- 1School of Pharmacy, State Key Laboratory of Applied Organic Chemistry, Lanzhou University, Lanzhou, China
- 2Translational Medicine Center, Zhengzhou Central Hospital Affiliated to Zhengzhou University, Zhengzhou, China
- 3Institutional Center for Shared Technologies and Facilities, Institute of Microbiology, Chinese Academy of Sciences, Beijing, China
- 4State Key Laboratory of Mycology, Institute of Microbiology, Chinese Academy of Sciences, Beijing, China
One novel diterpenoid lactone named caesalpinbondin A (1) that possesses an unprecedented tetracyclic ring system in which a 6/6/5-fused tricyclic ring and a 4,5-dimethyldihydrofuran-2(3H)-one were connected by a C-C single bond comprising a 5-(naphtho [2,3-b]furan-7-yl)dihydrofuran-2(3H)-one moiety was isolated from the seeds of Caesalpinia bonduc. Its chemical structure was established by extensive spectroscopic methods, and its absolute configuration was further determined by single-crystal X-ray diffraction analysis and electronic circular dichroism calculation. The biological evaluation suggested that compound 1 demonstrated potent anti-Alzheimer’s disease (AD) bioactivity, which could delay paralysis of transgenic AD Caenorhabditis elegans. A possible biogenetic pathway of 1 was also proposed.
Introduction
Between 1981 and 2019, approximately 50% of 1,881 new drugs approved worldwide were produced using or inspired by natural products or derivatives (Newman and Cragg, 2020). In particular, natural products, which are derived from medicinal plants with new types of architectures and amazing bioactivities, have been a rich source of drug leads and candidates.
The genus Caesalpinia, belonging to the Fabaceae family, comprises approximately 100 different kinds of plants that are mainly distributed in tropical and subtropical regions including China, Thailand, Vietnam, and other countries (Qiao et al., 2016). There are 17 Caesalpinia species in China, most of which are found in the southern regions (Wei et al., 2016). Many plants of the Caesalpinia genus have been used as a traditional medicine to treat multiple diseases in many countries all over the world (Wu et al., 2011). The chemical constituents from this genus have been reported to be terpenoids, flavonoids, steroids, lignans, phenylpropanoids, and alkaloids (Wu et al., 2011; Zanin et al., 2012). Among these constituents, the cassane-type diterpenoids, the main and characteristic chemical components of Caesalpinia, have been studied widely due to their bioactivities, such as anti-inflammatory, antitumor, antimalarial, antiviral, antioxidant, and antimicrobial properties (Jing et al., 2019). Furthermore, some diterpenoids with novel skeletons have been found in plants of the Caesalpina genus, such as caesalpinimin A (Zhang et al., 2014), spirocaesalmin (Jiang et al., 2001), tomocinol C, and spirocaesalmin C (Xiao et al., 2016).
Caesalpinia bonduc (Linn.) Roxb. is a famous medicinal plant that grows mainly in tropical and subtropical regions of Southeast Asia (Pudhom et al., 2007). In China, it is mainly distributed in the southern areas, such as Guangxi, Hainan, and Taiwan (Editorial Committee of Flora of China, 1998). The seeds of C. bonduc have been used as a traditional Chinese medicine (TCM) for the treatment of a variety of diseases, including common cold, fever, dysentery, epigastric pain, abdominal pain, eye swelling and pain, and sores (Jiangsu New Medical College, 1977; Editorial Board of Chinese Materia Medica, 1999). Extracts or constituents of C. bonduc have been reported to possess cytotoxicity (Wu et al., 2014), antiproliferative (Yadav et al., 2009), anti-inflammatory (Liu et al., 2020), antistress (Kannur et al., 2006), antimicrobial (Simin et al., 2001; Arif et al., 2009), and anti-insecticidal effects (Essoung et al., 2020). Previous phytochemical investigations of C. bonduc have demonstrated the presence of cassane-type diterpenoids (Wu et al., 2014; Zhang et al., 2016; Liu et al., 2020; Cao et al., 2021), sterols (Udenigwe et al., 2007), and flavonoids (Ata et al., 2009). Among these chemical constituents, cassane-type diterpenoids were found to be the most important components and were considered to be active constituents of C. bonduc.
In our continuing search for structurally novel and biologically interesting natural products of Chinese medicinal plants (Fei et al., 2016; Li et al., 2017; Wu et al., 2018; Zhang et al., 2018; Yu et al., 2020; Li et al., 2022), a novel diterpenoid, caesalpinbondin A (1) (Figure 1), was isolated from the 95% ethanol extract of the seeds of C. bonduc. Compound 1 represented a novel tetracyclic ring system in which a 6/6/5-fused tricyclic ring and a 4,5-dimethyldihydrofuran-2(3H)-one moiety were connected by a C-C single bond comprising an unprecedented 5-(naphtho [2,3-b]furan-7-yl)dihydrofuran-2(3H)-one scaffold. We present the isolation, structure and stereochemistry elucidation, plausible biosynthetic pathway, and anti-AD activity of 1.
Results and Discussion
The 95% EtOH extract of the seeds of C. bonduc was partitioned by successive extraction with EtOAc and n-BuOH. The EtOAc-soluble fraction was separated by repeated column chromatography on silica gel and Sephadex LH-20 to afford compound 1.
Compound 1 was obtained as colorless needle-like crystals with [α]20D +14.0 (c 0.10, CHCl3). Its IR spectrum showed absorption bands assignable to carbonyl (1773 cm−1) and aromatic ring (1,607 and 1,500 cm−1) functionalities. The molecular formula of C20H20O3, with 11 degrees of unsaturation, was deduced from HRESIMS at m/z 331.1310 [M + Na]+ (calcd for C20H20O3Na, 331.1305), which was supported by the NMR data (Table 1). The 1H NMR spectrum displayed signals of five aromatic protons at δH 7.60 (1H, d, J = 9.6 Hz, H-6), 8.00 (1H, d, J = 9.6 Hz, H-7), 8.05 (1H, s, H-11), 6.94 (1H, d, J = 2.4 Hz, H-15), and 7.72 (1H, d, J = 2.4 Hz, H-16); two benzylic angular methyls at δH 2.87 (3H, s, H-17) and 2.79 (3H, s, H-20); one secondary methyl at δH 1.32 (3H, d, J = 7.2 Hz, H-19); and one tertiary methyl at δH 1.82 (3H, s, H-18). In addition, the 1H NMR spectrum showed resonances due to one aliphatic methine at δH 3.08 (1H, m, H-3) and one aliphatic methylene at δH 2.18 (1H, dd, J = 18.0, 3.0 Hz, H-2a) and 2.59 (1H, dd, J = 18.0, 3.0 Hz, H-2b). The 13C and DEPT NMR spectra revealed 20 resonances ascribed to four methyls, one methylene, six methines (five olefinic and one aliphatic), and nine quaternary carbons (one carbonyl, seven olefinic, and one oxygenated aliphatic). Among them, one carbonyl and twelve sp2-hybridized carbons indicated seven degrees of unsaturation. These data suggested that compound 1 is a diterpenoid possessing a tetracyclic ring system in the scaffold.
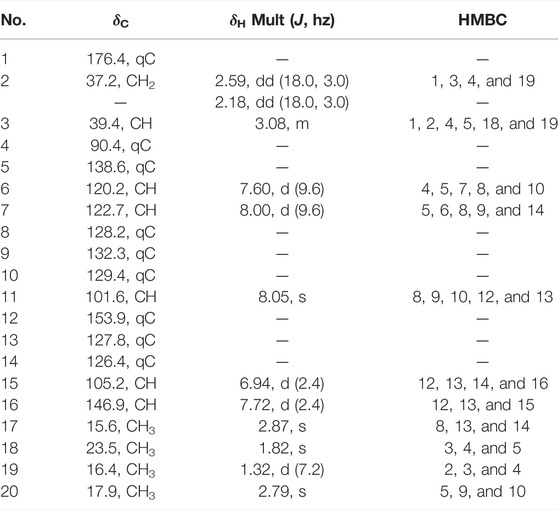
TABLE 1. 1H (600 MHz) and13C NMR (150 MHz) spectral data of compound 1 in CDCl3 (δ in ppm, J in Hz).
The planar structure of 1 was elucidated by extensive analysis of the two-dimensional (2D) NMR experiments. The proton and proton-bearing carbon resonances in the NMR spectra of 1 were assigned unambiguously by comprehensive interpretation of the 1H–1H COSY (Figure 2) and HSQC spectroscopic data. The proton spin–spin coupling system from H-6 to H-7 in the 1H–1H COSY spectrum, in combination with the HMBC correlations (Figure 2) from H3-20 to C-5, C-9, and C-10, from H-6 to C-5, C-7, C-8, and C-10, from H-7 to C-5, C-6, C-8, and C-9, demonstrated the presence of a benzene ring A with a methyl group at C-10. Moreover, the HMBC correlations of H3-17 with C-8, C-13, and C-14 and of H-11 with C-8, C-9, C-10, C-12, and C-13 indicated the existence of a benzene ring B fused with ring A through the positions between C-8 and C-9 with a methyl group at C-14. Subsequently, the 1H–1H COSY correlation of H-15 with H-16, the HMBC correlations of H-15 with C-12, C-13, C-14, and C-16, and the HMBC correlations of H-16 with C-12, C-13, and C-15, in combination with the dramatically downfield chemical shifts of C-12 (δC 153.9) and C-16 (δC 146.9), suggested the existence of a furan ring C fused with ring B through the positions between C-12 and C-13. Additionally, the 1H–1H COSY correlations of H2-2/H-3/H3-19, as well as the HMBC cross-peaks of H2-2 with C-1, C-3, and C-4, of H-3 with C-1, C-2, and C-4, and of H3-19 with C-2, C-3, and C-4, revealed that the carbonyl C-1 of 1 (δC 176.4) formed a γ-butyrolactone (ring D) with the oxygenated C-4 (downfield chemical shift at δC 90.4) to occupy the remaining one degree of unsaturation. The HMBC correlations of H3-19 with C-2, C-3, and C-4 and of H3-18 with C-3 and C-4 indicated that CH3-19 and CH3-18 were located at C-3 and C-4, respectively. Based on the HMBC correlations of H3-18 with C-5 and of H-6 with C-4, it was further apparent that the ring D and ring A were linked between C-4 and C-5 by a C-C single bond.
The relative configuration of 1 was determined by NOESY analysis. In the NOESY spectrum (Figure 3), the NOE correlation between H3-18 and H3-19 indicated that H3-18 and H3-19 were oriented on the same side of the γ-butyrolactone moiety (β-configuration). Thus, the planar structure and relative configuration of 1 were determined as shown in Figure 1. After many attempts to crystallize 1 using different solvents, a single crystal of 1 was finally obtained from CHCl3-MeOH (2:3). The subsequent X-ray crystallographic analysis (Cu Kα) clarified the planar structure and the relative configuration of 1 (Figure 4). However, the Flack parameter (-0.3 (3)) was somewhat large, precluding assignment of the absolute configuration of 1 by this method. Thus, ECD calculation was utilized to identify the absolute configuration of 1. Using quantum-mechanical time-dependent density functional theory (TDDFT) calculation, the theoretically calculated ECD spectrum of 1 (Figure 5) was in good agreement with the experimental ECD spectrum, supporting the assignment of the absolute configuration of 1 as 3 and 4R. Thus, the structure of 1 was established and named caesalpinbondin A.
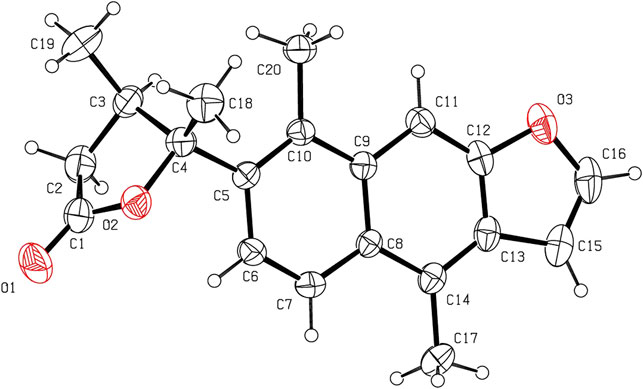
FIGURE 4. ORTEP plot of the X-ray crystallographic structure for 1 (displacement ellipsoids were drawn at the 30% probability level).
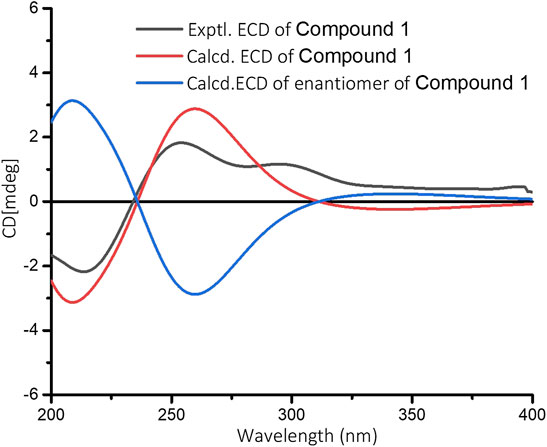
FIGURE 5. Experimental ECD spectrum of 1 and calculated ECD spectra for (3R, 4R)-1 and its enantiomer in MeOH.
Caesalpinbondin A (1) possessed an unconventional tetracyclic diterpenoid backbone, in which a 6/6/5-fused tricyclic ring system was connected to a 4,5-dimethyldihydrofuran-2(3H)-one moiety via a C-C single bond. The aforementioned analyses proved 1 as a diterpenoid with a new carbon skeleton architecture, and the name “caesalpinane” is suggested for this new skeleton type.
A hypothetical biosynthetic route was proposed, as shown in Scheme 1. Compound 1 should be originated from geranylgeranyl pyrophosphate (GGPP), which is a typical precursor of diterpenoids. First, labdadienyl pyrophosphate (LDPP) was produced from GGPP by an acid-induced intramolecular cyclization, and then the pimarane-type diterpenoid cation intermediate was generated from LDPP through further intramolecular cyclization and converted to the cassane-type diterpenoid via the migration of methyl 17 from C-13 to C-14 (Zentar et al., 2018; Jing et al., 2019). The cassane-type diterpenoid was transformed into furan-cassane diterpenoid through oxidation, nucleophilic ring closure, and dehydration reactions (Zhang et al., 2013). Next, compound A could be derived from the furan-cassane diterpenoid via oxidation reaction and further hydrogenated and dehydrated to afford a key carbocation intermediate C, which could be converted to D by the migration of methyl 19 from C-4 to C-3. Subsequent oxidation and addition of compound D may lead to the formation of compound E, which could go through Baeyer–Villiger oxidation to give compound F. Hydrolysis of compound F followed by dehydrated and intramolecular esterification reaction could produce compound I, which would give compound 1 by an enzyme-mediated aromatization.
Furthermore, the anti-Alzheimer’s disease (AD) bioactivity of compound 1 was evaluated using the transgenic AD Caenorhabditis elegans model with memantine as the positive control (Figure 6). The AD model (CL4176) is based on the transfer of the human Aβ1-42 gene downstream of the nematode myo-3 promoter. When incubated at a normal temperature of 15°C, the nematodes did not express Aβ1-42, and when the temperature was adjusted upward to 25°C, Aβ1-42 began to accumulate in the muscle cells and induced a toxic response that paralyzed the nematodes. If the number of nematode individuals in the paralyzed state was reduced in the administered group compared to the blank group, the compound was shown to have anti-AD activity. For its cheapness, easy and fast-breeding, and AD pathological similarity, the transgenic AD C. elegans model has been used as a powerful tool for rapid screening of anti-AD drugs (Xin et al., 2013). Using transgenic AD C. elegans paralysis assay, we have successfully discovered several natural products with potential anti-AD effects (Li et al., 2017; Wu et al., 2018; Su et al., 2021). As shown in Figure 6, compound 1 significantly delayed the Aβ-induced paralysis in CL4176 nematodes (p < 0.05). At 44th h of upregulated temperature induction, 33.40% of nematodes were still unparalyzed in the 100 μM compound 1-treated group, while only 22.24% of unparalyzed nematodes remained in the 0.1% DMSO-treated group. The result of the bioactivity assay indeed gave a notion that compound 1 may have the potential to be served as an anti-AD agent candidate.
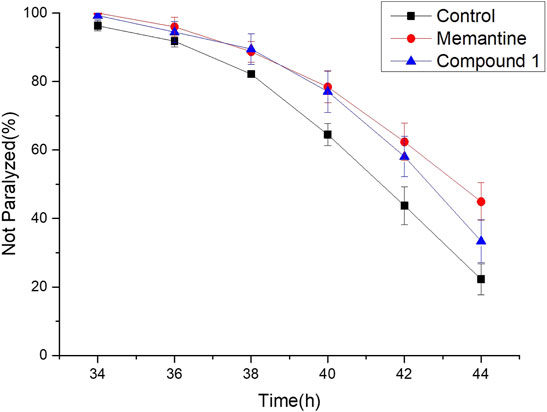
FIGURE 6. Anti-Alzheimer’s disease (AD) activity of compound 1, positive control, and control. Worms were treated with 100 μM compound 1, 100 μM memantine was regarded as the positive control, and 0.1% DMSO was regarded as the control.
Conclusion
In summary, our further exploration of the minor chemical constituents from C. bonduc yielded one new carbon skeleton diterpenoid, caesalpinbondin A (1). The novel diterpenoid 1 possessed an unprecedented 6/6/5-fused ring system with a 4,5-dimethyldihydrofuran-2(3H)-one moiety scaffold. The discovery of 1 has enriched the diversity and complexity of natural diterpenoids. In addition, compound 1 exhibited good delayed AD-like symptoms of worm paralysis phenotype activity, suggesting that this compound may be useful for the development of anti-AD drugs. These findings will attract much attention from both chemists and pharmacologists, and further research such as total synthesis and in-depth pharmacological tests are warranted.
Experimental Section
General Experimental Procedures
Melting points were measured using an X-4 digital display micromelting point apparatus (Beijing Tech Instrument Co., Ltd. Beijing, China) and are uncorrected. Optical rotations were obtained on a PerkinElmer 341 polarimeter (PerkinElmer, Waltham, MA, United States). IR spectra were run on a Nicolet NEXUS 670 FT-IR spectrometer (ThermoFisher Scientific Inc. Waltham, MA, United States). The ECD spectra were measured on an OLIS DSM1000 spectrophotometer (Olis, Inc. Bogart, GA, United States) at room temperature. The 1D and 2D NMR experiments were conducted on a Varian INOVA 600 MHz NMR spectrometer (Varian Inc. Palo Alto, CA, United States) and used TMS as the internal standard. The HRESIMS data were collected on a ThermoFisher LTQ Orbitrap Elite high-resolution mass spectrometer (Thermo Fisher Scientific Inc. Waltham, MA, United States). The X-ray crystallographic analysis was performed on an Agilent SuperNova dual-wavelength diffractometer with a microfocus X-ray source and multilayer optics monochromatized Cu Kα (λ = 1.54184 Å) radiation (Agilent Technologies, Santa Clara, CA, United States). Silica gel (200–300 mesh (Qingdao Marine Chemical Factory, Qingdao, China) and Sephadex LH-20 (GE Healthcare Amersham Biosciences, Uppsala, Sweden) for column chromatography were used to separate compounds. Silica gel GF254 (10–40 μm) used for TLC was supplied by Qingdao Marine Chemical Factory, Qingdao, China. Spots were detected on TLC under UV light or by heating after spraying with 5% H2SO4 in C2H5OH (v/v).
Plant Material
The seeds of C. bonduc were purchased in April 2014 from the Anguo Traditional Chinese Medicine Market in Hebei Province, China, and identified by Dr. Jian-yin Li of School of Pharmacy, Lanzhou University. A voucher specimen (No. 20140418CB) was deposited at the School of Pharmacy, Lanzhou University, China.
Extraction and Isolation
The air-dried and powdered seeds of C. bonduc (12 kg) were extracted with 95% EtOH (40 L × 4) at room temperature. Evaporation of the organic solvent afforded 920 g of a crude extract, which was suspended in water and successively partitioned into EtOAc and n-BuOH extracts. The EtOAc extract (300 g) was separated over a silica gel column with a petroleum ether/acetone gradient (from 40:1 to 0:1, v/v) eluent to provide seven fractions (Fr.1–Fr.7). Fraction 2 (25 g) was chromatographed over silica gel eluted with petroleum ether/EtOAc (from 30:1 to 3:1, v/v) to yield four subfractions (Fr.2A–Fr.2D). Fr.2C (900 mg) was subjected to passage over a silica gel column with petroleum ether/acetone (from 20:1 to 0:1. v/v) and then applied to Sephadex LH-20 column chromatography (CHCl3/CH3OH, 2:3, v/v) to obtain 1 (5 mg).
Physical and Chemical Data
Caesalpinbondin A (1): colorless needle-like crystals; m. p. 218–220°C [α]20D +14.0 (c 0.10, CHCl3); IR (KBr) νmax: 2,974, 2,926, 1773, 1,638, 1,607, 1,543, 1,500, 1,459, 1,385, 1,231, 1,142, 1,045, 934, 756, and 666 cm−1; 1H (600 MHz) and 13C (150 MHz) NMR data, see Table 1; HRESIMS m/z 331.1310 [M + Na]+ (calcd. for C20H20O3Na, 331.1305).
Single X-Ray Diffraction Data Analysis
Single crystals of compound 1 was obtained by recrystallization from a mixture of CHCl3 and MeOH. Crystal data for 1: C20H20O3, Mr = 308.36, orthorhombic, space group P212121, a = 6.26793 (18) Å, b = 12.7202 (4) Å, c = 20.3959 (6) Å, α = 90.00°, β = 90.00°, γ = 90.00°, V = 20.3959 (6) Å3, T = 293 (2) K, Z = 4, d = 1.260 g/cm3, μ(Cu Kα) = 0.67 mm−1, F (000) = 656, and crystal dimensions 0.24 × 0.09 × 0.07 mm were used for measurement on an Agilent Technologies SuperNova, Dual source, EOS CCD with mirror optics, using graphite monochromated Cu Kα radiation (λ = 1.54184 Å). The total of 13,844 reflections measured 3,009 independent reflections (Rint = 0.0371). The final R1 value was 0.0428 (I > 2σ (I)). The final wR (F2) value was 0.1116 ((I > 2σ(I)). The final R1 value was 0.0610 (all data). The final wR (F2) value was 0.0972 (all data). The goodness of fit on F2 was 1.031. The Flack parameter was -0.3 (3). Crystallographic data for the structure of compound 1 have been deposited with the Cambridge Crystallographic Data Centre (deposition no. CCDC 2106390). Copies of these data can be obtained free of charge via www.ccdc.cam.ac.uk/conts/retrieving.html (or from the Cambridge Crystallographic Data Centre, 12 Union Road, Cambridge CB21EZ, U.K.; fax (+44) 1223-336-033; or deposit@ccdc.cam.uk).
ECD Calculations
The conformational search for the enantiomers of all plausible stereoisomers of 1 was performed by the “random search” procedure implemented in the SYBYL-X-2.1.1 program using the Molecular Merck force field (MMFF94s). Gaussian 09 software was applied to screen stable conformers with the energy of the optimized structures at the B3LYP/6-31G(d) level (Frisch et al., 2010). The ECD curves of the conformers were determined by the TDDFT method at the B3LYP/6-31+G(d) level with the CPCM model in a methanol solution. SpecDis 1.7.1 software with UV correction was used to weigh the overall ECD curves by the Boltzmann distribution of each conformer (Bruhn et al., 2017). The calculated ECD curves of 1 were compared with the experimental results for the absolute configuration determination.
Bioactivity Assay for Alzheimer’s Disease-Like Phenotype of Delaying Paralysis in Caenorhabditis elegans
The genetically modified Caenorhabditis elegans CL4176 strain with genotype smg-1 [myo-3p:: A-Beta (1–42):: let-851 3′UTR) + rol-6 (su1006)] was purchased from the Caenorhabditis Genetics Center (CGC) (University of Minnesota, Minneapolis, MN). Using Escherichia coli OP50 as the standard food source, worms were cultured on nematode growth medium (NGM) at 16°C. A total of 60–80 eggs were placed in NGM, and 0.1% DMSO was used as a negative control for the entire biological activity determination experiment. After the larvae developed into L3 larvae, they were transferred to another 25°C incubator and incubated for 26 h. The paralyzed worms were observed and counted under a dissecting microscope every 2 h until they were all paralyzed. The non-paralysis curve was plotted by incubation time at 25°C as x-axis and non-paralytic worm percentage as y-axis throughout the experiment. The compound delaying the rate of worm paralysis more effectively means that it has higher anti-AD activity than any others. It can be identified that the non-paralysis curve of a compound with a higher anti-AD activity locates on the topside of the other inferior. The anti-AD activity was determined by using a log-rank survival test to compare the significance between treatments (Martorell et al., 2013). The p-value at a level of 0.05 or less was considered to be statistically significant.
Data Availability Statement
The original contributions presented in the study are publicly available. These data can be found at: https://www.ccdc.cam.ac.uk/, 2106390.
Author Contributions
D-QF, Z-XZ, and E-WL contributed to the conception and design of the research. H-HL, X-HC, X-QZ, and M-JW undertook the isolation, purification, and identification work. W-BC and Z-PZ performed the activity testing work. F-MQ carried out the 1D and 2D-NMR spectra data. D-QF, Z-XZ, and E-WL undertook the writing work of the manuscript. All authors contributed to the article and approved the submitted manuscript version.
Funding
This research was financially supported by the National Natural Science Foundation of China (Grant Nos. 31670350 and 31870324) and the Natural Science Foundation of Gansu Province, China (Grant Nos. 21JR7RA444 and 21JR7RA477).
Conflict of Interest
The authors declare that the research was conducted in the absence of any commercial or financial relationships that could be construed as a potential conflict of interest.
Publisher’s Note
All claims expressed in this article are solely those of the authors and do not necessarily represent those of their affiliated organizations, or those of the publisher, the editors, and the reviewers. Any product that may be evaluated in this article, or claim that may be made by its manufacturer, is not guaranteed or endorsed by the publisher.
Acknowledgments
We thank Professor Shao-hua Wang of the School of Pharmacy, Lanzhou University, for his valuable suggestions on the proposed biosynthetic pathway. We also acknowledge Yong-liang Shao of State Key Laboratory of Applied Organic Chemistry, Lanzhou University, for his professional measurements of the X-ray diffraction.
Supplementary Material
The Supplementary Material for this article can be found online at: https://www.frontiersin.org/articles/10.3389/fchem.2022.911543/full#supplementary-material
References
Arif, T., Mandal, T. K., Kumar, N., Bhosale, J. D., Hole, A., Sharma, G. L., et al. (2009). In Vitro and In Vivo Antimicrobial Activities of Seeds of Caesalpinia bonduc (Lin.) Roxb. J. Ethnopharmacol. 123, 177–180. doi:10.1016/j.jep.2009.02.040
Ata, A., Gale, E. M., and Samarasekera, R. (2009). Bioactive Chemical Constituents of Caesalpinia Bonduc (Fabaceae). Phytochem. Lett. 2, 106–109. doi:10.1016/j.phytol.2009.02.002
Bruhn, T., Schaumlöffel, A., Hemberger, Y., and Pecitelli, G. (2017). SpecDis Version 1.7.1. Berlin, Germany.
Cao, J., Xu, Y. S., Lou, R. H., Shi, W., Chen, J. L., Gan, L. S., et al. (2021). Cassane-Type Diterpenoids from the Seeds of Caesalpinia bonduc (L.)Roxb. Chem. Biodivers. 18, e2100309. doi:10.1002/cbdv.202100309
Editorial Board of Chinese Materia Medica (1999). Chinese Materia Medica, 11. Shanghai: Shanghai Science and Technology Press, 368–369.
Editorial Committee of Flora of China (1998). “Chinese Academy of Sciences,” in Flora of China. Beijing: Science Press, 42, 366.
Essoung, F. R. E., Mba'ning, B. M., Tcho, A. T., Chhabra, S. C., Mohamed, S. A., Lenta, B. N., et al. (2020). Antifeedant and Ovicidal Activities of A New Cassane and Other Compounds from Caesalpinia Welwitschiana Oliv. And Caesalpinia Bonduc L. Against Tuta Absoluta (Lepidoptera: Gelechiidae). Nat. Prod. Res. 35, 5681–5691. doi:10.1080/14786419.2020.1825424
Fei, D. Q., Dong, L. L., Qi, F. M., Fan, G. X., Li, H. H., Li, Z. Y., et al. (2016). Euphorikanin A, A Diterpenoid Lactone with A Fused 5/6/7/3 Ring System from Euphorbia Kansui. Org. Lett. 18, 2844–2847. doi:10.1021/acs.orglett.6b01093
Frisch, M. J., Trucks, G. W., Schlegel, H. B., Scuseria, G. E., Robb, M. A., Cheeseman, J. R., et al. (2010). Gaussian 09, Revision C.01. Wallingford, CT: Gaussian, Inc.
Jiang, R. W., Ma, S. C., But, P. P. H., and Mak, T. C. W. (2001). Isolation and Characterization of Spirocaesalmin, A Novel Rearranged Vouacapane Diterpenoid from Caesalpinia Minax Hance. J. Chem. Soc., Perkin Trans. 1 22, 2920–2923. doi:10.1039/b107473n
Jiangsu New Medical College (1977). Dictionary of Chinese Traditional Medicine. Shanghai: Shanghai People’s Publishing House, 1289.
Jing, W. H., Zhang, X. X., Zhou, H. X., Wang, Y., Yang, M. Q., Long, L. P., et al. (2019). Naturally Occurring Cassane Diterpenoids (CAs) of Caesalpinia: A Systematic Review of its Biosynthesis, Chemistry and Pharmacology. Fitoterapia 134, 226–249. doi:10.1016/j.fitote.2019.02.023
Kannur, D. M., Hukkeri, V., and Akki, K. S. (2006). Adaptogenic Activity of Caesalpinia Bonduc Seed Extracts in Rats. J. Ethnopharmacol. 108, 327–331. doi:10.1016/j.jep.2006.05.013
Li, Y, Q., Hou, B, L., Wang, M, J., Wang, R, Y., Chen, X. H., Liu, X., et al. (2022). Diterpenoids and C13 Nor-Isoprenoid Identified from the Leaves and Twigs of Croton Yanhuii Activating Apoptosis and Pyroptosis. Front. Chem. 10, 861278. doi:10.3389/fchem.2022.861278
Li, Z. Y., Qi, F. M., Zhi, D. J., Hu, Q. L., Liu, Y. H., Zhang, Z. X., et al. (2017). A Novel Spirocyclic Triterpenoid and A New Taraxerane Triterpenoid from Teucrium Viscidum. Org. Chem. Front. 4, 42–46. doi:10.1039/c6qo00460a
Liu, T., Wang, M., Qi, S. Z., Shen, X. Y., Wang, Y., Jing, W. H., et al. (2020). New Cassane-type Diterpenoids from Kernels of Caesalpinia Bonduc (Linn.) Roxb. And Their Inhibitory Activities on Phosphodiesterase (PDE) and Nuclear Factor-Kappa B (NF-Kappa B) Expression. Bioorg. Chem. 96, 103573. doi:10.1016/j.bioorg.2020.103573
Martorell, P., Bataller, E., Llopis, S., Gonzalez, N., Alvarez, B., Monton, F., et al. (2013). A Cocoa Peptide Protects Caenorhabditis elegans from Oxidative Stress and β-Amyloid Peptide Toxicity. PLoS One 8, e63283. doi:10.1371/journal.pone.0063283
Newman, D. J., and Cragg, G. M. (2020). Natural Products as Sources of New Drugs over the Nearly Four Decades from 01/1981 to 09/2019. J. Nat. Prod. 83, 770–803. doi:10.1021/acs.jnatprod.9b01285
Pudhom, K., Sommit, D., Suwankitti, N., and Petsom, A. (2007). Cassane Furanoditerpenoids from the Seed Kernels of Caesalpinia Bonduc from Thailand. J. Nat. Prod. 70, 1542–1544. doi:10.1021/np070330y
Qiao, Y. B., Xu, Q. Q., Hu, Z. X., Li, X. N., Xiang, M., Liu, J. J., et al. (2016). Diterpenoids of the Cassane Type from. Caesalpinia decapetala. J. Nat. Prod. 79, 3134–3142. doi:10.1021/acs.jnatprod.6b00910
Simin, K., Khaliq-uz-Zaman, S. M., and Ahmad, V. U. (2001). Antimicrobial Activity of Seed Extracts and Bondenolide from Caesalpinia bonduc (L.) Roxb. Phytother. Res. 15, 437–440. doi:10.1002/ptr.756
Su, P. J., Zhang, Z. P., Cui, W. B., Liu, X., Wang, R. Y., Zhi, D. J., et al. (2021). Polyoxygenated Sesquiterpenoids from Salvia Castanea and Their Potential Anti-alzheime's Disease Bioactivities. Fitoterapia 151, 104867. doi:10.1016/j.fitote.2021.104867
Udenigwe, C. C., Ata, A., and Samarasekera, R. (2007). Glutathione S-Transferase Inhibiting Chemical Constituents of Caesalpinia Bonduc. Chem. Pharm. Bull. 55, 442–445. doi:10.1248/cpb.55.442
Wei, H., Liu, X. Q., Zhu, J. J., Gao, L. L., Wang, Z. M., and Yan, L. H. (2016). A New Cassane Diterpenoid from the Seeds of Caesalpinia Decapetala. J. Asian Nat. Prod. Res. 18, 371–375. doi:10.1080/10286020.2015.1055255
Wu, L., Luo, J., Zhang, Y. M., Wang, X. B., Yang, L., and Kong, L. Y. (2014). Cassane-Type Diterpenoids from the Seed Kernels of Caesalpinia Bonduc. Fitoterapia 93, 201–208. doi:10.1016/j.fitote.2014.01.011
Wu, M., Wang, Y. F., Zhang, M. L., Huo, C. H., Dong, M., Shi, Q. W., et al. (2011). Chemical Constituents of Plants from the Genus Caesalpinia. Chem. Biodivers. 8, 1370–1399. doi:10.1002/cbdv.201000176
Wu, P. Q., Yu, Y. F., Zhao, Y., Yu, C. X., Zhi, D. J., Qi, F. M., et al. (2018). Four Novel Sesquiterpenoids with Their Anti-alzheimer’s Disease Activity from Nardostachys Chinensis. Org. Biomol. Chem. 16, 9038–9045. doi:10.1039/c8ob02319k
Xiao, F., Tang, C. P., Ke, C. Q., Yao, S., and Ye, Y. (2016). Rearranged Diterpenoids from the Seeds of Caesalpinia Sappan. Chin. Chem. Lett. 27, 1751–1754. doi:10.1016/j.cclet.2016.04.022
Xin, L. J., Yamujala, R., Wang, Y. H., Wang, H., Wu, W. H., Lawton, M. A., et al. (2013). Acetylcholineestarase-Inhibiting Alkaloids from Lycoris Radiata Delay Paralysis of Amyloid Beta-Expressing Transgenic C. elegans CL4176. PLoS One 8, e63874. doi:10.1371/journal.pone.0063874
Yadav, P. P., Maurya, R., Sarkar, J., Arora, A., Kanojiya, S., Sinha, S., et al. (2009). Cassane Diterpenes from Caesalpinia Bonduc. Phytochemistry 70, 256–261. doi:10.1016/j.phytochem.2008.12.008
Yu, C. X., Wang, R. Y., Qi, F. M., Su, P. J., Yu, Y. F., Li, B., et al. (2020). Eupulcherol A, A Triterpenoid with A New Carbon Skeleton from Euphorbia pulcherrima, and its Anti-alzheimer’s Disease Bioactivity. Org. Biomol. Chem. 18, 76–80. doi:10.1039/c9ob02334h
Zanin, J. L. B., De Carvalho, B. A., Salles Martineli, P., Dos Santos, M. H., Lago, J. H. G., Sartorelli, P., et al. (2012). The Genus Caesalpinia L. (Caesalpiniaceae): Phytochemical and Pharmacological Characteristics. Molecules 17, 7887–7902. doi:10.3390/molecules17077887
Zentar, H., Arias, F., Haidour, A., Alvarez-Manzaneda, R., Chahboun, R., and Alvarez-Manzaneda, E. (2018). Protecting-Group-Free Synthesis of Cassane-type Furan Diterpenes via A Decarboxylative Dienone-Phenol Rearrangement. Org. Lett. 20, 7007–7010. doi:10.1021/acs.orglett.8b02867
Zhang, J. L., Tian, H. Y., Chen, N. H., Bai, X. Y., Li, J., Zhang, R. R., et al. (2014). Caesalpinimin A, A Novel Rearranged Furanoditerpene with an Unprecedented Carbon Skeleton from the Seeds of Caesalpinia Minax Hance. RSC Adv. 4, 7440–7443. doi:10.1039/c3ra46502k
Zhang, J. Y., Abdel-Mageed, W. M., Liu, M. M., Huang, P., He, W. N., Li, L., et al. (2013). Caesanines A-D New Cassane Diterpenes with Unprecedented N Bridge from Caesalpinia Sappan. Org. Lett. 15, 4726–4729. doi:10.1021/ol402058z
Zhang, P. P., Tang, C. P., Yao, S., Ke, C. Q., Lin, G., Hua, H. M., et al. (2016). Cassane Diterpenoids from the Pericarps of Caesalpinia Bonduc. J. Nat. Prod. 79, 24–29. doi:10.1021/acs.jnatprod.5b00520
Keywords: Fabaceae, Caesalpinia bonduc, diterpenoid, caesalpinbondin A, Caenorhabditis elegans, anti-Alzheimer’s disease bioactivity
Citation: Fei D-Q, Li H-H, Chen X-H, Cui W-B, Zhang Z-P, Zhan X-Q, Wang M-J, Qi F-M, Zhang Z-X and Li E-W (2022) Caesalpinbondin A, a Novel Diterpenoid Lactone With an Unprecedented Carbon Skeleton from the Seeds of Caesalpinia bonduc. Front. Chem. 10:911543. doi: 10.3389/fchem.2022.911543
Received: 02 April 2022; Accepted: 29 April 2022;
Published: 24 June 2022.
Edited by:
Xiaoxiao Huang, Shenyang Pharmaceutical University, ChinaReviewed by:
Ming Bai, Shenyang Pharmaceutical University, ChinaRui Guo, Shanxi Medical University, China
Copyright © 2022 Fei, Li, Chen, Cui, Zhang, Zhan, Wang, Qi, Zhang and Li. This is an open-access article distributed under the terms of the Creative Commons Attribution License (CC BY). The use, distribution or reproduction in other forums is permitted, provided the original author(s) and the copyright owner(s) are credited and that the original publication in this journal is cited, in accordance with accepted academic practice. No use, distribution or reproduction is permitted which does not comply with these terms.
*Correspondence: Dong-Qing Fei, feidq@lzu.edu.cn; Zhan-Xin Zhang, zhangzhx@lzu.edu.cn; Er-Wei Li, liew@im.ac.cn