- 1Key Laboratory of Rubber-Plastics of Ministry of Education/Shandong Province (QUST), School of Polymer Science and Engineering, Qingdao University of Science and Technology, Qingdao, China
- 2School of Chemical Engineering of Guizhou Minzu University, Guiyang, China
A novel alternating donor–acceptor polymer PQ1 is designed and synthesized by palladium-catalyzed Stille coupling between quinoxaline as an electron-deficient unit and indacenodithiophene (IDT) as electron-rich groups. Polymer PQ1 presents not only a strong intramolecular charge transfer effect, which is beneficial for the charge transport within single molecules but also a narrow electrochemical band gap and a high highest occupied molecular orbital (HOMO) energy level. In addition, the optical absorption study indicates that the PQ1 film exhibits good aggregation, which is an advantage for the charge transport between neighboring molecules. As a consequence, PQ1 presents p-type semiconductor properties with a high hole mobility of up to 0.12 cm2 V−1 s−1. This study reveals the great potential of quinoxaline-type chromophores in constructing novel organic semiconductors.
Introduction
Since the first organic field-effect transistor (OFET) was successfully prepared by Tsumura with polythiophene in 1987, organic semiconductors have received more and more attention from the academic society due to their multi-advantages and potential applications such as low cost, ease of fabrication, and compatibility with flexible substrates (Tsumura et al., 1986; Bogdanov and Mironov, 2021; Wang et al., 2020; Yoon et al., 2017; Zhang et al., 2018). In the past few years, OFETs have made significant achievements in charge carrier mobility, open-circuit voltage (Voc), and current on/off ratio. Regarding semiconductor materials, most used are the organic π-conjugated small molecules and polymers (Cao et al., 2012; Donaghey et al., 2013; Zhang et al., 2020). Compared to small molecules, polymers are more popular and widely used as semiconductor layers in high-performance OFETs, since they are often present not only in high intracharge transport mobility but also in tunable chemical structures (Kettle et al., 2011; Sasikumar et al., 2016; Bhanuprakash et al., 2016; Bi et al., 2018). According to the composition of the polymer backbone, semiconductor polymers are usually classified into three types: A-A type (acceptor–acceptor), D-D type (donor–donor), and D-A type (donor–acceptor). Among them, the D-A type is the most popular and potential in high-performance OFETs due to its convenient molecular orbital modification capability and good intramolecular charge transfer (ICT) effect (Tomasz et al., 2013; Deng et al., 2018a). A suitable donor structure can modulate the highest occupied molecular orbital (HOMO), while a suitable acceptor group might adjust the lowest unoccupied molecular orbital (LUMO). This results in semiconductor materials with an appropriate Frontier molecular orbital level, which aligns with the working function of the electrode, in turn forming an Ohmic contact with it, and facilitates charge injection from the electrode into the semiconductor layer.
Thiophene is a commonly used electron donor unit in π-conjugated polymers. Indacenodithiophene (IDT) belongs to a derivative of thiophene with conjugation system extension. With its excellent planar symmetric structure, it can effectively promote intermolecular charge transfer. Thus, the devices prepared by this structure usually have excellent charge carrier mobility (Cai et al., 2014; Zhai and Zhou, 2016). Recent research has reversed that IDT has become a widely used feeder structure for photovoltaic materials, especially in organic solar cells (OPV), which can provide an energy conversion efficiency of over 9.21% (Andrew et al., 2020). In addition, it is also used as donor units to construct D-A type semiconductors with good hole transport mobility. Apart from the donor unit, a suitable acceptor group also plays a crucial role in designing a high-performance semiconductor (Kettle et al., 2011). The derivatives of quinoxaline, an electron-deficient chromophore, play an important role as a good planar and rigid conjugated structure in organic light-emitting diodes (OLEDs), dyes, or as ligands in light-emitting materials, but are rarely used in the construction of semiconductors in OFETs (Liu et al., 2017; Park et al., 2019; Hee et al., 2020; Sagita, et al., 2021).
In this work, a novel polymer PQ1 is designed and synthesized by the Stille coupling reaction between IDT and thiophene-substituted quinoxaline (Supplementary Scheme S1) (Li et al., 2012; Deng et al., 2018b). In order to improve the solubility of the polymer, multiple large sized alky chains are introduced into the polymer. The optical and electrochemical properties of the polymer are investigated. In addition, the OFETs are constructed using PQ1 as the semiconductor layer, which presents a hole mobility of around 0.1 cm2 V−1 s−1.
Results and Discussion
Computational Study
In order to investigate the Frontier molecular orbital features, backbone configuration, and the HOMO/LUMO energy levels of the two polymers, computational calculations were conducted by density-functional theory (DFT) at the B3LYP/6-31(d,p) level using the model compound containing a single repeating unit with a methyl group instead of an alky chain. As shown in Figure 1A, there is a 61.4 twisting angle between the donor units of IDT and the acceptor unit of quinoxaline. The electronic cloud distributions of LUMO are mainly located at the IDT part, while the LUMO electrons are localized on the quinoxaline groups. This indicates that once the polymer is excited, an electron transfer from the IDT part to the quinoxaline groups could take place, which means that the polymer has a stronger ICT effect. A good ICT effect is beneficial for the charge transport within the individual molecules. The calculated HOMO/LUMO level of PQ1 is -5.24 and -2.20 eV, resulting in a band gap of 3.04 eV.
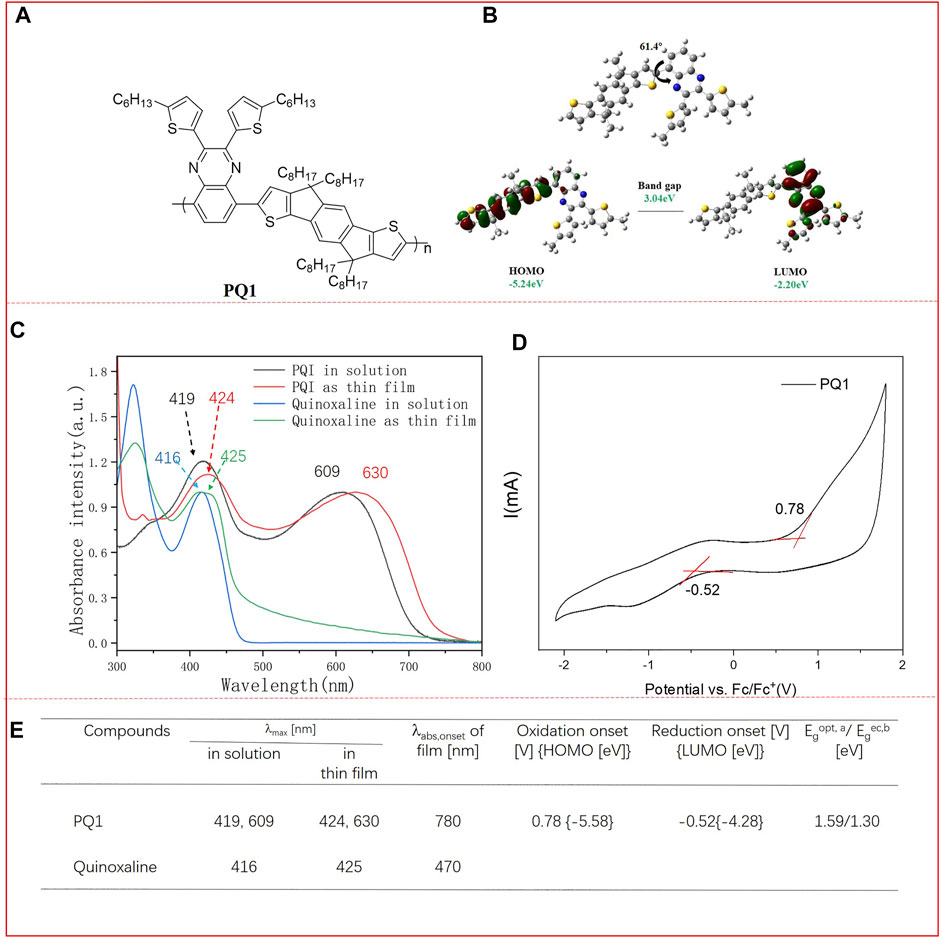
FIGURE 1. (A) Chemical structures of PQ1; (B) computational calculations of the simplified single repeat unit of the polymer obtained at the B3LYP/6-31G* level; (C) UV/vis absorption spectra of the monomer quinoxanline and polymer PQ1 in dichloromethane solution and the polymer thin film; (D) cyclic voltammograms of the polymer PQ1 as thin films deposited on ITO electrolyte: 0.1 M TBAPF6/acetonitrile. Potential calculated versus ferrocene. Scan rate: 100 mV s −1; T = 25°C. (E) Optical and electrochemical properties of the monomer and polymer PQ1. aEopt (optical bandgap) was measured at the onset of absorption in the film (Eopt = 1,240/?abs. onset eV). bEgec electrochemical bandgap. HOMO-LUMO gap was calculated according to the following equations: ELUMO = Eonset (red) + 4.8 eV and EHOMO = Eonset (ox) + 4.8 eV. Eonset (ox) and Eonset (red) are onset potentials for the oxidation and reduction processes vs Fc/Fc+ couple, respectively.
Optical Properties
In order to evaluate the optical properties, UV/vis spectroscopy of the PQ1 polymer is performed in a chloroform solution and thin-film state. The corresponding optical data are summarized in Supplementary Table S1. In Figure 1A, the UV/Vis absorption spectra of quinoxaline and PQI in dichloromethane and in the thin-film state are shown. The polymer PQ1 presents dark blue color in both the solution and thin-film state. The absorption spectrum of the quinoxaline in dichloromethane exhibited a strong absorption maximum (λabs.max) at 416 nm with an extinction coefficient of 3.2×104 L mol−1 cm−1, which was only blue-shifted around 9 nm compared to its thin-film state (λabs.max = 425 nm). After polymerization, absorption of polymer PQ1 exhibited a large shift (around 193 nm) to a longer wavelength in the solutions compared to the monomer (from 416 to 609 nm). The red-shift could be due to the π-conjugation extension and the donor–acceptor interaction within the polymer backbones, which inevitably caused the intramolecular charge transfer (ICT) process to generate more delocalized intramolecular π-orbitals and thus increased the efficient conjugation lengths[23] Regarding the polymer film, a 21-nm red-shifted absorption was observed compared to their solution counterparts. In addition, in the long-wavelength absorption range, the bathochromic shift appeared to be stronger (around 50 nm). These observations could be due to the aggregation, the π–π interaction, and the long-range ordered packing. From the onset of absorption, the optical band gap was calculated to be 1.59 eV.
Electrochemical Properties
The electrochemical properties of the polymer were investigated by cyclic voltammetry. The experimental details are described in the Supporting Information. Based on the onset reduction and oxidation potentials, the LUMO/HOMO energy levels of the monomer and polymers are estimated (Guo et al., 2014). As shown in Figure 1D, PQ1 presents quasi-reversible cathodic and anodic waves. The onset oxidation occurred at 0.78 V and reduction at -0.52 V, based on which the HOMO and LUMO energy levels were calculated to be -5.58 eV and -4.28 eV, respectively. The HOMO energy level of PQ1 is lower than the oxidation threshold of air, i.e., −5.27 eV, indicating the good stability of the materials in air. According to the HOMO/LUMO energy levels, the electrochemical band gap was calculated to be 1.3 eV. This value is smaller than the computation results, which is ascribed to the fact that the computation result is obtained using the single repeat unit, while the electrochemical band gap is obtained using the whole polymer. In addition, the electrochemical band gap is also 0.2 eV smaller than the optical band gap.
OFET Device
The charge transport properties of the PQ1 polymer are evaluated by fabricating the OFET devices of the materials with bottom-gate and bottom-contact (BGBC) configuration on a silicon wafer using a layer of 300 nm SiO2 as the dielectric material. The devices with PQ1 IS were fabricated by direct spin-casting of the polymer solution in toluene onto the OTS-treated silicon wafer with prepatterned gold source and drain electrodes, and measured under vacuum conditions. The devices were measured after thermal annealing at 100°C for 30 min in an argon-filled glove box to remove the remaining solvent residues. The detailed fabrication and testing procedures are described in the Supporting Information. As shown in Figure 2, the PQ1 polymer presents a p-type charge transport behavior with a hole mobility (μh) estimated to be 0.11 cm2 V−1 s−1 (average) for eight different devices. From the eight devices, the highest μh is 0.12 cm2 V−1 s−1. The high hole mobility could be ascribed to the strong ICT effect and the good aggregation (Du et al., 2018; Muhammad et al., 2020).
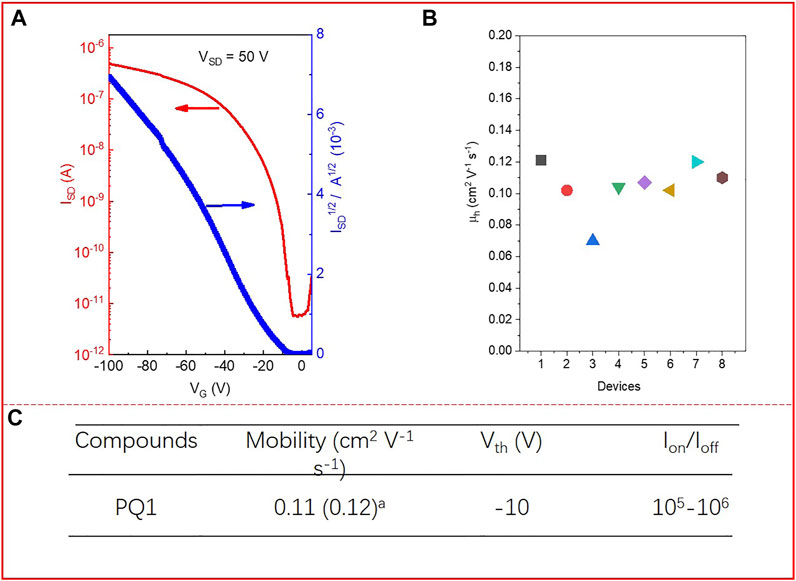
FIGURE 2. (A) Characteristics of the OFET devices of PQ1; (B) hole transport mobility obtained eight different devices. (C) Hole mobilities (µh), threshold voltage (VTh), and on/off ratios (Ion/Ioff) of the PQ1-based FET device. The mobility was provided in average (highest)a form and the performance is based on eight different FETs. Mobility extracted by fitting the linear part of the plot of IDS1/2 versus VG using the equation IDS = Ciµ(VG-VTh)2W/2L. a Hole mobility.
Conclusion
In conclusion, a D-A type polymer PQ1 is successfully designed and synthesized between quinoxaline as the electron-deficient unit and IDT as the electron-rich unit by the Stille coupling reaction. UV-vis spectra showed that after polymerization, there is a large red-shift from the monomer to the polymer, which indicates the strong ICT effect for D-A polymer PQ1. In addition, there is a 21-nm bathochromic shift from the solution to thin film for PQ1, which indicates that the PQ1 film presents good aggregation. The electrochemical study indicates that PQ1 exhibits a low band gap with a HOMO energy level of −5.48 eV, which is lower than the oxidation threshold of air, i.e., −5.27 eV, indicating the good stability of PQ1 in air. Using PQ1 as the semiconductor layer to construct OFET presents p-type behavior with a hole mobility of up to 0.12 cm2 V−1 s−1. This study demonstrated the great potential of quinoxaline-type chromophores in constructing novel organic semiconductors, and thus, further modification of quinoxaline structures is currently underway in our laboratory.
Data Availability Statement
The datasets presented in this study can be found in online repositories. The names of the repository/repositories and accession number(s) can be found in the article/Supplementary Material.
Author Contributions
DZ helped design, synthesize, and characterize the polymers. In addition, part of the manuscript was written by him. ZD synthesized and characterized the materials. In addition, part of the manuscript was written by him. HZ designed the materials, helped discuss the whole characterization, and revised the whole manuscript.
Conflict of Interest
The authors declare that the research was conducted in the absence of any commercial or financial relationships that could be construed as a potential conflict of interest.
Publisher’s Note
All claims expressed in this article are solely those of the authors and do not necessarily represent those of their affiliated organizations, or those of the publisher, the editors, and the reviewers. Any product that may be evaluated in this article, or claim that may be made by its manufacturer, is not guaranteed or endorsed by the publisher.
Acknowledgments
The authors acknowledge the support from Young Taishan Scholars under Grant 201909120, the Natural Science Foundation of China (Grant: 52163001), Guizhou Province Science and Technology Plan Projects (Grants: CXTD(2021)005 and (2018)1012), Baiyun District Science and Technology Plan Projects (Grant: (2020)28), and Polymer Composites Engineering Research Center of Guizhou Minzu University (Grant: GZMUGCZX (2021)01).
Supplementary Material
The Supplementary Material for this article can be found online at: https://www.frontiersin.org/articles/10.3389/fchem.2022.934203/full#supplementary-material
References
Andrew, W., Hu, C., Karl, T., Camila, C., Mark, N., Helen, B., et al. (2020). Modification of Indacenodithiophene-Based Polymers and its Impact on Charge Carrier Mobility in Organic Thin-Film Transistors. J. Am. Chem. Soc. 14, 2652–2664. doi:10.1021/jacs.9b09374
Arshad, M., Du, H., Javed, M. S., Maqsood, A., Ashraf, I., Hussain, S., et al. (2020). Fabrication, Structure, and Frequency-dependent Electrical and Dielectric Properties of Sr-Doped BaTiO3 Ceramics. Ceram. Int. 46, 2238–2246. doi:10.1016/j.ceramint.2019.09.208
Bi, S., Li, Y., He, Z., Ouyang, Z., Guo, Q., and Jiang, C. (2019). Self-assembly Diketopyrrolopyrrole-Based Materials and Polymer Blend with Enhanced Crystal Alignment and Property for Organic Field-Effect Transistors. Org. Electron. 65, 96–99. doi:10.1016/j.orgel.2018.11.008
Bogdanov, A. V., and Mironov, V. F. (2021). Recent Advances in the Application of Isoindigo Derivatives in Materials Chemistry. Beilstein J. Org. Chem. 17, 1533–1564. doi:10.3762/bjoc.17.111
Cai, Z., Luo, H., Chen, X., Zhang, G., Liu, Z., and Zhang, D. (2014). Extended Conjugated Donor-Acceptor Molecules withE-(1,2-Difluorovinyl) and Diketopyrrolopyrrole (DPP) Moieties toward High-Performance Ambipolar Organic Semiconductors. Chem. Asian J. 9, 1068–1075. doi:10.1002/asia.201301312
Cao, K., Sun, X., Zhang, Q., and Liu, Y. (2012). Synthesis and Characterization of a 2,4,6-Tri(2-Thienyl)pyridine-Based Conjugated Polymer for OFET Applications. Macromol. Chem. Phys. 213, 917–923. doi:10.1002/macp.201100660
Deng, Z., Li, L., Ai, T., Hao, X., and Bao, W. (2018a). Centrosymmetric Thiophenemethyleneoxindole-Based Donor-Acceptor Copolymers for Organic Field-Effect Transistors. Macromol. Rapid Commun. 39, 1800073. doi:10.1002/marc.201800073
Deng, Z., Yang, K., Li, L., Bao, W., Hao, X., Ai, T., et al. (2018b). Solution Processed Air-Stable P-Channel Organic Crystal Field-Effect Transistors of Aminobenzodifuranone. Dyes Pigments 151, 173–178. doi:10.1016/j.dyepig.2017.12.052
Donaghey, J. E., Sohn, E.-H., Ashraf, R. S., Anthopoulos, T. D., Watkins, S. E., Song, K., et al. (2013). Pyrroloindacenodithiophene Polymers: the Effect of Molecular Structure on OFET Performance. Polym. Chem. 4, 3537–3544. doi:10.1039/c3py00335c
Du, H., Ma, C., Ma, W., and Wang, H. (2018). Microstructure Evolution and Dielectric Properties of Ce-Doped SrBi4Ti4O15 Ceramics Synthesized via Glycine-Nitrate Process. Pac 12, 303–312. doi:10.2298/PAC1804303D
Guo, X., Facchetti, A., and Marks, T. J. (2014). Imide- and Amide-Functionalized Polymer Semiconductors. Chem. Rev. 114, 8943–9021. doi:10.1021/cr500225d
Kettle, J., Horie, M., Majewski, L. A., Saunders, B. R., Tuladhar, S., Nelson, J., et al. (2011). Optimisation of PCPDTBT Solar Cells Using Polymer Synthesis with Suzuki Coupling. Sol. Energy Mater. Sol. Cells 95, 2186–2193. doi:10.1016/j.solmat.2011.03.022
Kim, H. S., Rasool, S., Shin, W. S., Song, C. E., and Hwang, D.-H. (2020). Alkylated Indacenodithiophene-Based Non-fullerene Acceptors with Extended π-Conjugation for High-Performance Large-Area Organic Solar Cells. ACS Appl. Mat. Interfaces 12, 50638–50647. doi:10.1021/acsami.0c13277
Lee, Y. H., Jang, M., Lee, M. Y., Kweon, O. Y., and Oh, J. H. (2017). Flexible Field-Effect Transistor-type Sensors Based on Conjugated Molecules. Chem 3, 724–763. doi:10.1016/j.chempr.2017.10.005
Li, S., He, Z., Yu, J., Chen, S. a., Zhong, A., Wu, H., et al. (2012). 2,3‐bis(5‐Hexylthiophen‐2‐yl)‐6,7‐bis(octyloxy)‐5,8‐di(thiophen‐2‐yl) Quinoxaline: A Good Construction Block with Adjustable Role in the Donor‐π‐acceptor System for Bulk‐heterojunction Solar Cells. J. Polym. Sci. A Polym. Chem. 50, 2819–2828. doi:10.1002/pola.26086
Liu, M., Gao, Y., Zhang, Y., Liu, Z., and Zhao, L. (2017). Quinoxaline-based Conjugated Polymers for Polymer Solar Cells. Polym. Chem. 8, 4613–4636. doi:10.1039/C7PY00850C
Marszalek, T., Wiatrowski, M., Dobruchowska, E., Jung, J., and Ulanski, J. (2013). One-step Technique for Production of Bi-functional Low Molecular Semiconductor-Polymer Composites for Flexible OFET Applications. J. Mat. Chem. C 1, 3190–3193. doi:10.1039/C3TC30163J
Park, J. M., Jung, C. Y., Wang, Y., Choi, H. D., Park, S. J., Ou, P., et al. (2019). Effect of Regioisomeric Substitution Patterns on the Performance of Quinoxaline-Based Dye-Sensitized Solar Cells. Electrochimica Acta 298, 650–662. doi:10.1016/j.electacta.2018.12.133
Sagita, C. P., Lee, J. H., Lee, S. W., Whang, D. R., Kim, J. H., and Chang, D. W. (2021). Enhanced Photovoltaic Performance of Quinoxaline-Based Donor-Acceptor Type Polymers with Monocyano Substituent. J. Power Sources 491, 229588. doi:10.1016/j.jpowsour.2021.229588
Sasikumar, M., Bharath, D., Kumar, G. S., Chereddy, N. R., Chithiravel, S., Krishnamoorthy, K., et al. (2016). Role of Acceptor Strength on OFET Properties of Small Molecular Organic Semiconducting Materials with D-A-D Architecture. Synth. Met. 220, 236–246. doi:10.1016/j.synthmet.2016.06.006
Tsumura, A., Koezuka, H., and Ando, T. (1986). Macromolecular Electronic Device: Field‐effect Transistor with a Polythiophene Thin Film. Appl. Phys. Lett. 49, 1210–1212. doi:10.1063/1.97417
Wang, Y., Gong, Q., and Miao, Q. (2020). Structured and Functionalized Organic Semiconductors for Chemical and Biological Sensors Based on Organic Field Effect Transistors. Mat. Chem. Front. 4, 3505–3520. doi:10.1039/D0QM00202J
Zhai, W., and Zhou, E. (2016). Progress of Organic Photovoltaic Materials Based on Indacenodithiophene and its Derivatives. Chin. J. Org. Chem. 36, 2786–2812. doi:10.6023/cjoc201604052
Zhang, H., Li, R., Deng, Z., Cui, S., Wang, Y., Zheng, M., et al. (2020). π-Conjugated Oligomers Based on Aminobenzodifuranone and Diketopyrrolopyrrole. Dyes Pigments 181, 108552. doi:10.1016/j.dyepig.2020.108552
Keywords: organic field-effect transistor, conjugated polymers, quinoxaline, donor, acceptor
Citation: Dai Z, Zhang D and Zhang H (2022) Design, Synthesis, and Application in OFET of a Quinoxaline-Based D-A Conjugated Polymer. Front. Chem. 10:934203. doi: 10.3389/fchem.2022.934203
Received: 02 May 2022; Accepted: 10 May 2022;
Published: 16 June 2022.
Edited by:
Yue Liu, Liaoning Technical University, ChinaReviewed by:
Xu Qiu, Shandong University of Science and Technology, ChinaDetebg Zhang, Qingdao University, China
Copyright © 2022 Dai, Zhang and Zhang. This is an open-access article distributed under the terms of the Creative Commons Attribution License (CC BY). The use, distribution or reproduction in other forums is permitted, provided the original author(s) and the copyright owner(s) are credited and that the original publication in this journal is cited, in accordance with accepted academic practice. No use, distribution or reproduction is permitted which does not comply with these terms.
*Correspondence: Haichang Zhang, aGFpY2hhbmd6aGFuZ0Bob3RtYWlsLmNvbQ==