B) and detected low-concentration NO2 under UV illumination. It was found that under the irradiation of 254 nm ultraviolet light, the adsorbed oxygen could be removed, providing more active sites for NO2 adsorption, which greatly improved the sensitivity of the sensor, and the detection limit reached 252ppt. What’s more, such sensors can be fully reversible in a short time under UV light, compared with even less than half of the recovery rate under the condition of no light. In addition, the MoTe2 sensor has excellent selectivity to NO2 in the atmosphere and ignores humidity interference, indicating that this type of sensor has practical application prospects.
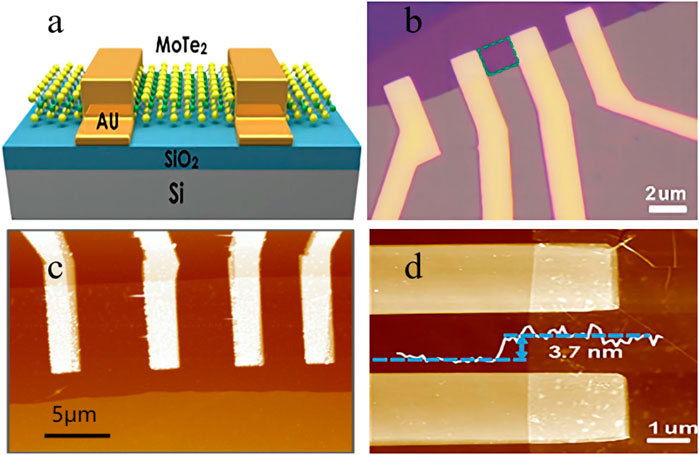
FIGURE 1. (A) Schematic diagram of MoTe2 transistor. (B) Optical image of the device. Scale bar is 2 μm. The sensing channel is highlighted by the green dashed rectangle. (C) Atomic force microscopy (AFM) topography image of the MoTe2 FET. Thickness of the MoTe2 is 3.4 nm. (D) AFM image of the device. The white curve is an AFM height profile.
Similarly, Feng, Z.H. and co-workers (Feng et al., 2017) reported that MoTe2 field effect transistors (FETs) (Figure 1A) performed control sensing detection of different concentrations of NH3 under both dark and 254 nm UV light irradiation conditions (Figure 1C). The experiment found that the gas sensor showed an ultra-high response speed to NH3 under ultraviolet light irradiation and reduced the detection limit. The results confirm that light strongly affects the gas sensing performance of MoTe2, and it is promising ultrasensitive sensing.
Wu et al. (2018a) fabricated MoTe2 FETs (Figure 1D) in another experiment to explore the effect of UV light on the detection performance of acetone. Under illumination conditions, the transistor showed the opposite response phenomenon, current increased with the increase of acetone concentration. The authors believe that acetone is adsorbed on the material’s surface to form holes, resulting in the reduction of the Fermi level of MoTe2 and the narrowing of the Schottky barrier width. In addition, the sensor also showed good sensitivity and a low detection limit under UV irradiation. Undoubtedly, this unique light-responsive property can discriminately detect acetone in the atmosphere and is expected to be developed into a high-performance gas sensor.
Additive doping
In addition to UV light activation, doping additives are also a common modification measure, especially in gas sensing (Zhang et al., 2022).
Mote2 sheet comprises three hexagonal layers, two atomic layers of Te and one of Mo between them, and the atoms in the plane pass through normal covalently bonded (Zappa, 2017). The layers are mainly maintained by van der Waals forces, and the bonding is relatively weak.
As far as we know, doping transition metals on the surface of nanomaterials can effectively improve their electron mobility and activity (Kumar et al., 2020), especially for some noble metal dopants, which can improve the electrical properties of materials and also improve the gas adsorption properties on the surface of materials (Hou et al., 2021). Doping transition metals can effectively improve the sensitivity of gas-sensing materials, introducing more chemically active sites. In addition, selecting appropriate transition metals for doping will change the material’s bulk resistance and amplify the material’s resistance change during the gas adsorption process, thereby improving the sensor’s sensitivity (Szary et al., 2022a). The mechanism of the MoTe2 gas sensor is inseparable from the charge transfer between the adsorbed gas and the gas-sensing material (Agrawal et al., 2021). The gas to be tested acts as a carrier donor or acceptor during the adsorption/desorption process, thereby changing the resistance of the gas-sensing element.
Liu, Y. and others (Liu et al., 2021) explored the effect of doping four transition metals Pd, Pt, Ag and Au (Figure 2) on the gas sensing properties of MoTe2 sensors based on density functional theory (DFT). When NO2 molecules are chemically adsorbed on the material’s surface, they show a strong electron accepting behavior, especially after Au doping. With the adsorption of NO2, the band gap of the Au-MoTe2 monolayer increases significantly, and the electrical conductivity changes greatly. It is demonstrated that the transition metal-doped MoTe2 monolayer has excellent potential for NO2 detection.
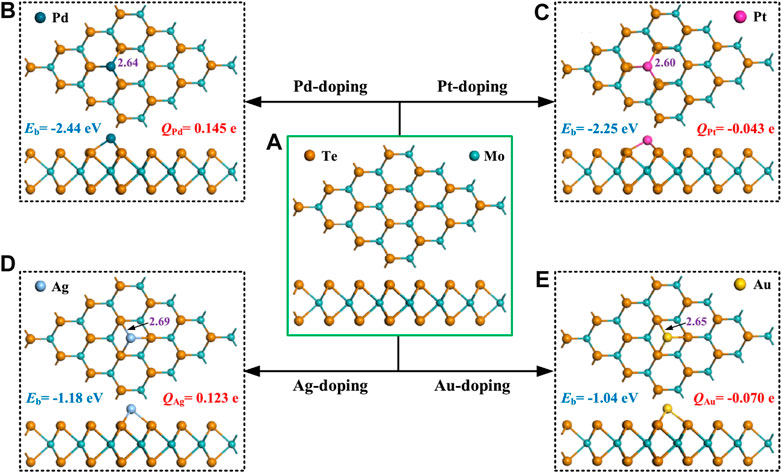
FIGURE 2. MSC of (A) pristine, (B) Pd-doped, (C) Pt-doped, (D) Ag-doped and (E) Au-doped MoTe2 monolayer. The violet values are bond lengths of TM-Te, unit in Å.
Cao et al. (2021) chose Ni with good activity and electron mobility as the doping metal, based on the first principle, to explore the gas sensitivity of monolayer Ni-MoTe2 to nitrogen oxide gas-sensing performance. The calculation shows that metal Ni reacts with the adsorbed gas to significantly increase the surrounding electron density, which shows a stronger binding force to NOx than MoTe2 without Ni doped. The change of band gap during the adsorption process is more prominent, which will also lead to a change in the conductivity of the gas sensor with NOx adsorption.
Panigrahi et al. (2019) used dispersion-corrected DFT to explore changes in the sensing performance of Sb-doped MoTe2 for nitrogen-containing gases. The substitution of Sb for Te increases the defect concentration and also enhances the binding energy. Under oxynitride conditions, substantial charge transfer occurs. At a doping concentration of 2.08%, the material exhibits a good affinity for NO and NO2. This theoretically proves that Sb-MoTe2 has broad application prospects in a new generation of gas sensors.
In other work, Szary, M.J. and others (Szary et al., 2022b) calculated the doping of Al, Si, P, S and Cl atoms on Te vacancies in MoTe2 based on density functional theory and discussed the sensing performance for CO and CO2. The calculation found that P-doped MoTe2 increased the charge transfer of the adsorption of the two gases, but the effect on the two gases was not correlated. The relative response value of the doped material to (CO/CO2) increased from 1.5 rose to 5.6, showing good selectivity for CO/CO2. It is expected to be further developed in the field of sensing.
In addition, Zhu, H.L. and others (Zhu et al., 2020) explored the gas-sensing properties of Rh-MoTe2 for SF6 decomposition products based on first-principles, and found that Rh-MoTe2 monolayer showed good adsorption performance for SOF2. Jiang et al. (2022) chose the metal ruthenium with high chemical activity as the doping element, and calculated the adsorption of SF6 decomposition products on Ru-MoTe2 monolayer based on density functional theory, the adsorption capacity of the system to the decomposition products was significantly improved after Ru doping. Liu, Z.C. and others (Liu et al., 2022) calculated the adsorption capacity of several harmful gases on monolayer Au-MoTe2 based on density functional theory, and Au-MoTe2 had the strongest interaction with NO2.
A series of ideal theoretical calculations illustrate the feasibility of additive doping in improving the sensitivity and selectivity of MoTe2, providing a theoretical basis for the further development of MoTe2-based gas sensors.
Conclusion
In general, this paper summarizes the research status of MoTe2-based gas sensors and finds that applying gate bias, UV light activation, and additive doping can effectively improve the performance of MoTe2-based sensors. Under UV light irradiation, the oxygen adsorbed on the material’s surface is effectively removed, providing more active sites for the analyte, thereby significantly improving the sensor’s sensitivity. Many calculations based on density functional theory and first-principles have also confirmed from the theoretical level that additive doping can effectively improve the charge transfer during gas adsorption, pointing the way to the future of high-performance MoTe2-based gas sensors. Although the current work has achieved specific results, more efforts are needed to apply the theory to practice, and it is hoped that our work can guide for exploration of MoTe2-based gas sensors.
Author contributions
JW: conceptualization, methodology, writing-original draft preparation. WZ: data curation, investigation. QZ and WZ: supervision.
Funding
This work was supported by Chongqing Postgraduate Research and Innovation Project (CYS22003).
Conflict of interest
The authors declare that the research was conducted in the absence of any commercial or financial relationships that could be construed as a potential conflict of interest.
Publisher’s note
All claims expressed in this article are solely those of the authors and do not necessarily represent those of their affiliated organizations, or those of the publisher, the editors and the reviewers. Any product that may be evaluated in this article, or claim that may be made by its manufacturer, is not guaranteed or endorsed by the publisher.
References
Agrawal, A. V., Kumar, N., and Kumar, M. (2021). Strategy and future prospects to develop room-temperature-recoverable NO2 gas sensor based on two-dimensional molybdenum disulfide. Nanomicro. Lett. 13, 38. doi:10.1007/s40820-020-00558-3
Balendhran, S., Walia, S., Nili, H., Ou, J. Z., Zhuiykov, S., Kaner, R. B., et al. (2013). Two-dimensional molybdenum trioxide and dichalcogenides. Adv. Funct. Mat. 23, 3952–3970. doi:10.1002/adfm.201300125
Cao, W., Zhao, Q., Yang, L., and Cui, H. (2021). Enhanced NOx adsorption and sensing properties of MoTe2 monolayer by Ni-doping: A first-principles study. Surfaces Interfaces 26, 101372. doi:10.1016/j.surfin.2021.101372
Feng, Z. H., Xie, Y., Chen, J. C., Yu, Y. Y., Zheng, S. J., Zhang, R., et al. (2017). Highly sensitive MoTe2 chemical sensor with fast recovery rate through gate biasing. 2D Mat. 4, 025018. doi:10.1088/2053-1583/aa57fe
Hou, W. J., Mi, H. W., Peng, R. C., Peng, S. D., Zeng, W., Zhou, Q., et al. (2021). First-principle insight into Ga-doped MoS2 for sensing SO2, SOF2 and SO2F2. Nanomaterials 11, 314. doi:10.3390/nano11020314
Jiang, K. R., Yue, L., Xu, X., Qian, X. Y., Yan, L., Zhao, L. Z., et al. (2022). Adsorption and sensing for SF6 decomposition products by Ru-MoTe2 monolayer. Phys. Status Solidi, 2200070. doi:10.1002/pssb.202200070
Kang, Y., Pyo, S., Jo, E., and Kim, J. (2019). Light-assisted recovery of reacted MoS2 for reversible NO2 sensing at room temperature. Nanotechnology 30, 355504. doi:10.1088/1361-6528/ab2277
Kim, Y., Lee, S., Song, J. G., Ko, K. Y., Woo, W. J., Lee, S. W., et al. (2020). 2D transition metal dichalcogenide heterostructures for p- and n-type photovoltaic self-powered gas sensor. Adv. Funct. Mat. 30, 2003360. doi:10.1002/adfm.202003360
Kozawa, D., Kumar, R., Carvalho, A., Amara, K. K., Zhao, W. J., Wang, S. F., et al. (2014). Photocarrier relaxation pathway in two-dimensional semiconducting transition metal dichalcogenides. Nat. Commun. 5, 4543. doi:10.1038/ncomms5543
Kumar, R., Zheng, W., Liu, X. H., Zhang, J., and Kumar, M. (2020). MoS2-Based nanomaterials for room-temperature gas sensors. Adv. Mat. Technol. 5, 1901062. doi:10.1002/admt.201901062
Lee, J., Choi, Y., Park, B. J., Han, J. W., Lee, H. S., Park, J. H., et al. (2022). Precise control of surface oxygen vacancies in ZnO nanoparticles for extremely high acetone sensing response. J. Adv. Ceram. 11, 769–783. doi:10.1007/s40145-022-0570-x
Liu, Y., Shi, T., Si, Q. L., and Liu, T. (2021). Adsorption and sensing performances of transition metal (Pd, Pt, Ag and Au) doped MoTe2 monolayer upon NO2: A DFT study. Phys. Lett. A 391, 127117. doi:10.1016/j.physleta.2020.127117
Liu, Z. C., Gui, Y. G., Xu, L. N., and Chen, X. P. (2022). Adsorption and gas-sensing properties of Aun (n = 1-3) cluster doped MoTe2 for NH3, NO2, and SO2 gas molecules. Surfaces Interfaces 30, 101883. doi:10.1016/j.surfin.2022.101883
Panigrahi, P., Hussain, T., Karton, A., and Ahuja, R. (2019). Elemental substitution of two-dimensional transition metal dichalcogenides (MoSe2 and MoTe2): Implications for enhanced gas sensing. ACS Sens. 4, 2646–2653. doi:10.1021/acssensors.9b01044
Rani, A., Dicamillo, K., Khan, M. a. H., Paranjape, M., and Zaghloul, M. E. (2019). Tuning the polarity of MoTe2 FETs by varying the channel thickness for gas-sensing applications. Sensors 19, 2551. doi:10.3390/s19112551
Shackery, I., Pezeshki, A., Park, J. Y., Palanivel, U., Kwon, H. J., Yoon, H. S., et al. (2018). Few- layered a-MoTe2 Schottky junction for a high sensitivity chemical- vapour sensor. J. Mat. Chem. C Mat. 6, 10714–10722. doi:10.1039/c8tc02635a
Song, S., Keum, D. H., Cho, S., Perello, D., Kim, Y., Lee, Y. H., et al. (2016). Room temperature semiconductor-metal transition of MoTe2 thin films engineered by strain. Nano Lett. 16, 188–193. doi:10.1021/acs.nanolett.5b03481
Su, J. W., Liu, K. L., Wang, F. K., Jin, B., Guo, Y. B., Liu, G. H., et al. (2019). Van der Waals 2D transition metal tellurides. Adv. Mat. Interfaces 6, 1900741. doi:10.1002/admi.201900741
Szary, M. J., Babelek, J. A., and Florjan, D. M. (2022a). Dopant-sheet interaction and its role in the enhanced chemical activity of doped MoTe2. Surf. Sci. 723, 122093. doi:10.1016/j.susc.2022.122093
Szary, M. J., Florjan, D. M., and Babelek, J. A. (2022b). Selective detection of carbon monoxide on P-block doped monolayers of MoTe2. ACS Sens. 7, 272–285. doi:10.1021/acssensors.1c02246
Wang, Y. M., Liu, A. P., Han, Y. Q., and Li, T. X. (2020). Sensors based on conductive polymers and their composites: A review. Polym. Int. 69, 7–17. doi:10.1002/pi.5907
Wetchakun, K., Samerjai, T., Tamaekong, N., Liewhiran, C., Siriwong, C., Kruefu, V., et al. (2011). Semiconducting metal oxides as sensors for environmentally hazardous gases. Sensors Actuators B Chem. 160, 580–591. doi:10.1016/j.snb.2011.08.032
Wu, E. X., Xie, Y., Yuan, B., Hao, D. Y., An, C. H., Zhang, H., et al. (2018a). Specific and highly sensitive detection of ketone compounds based on p-type MoTe2 under ultraviolet illumination. ACS Appl. Mat. Interfaces 10, 35664–35669. doi:10.1021/acsami.8b14142
Wu, E. X., Xie, Y., Yuan, B., Zhang, H., Hu, X. D., Liu, J., et al. (2018b). Ultrasensitive and fully reversible NO2 gas sensing based on p-type MoTe2 under ultraviolet illumination. ACS Sens. 3, 1719–1726. doi:10.1021/acssensors.8b00461
Zappa, D. (2017). Molybdenum dichalcogenides for environmental chemical sensing. Materials 10, 1418. doi:10.3390/ma10121418
Zhang, Y., Han, S., Wang, M. Y., Liu, S. W., Liu, G. W., Meng, X. F., et al. (2022). Electrospun Cu-doped In2O3 hollow nanofibers with enhanced H2S gas sensing performance. J. Adv. Ceram. 11, 427–442. doi:10.1007/s40145-021-0546-2
Zheng, W., Liu, X. H., Xie, J. Y., Lu, G. C., and Zhang, J. (2021). Emerging van der Waals junctions based on TMDs materials for advanced gas sensors. Coord. Chem. Rev. 447, 214151. doi:10.1016/j.ccr.2021.214151
Zhou, Y., Zou, C., Lin, X. G., and Guo, Y. C. (2018). UV light activated NO2 gas sensing based on Au nanoparticles decorated few-layer MoS2 thin film at room temperature. Appl. Phys. Lett. 113, 082103. doi:10.1063/1.5042061
Zhu, H. L., Cui, H., He, D., Cui, Z. W., and Wang, X. (2020). Rh-Doped MoTe2 monolayer as a promising candidate for sensing and scavenging SF6 decomposed species: A DFT study. Nanoscale Res. Lett. 15, 129. doi:10.1186/s11671-020-03361-6
Keywords: MoTe2, gas sensing, transition metal dichalcogenide, additive doping, UV activation
Citation: Wang J, Zeng W and Zhou Q (2022) Research status of gas sensing performance of MoTe2-based gas sensors: A mini review. Front. Chem. 10:950974. doi: 10.3389/fchem.2022.950974
Received: 24 May 2022; Accepted: 05 July 2022;
Published: 22 July 2022.
Edited by:
Ashok Mulchandani, University of California, Riverside, United StatesReviewed by:
Maciej Szary, Poznań University of Technology, PolandMohammed Sedki, University of California, Riverside, United States
Copyright © 2022 Wang, Zeng and Zhou. This is an open-access article distributed under the terms of the Creative Commons Attribution License (CC BY). The use, distribution or reproduction in other forums is permitted, provided the original author(s) and the copyright owner(s) are credited and that the original publication in this journal is cited, in accordance with accepted academic practice. No use, distribution or reproduction is permitted which does not comply with these terms.
*Correspondence: Wen Zeng, wenzeng@cqu.edu.cn; Qu Zhou, zhouqu@swu.edu.cn