- 1School of Chemistry and Chemical Engineering, Nantong University, Nantong, China
- 2The Key Laboratory of Synthetic and Biological Colloids, Ministry of Education, School of Chemical and Material Engineering, Jiangnan University, Wuxi, China
Multiple emulsions are widely used in pharmaceuticals, foods, and cosmetics. However, those stabilized by surfactants with different HLB values are generally unstable due to the diffusion of the surfactants between inner and outer interfaces. Here, we report that multiple W/O/W emulsions can be prepared by using the same particles in combination with a surfactant of different concentrations. The less surface-active raw CaCO3 nanoparticles can be hydrophobized to surface-active in situ by adsorption of the anionic surfactant SDS, and the wettability of the particles can be controlled to be suitable for stabilizing both O/W and W/O Pickering emulsions by adjusting the surfactant concentration. With toluene as oil phase, the CaCO3 particles at 1.0 wt% tend to stabilize a W/O emulsion in the presence of 3 mm SDS in an aqueous solution, which can then be further dispersed in an aqueous phase with 1.0 wt% CaCO3 and SDS below 1 mm to form a W/O/W multiple emulsion. The effects of the ratio of W/O emulsion to the outer water phase and the preparation methods on stabilization of multiple emulsions were examined. With a ratio smaller than 3:1 and by gentle magnetic stirring, the multiple emulsions obtained can stay stable for at least a month without coalescence. This simple method not only ensures stabilization of multiple emulsions but also avoids complicated synthesis of colloid particles with different wettability.
1 Introduction
Emulsions are heterogeneous systems of two immiscible liquids, with one liquid dispersed in the other as droplets (Becher, 1983). The immiscible liquids involved are mostly oil and water; therefore, emulsions are normally either oil-in-water (O/W) or water-in-oil (W/O) types. For stabilization of emulsions, it is necessary to add emulsifiers including surfactants, amphiphilic polymers, and colloid particles, which can reduce the interfacial tension and endow droplets with charges or steric films through interfacial adsorption. While emulsions stabilized by surfactants are relatively unstable, those stabilized by amphiphilic colloid particles are ultrastable due to the high adsorption free energy of the particles at the fluid interface, which makes the adsorption almost irreversible ( Binks, 2002; Aveyard et al., 2003; Kruglyakov and Nushtayeva, 2004; Binks et al., 2006).
Multiple emulsions are complex systems with both O/W and W/O microstructures in one system, in which droplets of emulsions in the internal phase are dispersed in the external phase (Florence and Whitehill, 1982; Bonnet et al., 2010; Li et al., 2012; Cizauskaite et al., 2017; Panagopoulou et al., 2017). There are mainly two types of multiple emulsions, W/O/W and O/W/O types. In W/O/W multiple emulsions, W/O emulsion is additionally dispersed in a water phase, whereas in O/W/O emulsions, O/W emulsion is additionally dispersed in an oil phase. Multiple emulsions were first found by Seifritz in 1925 (Seifritz, 1925) and first used in drug vehicles in 1968 (Engel et al., 1968). In recent years, multiple emulsions have aroused great interest because they have wide applications in pharmaceuticals (Moussaoui et al., 2016), chemicals (Liu et al., 2017), foods (Lutz et al., 2009; Jiménezcolmenero, 2013), cosmetics (Hoppel et al., 2015; Wang et al., 2017), materials (Akbaba et al., 2017), and many other fields (Cournarie et al., 2004; Zhao et al., 2022). The preparation of multiple emulsions includes one-step and two-step approaches (Morais et al., 2009; Fernández-Martín et al., 2017), with the two-step method being more common (Fernández-Martín et al., 2017). The stability of multiple emulsions against coalescence is determined by several factors including internal/external phase volume ratio, hydrophobic/hydrophilic emulsifiers, and the species of oil phase (Taisne et al., 1996; Keshmiri et al., 2016; Zhao et al., 2022), in which emulsifiers play normally a critical role. Traditional multiple emulsions are obtained by using two surfactants of different HLB, one is hydrophilic and the other one is hydrophobic. For example, Cole and Whateley, (1997) demonstrated the effects of Pluronic F127 and polyacrylic acid (PAA) on the stability of multiple W/O/W emulsions (Cole and Whateley, 1997).
As mentioned above, emulsions stabilized by surfactants are thermodynamically unstable, and the multiple emulsions generated with surfactants as emulsifiers are similarly thermodynamically unstable due to the diffusion of two surfactants between the inner and outer interfaces, which aggravatingly accelerates droplet coalescence. For improving the stability of the multiple emulsions, many researchers have used additional substances such as polymers, microparticles, and nanoparticles (Riess and Labbe, 2004; Hong et al., 2012; Clegg et al., 2015; Maciel et al., 2015; Mozafari, 2015; Zamani et al., 2018; Ghasemi et al., 2020a; Ghasemi et al., 2020b; Saffarionpour and Diosady, 2021; Zhao et al., 2022). Particles as emulsifiers were considered better than surfactants because of their firmer adsorption at the interface, which constitutes a solid obstacle against coalescence. However, most of these particles are functional polymers, and their synthesis is relatively complex.
CaCO3 nanoparticles are the commercial nanoparticles with probably the highest output and cheapest price in the world. Moreover, they are edible and friendly to the environment, which leads to their wide use in the fields of plastics, paper, medicine, food products, and so on. In previous studies, it has been found that although natural particles have no strong surface activity, they can be in situ hydrophobized by absorption of surfactants with opposite charges to become surface active (Cui et al., 2008; Cui et al., 2012). Different from ex situ hydrophobization, where chemical modifications are usually involved, in situ hydrophobization is a physical process in solution, where the wettability of the particles is modified by adsorption of the surfactant via electrostatic interaction, and the wettability can be adjusted by means of the concentration and structure of the surfactant (Cui et al., 2008). Herein, we report that Pickering multiple emulsions can be prepared using the same materials (CaCO3 nanoparticle and surfactant) as emulsifiers for the internal and external interfaces and demonstrate the behaviors of the multiple emulsions formed. The use of in situ surface-modified CaCO3 nanoparticles as an emulsifier is very convenient, which avoids the complicated synthesis of the functional particles.
2 Experimental
2.1 Materials
Calcium carbonate (CaCO3) nanoparticles with a purity of 98% were purchased from Keli New Materials Co. Ltd, Henan, China. Sodium dodecyl sulfate (SDS, 99%) was purchased from Sigma. All other chemicals including toluene (99.5%), HCl (≥99.5%), and NaOH (≥99.5%) were purchased from Sinopharm Chemical Reagent Co. Ultrapure water (resistance = 18.2 MΩ, pH = 6.8 at 25°C) was provided by Huawei Co. Ltd., Wuxi, China.
2.2 Methods
2.2.1 Aqueous dispersion of CaCO3 particles
Powdered CaCO3 particles were weighted into a screw-cap glass vial [2.5(d) cm × 6.5 (h) cm], and then pure water or SDS solutions were added. The particles were then dispersed by using an ultrasound probe (FS-250N, Shanghai ShengXi Co.) operating at 50 W for 2 min to obtain dispersion 1.
2.2.2 Preparation of W/O Pickering emulsions and W/O/W multiple emulsions
1) W/O Pickering emulsions.
7 ml dispersion 1 was transferred into a glass vial [2.5 (d) cm ×6.5 (h) cm], followed by the addition of 7 ml toluene, with oil/water volume ratio = 1:1. The mixture was then homogenized by using an ultraturrax homogenizer (IKA T18 basic) with S18N-10G head working at 7,000 rpm for 2 min.
2) W/O/W Pickering multiple emulsions.
Another dispersion (dispersion 2) of CaCO3 nanoparticles in SDS solutions with lower SDS concentration was prepared using a similar procedure as described above, which was transferred to a glass vial [2.5 (d) cm ×6.5 (h) cm], and then the W/O/W Pickering multiple emulsions were prepared by using two different methods as described below.
Method 1: The dispersion 2 was stirred using a magnetic stirrer (Guohua 76, CHangzhou Guohua Co.) operating at 1,400 rpm, and the W/O Pickering emulsions previously prepared were added drop by drop.
Method 2: Both dispersion 2 and the W/O Pickering emulsion were added to the glass vial at a certain volume ratio, and the mixture was then homogenized using the IKA T18 basic ultraturrax homogenizer at 3,500 rpm for 2 min.
2.2.3 Characterization of the emulsions prepared
The type of the simple emulsion prepared was verified by the drop test (Cui et al., 2010a), and the photos of the simple/multiple Pickering emulsions were taken using a digital camera at different times for observing the stability of the emulsions. For observing the microstructure of the emulsions, emulsion samples were dropped on a glass slide followed by diluting with the continuous phase, and the micrographs of the simple/multiple Pickering emulsions were taken by using a VHX-1000 microscope system (Keyence Co.).
2.2.4 Zeta potential and particle size
1 wt% CaCO3 nanoparticles were dispersed in pure water at different pH adjusted by HCl or NaOH at 25°C. The dispersions were then stood for about 24 h at the same temperature to reach equilibrium. The zeta potentials were then measured by using Zetasizer 2000 (Malvern) at 25°C. For measuring the size of CaCO3 nanoparticles, 1 wt% CaCO3 nanoparticles were dispersed in pure water and 1 mm SDS solution using the using ultrasound probe, and the size of the particles was measured using Zetasizer Nano-ZS90 (Malvern) at 25°C.
2.2.5 Adsorption isotherm of SDS on CaCO3 nanoparticles
The dispersions with 0.4 g CaCO3 nanoparticles and 20 ml aqueous solution of SDS at different concentrations were prepared as described above and left in a thermostatic chamber overnight at 25°C. Then, the suspended particles were removed from the dispersion by centrifugation (5,000 rpm, 15 min). To determine the equilibrium concentration of SDS in the supernatant, a two-phase titration method (Cui et al., 2010b) was used, and the adsorbed amount (Γ) of SDS on the CaCO3 nanoparticles was calculated by:
where C0 and C are the initial and equilibrium concentrations (mol/L) of SDS in the solution, respectively; V is the volume (ml) of the SDS solution; and w is the weight (g) of the CaCO3 particles. At the equilibrium concentration of SDS below 0.5 mm, the equilibrium concentration of SDS was determined by a spectrophotometric method (Cui et al., 2008).
2.2.6 Contact angles of SDS solution on a CaCO3 substrate
A piece of CaCO3 stone of 99 wt% was polished to be a smooth plane. The contact angles of drops of SDS solutions at different concentrations on the particle surface in the air were measured by Drop Meter-A-100, an optical drop shape analyzer from Ningbo HaishuMaishi Scientific Test Co. After one measurement (several drops) was finished, the surface of the CaCO3 stone was polished and washed for the next measurements.
3 Results and discussion
3.1 Characterization of CaCO3 nanoparticles
The CaCO3 nanoparticles used are quasi-spherical that have a primary diameter 80–120 nm, according to the SEM image shown in Figure 1. The surface area is 16.21 m2/measured by BET. Assuming the particle is spherical, the calculated diameter is 71.16 nm, which is in good agreement with the SEM image. Figure 2 shows the zeta potential of the particles at different pH values, which decreases with increasing pH, and the bare CaCO3 nanoparticles have an isoelectric point of 9.35. At pH < 9.35, the CaCO3 particles are positively charged, and on the contrary, the particles are negatively charged. Therefore, the CaCO3 particles are positively charged when dispersed in pure water with the pH of dispersion being 8.93 (<9.35).
The appearance of 1 wt% CaCO3 nanoparticles dispersed in pure water at different pH is illustrated in Figure 3. It is seen that the particles are almost all sediment after 1 h, and the sedimentation layer of the particles increases as pH increases, which can be explained by the DLVO theory. Sedimentation or dispersion depends on the attraction and electrostatic repulsion between the particles. When the electrostatic repulsion is stronger, the distance between particles increases. Figure 3 suggests that at high pH, the increased zeta potential results in stronger repulsion and, therefore, a thicker sedimentation layer.
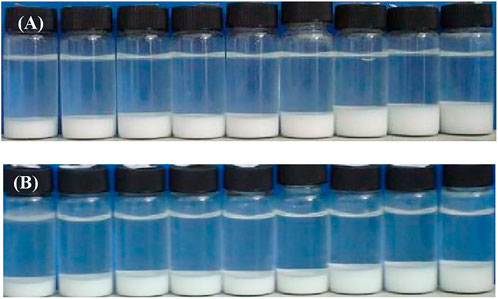
FIGURE 3. Appearance of 1.0 wt% CaCO3 nanoparticles dispersed in aqueous solutions with HCl or NaOH at different concentration (from left to right) (HCl)1 × 10−2,1 × 10−3,1 × 10−4, and 1 × 10−5 mol/L, water (NaOH)1 × 10−5,1 × 10−4,1 × 10−3, and 1 × 10−2 mol/L, taken 1 h (A) and 1 week (B) after preparation.
3.2 Pickering emulsions co-stabilized by CaCO3 nanoparticles and SDS
The emulsions (toluene as oil phase) formed by CaCO3 nanoparticles alone are not very stable. It seems to be stable from the appearance shown in Figure 4A, but coalesced oil appeared on the top of the vessels, which can be seen clearly after the emulsions were kept for 1 week, as shown in Figure 4B. This indicates that the bare CaCO3 nanoparticles are hydrophilic and weakly surface-active.
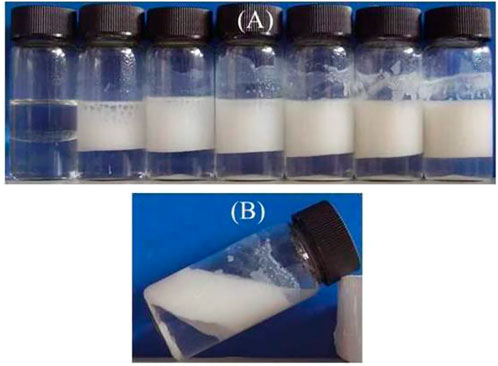
FIGURE 4. Appearance of toluene-in-water emulsions stabilized solely by CaCO3nanoparticles, taken 1 week after preparation. Particle concentration (A) left to right 0, 0.1, 0.3, 0.5, 1.0, 1.5, and 2.0 wt% (B) 1.0 wt%.
Figure 5 shows that using SDS alone as an emulsifier under 3 mm, no stable emulsion can be obtained. However, once CaCO3 nanoparticles and SDS solution coexist, toluene-in-water emulsions were formed, which kept still stable after a week. Meanwhile, double-phase inversion (Cui et al., 2008) of O/W (1)→W/O→O/W (2) was observed by increasing SDS concentration, as shown in Figure 6. When the concentration of SDS is lower than 1 mm, all the emulsions are O/W type Pickering emulsions, recorded as O/W (1), and when the concentration of SDS increases to 3 mm, the oil phase reverts to be continuous phase, leading to formation of W/O Pickering emulsions. Finally, when the concentration of SDS is beyond 10 mm, the second phase inversion of W/O→O/W (2) takes place, where the O/W (2) emulsion is stabilized by SDS. The micrographs in Figure 7 show that the average droplet sizes of O/W (1) and W/O emulsions have little difference but are much bigger than those stabilized by SDS alone and of O/W (2) emulsions, which suggests that both O/W (1) and W/O emulsions are stabilized by the in situ hydrophobized particles instead of SDS molecules, while O/W (2) emulsions are stabilized mainly by SDS, where the concentration is over its CMC.
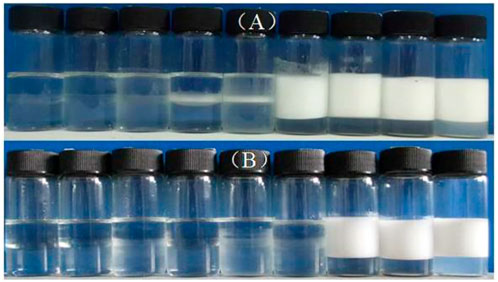
FIGURE 5. Photographs of toluene-in-water emulsions stabilized by SDS alone at different concentration, taken (A) 24 h and (B) 1 week after preparation. Concentration of SDS (from left to right):3 × 10−5, 6 × 10−5, 1 × 10−4, 3 × 10−4, 6 × 10−4, 1 × 10−3, 3 × 10−3, 6 × 10−3, and 1 × 10−2 mol/L.
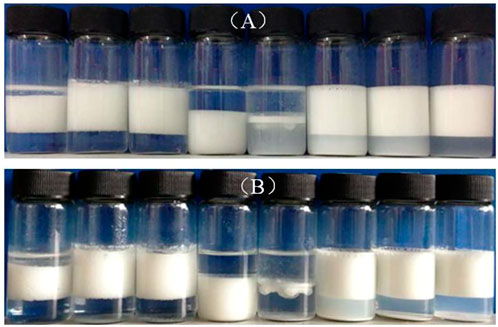
FIGURE 6. Appearance of toluene–water emulsions stabilized by 1.0 wt% CaCO3 nanoparticles together with SDS at different concentration (from left to right): 3 × 10−4, 6 × 10−4, 1 × 10−3 (O/W (1)), 3 × 10−3 (W/O), 6 × 10−3 (unstable), 1 × 10−2, 3 × 10−2, and 6 × 10−2 [O/W (2)] mol/L, taken (A) 24 h and (B) 1 week after preparation.
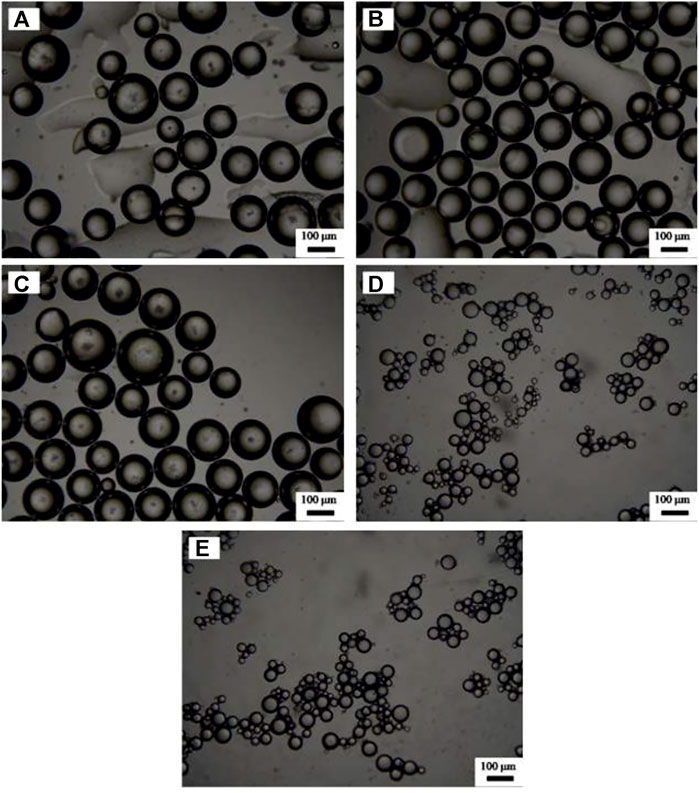
FIGURE 7. Optical micrographs of emulsions stabilized by (A–D) 1.0 wt% CaCO3 nanoparticles and SDS of various concentrations and by (E) SDS solely, taken 24 h after preparation. The concentration of SDS (from A to E) is 0.6, 1, 3, 30, and 10 mm.
3.3 Preparation and characterization of W/O/W multiple emulsions stabilized by CaCO3 nanoparticles in combination with SDS
A W/O emulsion including 1.0 wt.% CaCO3 nanoparticles and 3 mm SDS was chosen as the internal phase, which was then added to an aqueous dispersion with 1.0 wt% CaCO3 nanoparticles and 0.1 mm SDS, at a volume ratio of 2:1. Subsequently, the two phases were mixed by a magnetic stirrer to obtain W/O/W multiple emulsions. The appearance of the multiple emulsions showed no change after 24 h and 1 week, as shown in Figures 8A,B. The micrographs in Figure 8C proved that multiple emulsion has been formed, where a big outer droplet contains several small inner droplets. Also, the diameter of the external droplets of around 400 μm was much bigger than those stabilized by the surfactants alone.
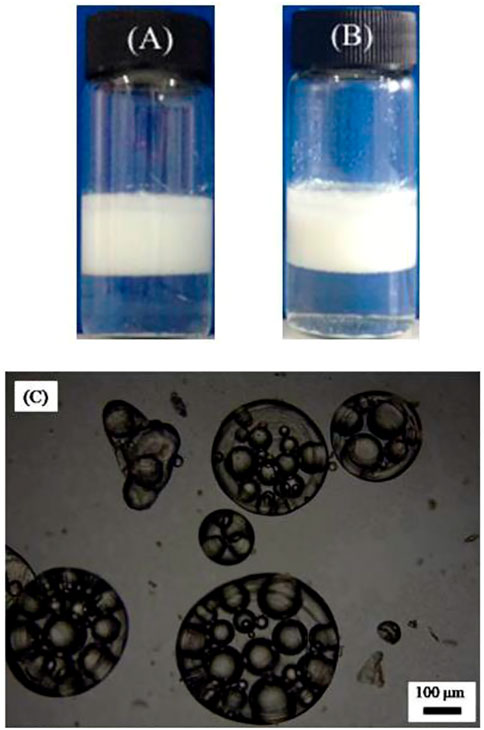
FIGURE 8. Appearance of the water-in-toluene-in-water multiple emulsion, taken (A) 1 day, (B) 1 week after preparation, and (C) optical micrograph recorded 24 h after preparation. The inner W/O emulsion is stabilized by 1.0 wt% CaCO3 nanoparticles and 3 × 10−3 mol/L SDS, and the outer O/W emulsion is stabilized by 1.0 wt% CaCO3 nanoparticles and 3 × 10−4 mol/L SDS. Volume ratio of inner W/O emulsion to the outer water phase = 2:1.
The influence of the volume ratio of the inner phase (W/O emulsion) to the outer phase (water phase) on the stability of the multiple emulsions was then studied. It is observed that when the volume ratio was 1:1 and 2:1, the multiple emulsions can be stable for 1 week, whereas with increasing the volume ratio to 3:1, the multiple emulsion became unstable with coalesced oil appeared, as shown in Figure 9.
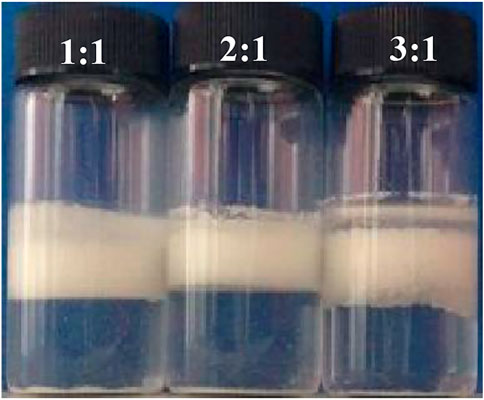
FIGURE 9. Appearance of the water-in-toluene-in-water multiple emulsions, taken 1 week after preparation. The inner W/O emulsion is stabilized by 1.0 wt% CaCO3 nanoparticles in combination with 3 × 10−3 mol/L SDS, and the outer aqueous phase is stabilized by 1.0 wt% CaCO3 nanoparticles together with 3 × 10−4 mol/L SDS. The volume ratio of inner water-in-toluene/outer aqueous phase is shown in the legend.
In addition, the influence of preparation methods (magnetic stirrer and homogenization) on multiple emulsions was also explored. It was noticed that although the appearance was almost not different (Figure 10), the oil droplets in the emulsion prepared by a magnetic stirrer were bigger, and each contains several water droplets, compared with that in emulsions prepared by homogenization, where oil droplets were relatively smaller, and each contains only 1–2 water droplets (Figure 11).
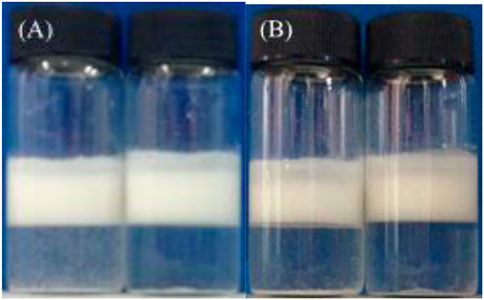
FIGURE 10. Appearance of the water-in-toluene-in-water multiple emulsions, taken (A) 1 day and (B)1 week after preparation by magnetic stirring (left) and homogenization (right). The inner W/O emulsion is stabilized by 1.0 wt% CaCO3 nanoparticles in combination with 3 × 10−3 mol/L SDS, and the outer water phase contains 1.0 wt% CaCO3 nanoparticles and 3 × 10−4 mol/L SDS. The volume ratio in inner water-in-toluene emulsion is 1:1, and the volume ratio of inner water-in-toluene emulsion to outer water phase is 1:1.
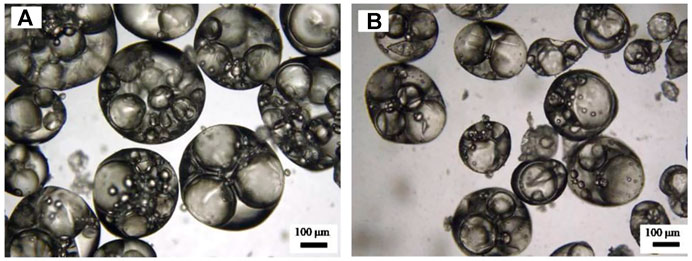
FIGURE 11. Micrograph of the water-in-toluene-in-water (W/O/W) multiple emulsions shown in Figure 10 prepared by (A) magnetic stirring and (B) homogenization, taken 24 h after preparation.
3.4 Surface activation mechanism of CaCO3 nanoparticles by in situ hydrophobization
It is known that ionic surfactant can adsorb on oppositely charged particle surfaces via electrostatic attraction, and by the formation of a monolayer, the hydrophilicity of particles surface is reduced. The adsorption of SDS on CaCO3 particles can be proved by the change of zeta potentials of the 1.0 wt% CaCO3 particles dispersed in SDS solutions of varied concentrations, as shown in Figure 12, and the adsorption isotherms are demonstrated in Figure 13. As the concentration of SDS increases, the charges on the particles are neutralized with the zeta potential changing from positive to negative, and the adsorption amounts increase, indicating adsorption of SDS molecules through electrostatic interaction. The adsorption of SDS on particle surfaces with hydrocarbon chain toward water modifies the wettability of CaCO3 nanoparticles. At lower SDS concentration (below 1 mm), the particles are weak hydrophobic, tending to stabilize O/W emulsion. However, at middle SDS concentration (3 mm), the particle surface turns more hydrophobic suitable to stabilize W/O Pickering emulsions.
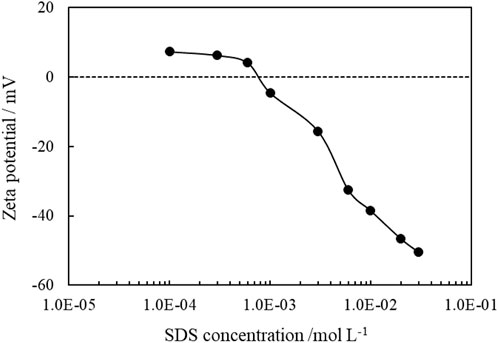
FIGURE 12. Zeta potential of 1.0 wt% CaCO3 nanoparticles dispersed in SDS solutions of different concentration at 25°C.
The configuration of the adsorbed SDS at the particle surface can be determined by the contact angle measurement, as shown in Figure 14. It is noticed that the contact angle of pure water on the CaCO3 surface is as low as 10°, whereas the contact angle of the SDS solution increases with increasing SDS concentration, reaching a maximum around 23° at an SDS concentration of 3 mm, and it is believed that the maximum contact angle of the SDS solution on CaCO3 surface in oil (toluene) should be much larger, or >90°, so that particles are hydrophobic enough to stabilize W/O emulsions. With further increasing SDS concentration, the contact angle decreases due to bilayer formation, which makes particle surface hydrophilic again since the second layer has an opposite configuration. It is then suggested that in the region of O/W (1) and W/O, the SDS molecules form a monolayer on particle surfaces with their head groups toward particle and hydrocarbon chains toward water, whereas in the region of O/W (2), SDS molecules form a bilayer on the CaCO3 particles, making the particles hydrophilic again with their head groups toward water.
It is noticed that the size of the oil droplets and the inner water droplets is relatively larger, as shown in Figure 11. This is because the CaCO3 nanoparticles aggregate to relatively larger aggregations in either water or SDS solution, as shown in Figure 15, although they have a small primary size (Figure 1). It is suggested that using nanoparticles with smaller sizes may be beneficial to obtain multiple Pickering emulsions with smaller droplet sizes.
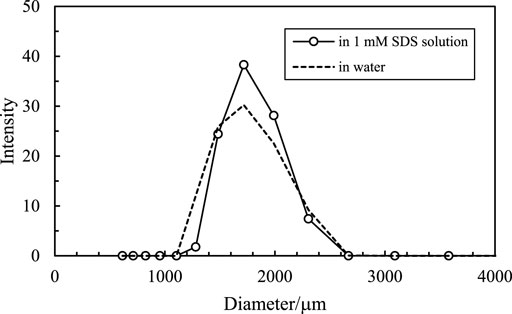
FIGURE 15. Size distribution of 1 wt% CaCO3 nanoparticles dispersed in water and 1 mm SDS solutions at 25°C.
4 Conclusion
A simple method is demonstrated to be effective for preparing multiple W/O/W emulsions by using the same particle (CaCO3 nanoparticles) as emulsifiers of both the internal and external interphases. The raw CaCO3 nanoparticles, which are less surface-active, can be made more surface-active by in situ hydrophobization via adsorption of an oppositely charged surfactant (SDS) and can then self-assemble at the fluid interface to stabilize simple Pickering emulsions and multiple Pickering emulsions. In addition, the wettability of the particles can be adjusted by controlling the SDS concentration, and phase inversion can then occur. At 3 mm SDS in an aqueous solution, the particles tend to stabilize W/O emulsion, whereas, at lower SDS concentrations (below 1 mm), the particles tend to stabilize O/W emulsion. Multiple emulsions can, therefore, be prepared using the same particles in combination with different concentrations of SDS. The multiple emulsions stabilized by CaCO3 nanoparticles can stay stable for at least a month avoiding coalescence, which makes them more stable compared with those stabilized by surfactants of different hydrophilicity. This protocol avoids complicated synthesis of the particles with different hydrophilicity and has potential applications in the fields of pharmaceuticals, foods, and cosmetics, where stable Pickering of multiple emulsions is expected.
Data availability statement
The original contributions presented in the study are included in the article/Supplementary Material; further inquiries can be directed to the corresponding authors.
Author contributions
YZ wrote the manuscript and drafted part of the experiment. TC drafted part of the experiment. ZC revised the article. All authors contributed to the article and approved the submitted version.
Funding
Financial support from the National Natural Science Foundation of China (NSFC 21802075) is gratefully acknowledged and open access publication fees.
Conflict of interest
The authors declare that the research was conducted in the absence of any commercial or financial relationships that could be construed as a potential conflict of interest.
Publisher’s note
All claims expressed in this article are solely those of the authors and do not necessarily represent those of their affiliated organizations, or those of the publisher, the editors, and the reviewers. Any product that may be evaluated in this article, or claim that may be made by its manufacturer, is not guaranteed or endorsed by the publisher.
References
Akbaba, H., Karagöz, U., Selamet, Y., and Kantarci, A. G. (2017). Synthesis and characterization of cationic lipid coated magnetic nanoparticles using multiple emulsions as microreactors. J. Magn. Magn. Mat. 426, 518–524. doi:10.1016/j.jmmm.2016.11.126
Aveyard, R., Binks, B. P., and Clint, J. H. (2003). Emulsions stabilised solely by colloidal particles. Adv. Colloid Interface Sci. 100-102, 503–546. doi:10.1016/s0001-8686(02)00069-6
Binks, B. P., and Horozov, T. S. (2006). in Colloidal particles at liquid interfaces. Editors B. P. Binks, and T. S. Horozov (Cambridge, U.K.: Cambridge University Press), 1–74.
Binks, B. P. (2002). Particles as surfactants-similarities and differences. Curr. Opin. Colloid Interface Sci. 7, 21–41. doi:10.1016/s1359-0294(02)00008-0
Bonnet, M., Cansell, M., Placin, F., Monteil, J., Anton, M., Lealcalderon, F., et al. (2010). Influence of the oil globule fraction on the release rate profiles from multiple W/O/W emulsions. Colloids Surfaces B Biointerfaces 78, 44–52. doi:10.1016/j.colsurfb.2010.02.010
Cizauskaite, U., Marksiene, R., Viliene, V., Gruzauskas, R., and Bernatoniene, J. (2017). New strategy of multiple emulsion formation based on the interactions between polymeric emulsifier and natural ingredients. Colloids Surfaces A Physicochem. Eng. Aspects 515, 22–33. doi:10.1016/j.colsurfa.2016.12.002
Clegg, P. S., Tavacoli, J. W., and Wilde, P. J. (2015). One-step production of multiple emulsions: microfluidic, polymer-stabilized and particlestabilized approaches. Soft Matter 12, 998–1008. doi:10.1039/c5sm01663k
Cole, M. L., and Whateley, T. L. (1997). Release rate profiles of the ophylline and insulin from stable multiple w/o/w emulsions. J. Control. Release 49, 51–58. doi:10.1016/s0168-3659(97)00056-4
Cournarie, F., Rosilio, V., Chéron, M., Vauthier, C., Lacour, B., Grossiord, J. L., et al. (2004). Improved formulation of W/O/W multiple emulsion for insulin encapsulation. Influence of the chemical structure of insulin. Colloid Polym. Sci. 282, 562–568. doi:10.1007/s00396-003-0960-8
Cui, Z.-G., Cui, C.-F., Zhu, Y., and Binks, B. P. (2012). Multiple phase inversion of emulsion stabilized by in situ surface activation of CaCO3 nanoparticle via adsorption of fatty acids. Langmuir 28, 314–320. doi:10.1021/la204021v
Cui, Z.-G., Cui, Y.-Z., Cui, C.-F., Chen, Z., and Binks, B. P. (2010). Aqueous foams stabilized by in situ surface activation of CaCO3 nanoparticles via adsorption of anionic surfactant. Langmuir 26, 12567–12574. doi:10.1021/la1016559
Cui, Z.-G., Shi, K.-Z., Cui, Y.- Z., and Binks, B. P. (2008). Double phase inversion of emulsions stabilized by a mixture of CaCO3 nanoparticles and sodium dodecyl sulphate. Colloids Surfaces A Physicochem. Eng. Aspects 329, 67–74. doi:10.1016/j.colsurfa.2008.06.049
Cui, Z.-G., Yang, L.-L., Cui, Y.-Z., and Binks, B. P. (2010). Effects of surfactant structure on the phase inversion of emulsions stabilized by mixtures of silica nanoparticles and cationic surfactant. Langmuir 26, 4717–4724. doi:10.1021/la903589e
Engel, R. H., Riggi, S. J., and Fahrenbach, M. J. (1968). Insulin: Intestinal absorption as water-in-oil-in-water emulsions. Nature 219, 856–857. doi:10.1038/219856a0
Fernández-Martín, F., Freire, M., Bou, R., Cofrades, S., and Jiménez-Colmenero, F. (2017). Olive oil based edible W/O/W emulsions stability as affected by addition of some acylglycerides. J. Food Eng. 196, 18–26. doi:10.1016/j.jfoodeng.2016.10.011
Florence, A. T., and Whitehill, D. (1982). The formulation and stability of multiple emulsions. Int. J. Pharm. X. 11 (4), 277–308. doi:10.1016/0378-5173(82)90080-1
Ghasemi, H., Aghabarari, B., Alizadeh, M., AliKhanlarkhani, A., and Abu-Zahra, N. (2020). High efficiency decolorization of wastewater by fenton catalyst: magnetic iron-copper hybrid oxides. J. Water Process Eng. 37, 101540. doi:10.1016/j.jwpe.2020.101540
Ghasemi, H., Darjani, S., Mazloomi, H., and Mozaffari, S. (2020). Preparation of stable multiple emulsions using food-grade emulsifiers: evaluating the effects of emulsifier concentration, W/O phase ratio, and emulsification process. SN Appl. Sci. 2, 2002. Article number: 2002. doi:10.1007/s42452-020-03879-5
Hong, L., Sun, G., Cai, J., and Ngai, T. (2012). One-step formation of w/o/w multiple emulsions stabilized by single amphiphilic block copolymers. Langmuir 28 (5), 2332–2336. doi:10.1021/la205108w
Hoppel, M., Juric, S., Reznicek, G., Wirth, M., and Valenta, C. (2015). Multiple W/O/W emulsions as dermal peptide delivery systems. J. Drug Deliv. Sci. Technol. 25, 16–22. doi:10.1016/j.jddst.2014.11.001
Jiménezcolmenero, F. (2013). Potential applications of multiple emulsions in the development of healthy and functional foods. Food Res. Int. 52 (1), 64–74. doi:10.1016/j.foodres.2013.02.040
Keshmiri, K., Mozaffari, S., Tchoukov, P., and Nazemifard, N. (2016). “Using microfluidic device to study rheological properties of heavy oil,” in AIChE conference, San Francisco, CA, November 14, 2016.
Kruglyakov, P. M., and Nushtayeva, A. V. (2004). Phase inversion in emulsions stabilised by solid particles. J. Colloid Interface Sci. 108-109, 151–158. doi:10.1016/j.cis.2003.10.012
Li, J., Zhang, J., Han, B., Zhao, Y., and Yang, G. (2012). Formation of multiple water-in-ionic liquid-in-water emulsions. J. Colloid Interface Sci. 368 (1), 395–399. doi:10.1016/j.jcis.2011.10.083
Liu, H., Zhang, Y., Huang, J., Liu, T., Xue, N., Wang, K., et al. (2017). Selective separation and recovery of vanadium from a multiple impurity acid leaching solution of stone coal by emulsion liquid membrane using di-(2-ethylhexyl)phosphoric acid. Chem. Eng. Res. Des. 122, 289–297. doi:10.1016/j.cherd.2017.04.026
Lutz, R., Aserin, A., Wicker, L., and Garti, N. (2009). Double emulsions stabilized by a charged complex of modified pectin and whey protein isolate. Colloids Surfaces B Biointerfaces 72 (1), 121–127. doi:10.1016/j.colsurfb.2009.03.024
Maciel, N. R., Oliveira, E. C. V., Okuma, C. H., Topan, J. F., Amaral, L. Q., Rochafilho, P., et al. (2015). A New System of multiple emulsions with lamellar gel phases from vegetable oil. J. Dispers. Sci. Technol. 37 (5), 646–655. doi:10.1080/01932691.2015.1054506
Morais, J. M., Rochafilho, P. A., and Burgess, D. J. (2009). Influence of phase inversion on the formation and stability of one-step multiple emulsions. Langmuir 25 (14), 7954–7961. doi:10.1021/la9007125
Moussaoui, N., Hammadi, L., Boudjenane, N. E., and Denine, R. R. (2016). Development of multiple W/O/W emulsions used in pharmaceutical field: effect of additives and insulin on physicochemical and rheological stability of emulsions. Colloid Polym. Sci. 295, 125–133. doi:10.1007/s00396-016-3989-1
Mozafari, S. (2015). “Rheology of Bitumen at the onset of asphaltene aggregation and its effects on the stability of water-in-oil emulsion,” Masters Thesis. (Canada: University of Alberta).
Panagopoulou, E., Evageliou, V., Kopsahelis, N., Ladakis, D., Koutinas, A., Mandala, I., et al. (2017). Stability of double emulsions with PGPR, bacterial cellulose and whey protein isolate. Colloids Surfaces A Physicochem. Eng. Aspects 522, 445–452. doi:10.1016/j.colsurfa.2017.03.020
Riess, G., and Labbe, C. (2004). Block copolymers in emulsion and dispersionpolymerization. Macromol. Rapid Commun. 25 (2), 401–435. doi:10.1002/marc.200300048
Saffarionpour, S., and Diosady, L. L. (2021). Multiple emulsions for enhanced delivery of vitamins and iron micronutrients and their application for food fortification. Food Bioproc. Tech. 14, 587–625. doi:10.1007/s11947-021-02586-2
Seifritz, W. (1925). Studies in emulsions: Double reversal of oil emulsions occasioned by the same electrolyte. Journey Phys. Chem. 29 (6), 738–749.
Taisne, L., Walstra, P., and Cabane, B. (1996). Transfer of oil between emulsion droplets. J. Colloid Interface Sci. 184 (2), 378–390. doi:10.1006/jcis.1996.0632
Wang, Q., Hu, C., Zoghbi, A., Huang, J., and Xia, Q. (2017). Oil-in-oil-in water pre-double emulsions stabilized by nonionic surfactants and silica particles: a new approach for topical application of rutin. Colloids Surfaces A Physicochem. Eng. Aspects 522, 399–407. doi:10.1016/j.colsurfa.2017.02.067
Zamani, S., Malchione, N., Selig, M. J., and Abbaspourrad, A. (2018). Formation of shelf stable pickering high internal phase emulsions (HIPE) through the inclusion of whey protein microgels. Food Funct. 9 (2), 982–990. doi:10.1039/c7fo01800b
Keywords: multiple emulsions, CaCO3 nanoparticles, hydrophobization in situ, anionic surfactant, stabilization
Citation: Zhu Y, Chen T and Cui Z (2022) Multiple Pickering emulsions stabilized by the same particles with different extent of hydrophobization in situ. Front. Chem. 10:950932. doi: 10.3389/fchem.2022.950932
Received: 23 May 2022; Accepted: 07 July 2022;
Published: 19 August 2022.
Edited by:
Tangxin Xiao, CHangzhou University, ChinaReviewed by:
Abbul Bashar Khan, Jamia Millia Islamia, IndiaRakesh K. Sharma, Maharaja Sayajirao University of Baroda, India
Copyright © 2022 Zhu, Chen and Cui. This is an open-access article distributed under the terms of the Creative Commons Attribution License (CC BY). The use, distribution or reproduction in other forums is permitted, provided the original author(s) and the copyright owner(s) are credited and that the original publication in this journal is cited, in accordance with accepted academic practice. No use, distribution or reproduction is permitted which does not comply with these terms.
*Correspondence: Tingting Chen, ttchen1980@126.com; Zhenggang Cui, cuizhenggang@hotmail.com