- 1College of Science, Donghua University, Shanghai, China
- 2College of Chemistry and Chemical Engineering, Shanghai University of Engineering Science, Shanghai, China
CeO2 with fusiform structures were prepared by the combined microwave–ultrasonic method, and their morphologies and surface structure were changed by simply adding different amounts of H2O (1–5 ml) to the precursor system. The addition of H2O changed the PVP micelle structure and the surface state, resulting in CeO2 with a different specific surface area (64–111 m2 g−1) and Ce3+ defects (16.5%–28.1%). The sample with 2 ml H2O exhibited a high surface area (111.3 m2∙g−1) and relatively more surface defects (Ce3+%: 28.1%), resulting in excellent catalytic activity (4.34 mmol g−1 h−1).
Highlights
1. Fusiform-like CeO2 was prepared by the combined microwave–ultrasonic method.
2. 1–5 ml H2O in the precursor can influence the morphology, surface area, and Ce3+% of the CeO2 catalyst.
3. H2O changed the PVP micelle structure, leading to modulation of the CeO2 surface state.
4. For imine synthesis, CeO2 with 2 ml H2O showed 2-times higher activity than the control without H2O.
Introduction
Imine compounds are important intermediates for many biological, agricultural, and pharmaceutical compounds, such as alkaloids, membered heterocycles, and nitrogen heterocycles (Martin, 2009; Kaldas et al., 2017); thus, it is of great importance to develop a new synthesis approach for imine. CeO2 is a new promising catalyst for imine synthesis, which can overcome the problems caused by traditional acid/base catalysts (Tamura and Tomishige, 2015) and bring a lot of advantages, such as mild synthesis conditions and fast separation. Many research studies have focused on CeO2 application in imine synthesis (Geng et al., 2016; Long et al., 2019a; Long et al., 2019b; Cao et al., 2020; Rizzuti et al., 2020; Tamura and Tomishige, 2020), and it has been reported that CeO2 morphologies can greatly influence the catalytic activity for imine synthesis (Zhang et al., 2017; Yang et al., 2018; Zhang et al., 2018; Yang et al., 2020a; Yang et al., 2020b), which have a close relationship with the CeO2 specific surface area and Ce3+ defects.
The preparation approach can effectively change the structure of CeO2, especially using the microwave method. Microwaves can heat the target solution by interacting with the solution molecules; thus, the solution can be heated fast and uniformly, which can effectively improve the product quality (Reddy et al., 2012; Li et al., 2021; Ma et al., 2021). Besides, other energies, such as UV-light and ultrasonic force, can work together with microwaves to achieve a new CeO2 material structure via the Multifunctional Microwave Synthesis and Extraction Workstation (Yang et al., 2020a). Ultrasonic force is a kind of energy-accumulated mechanical vibration waves with thermal effect, mechanical effect, and cavitation effect (Thompson and Doraiswamy, 2000; Dalas, 2001; Shen, 2009), which can be used to accelerate the reaction rate and improve particle dispersion during material synthesis.
Microwave- or ultrasonic-assisted technology for CeO2 synthesis has developed very rapidly in recent years (Leonelli and Mason, 2010; Phuruangrat et al., 2017; Zhao et al., 2018; Mousavi-Kamazani and Ashrafi, 2020; Chen et al., 2021; Zhai et al., 2021), and it has been found that different CeO2 structures were obtained by using different energy inputs with the same solution (Yang et al., 2020a). Applying microwaves and ultrasonic waves together to the same solution can synthesize materials with a novel structure. In this way, the heat and mass can be transferred much better, and a new material structure can be achieved by changing the solution very slightly.
In this work, a series of CeO2 nanomaterials was synthesized by a combined microwave–ultrasonic method. Different CeO2 structures can be achieved only by changing the amount of deionized water in the solvent. The obtained CeO2 exhibited different catalytic activities for imine synthesis, and their structures were further characterized by XRD, IR, SEM, XPS, and BET to figure out the materials’ structure and the reason behind it.
Experimental
Synthesis of CeO2
All chemicals were provided by Adamas Reagents and used as received. The combined microwave-ultrasonic method was used to synthesis CeO2, using Ce(NO3)3·6H2O as the starting precursor, PVP as the structure directing agent, and acetic acid (HAC) as the mineralizer. The solvents were formed by ethylene glycol (EG) and DI-H2O, with a fixed total volume of 35.0 ml. The synthesis procedures were all the same; only the solvent content was changed by using different amounts of H2O, as shown in Table 1. The typical synthesis procedure was described using CeO2-H2 as an example. Ce(NO3)3·6H2O (2.17 g) was dissolved into the mixed solvent (33 ml EG + 2 ml DI-H2O) in a three-neck flask; then PVP (1.00 g) was added, and the resultant solution was stirred for 2 h. Finally, HAC (2.0 ml) was dropwise added, and the solution was further stirred for 15 min. Then the three-neck flask was transferred to a Multifunctional Microwave Synthesis and Extraction Workstation [Uwave-2000 from SINEO Microwave Chemistry Technology (China) Co. Ltd.] using microwave and ultrasonic force as the energy input, as shown in Figure 1A. The heating program is shown in Figure 1B, where the temperature is maintained at 100°C for 5 min and at 180°C for 12 min. After cooling naturally, the solids were centrifuged, washed by ethanol and DI-H2O four times, and dried in an oven (80°C) overnight. After that, they were calcined at 500°C (2°C/min) for 1 h in air to remove surface organic residues. In the sample name CeO2-Hx, x is the volume of DI-H2O added to the solvent.
Structure Characterization
The XRD patterns were acquired using a Shimadzu (Japan) D/Max-2500 diffractometer (nickel-filtered CuKα radiation). The morphologies of the CeO2 samples were observed using a scanning electron microscope (SEM, Hitachi S-8000, Japan) in the secondary electron scattering mode at 5 kV. N2 adsorption/desorption was measured at 77 K by using a Micromeritics ASAP 2020 instrument, and all samples were degassed at 120°C for at least 5 h before testing. X-ray photoelectron spectra (XPS) were recorded using a Thermo Fisher ESCALAB 250 xi (England) using AlKα radiation (1,486.6 eV).
CeO2 as Catalyst for Imine Synthesis
Calcined CeO2 (50 mg) was added to a mixture of benzyl alcohol (10 mmoL) and aniline (20 mmoL). The resulting mixtures were stirred vigorously at 500 rpm at 50°C. After a certain time (at least 2 h), the imine product was analyzed using HPLC (Shimadzu LC-20AT, Japan) equipped with a Hypersil ODS C18 column (5 μm in a size of 4.6 mm × 250 mm), and the mobile phase was methanol: water = 8:2. In order to compare with the other reactions, the reaction rates are given as the amount of imine formation per hour per catalyst mass (mmol h−1 g−1).
Results and Discussions
The x-ray diffraction (XRD) patterns of all samples are shown in Figure 2A. Typical CeO2 crystalline phases (JCPDF No: 34-0394) appeared for all samples, which were prepared by the combined microwave–ultrasonic method and calcined at 500°C in air for 1 h, similar to the one reported before (Yang et al., 2021), but the crystalline particle sizes were different when calculated by Scherrer equations based on the strongest (111) peak at 28.5°. The crystalline particle size changed in the range of 6.6–10.8 nm, which has a close relationship with the DI-H2O amount in the solvent. The CeO2-H0 sample, prepared without extra DI-H2O, possessed the biggest crystalline particle size of 10.8 nm. By adding extra DI-H2O to the precursor solution, the crystalline particle size decreased first (1–3 ml DI-H2O) and then increased again (4–5 ml DI-H2O). The smallest crystalline particle (6.6 nm) appeared when the extra DI-H2O was 3 ml (CeO2-H3).
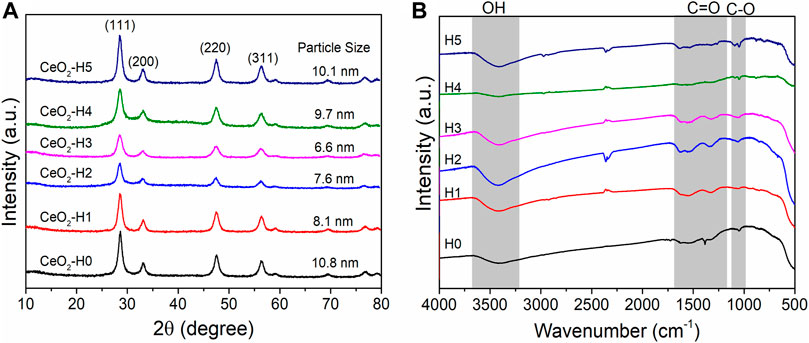
FIGURE 2. XRD (A) and IR (B) spectra of CeO2 by the combined microwave–ultrasonic method and calcined at 500°C in air for 1 h. The particle sizes in (A) are calculated by Scherrer equations based on the strongest peak at 28.5°.
The groups on the particle surfaces were characterized by FT-IR (Figure 2B). After calcination at 500°C for 1 h, most of the organic groups were removed, and only a small amount of C=O (1,300–1,650 cm−1) and C-O (1,060 cm−1) groups can be observed besides the OH group at c.a. 3,500 cm−1 (Guo et al., 2021), indicating a relatively clean surface. These groups may be caused by the adsorption of CO2 and H2O from the air, which can be further confirmed by XPS results in Figure 5.
The DI-H2O amount in the solvent can influence the morphologies of CeO2 particles, as shown in Figure 3. When there was no extra H2O in the solution, the CeO2-H0 sample (Figure 3A) exhibited an octahedral shape (diameter: 280 nm, length: 460 nm, and aspect ratio: 1.6), formed by aggregation of nanoparticles. The CeO2-H1 sample (Figure 3B), with 1 ml extra DI-H2O in the solution, also showed a roughly octahedron structure, much more like a shuttle shape (diameter: 180 nm, length: 380 nm, and aspect ratio: 2), and small particles became more obvious in the aggregate. CeO2-H2 (Figure 3C) possessed a similar structure to CeO2-H1; only the diameter became smaller, which was about 140 nm. When increasing H2O to 3 ml, CeO2-H3 (Figure 3D) showed a smaller aggregation structure, with a diameter of 80 nm and length of 200 nm (aspect ratio: 2.5). By further increasing H2O to 4 ml, the size of the aggregate in CeO2-H4 (Figure 3E) increased again, exhibiting a structure similar to that of samples CeO2-H1 and CeO2-H2. Nevertheless, the octahedron/shuttle shape collapsed for CeO2-H5 (Figure 3F); most were small particles, and only few aggregates can be observed. CeO2 shuttles were previously synthesized by the hydrothermal method under similar conditions (Guo et al., 2008), but the size (diameter: 800 nm) was much larger than that of the samples in this work.
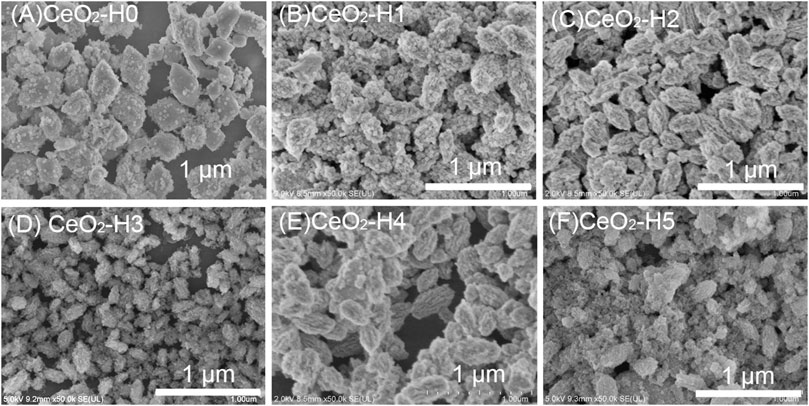
FIGURE 3. SEM morphologies of CeO2 prepared by the combined microwave–ultrasonic method and calcined at 500°C in air for 1 h. (A) CeO2-H0, (B) CeO2-H1, (C) CeO2-H2, (D) CeO2-H3, (E) CeO2-H4, (F) CeO2-H5.
The N2 adsorption–desorption isotherm is used to characterize the materials’ surface area, as shown in Figure 4A, and the pore diameter distribution is shown in Figure 4B. According to the IUPAC classification (Sing et al., 1985; Yang et al., 2020c; Zhang et al., 2021), the hysteresis loop of the N2 adsorption isotherm belonged to type VI, indicating that the materials were mesoporous. The specific surface area of CeO2-H2 (SBET: 111.3 m2/g) was similar to that of CeO2-H1 (SBET: 111.4 m2/g), larger than the values of other samples, while the minimum SBET (64.4 m2/g) belonged to CeO2-H5. Besides the surface area, the H2O amount also influenced the pore size distribution, as shown in Figure 4B. When there was no additional H2O added (sample CeO2-H0), the pore size distribution was relatively narrow, and almost all pore sizes were below 10 nm. When H2O was added to the system, the pore size distribution became broader, especially for sample CeO2-H2. This confirmed that adding H2O can influence the samples’ morphologies. This may be related to the change in the PVP micelle structure. The extra H2O addition to the synthesis system changed the arrangement of PVP, resulting in the agglomeration alternation in CeO2 particles, and the shape of CeO2 became irregular along with H2O addition, as shown in Figure 3 and illustrated in Figure 6. The agglomeration variations in CeO2 particles presented a different surface area and pore size distribution.
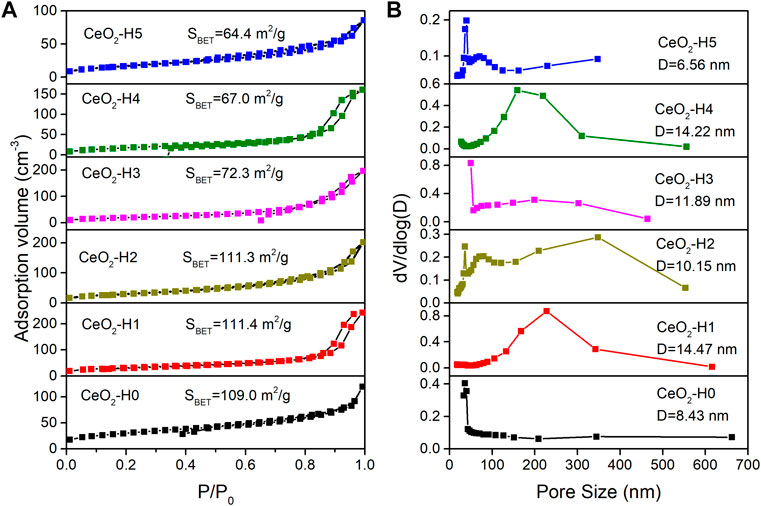
FIGURE 4. N2 sorption isotherm (A) and pore size distribution (B) of CeO2 prepared by the combined microwave–ultrasonic method and calcined at 500°C in air for 1 h.
XPS is used to characterize the CeO2 surface states (Figure 5). Ce3+ defects are important and have a close relationship with the catalytic activity of CeO2. In the Ce3d region (Figure 5A), Ce3+ ratios were calculated using the previous method, by fitting the area of Ce3+ and Ce4+ peaks (Zhang et al., 2017; Yang et al., 2018; Yang et al., 2020a). The CeO2-H0 sample had a Ce3+ ratio of 24.2%. The addition of H2O increased the Ce3+ ratio first and then decreased the number of Ce3+ defects. The highest Ce3+ ratio (32.0%) belonged to the CeO2-H3 sample, with 3 ml H2O added to the synthesizing system. CeO2-H2 possessed a Ce3+ ratio of 28.1%, which was the second top of all Ce3+ ratios. Besides, the O1s region can be fitted into three peaks: OH*/CO32−, O22−, and O2− (Chen et al., 2013). OH*/CO32− and O22− were normally caused by the organic residuals or the surface impurity caused by CO2 and H2O adsorption, and they can block the active sites of CeO2 (Ferreira et al., 2012; Jiang et al., 2015; Yang et al., 2018). Thus, the higher the O2− ratio is, the cleaner the CeO2 surface is, indicating more active sites can be exposed. From the O1s region in Figure 5B, it can be seen that the CeO2-H2 sample possessed the highest O2− ratio (68%), suggesting that it has the cleanest surface, which is beneficial to the catalytic activity. The highest O2− ratio of CeO2-H2 may be because a suitable amount of H2O promoted the cleavage of organic residuals, as illustrated in Figure 6. This may compensate the slightly lower Ce3+ ratio of the CeO2-H2 sample, resulting in the highest catalytic activity for imine synthesis, as shown in Figure 7.
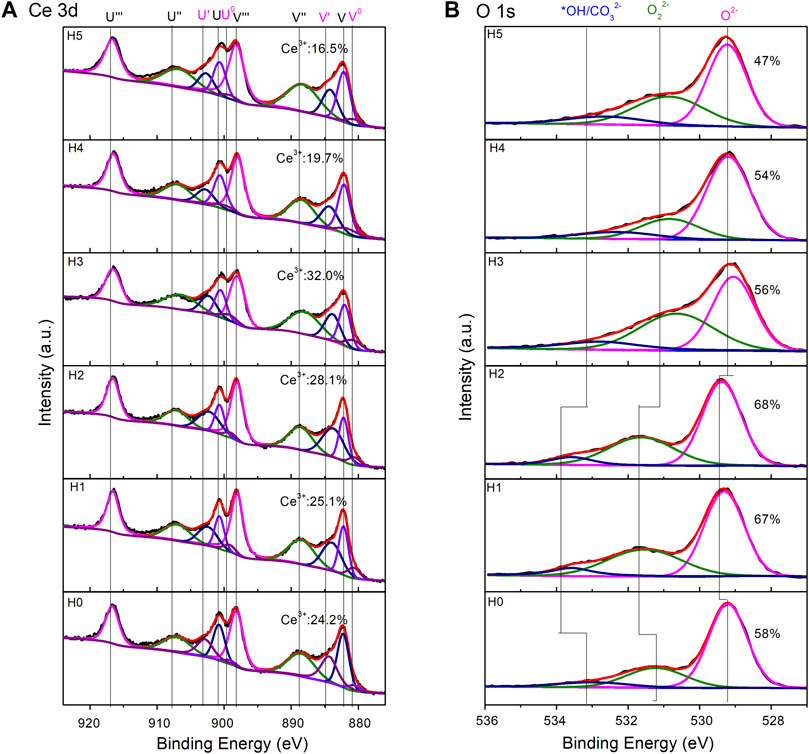
FIGURE 5. XP spectra of CeO2 by the combined microwave–ultrasonic method and calcined at 500°C in air for 1 h. (A) is the region of Ce 3d, in which V0, V′, U0, and U′ belong to Ce3+. (B) is the region of O 1 s.
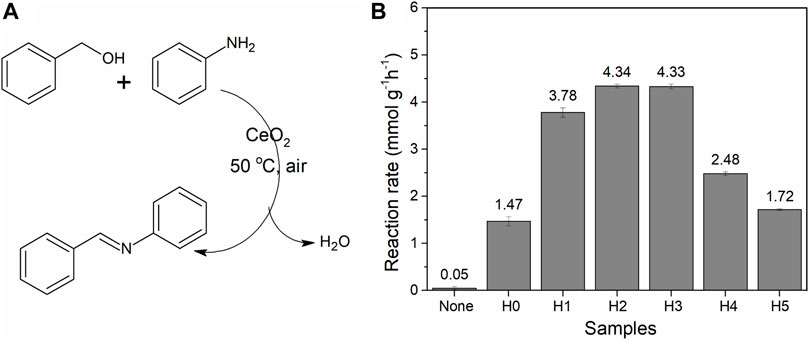
FIGURE 7. (A) Reaction for imine synthesis. (B) Imine conversion of CeO2 (50 mg) synthesized in different amounts of water (amount of catalyst per hour; all values of conversion are calculated below 20%). The experiment was repeated three times, and the average conversion value was given with an error bar.
From the above-mentioned characterization, the addition of H2O can change the morphology of CeO2, which can further alter the specific surface area and the surface Ce3+ defects. These changes may be related to the PVP micelle structure change and the CeO2 surface coordination state induced by H2O, as sketched in Figure 6. When no H2O is added to the system, the CeO2 surface is covered with EG molecules, and the surface is hydrophobic. Thus, the PVP formed a micelle structure, with the hydrophobic ends toward the CeO2 agglomeration particles. When H2O is added to the system, it can react with the Ce-precursor, forming a Ce-OH structure; thus, the CeO2 surface transformed from hydrophobic to hydrophilic, and the PVP formed a micelle structure, with the hydrophilic ends toward to the CeO2 particles. Besides, Ce-OH can also yield a cleaner surface, and the condensation between -OH can happen to yield oxygen vacancies and H2O, yielding a higher Ce3+ ratio. Meanwhile, H2O can also be adsorbed onto the CeO2 surface, which can interact with the microwave and sonic energy much better than EG can, causing more wrinkles on the CeO2 particles to increase the specific surface area. However, when more than 4 ml of H2O was added into the system, the PVP micelle structure was destroyed, and no shuttle structure can be observed.
When synthesized CeO2 was used for imine synthesis, they exhibit different activities (Figure 7). The order of imine conversion is CeO2-H2 (4.34 mmol g−1 h−1) ≈ CeO2-H3 (4.33 mmol g−1 h−1) > CeO2-H1 (3.78 mmol g−1 h−1) > CeO2-H4 (2.48 mmol g−1 h−1) > CeO2-H5 (1.72 mmol g−1 h−1) > CeO2-H0 (1.47 mmol g−1 h−1). The reaction rates of all the samples were far better than the 0.46 mmol g−1 h−1 reported in Angew (Tamura and Tomishige, 2015). It has been reported earlier that CeO2 can be used as an easily separable catalyst for imine synthesis at low temperature (<100°C) (Zhang et al., 2017; Zhang et al., 2018; Chen et al., 2020; Wu et al., 2021) and oxygen vacancies (Ce3+ defects) played an important role during this reaction. The Ce3+ vacancies can promote the transition of benzyl alcohol to benzaldehyde, and the latter can easily couple with aniline to form an imine compound (Tamura and Tomishige, 2015; Zhang et al., 2017; Qin et al., 2019). From the above-mentioned results, it can be seen that CeO2-H2 possessed the largest surface area (111.4 m2/g) and a relatively high Ce3+ ratio (28.1%), which contributed to the highest imine reaction rate. CeO2-H3 exhibited a similar catalytic activity, which may be due to its highest Ce3+ ratio (32.0%), but its surface area (72.3 m2/g) is a little bit lower than that of CeO2-H2. The results confirmed that both surface area and Ce3+ ratio are important to the CeO2 catalytic properties for imine synthesis.
Conclusion
Different sizes of fusiform CeO2 were synthesized by the combined microwave–ultrasonic method, and H2O was added to change the structure of CeO2. By changing the amount of H2O from 1 to 5 ml, the specific surface area of CeO2 changed from 111 m2/g to 64 m2/g, while the Ce3+ ratio varied in the range of 16.5% and 32.0%. The sample with 2 ml H2O has the largest surface area (111.4 m2/g) and a relatively high Ce3+ ratio (28.1%), indicating that the CeO2-H2 sample has the largest amount of exposed surface oxygen vacancies, and the imine reaction rate is 4.34 mmol g−1 h−1, which is almost three times the rate (1.47 mmol g−1 h−1) produced by the sample without addition of water.
Data Availability Statement
The original contributions presented in the study are included in the article/Supplementary Material; further inquiries can be directed to the corresponding author.
Author Contributions
XC and JY conceived this work, HD collected the data. XC wrote the original manuscript, and JY reviewed and edited the manuscript.
Funding
This research was supported by the National Natural Science Foundation of China (Grant No. 12175035).
Conflict of Interest
The authors declare that the research was conducted in the absence of any commercial or financial relationships that could be construed as a potential conflict of interest.
Publisher’s Note
All claims expressed in this article are solely those of the authors and do not necessarily represent those of their affiliated organizations, or those of the publisher, the editors, and the reviewers. Any product that may be evaluated in this article, or claim that may be made by its manufacturer, is not guaranteed or endorsed by the publisher.
References
Cao, X., Qin, J., Gou, G., Li, J., Wu, W., Luo, S., et al. (2020). Continuous Solvent-free Synthesis of Imines over Uip-γ-Al2O3-CeO2 Catalyst in a Fixed Bed Reactor. Appl. Catal. B Environ. 272, 118958. doi:10.1016/j.apcatb.2020.118958
Chen, B., Ma, Y., Ding, L., Xu, L., Wu, Z., Yuan, Q., et al. (2013). Reactivity of Hydroxyls and Water on a CeO2(111) Thin Film Surface: The Role of Oxygen Vacancy. J. Phys. Chem. C 117, 5800–5810. doi:10.1021/jp312406f
Chen, H., Shen, J., and Zhang, Y. (2021). Nanocomposite Synthesis of MoS2/nano-CeO2 for High-Performance Electromagnetic Absorption. J. Mat. Sci. Mat. Electron. 32, 22689–22698. doi:10.1007/s10854-021-06755-z
Chen, L.-Y., Xu, F.-F., Zhang, J., Ding, H., and Yang, J. (2020). Structure Design of CeO2-MoS2 Composites and Their Efficient Activity for Imine Synthesis. Appl. Nanosci. 10, 233–241. doi:10.1007/s13204-019-01114-1
Dalas, E. (2001). The Effect of Ultrasonic Field on Calcium Carbonate Scale Formation. J. Cryst. Growth 222, 287–292. doi:10.1016/S0022-0248(00)00895-2
Ferreira, V. J., Tavares, P., Figueiredo, J. L., and Faria, J. L. (2012). Effect of Mg, Ca, and Sr on CeO2 Based Catalysts for the Oxidative Coupling of Methane: Investigation on the Oxygen Species Responsible for Catalytic Performance. Ind. Eng. Chem. Res. 51, 10535–10541. doi:10.1021/ie3001953
Geng, L., Song, J., Zhou, Y., Xie, Y., Huang, J., Zhang, W., et al. (2016). CeO2 Nanorods Anchored on Mesoporous Carbon as an Efficient Catalyst for Imine Synthesis. Chem. Commun. 52, 13495–13498. doi:10.1039/c6cc05496j
Guo, L., Yang, J., Zhang, H., Wang, R., Xu, J., and Wang, J. (2021). Highly Enhanced Visible‐light Photocatalytic Activity via a Novel Surface Structure of CeO 2 /g−C 3 N 4 toward Removal of 2,4‐dichlorophenol and Cr(VI). ChemCatChem 13, 2034–2044. doi:10.1002/cctc.202001939
Guo, Z., Du, F., Li, G., and Cui, Z. (2008). Synthesis of Single-Crystalline CeCO3OH with Shuttle Morphology and Their Thermal Conversion to CeO2. Cryst. Growth & Des. 8, 2674–2677. doi:10.1021/cg070556z
Jiang, D., Wang, W., Zhang, L., Qiu, R., Sun, S., and Zheng, Y. (2015). A Strategy for Improving Deactivation of Catalytic Combustion at Low Temperature via Synergistic Photocatalysis. Appl. Catal. B Environ. 165, 399–407. doi:10.1016/j.apcatb.2014.10.040
Kaldas, S. J., O'keefe, K. T. V., Mendoza-Sanchez, R., and Yudin, A. K. (2017). Amphoteric Borylketenimines: Versatile Intermediates in the Synthesis of Borylated Heterocycles. Chem. Eur. J. 23, 9711–9715. doi:10.1002/chem.201702008
Leonelli, C., and Mason, T. J. (2010). Microwave and Ultrasonic Processing: Now a Realistic Option for Industry. Chem. Eng. Process. Process Intensif. 49, 885–900. doi:10.1016/j.cep.2010.05.006
Li, Y., Lu, Y.-L., Wu, K.-D., Zhang, D.-Z., Debliquy, M., and Zhang, C. (2021). Microwave-Assisted Hydrothermal Synthesis of Copper Oxide-Based Gas-Sensitive Nanostructures. Rare Met. 40, 1477–1493. doi:10.1007/s12598-020-01557-4
Long, Y., Gao, Z., Qin, J., Wang, P., Wu, W., Zhang, L., et al. (2019a). CeO2 Immobilized on Magnetic Core-Shell Microparticles for One-Pot Synthesis of Imines from Benzyl Alcohols and Anilines: Support Effects for Activity and Stability. J. Colloid Interface Sci. 538, 709–719. doi:10.1016/j.jcis.2018.11.092
Long, Y., Zhang, H., Gao, Z., Qin, J., Pan, Y., Zhao, J., et al. (2019b). A Protective Roasting Strategy for Preparation of Stable Mesoporous Hollow CeO2 Microspheres with Enhanced Catalytic Activity for One-Pot Synthesis of Imines from Benzyl Alcohols and Anilines. Inorg. Chem. Front. 6, 829–836. doi:10.1039/c9qi00024k
Ma, X., Zhang, X.-Y., Yang, M., Martin, S. F., Xie, J.-Y., Lv, R.-Q., and Chai, Y.-M. (2021). High-Pressure Microwave-Assisted Synthesis of WSx/Ni9S8/NF Hetero-Catalyst for Efficient Oxygen Evolution Reaction. Rare Met. 40, 1048–1055. doi:10.1007/s12598-020-01704-x
Martin, S. F. (2009). Recent Applications of Imines as Key Intermediates in the Synthesis of Alkaloids and Novel Nitrogen Heterocycles. Pure Appl. Chem. 81, 195–204. doi:10.1351/PAC-CON-08-07-03
Mousavi-Kamazani, M., and Ashrafi, S. (2020). Single-step Sonochemical Synthesis of Cu2O-CeO2 Nanocomposites with Enhanced Photocatalytic Oxidative Desulfurization. Ultrason. Sonochemistry 63, 104948. doi:10.1016/j.ultsonch.2019.104948
Phuruangrat, A., Thongtem, S., and Thongtem, T. (2017). Microwave-assisted Hydrothermal Synthesis and Characterization of CeO2 Nanowires for Using as a Photocatalytic Material. Mater. Lett. 196, 61–63. doi:10.1016/j.matlet.2017.03.013
Qin, J., Long, Y., Wu, W., Zhang, W., Gao, Z., and Ma, J. (2019). Amorphous Fe2O3 Improved [O] Transfer Cycle of Ce4+/Ce3+ in CeO2 for Atom Economy Synthesis of Imines at Low Temperature. J. Catal. 371, 161–174. doi:10.1016/j.jcat.2019.01.032
Reddy, L. H., Reddy, G. K., Devaiah, D., and Reddy, B. M. (2012). A Rapid Microwave-Assisted Solution Combustion Synthesis of CuO Promoted CeO2-MxOy (M=Zr, La, Pr and Sm) Catalysts for CO Oxidation. Appl. Catal. A General 445-446, 297–305. doi:10.1016/j.apcata.2012.08.024
Rizzuti, A., Dipalo, M. C., Allegretta, I., Terzano, R., Cioffi, N., Mastrorilli, P., et al. (2020). Microwave-Assisted Solvothermal Synthesis of Fe3O4/CeO2 Nanocomposites and Their Catalytic Activity in the Imine Formation from Benzyl Alcohol and Aniline. Catalysts 10, 1325. doi:10.3390/catal10111325
Shen, X.-F. (2009). Combining Microwave and Ultrasound Irradiation for Rapid Synthesis of Nanowires: a Case Study on Pb(OH)Br. J. Chem. Technol. Biotechnol. 84, 1811–1817. doi:10.1002/jctb.2250
Sing, K. S. W., Everett, D. H., Haul, R. a. W., and Moscou, L. R. A. (1985). Reporting Physisorption Data for Gas/solid Systems with Special Reference to the Determination of Surface Area and Porosity (Recommendations 1984). Pure Appl. Chem. 57, 603–619. doi:10.1351/pac19825411220110.1351/pac198557040603
Tamura, M., and Tomishige, K. (2015). Redox Properties of CeO2at Low Temperature: The Direct Synthesis of Imines from Alcohol and Amine. Angew. Chem. Int. Ed. 54, 864–867. doi:10.1002/anie.201409601
Tamura, M., and Tomishige, K. (2020). Scope and Reaction Mechanism of CeO2-Catalyzed One-Pot Imine Synthesis from Alcohols and Amines. J. Catal. 389, 285–296. doi:10.1016/j.jcat.2020.05.031
Thompson, L. H., and Doraiswamy, L. K. (2000). The Rate Enhancing Effect of Ultrasound by Inducing Supersaturation in a Solid-Liquid System. Chem. Eng. Sci. 55, 3085–3090. doi:10.1016/S0009-2509(99)00481-9
Wu, S., Wang, Y., Cao, Q., Zhao, Q., and Fang, W. (2021). Efficient Imine Formation by Oxidative Coupling at Low Temperature Catalyzed by High‐Surface‐Area Mesoporous CeO 2 with Exceptional Redox Property. Chem. Eur. J. 27, 3019–3028. doi:10.1002/chem.202003915
Yang, J., Ding, H., Wang, J., Yigit, N., Xu, J., Rupprechter, G., et al. (2020a). Energy-Guided Shape Control towards Highly Active CeO2. Top. Catal. 63, 1743–1753. doi:10.1007/s11244-020-01357-1
Yang, J., Ding, H., Zhu, Z., Wang, Q., Wang, J., Xu, J., et al. (2018). Surface Modification of CeO2 Nanoflakes by Low Temperature Plasma Treatment to Enhance Imine Yield: Influences of Different Plasma Atmospheres. Appl. Surf. Sci. 454, 173–180. doi:10.1016/j.apsusc.2018.05.135
Yang, J., Peng, S., Shi, Y., Ma, S., Ding, H., Rupprechter, G., et al. (2020b). Fast Visual Evaluation of the Catalytic Activity of CeO2: Simple Colorimetric Assay Using 3,3′,5,5′-tetramethylbenzidine as Indicator. J. Catal. 389, 71–77. doi:10.1016/j.jcat.2020.05.016
Yang, J., Yigit, N., Möller, J., and Rupprechter, G. (2021). Co 3 O 4 −CeO 2 Nanocomposites for Low‐Temperature CO Oxidation. Chem. A Eur. J. 27, 16947–16955. doi:10.1002/chem.202100927
Yang, J., Zhang, J., Zou, B., Zhang, H., Wang, J., Schubert, U., et al. (2020c). Black SnO2-TiO2 Nanocomposites with High Dispersion for Photocatalytic and Photovoltalic Applications. ACS Appl. Nano Mat. 3, 4265–4273. doi:10.1021/acsanm.0c00432
Zhai, Y., Xin, G., Wang, J., Zhang, B., Song, J., and Liu, X. (2021). Microwave-assisted Synthesis of rGO/CeO2 Supercapacitor Electrode Materials with Excellent Electrochemical Properties. Acta Chim. Sin. 79, 1129–1137. doi:10.6023/a21050216
Zhang, H., Wu, C., Wang, W., Bu, J., Zhou, F., Zhang, B., et al. (2018). Effect of Ceria on Redox-Catalytic Property in Mild Condition: A Solvent-free Route for Imine Synthesis at Low Temperature. Appl. Catal. B Environ. 227, 209–217. doi:10.1016/j.apcatb.2018.01.012
Zhang, H., Yang, J., Guo, L., Wang, R., Peng, S., Wang, J., et al. (2021). Microwave-aided Synthesis of BiOI/g-C3n4 Composites and Their Enhanced Catalytic Activities for Cr(VI) Removal. Chem. Phys. Lett. 762, 138143. doi:10.1016/j.cplett.2020.138143
Zhang, J., Yang, J., Wang, J., Ding, H., Liu, Q., Schubert, U., et al. (2017). Surface Oxygen Vacancies Dominated CeO2 as Efficient Catalyst for Imine Synthesis: Influences of Different Cerium Precursors. Mol. Catal. 443, 131–138. doi:10.1016/j.mcat.2017.09.030
Keywords: CeO2, catalyst, imine synthesis, microwave synthesis, microwave-ultrasonic combined method
Citation: Chang X, Ding H and Yang J (2022) CeO2 Structure Adjustment by H2O via the Microwave–Ultrasonic Method and Its Application in Imine Catalysis. Front. Chem. 10:916092. doi: 10.3389/fchem.2022.916092
Received: 08 April 2022; Accepted: 25 April 2022;
Published: 31 May 2022.
Edited by:
Xijun Wei, Southwest University of Science and Technology, ChinaReviewed by:
Yi Liu, Zhejiang University, ChinaShifei Kang, University of Shanghai for Science and Technology, China
Copyright © 2022 Chang, Ding and Yang. This is an open-access article distributed under the terms of the Creative Commons Attribution License (CC BY). The use, distribution or reproduction in other forums is permitted, provided the original author(s) and the copyright owner(s) are credited and that the original publication in this journal is cited, in accordance with accepted academic practice. No use, distribution or reproduction is permitted which does not comply with these terms.
*Correspondence: Jingxia Yang, yjx09tj@foxmail.com, jxyang@sues.edu.cn