- 1Department of Urology, Tianjin First Central Hospital, Tianjin, China
- 2Department of Urology, Qingdao Hospital of Traditional Chinese Medicine (Qingdao Hiser Hospital), ShanDong, China
- 3Department of Urology, Weihai Central Hospital, ShanDong, China
- 4Department of Urology, First Central Clinical College, Tianjin Medical University, Tianjin, China
- 5School of Medicine, Nankai University, Tianjin, China
Cancer is still a global public health problem. Although remarkable success has been achieved in cancer diagnosis and treatment, the high recurrence and mortality rates remain severely threatening to human lives and health. In recent years, peptide nanomedicines with precise selectivity and high biocompatibility have attracted intense attention in biomedical applications. In particular, there has been a significant increase in the exploration of peptides and their derivatives for malignant tumor therapy and diagnosis. Herein, we review the applications of peptides and their derivatives in the diagnosis and treatment of bladder cancer, providing new insights for the design and development of novel peptide nanomedicines for the treatment of bladder cancer in the future.
Introduction
One recent report from the World Health Organization’s International Agency for Research on Cancer (IARC) released the latest global cancer burden data, showing that 4.57 million cancer cases and 3 million resultant deaths increased in 2020 in China. Among them, bladder cancer is one of the common urinary malignancies and ranks among the top ten cancers in terms of morbidity and mortality. Bladder cancer is one of the most expensive cancers to cure because of its high recurrence rate (Barani et al., 2021). Although new techniques involving radiotherapy, immunotherapy, chemotherapy, etc., are blossoming in the treatment of bladder cancer (Booth et al., 2018; Tree et al., 2018; Wołącewicz et al., 2020), their toxic side effects and high costs limit their broad applications in clinical applications. Early diagnoses, including the examination of circulating tumor cells, CT scan, magnetic resonance imaging, positron emission tomography, bone scan, chest X-ray, etc., are crucial for the diagnosis and treatment of bladder cancer (Todenhöfer et al., 2018; van der Pol et al., 2018; Wu et al., 2018), but their disadvantages, such as nonspecificity, heterogeneity, and excessive detection, still limit their potential clinical applications (Faba et al., 2019). Cystoscopic biopsy can improve the diagnostic accuracy, but it is difficult to identify superficial mucosal lesions such as carcinoma in situ, and it is invasive. Abscission cytology is a standard non-invasive test for the diagnosis and monitoring of bladder cancer. It has the disadvantage of being insensitive to low-grade tumors and depends on accurate diagnosis by the pathologist (DeGeorge et al., 2017).
The primary purpose of drug delivery is to send enough drug payloads to the lesion sites while minimizing their exposure to healthy tissues. To improve the specificity and pharmacokinetics of anticancer drugs and avoid the side effects of traditional therapies, two main strategies involving drug carriers and covalent modifications are widely used. Drug carriers such as nanoparticles and hydrogels can protect drugs from the external environment before on-demand release when they reach lesion sites. Meanwhile, the physical and chemical properties of the drug carriers significantly determine their biological distributions (Fan et al., 2021; Huang et al., 2021). Covalent modifications enable temporarily masking or limiting the bioactivity of the drugs and confer them with the desired pharmacokinetics (Lin et al., 2019; Cooper et al., 2021; Yang et al., 2021). It is noteworthy that both the abovementioned strategies can alleviate the burden of drug metabolism and improve the therapeutic effects of the original drugs. Among numerous drug molecules, peptides are highly competitive candidates for the treatment of bladder cancer because of their small sizes, high specificity, low systemic toxicity, etc. In addition, the diagnosis of bladder cancer mainly depends on pathology and imaging examinations, while the detection accuracy is still low. Using specific biomarkers on bladder tumor cells, peptide nanotechnology can significantly improve the sensitivity and specificity for the diagnosis of bladder cancer. (Pan et al., 2014; Tummers et al., 2017).
Peptide-Instructed Tumor Diagnosis
Magnetic resonance imaging (MRI) is a noninvasive technique for tumor diagnosis in current clinical medicine. Although it has been shown that MRI has the ability to display three-dimensional anatomical details without injury and provide high spatial resolution without invasiveness, MRI is still less sensitive than fluorescence imaging for monitoring tiny tissue damage, cellular activity, molecular activity, etc (Chandra et al., 2010; Schroeder, 2008). Therefore, the development of new contrast agents is expected to enable the improvement of the detection accuracy of MRI. Paramagnetic Gd3+ complexes and superparamagnetic iron oxide (SPIO) nanoparticles are two widely used contrast agents in MRI detection. Compared with the paramagnetic Gd3+ complex, SPIO is a better alternative to MRI contrast agents, of which the signal contrast is several orders of magnitude higher than that of the traditional Glacki contrast agent (Jun et al., 2008). In a study of human bladder tumors, the researchers reported that 1.5T magnetic resonance imaging using SPIO as the contrast agent realized in situ detection of malignant tumors with a small size to ∼4 mm, while it was unable to effectively distinguish the depth of tumor invasion into the bladder walls (Beyersdorff et al., 2000). The main reason is that the cellular internalization levels of SPIO are limited, and less than 1% of SPIO is internalized by nonspecific endocytosis pathways (Moore et al., 2001). Due to the great promise of SPIO in MRI applications, researchers have endeavored to develop a variety of SPIO conjugates to enhance its cellular uptake ratio, in which cell-penetrating peptides (CPPs), such as R11, are considered to be one of the best transporters to improve the active internalization of nanoparticles into target cells (Hsieh et al., 2011; Ding et al., 2017). Ding et al. recently also developed an SPIO nanoparticle whose surfaces are unctionalized with bladder cancer-specific fluorescein isothiocyanate (FITC) labeled cell-penetrating peptide (CPP) -polyarginine peptide (R11) for active targeting and imaging for bladder cancer, respectively. Their study showed that SPIO-R11 nanoparticles can be internalized by T24 cells in a dose-dependent manner, and that SPIO-R11 internalized dose is higher than that of SPIO itself, since R11 is a cell-permeable peptide that enables efficient drug delivery. Transmission electron microscopy (TEM) results indicated that SPIO-R11 is mainly located in cellular vesicles and lysosomes, but no signals in the nucleus were found. Due to the cellular specificity of SPIO-R11, the uptake of nanoparticles into bladder cancer cells was significantly higher than that of immortalized bladder epithelial cells. In addition, SPIO-R11 had a lower T2 relaxation time in MRI than SPIO. These results suggested that SPIO-R11 has great potential as a targeted contrast agent for the diagnosis and treatment of bladder cancer (Ding et al., 2017).
Tumor cells are mutated from normal cells, of which the signal transduction pathways are significantly different from those of normal cells. Therefore, many signaling regulators or regulatory proteins are overexpressed in tumor cells and can be used as specific targets for tumor diagnosis (Oh and Bang, 2020; Wilson et al., 2021). Recently, targeted peptides have attracted intense attention because they can specifically bind with receptors on tumor cells. By conjugating with radioactive or fluorescent probes, scientists have prepared a variety of peptide probes to specifically orient and image tumors (Ciobanasu, 2021; Kwak et al., 2021; Lu et al., 2021; Sonju et al., 2021; Wang et al., 2021). For instance, Wei et al. recently constructed a loaded nanoscale oxygen generator (PLZ4@SED) by conjugating superparamagnetic iron oxide nanoparticles (SPIOns) with peptide motifs specific to bladder cancer cells. PLZ4@SED showed good tumor targeting and permeability to patient-derived bladder cancer cells. Meanwhile, they illustrated that the presence of PLZ4@SED can improve the contrast of MRI and promote chemotherapeutic efficacy by producing oxygen through the Fenton reaction to relieve hypoxia. It was also reported that PLZ4@SED presented great potential in the diagnosis and treatment of bladder cancer (Lin et al., 2021). Sweeney et al. (2017) demonstrated one successful application of mesoporous silica nanoparticles (MSN), which are functionalized using a bladder cancer-specific peptide CyC6, as the magnetic resonance contrast agent. Due to the effective binding of the modified MSN to tumor cells, tumor boundaries were much clearer in the T1-and T2-weighted MRI and fluorescence cystoscopic inspections compared to the traditional technique.
Cystoscopy is one gold standard for the diagnosis of bladder cancer. However, cystoscopy is an invasive and costly technique, and it is difficult to detect flat malignancies using this technique. Meanwhile, urine cytology is low-sensitivity for detecting low-grade lesions, of which the detection accuracy is highly dependent on the experience of the cytopathologists (Grossman et al., 2006; Alfred Witjes et al., 2017; Babjuk et al., 2017). Recently, several potential biomarkers have been identified that could potentially provide noninvasive and objective approaches for the detection of bladder cancers (Kluth et al., 2015). Lee et al. presented one peptide conjugate consisting of fluorescein and the peptide sequence of CSNRDARRC. They reported that the peptide conjugate can specifically bind to bladder cancer tissue using frozen sections. Meanwhile, the peptide conjugate could selectively bind to bladder tumor epithelial cells when it was injected into the bladder cavity using a tumor-bearing rat model. Furthermore, Lee et al. found that the peptide conjugate had the ability to indicate bladder tumor cells in urine, presenting great potential to be exploited as a real-time diagnostic probe to detect bladder cancer (Lee et al., 2007). Prothrombin activators (TSPs) can prevent angiogenesis in a variety of pathological conditions. Some structural domains and peptide derivatives of TSP-2 enable the promotion of angiogenesis in BC tissues. 4N1K (KRFYVVMWKK), derived from the C-terminal cell-binding domain of TSP-2, plays an important role in the pathology and prognosis of bladder cancer. Using the hematoxylin-eosin (H&E) staining technique to examine tumor tissues from bladder cancer patients, Nakamura et al. verified that 4N1K was significantly correlated with the tumor apoptosis index and microvascular density but negatively related to T stage, metastasis and tumor grade; promising 4N1K may be a useful biomarker and a new therapeutic target for UC-UUT patients (Nakamura et al., 2019).
Peptide-instructed Local Chemotherapy
Transurethral resection combined with chemotherapeutic infusion is the standard treatment protocol for nonmuscular invasive bladder cancer. However, the low bioavailability (GuhaSarkar and Banerjee, 2010) and short retention period of the current chemotherapeutic drugs (Tyagi et al., 2006; Wirth et al., 2009) restricted their exposure time at the lesion sites. Along with the advancement of nanotechnology, nanocarrier drug delivery systems show advantages in solving these problems. Guo et al. (2017) designed and synthesized a kind of positively charged intelligent peptide nanocarrier cross-linked with disulfide bonds [i.e., PLL-P (LP-co-LC). They prepared one nanogel system (NG/HCPT) using this nanocarrier by artificially loading 10-hydroxycamptothecin (10-HCPT). Compared with free 10-HCPT, NG/HCPT not only has a higher drug loading rate, longer retention time, and stronger tissue penetration ability but can also accurately and rapidly release 10-HCPT into bladder cancer cells, significantly enhancing the corresponding antitumor effects and reducing the side effects (Figure 1). In 2020, Guo et al. further synthesized a new R9-polyethylene glycol poly (L-phenylalanine-L-cysteine) nanogel (R9-PEG-P (LP-co-LC)] based on NG/HCP, which can improve the adhesion and permeability of chemotherapeutic drugs. They prepared the R9NG/HCPT nanogel using 10-HCPT as a model drug. The morphology of R9NG/HCPT is similar to that of an octopus with a spear. Highly positively charged R9 with strong membrane penetrability can help R9NG/HCPT pass across the bladder walls and enhance its cellular adhesion interactions through nonspecific and electrostatic interactions, thus enabling prolonged exposure to chemotherapeutic drugs at the lesion sites. This system significantly improved the tumor suppression efficiencies of 10-HCPT in both in situ mice and rat tumor models, suggesting great potential in the local chemotherapy of bladder cancer (Guo et al., 2020).
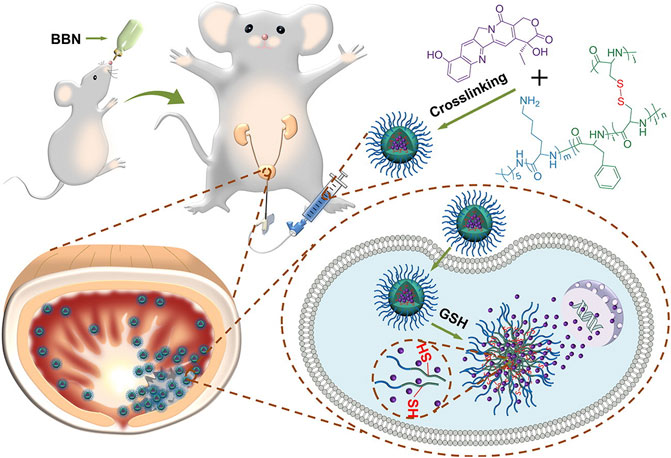
FIGURE 1. After intravesical instillation of NG/HCPT, the selective accumulation of nanodrugs in tumor tissue finally releases HCPT triggered by GSH(Guo et al., 2017).
Polymeric micelles constructed using amphiphilic block copolymers have been widely explored in recent decades due to their high drug loading efficiency, long cycle time, well-controlled release ability, and good targeting properties (Xiao et al., 2012). Recently, Zhou et al. developed an amphiphilic diblock copolymer poly (ε-caprolactone)-b-polyoxyethylene (PCL-b-PEO) containing integrin targeting motif c (RGDfK) and imaging dye FITC. The copolymer assembled into micelles and strongly interacted with bladder cancer T24 cells. After encapsulation with doxorubicin (DOX), the micelle could efficiently prevent the proliferation of T24 cells and was expected to be used as a nanoscale drug delivery system for bladder perfusion chemotherapy (Zhou et al., 2013).
The combination usages of two or more drugs showed great advantages in cancer treatments involving improving therapeutic efficacy, lowering side effects, and preventing drug resistance, which are promising strategies for the treatment of refractory cancers. The positively charged adhesive chitosan-polymethacrylic acid (CM) nanocapsules loaded with DOX and cisplatin modified with peptide (Pt-Aly) presented high drug loading efficiency and sustained drug release properties. Meanwhile, CM nanocapsules can be firmly attached to the surface of the bladder cavity, prolong the retention time of the payload in the bladder, and have the effect of synergistically killing UMUC3 bladder cancer cells. In addition, CM nanocapsules have no obvious damage to the urothelium, which is expected to cooperate with intravesical chemotherapy in the treatment of non-muscle invasive bladder cancer (Lu et al., 2016). Overall, the intelligent peptide nanogel systems have much more powerful retention efficiency and permeability, providing a promising drug delivery platform for local chemotherapy of bladder cancer.
Peptide-assisted Systemic Chemotherapy
Systemic chemotherapy is one of the dominant techniques used to treat musculoskeletal invasive bladder cancer (Calabrò and Sternberg, 2009; Yin et al., 2016). However, nonspecific distributions of traditional chemotherapeutic drugs in human bodies have caused severe toxicity to normal tissues, including liver and kidney organs, bone marrow, gastrointestinal tract tissues, etc., and significantly limited their clinical applications. Therefore, researchers are endeavoring to develop new drug delivery systems that can transport the chemical drugs into the desired sites to improve their therapeutic effects (Cheng et al., 2019; Sonju et al., 2021). Peptide-drug conjugates are promising prodrugs for the treatment of cancer that combine one or more traditional chemical drugs with short peptides through biodegradable linkers. This prodrug strategy can uniquely and specifically employ the bioactivity and self-assembling properties of short peptides to enhance the therapeutic efficacy of traditional drugs (Cooper et al., 2021). Zeng et al. (2021b) recently developed one short-peptide prodrug, HCPT-FF-GFLG-EEYASYPDSVPMMS, consisting of 1) a self-assembling motif (i.e., -FF-); 2) an EphA2 targeting sequence on T24 cancer cells (i.e., YSAYPDSVPMMS); and 3) one short peptide linker responsive to the CtsB enzyme (i.e., GFLG). They found that this prodrug could be efficiently encapsulated by T24 cells and cleaved intracellularly by CtsB, resulting in nanofibrils in T24 cells (Figure 2). The formation of nanofibrils loaded with HCPT prolonged its circulation period in vivo. Moreover, this prodrug system could precisely deliver HCPT into T24 cancer cells, reduce its accumulation in normal tissue and lower the side effects. Pan et al. prepared one kind of nanomedicine, DC-PNM-PTX, in which one bladder targeting peptide sequence, PLZ4, one polymeric micelle, and the chemical drug paclitaxel (PTX) were involved. They reported that DC-PNM-PTX could specifically target bladder cancer cells, prevent bladder tumor growth in a xenograft tumor model, and efficiently prolong mouse survival compared to unmodified PTX. Nanomaterials modified with multiple ligands targeting cell membrane receptors play positive roles in tumor therapy, which is beneficial for reducing the toxicity and side effects of traditional chemotherapy and improving antitumor outcomes (Pan et al., 2016).
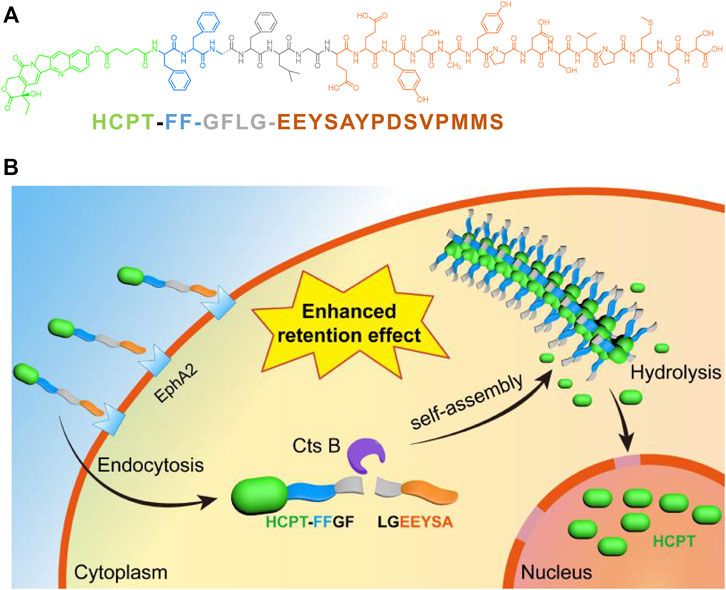
FIGURE 2. (A) The molecular structure of HCPT-FF-GFLG-EEYSAYPDSVPMMS; (B) The illustration of the bladder tumor cell targeting, intracellular fibrillation and drug release of HCPT-FF-GFLG-EEYSAYPDSVPMMS(Zeng et al., 2021a).
Peptide-instructed Gene Therapy
Gene therapy is a revolutionary technique that directly uses therapeutic genes to treat various diseases. As one alternative to traditional treatments (Dunbar et al., 2018; High and Roncarolo, 2019), the first clinical trial of gene therapy was approved in 1989, and nearly 2,600 trials have been completed or are under their ways worldwide until now (Ginn et al., 2018). However, it is still challenging to direct the genes into targeted cells without damaging other cells. It has been shown that virus-like particles (VLPs) from human JC polyomavirus (JCPyV) can package and deliver exogenous DNA into sensitive cells for gene expression (Chang et al., 1997). To improve the specificity of gene therapy, Lai et al. (2021) conjugated SPB peptides targeting bladder cancer cells onto JC polyomavirus (JCPyV) virus-like particles (VLPs) and succeeded in the delivery of the suicide gene thymidine kinase. Both in vitro and in vivo experiments illustrated that the suicide gene was only expressed in human bladder cancer cells but not in lung cancer and neuroblastoma cells that were sensitive to JCPyV VLP infection, implying the great specificity of VLP-SPBs. Meanwhile, the gene transduction efficiency of VLP-SPBs is approximately 100-fold that of the VLP itself. The binding of JCPyV VLPs with specific peptides can improve their original affinities and change the expression directions of the packed genes. Moreover, VLP-SPBs presented the ability to selectively prevent the growth of bladder tumors but had no significant inhibitory effects against lateral lung tumors. In general, gene therapy is one flourishing technique to treat various diseases, and malignancies are their main enemy. The applications of the targeted peptide delivery systems enable artificial control and regulation of gene expression at the cellular level, thus succeeding in disease treatments but not affecting normal tissues and organs.
Epidemiological data have shown that more than 50% of human malignancies, including bladder cancer, are related to mutations in the p53 gene (Hainaut et al., 1997). Mutant p53 protein enables the acceleration of tumor formation and metastasis and is associated with resistance to radiotherapy and chemotherapy, as well as poor prognosis (Al-Sukhun and Hussain, 2003). The functional restoration of p53 protein can promote the expression of downstream genes to block cell cycles or induce cell apoptosis, resulting in the suppression of tumor progression. It has been shown that one C-terminal peptide sequence (p53c) can restore the binding ability to specific DNA sequences and the transactivation function of the mutant p53 gene, leading to p53-dependent apoptosis of tumor cells (Selivanova et al., 1997). However, due to the lipophilicity of biological membranes and their roles as biological barriers to defeat exterior enemies, many synthetic compounds cannot cross cell membranes. R11 can be specifically captured by bladder and prostate tissues and is promising for use as a drug or probe carrier for the treatment and detection of upper urinary tract tumors (Hsieh et al., 2011; Ding et al., 2017). Zhang et al. showed that the synthetic peptide R11-p53c can be effectively and preferentially delivered into bladder cancer cells, resulting in the reactivation of the p53 gene and inhibition of tumor growth. More interestingly, R11-p53c also presented excellent antitumor effects in primary and metastatic tumor models, which could prolong the survival period while having no significant systemic toxicities. In addition, their study also illustrated that R11-p53c could prevent the growth of both mutant and recombinant p53c tumor cells but had no significant inhibitory effects on normal cells. It was also noted that transcriptional levels of several p53 target genes were upregulated after treatment with R11-p53c. Overall, R11-p53c has the potential to treat both primary and metastatic bladder cancer and should be a promising therapeutic agent for the treatment of upper urinary tract tumors. (Hsieh et al., 2011).
Peptide-mediated Photothermal Therapy
Photothermal therapy (PTT) is a highly promising strategy to defeat malignancies that mainly utilizes photothermal materials to convert light energy into heat in situ, finally raising the local temperature to result in cell apoptosis and tumor killing (Gao et al., 2019; Chen et al., 2020; Jiang et al., 2020). By taking advantage of photothermal conversion, PTT has been widely used in a variety of tumor treatments, and some of them are under clinical trials (Timko et al., 2010; Chen et al., 2014). One crucial issue for PTT applications is to develop carrier materials with good selectivity to tumor cells. Tao et al. (Tao et al., 2019) loaded folate-modified vincristine into polydopamine-coated Fe3O4 (Fe3O4@PDA-VCR-FA SPs) and applied them for the treatment of bladder cancer. PDA shells can not only improve colloid stability and biocompatibility but also enhance photothermal effects and prolong the blood circulation period. The half-life period in blood and the tumor retention rate of Fe3O4@PDA-VCR-FA SPs are 2.83 h and 5.96% ID g−1, respectively, which are significantly improved compared with those before folic acid modification. The superparamagnetism of Fe3O4 and the loading of vincristine enable arming Fe3O4@PDA-VCR-FA SPs with nuclear magnetic resonance imaging (NMRI) and chemotherapy abilities. With the further help of near-infrared laser-triggered photothermal therapy, Fe3O4@PDA-VCR-FA SPs can completely remove bladder cancer and prevent its recurrence. Moreover, no obvious toxicity to the liver, kidney or other organs was detected through biochemical and pathological tests, suggesting the good biocompatibility of Fe3O4@PDA-VCR-FA SPs. Zeng et al. (2021b) recently reported a novel RGD-mediated photosensitive drug-peptide conjugate (BBTD + GA/PEG-RGD) for the treatment of musculoskeletal invasive bladder cancer. This system can specifically target integrin αvβ3 outside the membrane of bladder cancer. Meanwhile, the system can prevent the overexpression of heat shock protein 90 and reduce the resistance of cancer cells to heat stress, finally succeeding in low-temperature PTT with great antitumor properties (Figure 3). Furthermore, the results of animal experiments showed that this system had advantages involving 1) good tumor targeting ability and stability; 2) less thermal damage to normal tissue; 3) great therapeutic effects against musculoskeletal invasive bladder cancer; and 4) a longer survival period compared to the control groups. Low-temperature PTT is highly effective in preventing tumor growth without damaging normal tissues, promising great clinical applications for optical tumor therapy in the future.
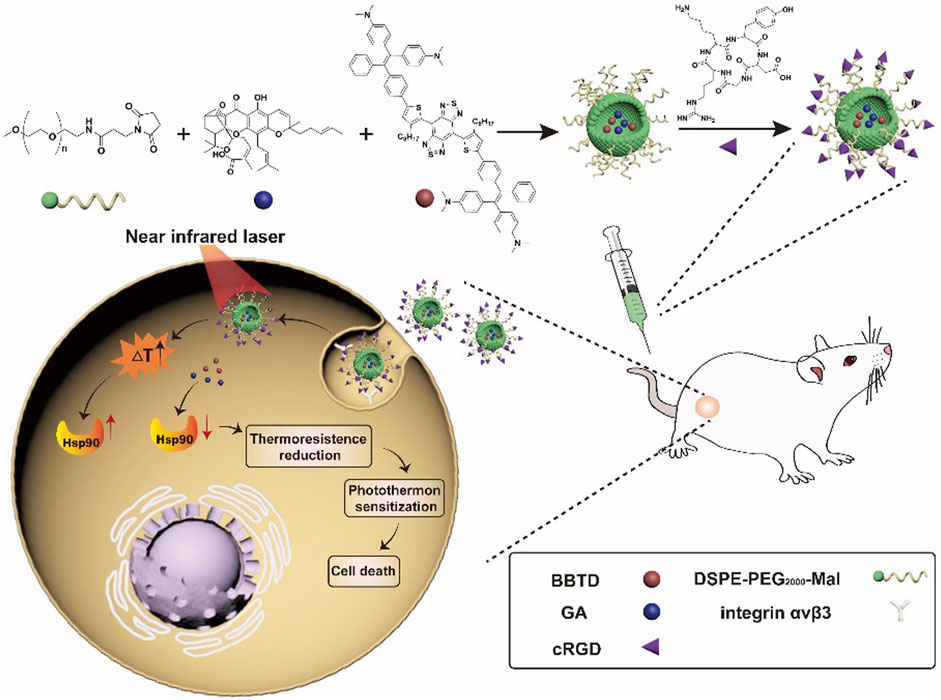
FIGURE 3. Schematic illustration of synthesizing BBTD + GA/PEG-cRGD nanoparticles for photohyperthermia therapy of MIBC(Zeng et al., 2021a).
Therapeutic Peptides
Mitochondria play an important role in apoptotic death (Bock and Tait, 2020), and some anticancer agents can destroy mitochondrial functions and induce tumor cell apoptosis (Vasan et al., 2020). One typical example is the cationic amphiphilic peptide KLAKLAKKLAKLAK (i.e., KLA). KLA is a natural antibacterial peptide that can bind and damage negatively charged bacterial membranes. Normally, KLA does not damage eukaryotic membranes and has no toxicity to eukaryotic cells. However, internalized KLA can rupture the mitochondrial membrane, resulting in cytochrome C release and cell apoptosis (Huang et al., 2017). KLA is always conjugated with transmembrane peptides (CPPs) to promote its internalization efficiency by tumor cells; however, the conjugated KLA-CPPs also have high cytotoxicity to normal cells because of their nonspecific interactions (Wang et al., 2016). To overcome the potential nonspecific interactions, Jung et al. designed and synthesized a mixed peptide (Bld-1-KLA) consisting of 1) a targeting peptide to bladder cancer cells CSNRDARRC (Bld-1) and 2) an effector peptide D-KLAKLAKKLAKLAK (KLA) that can destroy the mitochondrial membrane and induce apoptosis. Bld-1-KLA can selectively bind and internalize into bladder cancer cells to induce cell apoptosis without significant toxicity to other tumor cells and normal cells. After intravenous administration of Bld-1-KLA in the HT1376 tumor-bearing mouse model, it was shown that Bld-1-KLA had a higher tumor homing and inhibition ability than the control groups (Figure 4). Together, these results suggest that Bld-1-KLA is a promising targeted therapeutic against bladder cancer (Jung et al., 2016).
Fibroblast cytokine 9 (FGF9) is overexpressed in many cancer cells (Ren et al., 2016; Mizukami et al., 2017), and its targeted receptor FGFR3c is an important driver of bladder cancer progression (Iyer and Milowsky, 2013; Wang et al., 2020). The important role of FGFR3c makes it an important therapeutic target for the treatment of bladder cancer. Wang et al. (2020) reported one FGF9 binding peptide, P4, using the phage display technique. Meanwhile, they found that P4 is highly homologous to the immunoglobulin-like domain II-III (D2-D3) of FGFR3c using sequence comparison. Functional analysis showed that P4 had the ability to prevent the FGF9-induced aggressive phenotypes, including cell proliferation, migration, and invasion, and inhibit tumor progression by downregulating the MAPK and Akt cascade pathways. More importantly, FGF9 was found to be a potential driver of drug resistance in gastric and bladder cancer cells, in which the presence of P4 can increase the sensitivity of chemical drugs. In conclusion, Wang’s study identified a novel FGF9-binding peptide that may serve as a potential agent to treat malignancies with abnormally upregulated FGF9.
Conclusion
In recent decades, significant success has been achieved in the diagnosis, treatment, and prevention of bladder cancer. However, bladder cancer is characterized by polycentricity, multiple occurrences, and recurrence, suggesting great challenges for its clinical treatment. With the development of modern biosynthesis technology, peptide nano drugs have become one of the hot spots in drug research. Compared with monoclonal antibody drugs, recombinant protein drugs and small molecule drugs, peptide nano drugs have the characteristics of simple spatial structure, significant curative effect and high safety, and have been widely used in the diagnosis and treatment of tumors. With the continuous progress of related technologies, the clinical application of peptide nano drugs is more and more in-depth, and the development space is broad. Peptides are promising for intracellular delivery of chemical drugs, DNA, siRNA, fluorescent molecules and nanoparticles. Compared with other chemical entities, peptides have the advantages of low molecular weight, low cost and good stability. At the same time, polypeptides can be easily modified to attach and enter tumor cells, and finally transport the goods to the desired and desired places. In general, peptide nanodrugs can improve tumor targeting and permeability, reduce systemic toxicity, reduce and prevent recurrence, shorten treatment time and reduce treatment cost. They are of great value for the clinical application of bladder cancer. Peptide drugs have outstanding advantages. With the continuous progress of biotechnology and peptide synthesis technology, peptide drugs have broad market development space and are expected to become one of the main drugs for cancer diagnosis and treatment (Lin et al., 2021).
Author Contributions
SZ and XF wrote the manuscript. SX, ZX, and ZM collected references. QL supervised the whole work. All the authors approved this manuscript.
Funding
This work was financially supported by the General Project of Tianjin Natural Science Foundation (21JCYBJC00220), Tianjin Health Science and technology project (ZC20162) and Tianjin Key Medical Discipline (Specialty) Construction Project.
Conflict of Interest
The authors declare that the research was conducted in the absence of any commercial or financial relationships that could be construed as a potential conflict of interest.
Publisher’s Note
All claims expressed in this article are solely those of the authors and do not necessarily represent those of their affiliated organizations, or those of the publisher, the editors and the reviewers. Any product that may be evaluated in this article, or claim that may be made by its manufacturer, is not guaranteed or endorsed by the publisher.
References
Al-Sukhun, S., and Hussain, M. (2003). Current Understanding of the Biology of Advanced Bladder Cancer. Cancer 97, 2064–2075. doi:10.1002/cncr.11289
Alfred Witjes, J., Lebret, T., Compérat, E. M., Cowan, N. C., De Santis, M., Bruins, H. M., et al. (2017). Updated 2016 EAU Guidelines on Muscle-Invasive and Metastatic Bladder Cancer. Eur. Urol. 71, 462–475. doi:10.1016/j.eururo.2016.06.020
Babjuk, M., Böhle, A., Burger, M., Capoun, O., Cohen, D., Compérat, E. M., et al. (2017). EAU Guidelines on Non-muscle-invasive Urothelial Carcinoma of the Bladder: Update 2016. Eur. Urol. 71, 447–461. doi:10.1016/j.eururo.2016.05.041
Barani, M., Hosseinikhah, S. M., Rahdar, A., Farhoudi, L., Arshad, R., Cucchiarini, M., et al. (2021). Nanotechnology in Bladder Cancer: Diagnosis and Treatment. Cancers 13, 2214. doi:10.3390/cancers13092214
Beyersdorff, D., Taupitz, M., Giessing, M., Türk, I., Schnorr, D., Loening, S., et al. (2000). Staging von Harnblasentumoren in der MRT: Wertigkeit der intravesikalen Applikation von eisenoxidhaltigem Kontrastmittel in Kombination mit hochaufgelöster T2-gewichteter Bildgebung. Rofo Fortschr Geb. Rontgenstr Neuen Bildgeb. Verfahr 172, 504–508. doi:10.1055/s-2000-3751
Bock, F. J., and Tait, S. W. G. (2020). Mitochondria as Multifaceted Regulators of Cell Death. Nat. Rev. Mol. Cell. Biol. 21, 85–100. doi:10.1038/s41580-019-0173-8
Booth, C. M., Karim, S., Brennan, K., Siemens, D. R., Peng, Y., and Mackillop, W. J. (2018). Perioperative Chemotherapy for Bladder Cancer in the General Population: Are Practice Patterns Finally Changing? Urologic Oncol. Seminars Orig. Investigations 36, 89.e13–89.e20. doi:10.1016/j.urolonc.2017.11.015
Calabrò, F., and Sternberg, C. N. (2009). Neoadjuvant and Adjuvant Chemotherapy in Muscle-Invasive Bladder Cancer. Eur. Urol. 55, 348–358. doi:10.1016/j.eururo.2008.10.016
Chandra, V., Park, J., Chun, J. W., Hwang, I. C., and Kim, K. S. (2010). Water-Dispersible Magnetite-Reduced Graphene Oxide Composites for Arsenic Removal. ACS Nano 4, 3979–3986. doi:10.1021/nn1008897
Chang, D., Tsai, R. T., Wang, M., Ou, W. C., Tzeng, T. Y., Fung, C. Y., et al. (1997). Self-assembly of the JC Virus Major Capsid Protein, VP1, Expressed in Insect Cells. J. Gen. Virol. 78 (Pt 6), 1435–1439. doi:10.1099/0022-1317-78-6-1435
Chen, Q., Liang, C., Wang, X., He, J., Li, Y., and Liu, Z. (2014). An Albumin-Based Theranostic Nano-Agent for Dual-Modal Imaging Guided Photothermal Therapy to Inhibit Lymphatic Metastasis of Cancer Post Surgery. Biomaterials 35, 9355–9362. doi:10.1016/j.biomaterials.2014.07.062
Chen, M., Zhang, X., Liu, J., Liu, F., Zhang, R., Wei, P., et al. (2020). Evoking Photothermy by Capturing Intramolecular Bond Stretching Vibration-Induced Dark-State Energy. Acs. Nano. 14, 4265–4275. doi:10.1021/acsnano.9b09625
Cheng, D.-B., Wang, D., Gao, Y.-J., Wang, L., Qiao, Z.-Y., and Wang, H. (2019). Autocatalytic Morphology Transformation Platform for Targeted Drug Accumulation. J. Am. Chem. Soc. 141, 4406–4411. doi:10.1021/jacs.8b13512
Ciobanasu, C. (2021). Peptides-based Therapy and Diagnosis. Strategies for Non-invasive Therapies in Cancer. J. Drug Target. 29, 1063–1079. doi:10.1080/1061186X.2021.1906885
Cooper, B. M., Iegre, J., O' Donovan, D. H., Ölwegård Halvarsson, M., and Spring, D. R. (2021). Peptides as a Platform for Targeted Therapeutics for Cancer: Peptide-Drug Conjugates (PDCs). Chem. Soc. Rev. 50, 1480–1494. doi:10.1039/d0cs00556h
DeGeorge, K. C., Holt, H. R., and Hodges, S. C. (2017). Bladder Cancer: Diagnosis and Treatment. Am. Fam. Physician. 96, 507–514. Available at: https://www.aafp.org/journals/afp.html
Ding, C., Wu, K., Wang, W., Guan, Z., Wang, L., Wang, X., et al. (2017). Synthesis of a Cell Penetrating Peptide Modified Superparamagnetic Iron Oxide and MRI Detection of Bladder Cancer. Oncotarget 8, 4718–4729. doi:10.18632/oncotarget.13578
Dunbar, C. E., High, K. A., Joung, J. K., Kohn, D. B., Ozawa, K., and Sadelain, M. (2018). Gene Therapy Comes of Age. Science 12, eaan4672. doi:10.1126/science.aan4672
Faba, O. R., Tyson, M. D., Artibani, W., Bochner, B. H., Burkhard, F., Gilbert, S. M., et al. (2019). Update of the ICUD-SIU International Consultation on Bladder Cancer 2018: Urinary Diversion. World. J. Urol. 37, 85–93. doi:10.1007/s00345-018-2484-3
Fan, L., Zhang, X., Liu, X., Sun, B., Li, L., and Zhao, Y. (2021). Responsive Hydrogel Microcarrier‐Integrated Microneedles for Versatile and Controllable Drug Delivery. Adv. Healthc. Mat. 10, 2002249. doi:10.1002/adhm.202002249
Gao, G., Jiang, Y. W., Sun, W., Guo, Y., Jia, H. R., Yu, X. W., et al. (2019). Molecular Targeting‐Mediated Mild‐Temperature Photothermal Therapy with a Smart Albumin‐Based Nanodrug. Small 15, 1900501. doi:10.1002/smll.201900501
Ginn, S. L., Amaya, A. K., Alexander, I. E., Edelstein, M., and Abedi, M. R. (2018). Gene Therapy Clinical Trials Worldwide to 2017: An Update. J. Gene. Med. 20, e3015. doi:10.1002/jgm.3015
Grossman, H. B., Soloway, M., Messing, E., Katz, G., Stein, B., Kassabian, V., et al. (2006). Surveillance for Recurrent Bladder Cancer Using a Point-Of-Care Proteomic Assay. JAMA 295, 299–305. doi:10.1001/jama.295.3.299
GuhaSarkar, S., and Banerjee, R. (2010). Intravesical Drug Delivery: Challenges, Current Status, Opportunities and Novel Strategies. J. Control. Release 148, 147–159. doi:10.1016/j.jconrel.2010.08.031
Guo, H., Xu, W., Chen, J., Yan, L., Ding, J., Hou, Y., et al. (2017). Positively Charged Polypeptide Nanogel Enhances Mucoadhesion and Penetrability of 10-hydroxycamptothecin in Orthotopic Bladder Carcinoma. J. Control. Release 259, 136–148. doi:10.1016/j.jconrel.2016.12.041
Guo, H., Li, F., Qiu, H., Xu, W., Li, P., Hou, Y., et al. (2020). Synergistically Enhanced Mucoadhesive and Penetrable Polypeptide Nanogel for Efficient Drug Delivery to Orthotopic Bladder Cancer. Research 2020, 1–14. doi:10.34133/2020/8970135
Hainaut, P., Soussi, T., Shomer, B., Hollstein, M., Greenblatt, M., Hovig, E., et al. (1997). Database of P53 Gene Somatic Mutations in Human Tumors and Cell Lines: Updated Compilation and Future Prospects. Nucleic Acids Res. 25, 151–157. doi:10.1093/nar/25.1.151
High, K. A., and Roncarolo, M. G. (2019). Gene Therapy. N. Engl. J. Med. 381, 455–464. doi:10.1056/NEJMra1706910
Hsieh, J.-T., Zhou, J., Gore, C., and Zimmern, P. (2011). R11, a Novel Cell-Permeable Peptide, as an Intravesical Delivery Vehicle. Bju. Int. 108, 1666–1671. doi:10.1111/j.1464-410X.2011.10185.x
Huang, Y., Li, X., Sha, H., Zhang, L., Bian, X., Han, X., et al. (2017). Tumor-penetrating Peptide Fused to a Pro-apoptotic Peptide Facilitates Effective Gastric Cancer Therapy. Oncol. Rep. 37, 2063–2070. doi:10.3892/or.2017.5440
Huang, X., Chen, T., Mu, N., Lam, H. W., Sun, C., Yue, L., et al. (2021). Supramolecular Micelles as Multifunctional Theranostic Agents for Synergistic Photodynamic Therapy and Hypoxia-Activated Chemotherapy. Acta Biomater. 131, 483–492. doi:10.1016/j.actbio.2021.07.014
Iyer, G., and Milowsky, M. I. (2013). Fibroblast Growth Factor Receptor-3 in Urothelial Tumorigenesis. Urologic Oncol. Seminars Orig. Investigations 31, 303–311. doi:10.1016/j.urolonc.2011.12.001
Jiang, Y., Duan, X., Bai, J., Tian, H., Ding, D., and Geng, Y. (2020). Polymerization-induced Photothermy: A Non-donor-acceptor Approach to Highly Effective Near-Infrared Photothermal Conversion Nanoparticles. Biomaterials 255, 120179. doi:10.1016/j.biomaterials.2020.120179
Jun, Y.-w., Seo, J.-w., and Cheon, J. (2008). Nanoscaling Laws of Magnetic Nanoparticles and Their Applicabilities in Biomedical Sciences. Acc. Chem. Res. 41, 179–189. doi:10.1021/ar700121f
Jung, H.-K., Kim, S., Park, R.-W., Park, J.-Y., Kim, I.-S., and Lee, B. (2016). Bladder Tumor-Targeted Delivery of Pro-apoptotic Peptide for Cancer Therapy. J. Control. Release 235, 259–267. doi:10.1016/j.jconrel.2016.06.008
Kluth, L. A., Black, P. C., Bochner, B. H., Catto, J., Lerner, S. P., Stenzl, A., et al. (2015). Prognostic and Prediction Tools in Bladder Cancer: A Comprehensive Review of the Literature. Eur. Urol. 68, 238–253. doi:10.1016/j.eururo.2015.01.032
Kwak, M. H., Yang, S. M., Yun, S. K., Kim, S., Choi, M.-G., and Park, J. M. (2021). Identification and Validation of LGR5-Binding Peptide for Molecular Imaging of Gastric Cancer. Biochem. Biophysical Res. Commun. 580, 93–99. doi:10.1016/j.bbrc.2021.09.073
Lai, W.-H., Fang, C.-Y., Chou, M.-C., Lin, M.-C., Shen, C.-H., Chao, C.-N., et al. (2021). Peptide-guided JC Polyomavirus-like Particles Specifically Target Bladder Cancer Cells for Gene Therapy. Sci. Rep. 11, 11889. doi:10.1038/s41598-021-91328-7
Lee, S.-M., Lee, E.-J., Hong, H.-Y., Kwon, M.-K., Kwon, T.-H., Choi, J.-Y., et al. (2007). Targeting Bladder Tumor Cells In Vivo and in the Urine with a Peptide Identified by Phage Display. Mol. Cancer. Res. 5, 11–19. doi:10.1158/1541-7786.MCR-06-0069
Lin, Y.-X., Wang, Y., An, H.-W., Qi, B., Wang, J., Wang, L., et al. (2019). Peptide-Based Autophagic Gene and Cisplatin Co-delivery Systems Enable Improved Chemotherapy Resistance. Nano Lett. 19, 2968–2978. doi:10.1021/acs.nanolett.9b00083
Lin, W., Liu, H., Chen, L., Chen, J., Zhang, D., Cheng, Q., et al. (2021). Pre-clinical MRI-Guided Intravesical Instillation Theranosis of Bladder Cancer by Tumor-Selective Oxygen Nanogenerator. Nano. Today. 38, 101124. doi:10.1016/j.nantod.2021.10112410.1016/j.nantod.2021.101124
Lu, S., Xu, L., Kang, E. T., Mahendran, R., Chiong, E., and Neoh, K. G. (2016). Co-delivery of Peptide-Modified Cisplatin and Doxorubicin via Mucoadhesive Nanocapsules for Potential Synergistic Intravesical Chemotherapy of Non-muscle-invasive Bladder Cancer. Eur. J. Pharm. Sci. 84, 103–115. doi:10.1016/j.ejps.2016.01.013
Lu, L., Zhang, Q., Wang, Z., Gao, L., and Shen, J. (2021). Peptide-Modified Nanoparticles for Tumor Targeting and Molecular Imaging. Cmc 28, 6411–6436. doi:10.2174/0929867327666201022122131
Mizukami, T., Togashi, Y., Naruki, S., Banno, E., Terashima, M., de Velasco, M. A., et al. (2017). Significance of FGF9 Gene in Resistance to Anti-EGFR Therapies Targeting Colorectal Cancer: A Subset of Colorectal Cancer Patients withFGF9upregulation May Be Resistant to Anti-EGFR Therapies. Mol. Carcinog. 56, 106–117. doi:10.1002/mc.22476
Moore, A., Josephson, L., Bhorade, R. M., Basilion, J. P., and Weissleder, R. (2001). Human Transferrin Receptor Gene as a Marker Gene for MR Imaging. Radiology 221, 244–250. doi:10.1148/radiol.2211001784
Nakamura, Y., Miyata, Y., Takehara, K., Asai, A., Mitsunari, K., Araki, K., et al. (2019). The Pathological Significance and Prognostic Roles of Thrombospondin-1, and -2, and 4N1K-Peptide in Bladder Cancer. Anticancer. Res. 39 (5), 2317–2324. doi:10.21873/anticanres.13348
Oh, D.-Y., and Bang, Y.-J. (2020). HER2-targeted Therapies - a Role beyond Breast Cancer. Nat. Rev. Clin. Oncol. 17, 33–48. doi:10.1038/s41571-019-0268-3
Pan, Y., Volkmer, J.-P., Mach, K. E., Rouse, R. V., Liu, J.-J., Sahoo, D., et al. (2014). Endoscopic Molecular Imaging of Human Bladder Cancer Using a CD47 Antibody. Sci. Transl. Med. 6, 260ra148. doi:10.1126/scitranslmed.3009457
Pan, A., Zhang, H., Li, Y., Lin, T.-y., Wang, F., Lee, J., et al. (2016). Disulfide-crosslinked Nanomicelles Confer Cancer-specific Drug Delivery and Improve Efficacy of Paclitaxel in Bladder Cancer. Nanotechnology 27, 425103. doi:10.1088/0957-4484/27/42/425103
Ren, C., Chen, H., Han, C., Fu, D., Wang, F., Wang, D., et al. (2016). The Anti-apoptotic and Prognostic Value of Fibroblast Growth Factor 9 in Gastric Cancer. Oncotarget 7, 36655–36665. doi:10.18632/oncotarget.9131
Schroeder, T. (2008). Imaging Stem-Cell-Driven Regeneration in Mammals. Nature 453, 345–351. doi:10.1038/nature07043
Selivanova, G., Iotsova, V., Okan, I., Fritsche, M., Ström, M., Groner, B., et al. (1997). Restoration of the Growth Suppression Function of Mutant P53 by a Synthetic Peptide Derived from the P53 C-Terminal Domain. Nat. Med. 3, 632–638. doi:10.1038/nm0697-632
Sonju, J. J., Dahal, A., Singh, S. S., and Jois, S. D. (2021). Peptide-functionalized Liposomes as Therapeutic and Diagnostic Tools for Cancer Treatment. J. Control. Release 329, 624–644. doi:10.1016/j.jconrel.2020.09.055
Sweeney, S., Luo, Y., O'Donnell, M., and Assouline, J. (2017). Peptide-Mediated Targeting Mesoporous Silica Nanoparticles: A Novel Tool for Fighting Bladder Cancer. J. Biomed. Nanotechnol. 13, 232–242. doi:10.1166/jbn.2017.2339
Tao, K., Liu, S., Wang, L., Qiu, H., Li, B., Zhang, M., et al. (2019). Targeted Multifunctional Nanomaterials with MRI, Chemotherapy and Photothermal Therapy for the Diagnosis and Treatment of Bladder Cancer. Biomater. Sci. 8, 342–352. doi:10.1039/c9bm01377f
Timko, B. P., Dvir, T., and Kohane, D. S. (2010). Remotely Triggerable Drug Delivery Systems. Adv. Mat. 22, 4925–4943. doi:10.1002/adma.201002072
Todenhöfer, T., Struss, W. J., Seiler, R., Wyatt, A. W., and Black, P. C. (2018). Liquid Biopsy-Analysis of Circulating Tumor DNA (ctDNA) in Bladder Cancer. Bladder Cancer 4, 19–29. doi:10.3233/BLC-170140
Tree, A. C., Jones, K., Hafeez, S., Sharabiani, M. T. A., Harrington, K. J., Lalondrelle, S., et al. (2018). Dose-limiting Urinary Toxicity with Pembrolizumab Combined with Weekly Hypofractionated Radiation Therapy in Bladder Cancer. Int. J. Radiat. Oncology*Biology*Physics 101, 1168–1171. doi:10.1016/j.ijrobp.2018.04.070
Tummers, W. S., Warram, J. M., Tipirneni, K. E., Fengler, J., Jacobs, P., Shankar, L., et al. (2017). Regulatory Aspects of Optical Methods and Exogenous Targets for Cancer Detection. Cancer. Res. 77, 2197–2206. doi:10.1158/0008-5472.CAN-16-3217
Tyagi, P., Wu, P.-C., Chancellor, M., Yoshimura, N., and Huang, L. (2006). Recent Advances in Intravesical Drug/gene Delivery. Mol. Pharm. 3, 369–379. doi:10.1021/mp060001j
van der Pol, C. B., Chung, A., Lim, C., Gandhi, N., Tu, W., McInnes, M. D. F., et al. (2018). Update on Multiparametric MRI of Urinary Bladder Cancer. J. Magn. Reson. Imaging 48, 882–896. doi:10.1002/jmri.26294
Vasan, K., Werner, M., and Chandel, N. S. (2020). Mitochondrial Metabolism as a Target for Cancer Therapy. Cell. Metab. 32, 341–352. doi:10.1016/j.cmet.2020.06.019
Wang, H., Ma, J., Yang, Y., Zeng, F., and Liu, C. (2016). Highly Efficient Delivery of Functional Cargoes by a Novel Cell-Penetrating Peptide Derived from SP140-like Protein. Bioconjugate Chem. 27, 1373–1381. doi:10.1021/acs.bioconjchem.6b00161
Wang, J., Tan, X., Guo, Q., Lin, X., Huang, Y., Chen, L., et al. (2020). FGF9 Inhibition by a Novel Binding Peptide Has Efficacy in Gastric and Bladder Cancer Per Se and Reverses Resistance to Cisplatin. Pharmacol. Res. 152, 104575. doi:10.1016/j.phrs.2019.104575
Wang, Y., Zhang, X., Wan, K., Zhou, N., Wei, G., and Su, Z. (2021). Supramolecular Peptide Nano-Assemblies for Cancer Diagnosis and Therapy: from Molecular Design to Material Synthesis and Function-specific Applications. J. Nanobiotechnol 19, 253. doi:10.1186/s12951-021-00999-x
Wilson, K., Shiuan, E., and Brantley-Sieders, D. M. (2021). Oncogenic Functions and Therapeutic Targeting of EphA2 in Cancer. Oncogene 40, 2483–2495. doi:10.1038/s41388-021-01714-8
Wirth, M., Plattner, V., and Gabor, F. (2009). Strategies to Improve Drug Delivery in Bladder Cancer Therapy. Expert Opin. Drug Deliv. 6, 727–744. doi:10.1517/17425240903022758
Wołącewicz, M., Hrynkiewicz, R., Grywalska, E., Suchojad, T., Leksowski, T., Roliński, J., et al. (2020). Immunotherapy in Bladder Cancer: Current Methods and Future Perspectives. Cancers 12, 1181. doi:10.3390/cancers12051181
Wu, S., Zheng, J., Li, Y., Wu, Z., Shi, S., Huang, M., et al. (2018). Development and Validation of an MRI-Based Radiomics Signature for the Preoperative Prediction of Lymph Node Metastasis in Bladder Cancer. EBioMedicine 34, 76–84. doi:10.1016/j.ebiom.2018.07.029
Xiao, K., Li, Y., Lee, J. S., Gonik, A. M., Dong, T., Fung, G., et al. (2012). "OA02" Peptide Facilitates the Precise Targeting of Paclitaxel-Loaded Micellar Nanoparticles to Ovarian Cancer In Vivo. Cancer. Res. 72, 2100–2110. doi:10.1158/0008-5472.CAN-11-3883
Yang, J., An, H.-W., and Wang, H. (2021). Self-Assembled Peptide Drug Delivery Systems. ACS Appl. Bio Mat. 4, 24–46. doi:10.1021/acsabm.0c00707
Yin, M., Joshi, M., Meijer, R. P., Glantz, M., Holder, S., Harvey, H. A., et al. (2016). Neoadjuvant Chemotherapy for Muscle-Invasive Bladder Cancer: A Systematic Review and Two-step Meta-Analysis. Oncologist 21, 708–715. doi:10.1634/theoncologist.2015-0440
Zeng, S., Gao, H., Li, C., Xing, S., Xu, Z., Liu, Q., et al. (2021a). Boosting Photothermal Theranostics via TICT and Molecular Motions for Photohyperthermia Therapy of Muscle‐Invasive Bladder Cancer. Adv. Healthc. Mater. 10, 2101063. doi:10.1002/adhm.202101063
Zeng, S., Ou, H., Gao, Z., Zhang, J., Li, C., Liu, Q., et al. (2021b). HCPT-peptide Prodrug with Tumor Microenvironment -responsive Morphology Transformable Characteristic for Boosted Bladder Tumor Chemotherapy. J. Control. Release 330, 715–725. doi:10.1016/j.jconrel.2020.12.042
Keywords: peptide, bladder cancer, diagnosis, treatment, nanomedicines
Citation: Zeng S, Feng X, Xing S, Xu Z, Miao Z and Liu Q (2022) Advanced Peptide Nanomedicines for Bladder Cancer Theranostics. Front. Chem. 10:946865. doi: 10.3389/fchem.2022.946865
Received: 18 May 2022; Accepted: 13 June 2022;
Published: 05 August 2022.
Edited by:
Fan Huang, China Academy of Chinese Medical Sciences, ChinaReviewed by:
Jingyu Wang, Tianjin Medical University, ChinaShenglu Ji, Xinxiang Medical University, China
Ruoyao Zhang, Beijing Institute of Technology, China
Copyright © 2022 Zeng, Feng, Xing, Xu, Miao and Liu. This is an open-access article distributed under the terms of the Creative Commons Attribution License (CC BY). The use, distribution or reproduction in other forums is permitted, provided the original author(s) and the copyright owner(s) are credited and that the original publication in this journal is cited, in accordance with accepted academic practice. No use, distribution or reproduction is permitted which does not comply with these terms.
*Correspondence: Qian Liu, simomlq@163.com
†These authors have contributed equally to this work