- 1School of Chemistry and Chemical Engineering, Henan Normal University, Xinxiang, China
- 2School of Basic Medical Sciences, Xinxiang Medical University, Xinxiang, China
Ferroptosis is a type of iron-dependent programmed cell death. Once such kind of death occurs, an individual cell would undergo a series of changes related to reactive oxygen species (ROS) in mitochondria. A mitochondrial-targeted ratiometric fluorescent probe (MBI-OMe) was developed to specifically detect ferroptosis-induced ClO−, whose recognition group is p-methoxyphenol, and the mitochondrial-targeted group is benzimidazole. The fluorescence of MBI-OMe was first quenched by 30 μM of Fe3+, and then MBI-OMe appeared as a ratiometric signal at 477 nm and 392 nm in response to ferroptosis-induced ClO− in living cells. MBI-OMe was successfully used to evaluate changes in ClO− induced by ferroptosis.
Introduction
Ferroptosis is a kind of programmed cell death that mainly results from the imbalance between the generation and degradation of intracellular reactive oxygen species (ROS) caused by iron accumulation (Chen et al., 2021; Cheshchevik et al., 2021; Dietrich and Hofmann, 2021). Mitochondria, the centers of energy metabolism in cells, are important sites for ROS generation (Yin et al., 2011; Li et al., 2019). Ferroptosis is frequently accompanied by the accumulation of ROS in mitochondria and is involved in many other pathophysiological processes (Gao et al., 2019; Floros et al., 2021). For example, iron homeostasis plays an important role in the nervous system, and the occurrence of ferroptosis induces neurodegenerative diseases, causing the degradation of mitochondrial activity (Wu et al., 1999; Torti et al., 2013; Zhou et al., 2022). In theory, real-time monitoring of ferroptosis could provide us with necessary information for disease diagnosis. Therefore, it is crucial to develop a selective and sensitive assay for ferroptosis-induced ROS at the solution and cellular levels, which is extremely helpful to understand the physiological and pathological roles of ferroptosis for the living organisms.
Hypochlorous acid, a kind of ROS, usually exists in the form—ClO− in physiological pH (Li et al., 2012; Ren et al., 2019; Zhang et al., 2020a). Due to its oxidative properties, ClO− is used in the immune defense system and plays an important role in a variety of physiological and pathological processes. Iron-dependent ferroptosis leads to the accumulation of ClO− concentrations which can reach the values of 20–400 μM, leading to oxidative damage to mitochondria (Tang et al., 2021; Yan et al., 2021). Fluorescent probes are becoming more and more popular in the detection of biological objects, such as ClO−, because of their simplicity, high temporal and spatial resolution, and real-time and non-destructive biological imaging. To date, fluorescent probes based on the detection of ClO− targeting different organelles have been designed (Wang et al., 2019; Li et al., 2020; Zhang et al., 2020b). In addition, a number of fluorescent probes have been prepared that specifically monitor ClO− changes in different disease models, such as sepsis, pneumonia, and cancer (Zhang et al., 2018; Long et al., 2020; Wang et al., 2021; Yang et al., 2021). Although these probes can achieve specific, fast, and sensitive responses to ClO−, these probes cannot be used to detect ClO− content changes in suborganelles during ferroptosis. This is mainly because the concentration of ClO− produced by iron death is larger than that in normal cells, so it is impossible to determine whether ferroptosis has occurred. Therefore, it is necessary to develop related reactive oxygen species probes to selectively detect ClO− and perform fluorescence imaging during ferroptosis.
With this in mind, we developed a mitochondrial-targeted fluorescent probe (MBI-OMe) for monitoring the changing behavior of ClO− that was induced by ferroptosis in this work. MBI-OMe was synthesized by linking p-methoxyphenol with a fluorophore benzimidazole via a conjugated vinyl bond. p-Methoxyphenol can enhance the fluorescence intensity of the probe and act as a selective recognition site for ClO− (Hu et al., 2014). MBI-OMe could coordinate with iron ions to quench fluorescence. When encountering ClO− induced by ferroptosis, MBI-OMe could produce a ratiometric fluorescence signal. More importantly, MBI-OMe with a high fidelity signal can be used to monitor ClO− production that accompanies ferroptosis, suggesting that mitochondrial ClO− levels may play an important role in ferroptotic cancer cells. Therefore, MBI-OMe is considered to be instructive to study the detection of ClO− in ferroptosis.
Materials and Methods
Instruments and Reagents
All chemicals and solvents used in synthesis are commercially purchased, and no further purification is performed unless otherwise stated. The structures were characterized by AVANCE III HD 600MHZ (600 MHz 1H, 151 MHz 13C) NMR spectroscopy. The solvent was DMSO (TMS as an internal standard). An ultrahigh resolution electrospray time-of-flight mass spectrometry system was used to determine the molecular weight of compounds. Fluorescence spectra were determined using the HORIBA FluoroMax-Plus spectrophotometer. The UV spectrum was determined by the Cintra 2020 spectrophotometer of GBC. Fluorescence images were collected using an Olympus FV1200 confocal laser fluorescence microscope.
Synthesis of Probe MBI-OMe
Here, 2-methylbenzimidazole (1.5 mmol, 200.0 mg) and 2-hydroxy-5-methoxybenzaldehyde (1.8 mmol, 276.3 mg) were added to a mixed solution of acetic acid (6.0 ml) and acetic anhydride (12.0 ml). The mixture was obtained after reacting at 120°C for 6 h. The solution was cooled with water; 5 ml of concentrated hydrochloric acid was added overnight, and a solid was obtained by filtration. The yellow solid MBI-OMe was obtained by column chromatography. The yield of the probe MBI-OMe was 64%. 1H NMR (600 MHz, DMSO-d6) δ 12.76 (s, 1H), 9.66 (s, 1H), 7.86 (d, J = 16.6 Hz, 1H), 7.52 (dd, J = 5.9, 3.2 Hz, 2H), 7.27 (d, J = 16.6 Hz, 1H), 7.20–7.10 (m, 3H), 6.85 (d, J = 8.8 Hz, 1H), 6.80 (dd, J = 8.8, 2.9 Hz, 1H), and 3.75 (s, 3H). 13C NMR (151 MHz, DMSO-d6) δ 152.81, 151.99, 150.39, 130.86, 123.29, 122.49, 117.55, 117.34, 116.86, 111.74, and 55.91. High-resolution mass spectrometry (HRMS) m/z calcd for C16H14N2O2 (M + H)+: 267.1128; found 267.1156.
Cell Imaging
HL-7702 cells and HepG 2 cells were cultured in Dulbecco’s modified Eagle’s medium (Corning) containing 10% fetal bovine serum (Sigma Aldrich) and 1% penicillin/streptomycin (Corning). Cell culture conditions were 37°C and 5% CO2.
For the imaging experiment of exogenous ClO−, HepG 2 cells were incubated with ClO− solution of different concentrations for 30 min, and then the probe MBI-OMe was added for further incubation for 30 min. In the endogenous ClO− imaging experiment, lipopolysaccharides (LPSs) were added to HepG 2 cells and incubated for 12 h; phorbol-12-myristate-13-acetate (PMA) was added for further incubation for 90 min, and then the probe was added for 30 min for imaging. The cells treated with LPS and PMA were incubated with N-acetylcysteine (NAC) and 4-azidobenzohydrazide (ABH) for 1 h and then incubated with MBI-OMe for 30 min for imaging. The HepG 2 cells were incubated with erastin for 6 h to construct the ferroptosis cell model and then incubated with the probe for 30 min for imaging. Deferoxamine was added and incubated for 6 h, and MBI-OMe was added and incubated for 30 min before imaging.
Results and Discussions
Design and Synthesis of MBI-OMe
In order to monitor the changing behavior of ClO− during ferroptosis, a ClO− responsive fluorescent probe (MBI-OMe) was designed. In molecular design, the p-methoxyphenol group was selected as the recognition site because it can produce benzoquinone through ClO− oxidation. In order to realize the detection of ClO− in the process of ferroptosis, imidazole and hydroxyl groups, which can complex with Fe3+, were introduced into the probe as the turn-on signal for the detection of ferroptosis. To form the typical intramolecular charge transfer (ICT) molecular system, MBI-OMe was synthesized by connecting p-methoxyphenol as the electron-donor group, benzimidazole compound as the electron-acceptor group, and vinyl as the conjugated bridge. When the probe is complexed with Fe3+, the ICT effect of the probe is weakened, and the fluorescence is quenched. When the system encounters ClO−, the complex system is destroyed and the fluorescence is recovered. As the ClO− concentration increased, methoxyphenol was oxidized to benzoquinone, thereby exhibiting a proportional fluorescence signal.
As shown in Scheme 1, the synthetic route of MBI-OMe is simple, and MBI-OMe is obtained by the Knoevenagel condensation reaction of 2-methylbenzimidazole (compound 1) and 2-hydroxy-5-methoxy-benzaldehyde (compound 2) directly. The chemical structure of MBI-OMe was characterized by 1H NMR, 13C NMR, and ESI-TOF-MS.
Spectral Properties
The optical properties of the MBI-OMe (2.0 μM) were investigated by UV-vis absorption and fluorescence spectroscopy in PBS buffer (pH = 7.4). As shown in Figure 1A, MBI-OMe showed bright fluorescence at 477 nm that was excited by the one-photon light source (λexone-photon = 325 nm, Φ = 0.28) and the two-photon light source (λextwo-photon = 750 nm, Supplementary Figure S1). When MBI-OMe encountered 30 μM of Fe3+ in PBS buffer (pH = 7.4), the fluorescence decreased to 1.5×105 at 477 nm (Φ = 0.015) within 1 min (Supplementary Figure S2). Also, the dosage of Fe3+ for the MBI-OMe is 30 μM, which is far greater than the endogenous amount of Fe3+ in living organisms. Therefore, the Fe3+ concentration increased to 30 μM when ferroptosis occurred (Hentze et al., 2010). Due to the complexation of a large amount of Fe3+ with MBI-OMe, resulting in the change of the intramolecular conjugated system, the fluorescence intensity is quenched (Zhao et al., 2016). After that, by adding ClO− into the system, the fluorescence of MBI-OMe at 477 nm (Φ = 0.25) was recovered within 1 min (Supplementary Figure S2). When ClO− was continually added into the system, fluorescence strength at 477 nm decreased and was accompanied by another increase at a new emission peak (392 nm, Figure 1B).
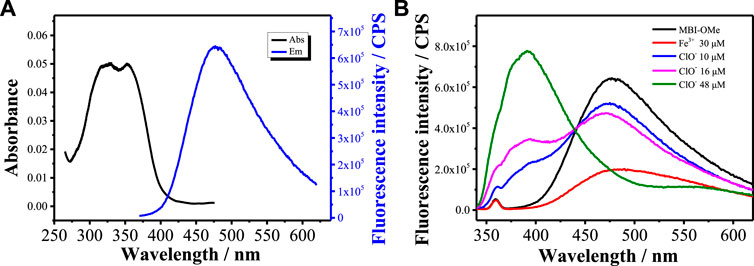
FIGURE 1. (A) Absorption and emission spectra of MBI-OMe (2.0 μM) in PBS buffer (pH = 7.4). (B) Emission spectra of MBI-OMe in response to Fe3+ (30 μM) and ClO− (10, 16, and 48 μM).
Figure 2A shows the fluorescence spectra of MBI-OMe in PBS buffer (pH = 7.4) containing different concentrations of ClO− (0–56 μM) at an excitation wavelength of 325 nm. With the increase of ClO− concentration, the fluorescence at 477 nm gradually weakened, a new fluorescence emission peak appeared at 392 nm, and the fluorescence intensity gradually increased with the increase of ClO− concentration. The emission peak at 392 nm increased 40-fold after the addition of 56 μM ClO−. As can be seen in Figure 2B, the fluorescence intensity has a good linear relationship with the concentration of ClO− in the range of 8–56 μM. The detection limit of MBI-OMe for ClO− is 1.2 μM. The results showed that the probe could interact with ClO− with good sensitivity.
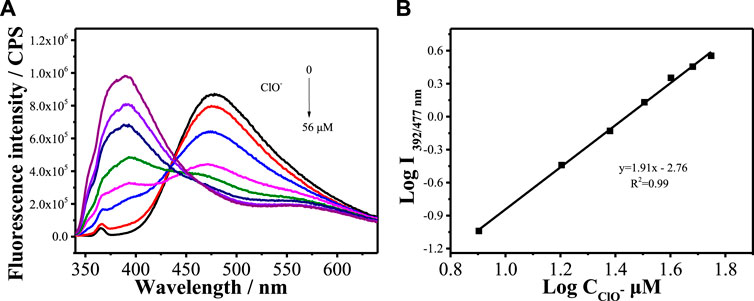
FIGURE 2. (A) Fluorescence spectra of MBI-OMe (2.0 µM) in PBS buffer (pH = 7.4) containing different concentrations of ClO− (0–56 μM). (B) Linear relationship between MBI-OMe fluorescence intensity and ClO− concentration.
To evaluate the selectivity of MBI-OMe for ClO−, the fluorescence responses to various analytes, including biologically reactive oxygen species, amino acids, and cationic and anionic species, were investigated in PBS buffer (pH = 7.4). As shown in Supplementary Figure S4, there were no significant changes in other bioactive species, except for the ClO−-induced spectra change. Meanwhile, in order to prove that MBI-OMe can have good photostability in vivo, the fluorescence intensity of the PBS solution containing MBI-OMe after illumination at different times was recorded. Supplementary Figure S5 shows that the fluorescence intensity decreases slightly with the increase of time, but the intensity remains above 80% after 5 h, which indicates that MBI-OMe has good photostability. The results showed that MBI-OMe has a good fluorescence response to ClO−, which is very suitable for biological applications.
Sensing Mechanism
Based on the aforementioned spectroscopic evidence, a possible mechanism for the detection of ClO− and Fe3+ by MBI-OMe was proposed (Scheme 2). The sensing mechanism was detected by adding ClO− and Fe3+ to the MBI-OMe probe solution. The reaction solution was detected by high-resolution mass spectrometry (HRMS). When Fe3+ (30 μM) was added into MBI-OMe (2.0 μM), the mass spectrum showed a peak at 267.1126 M/z [M + H+]. This indicated that the probe only complexed with Fe3+ without changing the molecular structure. When ClO− (20 μM) was added into it, there were two peaks showed by the mass spectrum at 267.1121 M/z [M + H+] and 249.0663 M/z [M-H+]. This indicated that with the addition of ClO−, the phenolic hydroxyl and methoxy groups in the probe were oxidized to benzoquinone. In addition, mass spectrometry analysis showed 251.0790 M/z [M + H+] after adding amounts of ClO− to the probe-containing solution (Supplementary Figure S6). It was further proved that p-methoxyphenol was oxidized to benzoquinone in the probe, and a proportional fluorescence signal was generated.
Chemical Stability and Biological Toxicity
To verify whether MBI-OMe can be used for bioimaging detection, the chemical stability of MBI-OMe in the physiological pH range was first investigated. As shown in Figure 3A, it can be seen that the fluorescence intensity of MBI-OMe remains almost unchanged with the change of pH 6.0–9.0. This indicates that MBI-OMe has good chemical stability and can be used for testing in a physiological environment. To further illustrate the feasibility of the probe for the imaging detection in living cells, the cytotoxicity was measured by MTT assay in HL-7702 cells and HepG 2 cells. Figure 3B showed that the cell viability was maintained above 95% under incubation by different concentrations of MBI-OMe, showing good cell viability. MBI-OMe proved to be a potential tool for detecting and imaging in living cells.
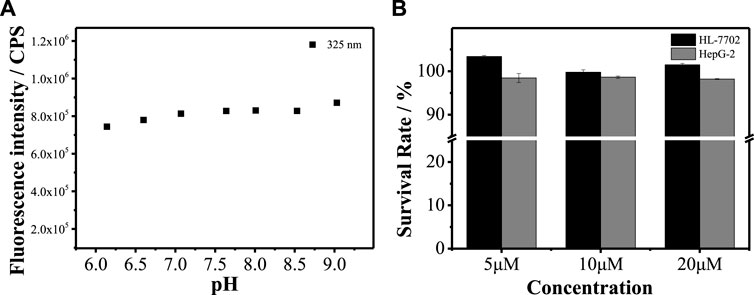
FIGURE 3. (A) Fluorescence signal changes of MBI-OMe (2.0 µM) at different pH (6.0–9.0). (B) Cytotoxicity of MBI-OMe at different concentrations (5, 10, and 20 µM).
Ratiometric Fluorescence Detection and Imaging of ClO−
We first explored whether this probe could be used for imaging (Supplementary Figure S7A) in living cells. When HepG 2 cells were incubated with MBI-OMe, there appeared a distinct fluorescence and presented good regional characteristics. The colocalization experiments demonstrated that MBI-OMe was able to localize in the mitochondria (Supplementary Figure S7). In addition, two-photon imaging in cells showed that the probe had a good two-photon effect (Supplementary Figure S8). These results suggested that MBI-OMe can be used to image intracellular mitochondria. By adding ClO− to cells, it was demonstrated that the probe could detect and image ClO− in cells (Supplementary Figure S9). Subsequently, the endogenous ClO− production was induced in cells by adding lipopolysaccharide and phorbol, as shown in Figures 4A,B. The fluorescence of MBI-OMe in both the red channel (460–560 nm) and green channel (415–450 nm) was weakened. This may be due to the fact that the excitation wavelength is not optimal (Ex = 405 nm, the best excitation wavelength is 325 nm), which leads to the decreased fluorescence intensity of the probe in the green channel. In order to verify whether endogenous ClO− responded to the probe, NAC and ABH were added to cells, as shown in Figures 4C,D. When ClO− was removed from cells, the probe was added for incubation, and the fluorescence intensity was basically the same as that of the probe alone. The aforementioned experiments indicate that MBI-OMe may be an effective tool for detecting and imaging ClO− in mitochondria of ferroptosis cells.
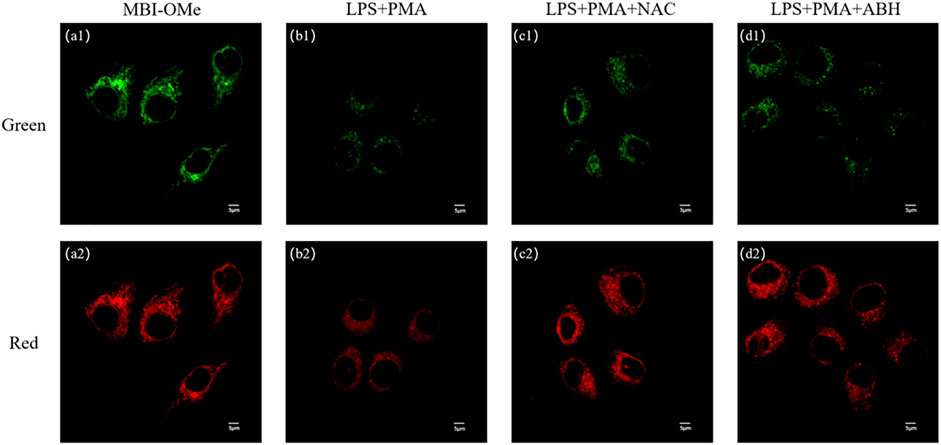
FIGURE 4. (A) Imaging of MBI-OMe in HepG 2 cells. (B) Cell imaging of MBI-OMe performed after LPS (1.0 μg/ml, 12 h) and PMA (10 mg/ml, 90 min) were added to the cells. (C) Cell imaging of MBI-OMe after incubation with NAC (2.0 mM, 1 h) under the condition of (B). (D) Cell imaging of MBI-OMe after incubation with ABH (200 μM, 1 h) under the condition of (B). Green: 415–450 nm; Red: 460–560 nm; MBI-OMe: 30 µM.
Changes of ClO− During Cell Ferroptosis
Ferroptosis is a novel mode of cell death distinct from apoptosis, necrosis, and autophagy (Dixon et al., 2012; Li, 2020). The most characteristic feature of ferroptosis is the need for iron, and some iron chelators can inhibit this process. Studies have shown that the production of ROS is observed during ferroptosis (Li et al., 2019; Chen et al., 2020; Zhang et al., 2020a). Here, the ability of MBI-OMe to image ClO− during ferroptosis in HepG 2 cells was further investigated by constructing a cellular ferroptosis model. As shown in Figure 5, the fluorescence intensity of the green and red channels was significantly attenuated after treatment of cells with erastin, a classic iron ion inducer (Dixon et al., 2012), for 6 h, compared with untreated HepG 2 cells. Deferoxamine (DFO, an inhibitor of ferroptosis) significantly inhibits the onset of ferroptosis (Dixon et al., 2012). When DFO was added to the treated cells and incubated, the fluorescence intensity of the probe was basically the same as that of the probe only, which was the same as that of the in vitro spectroscopic experiment and intracellular ClO− imaging experiment. The aforementioned results suggest that the probe MBI-OMe can be used as an imaging sensor for ClO− changes during ferroptosis in cancer cells.
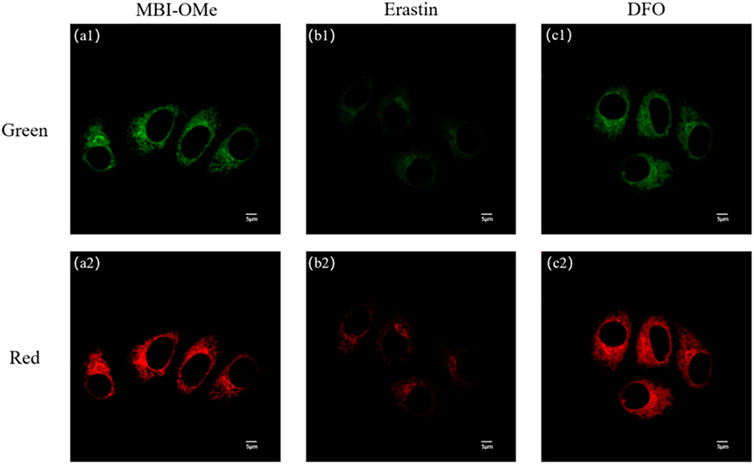
FIGURE 5. (A) Imaging of MBI-OMe in HepG 2 cells. (B) Cell imaging of MBI-OMe performed after erastin (10 μM, 6 h) was added to the cells. (C) Cell imaging of MBI-OMe after incubation with DFO (100 μM, 6 h) under the condition of (B). Green: 415–450 nm; Red: 460–560 nm; MBI-OMe: 30 µM.
Conclusion
In summary, MBI-OMe based on benzimidazole was developed for selective detection of ClO− in mitochondria during ferroptosis. The p-methoxyphenol group is used as the specific reaction site of ClO−, and methylbenzimidazole is used for the mitochondrial targeting. MBI-OMe appears as a fluorescence at 477 nm. MBI-OMe can form a complex with Fe3+, and its fluorescence also was quenched (Φ = 0.015). More importantly, MBI-OMe can not only complex with Fe3+ for fluorescence quenching but also show a good ratiometric fluorescence signal to ClO− that was caused by ferroptosis stimulation. The fluorescence intensity ratio (I392 nm/I477 nm) was linearly related to the concentration of ClO− with a detection limit of 1.2 μM. This can mainly be attributed to the formation of benzoquinone through the redox reaction between ClO− and p-methoxyphenol. MBI-OMe has good two-photon properties, which is beneficial to the detection and imaging of ClO− in ferroptosis. This work provides an effective tool for the detection of ferroptosis and its ClO−-related diseases. In this work, the probe was able to achieve a proportional fluorescence signal in vitro to detect ClO−. However, the excitation light wavelength is in the range of 275–400 nm in one-photon mode. Although the probe has two-photon properties, in order to broaden the application of this type of probe in biological detection and imaging, it is still necessary to improve the wavelength of the probe under single-photon excitation. Therefore, more long-wavelength fluorescent probes need to be designed for the diagnosis of biological diseases.
Data Availability Statement
The original contributions presented in the study are included in the article/Supplementary Material; further inquiries can be directed to the corresponding author.
Author Contributions
All the authors listed have made a substantial, direct, and intellectual contribution to the work and approved it for publication.
Funding
This work was supported by the National Natural Science Foundation of China (U21A20314, 22107089, 21722501, and 21803018), National Natural Science Foundation of Henan Province (222300420207), and Postdoctoral Research Funding (5101219470256).
Conflict of Interest
The authors declare that the research was conducted in the absence of any commercial or financial relationships that could be construed as a potential conflict of interest.
Publisher’s Note
All claims expressed in this article are solely those of the authors and do not necessarily represent those of their affiliated organizations, or those of the publisher, the editors, and the reviewers. Any product that may be evaluated in this article, or claim that may be made by its manufacturer, is not guaranteed or endorsed by the publisher.
Acknowledgments
HZ wishes to thank the Zhongyuan High Level Talents Special Support Plan-Science and Technology Innovation Leading Talents (204200510006); GW wishes to thank the National Natural Science Foundation of Henan Province (212300410226).
Supplementary Material
The Supplementary Material for this article can be found online at: https://www.frontiersin.org/articles/10.3389/fchem.2022.909670/full#supplementary-material
References
Chen, X., Yu, C., Kang, R., Kroemer, G., and Tang, D. (2021). Cellular Degradation Systems in Ferroptosis. Cell Death Differ. 28, 1135–1148. doi:10.1038/s41418-020-00728-1
Chen, Y.-C., Oses-Prieto, J. A., Pope, L. E., Burlingame, A. L., Dixon, S. J., and Renslo, A. R. (2020). Reactivity-based Probe of the Iron(II)-dependent Interactome Identifies New Cellular Modulators of Ferroptosis. J. Am. Chem. Soc. 142, 19085–19093. doi:10.1021/jacs.0c06710.1021/jacs.0c06709
Cheshchevik, V. T., Krylova, N. G., Сheshchevik, N. G., Lapshina, E. A., Semenkova, G. N., and Zavodnik, I. B. (2021). Role of Mitochondrial Calcium in Hypochlorite Induced Oxidative Damage of Cells. Biochimie 184, 104–115. doi:10.1016/j.biochi.2021.02.009
Dietrich, C., and Hofmann, T. G. (2021). Ferroptosis Meets Cell-Cell Contacts. Cells 10, 2462. doi:10.3390/cells10092462
Dixon, S. J., Lemberg, K. M., Lamprecht, M. R., Skouta, R., Zaitsev, E. M., Gleason, C. E., et al. (2012). Ferroptosis: an Iron-dependent Form of Nonapoptotic Cell Death. Cell 149, 1060–1072. doi:10.1016/j.cell.2012.03.042
Floros, K. V., Cai, J., Jacob, S., Kurupi, R., Fairchild, C. K., Shende, M., et al. (2021). MYCN-amplified Neuroblastoma Is Addicted to Iron and Vulnerable to Inhibition of the System Xc-/glutathione axis. Cancer Res. 81, 1896–1908. doi:10.1158/0008-5472.CAN-20-1641
Gao, M., Yi, J., Zhu, J., Minikes, A. M., Monian, P., Thompson, C. B., et al. (2019). Role of Mitochondria in Ferroptosis. Mol. Cell 73, 354–363. doi:10.1016/j.molcel.2018.10.042
Hentze, M. W., Muckenthaler, M. U., Galy, B., and Camaschella, C. (2010). Two to Tango: Regulation of Mammalian Iron Metabolism. Cell 142, 24–38. doi:10.1016/j.cell.2010.06.028
Hu, J. J., Wong, N.-K., Gu, Q., Bai, X., Ye, S., and Yang, D. (2014). HKOCl-2 Series of Green BODIPY-Based Fluorescent Probes for Hypochlorous Acid Detection and Imaging in Live Cells. Org. Lett. 16, 3544–3547. doi:10.1021/ol501496n
Li, H., Cao, Z., Moore, D. R., Jackson, P. L., Barnes, S., Lambeth, J. D., et al. (2012). Microbicidal Activity of Vascular Peroxidase 1 in Human Plasma via Generation of Hypochlorous Acid. Infect. Immun. 80, 2528–2537. doi:10.1128/IAI.06337-11
Li, H., Shi, W., Li, X., Hu, Y., Fang, Y., and Ma, H. (2019). Ferroptosis Accompanied by OH Generation and Cytoplasmic Viscosity Increase Revealed via Dual-Functional Fluorescence Probe. J. Am. Chem. Soc. 141, 18301–18307. doi:10.1021/jacs.9b09722
Li, M.-Y., Li, K., Liu, Y.-H., Zhang, H., Yu, K.-K., Liu, X., et al. (2020). Mitochondria-immobilized Fluorescent Probe for the Detection of Hypochlorite in Living Cells, Tissues, and Zebrafishes. Anal. Chem. 92, 3262–3269. doi:10.1021/acs.analchem.9b05102
Li, Z. (2020). Imaging of Hydrogen Peroxide (H2O2) during the Ferroptosis Process in Living Cancer Cells with a Practical Fluorescence Probe. Talanta 212, 120804. doi:10.1016/j.talanta.2020.120804
Long, L., Han, Y., Liu, W., Chen, Q., Yin, D., Li, L., et al. (2020). Simultaneous Discrimination of Hypochlorite and Single Oxygen during Sepsis by a Dual-Functional Fluorescent Probe. Anal. Chem. 92, 6072–6080. doi:10.1021/acs.analchem.0c00492
Ren, M., Li, Z., Deng, B., Wang, L., and Lin, W. (2019). Single Fluorescent Probe Separately and Continuously Visualize H2S and HClO in Lysosomes with Different Fluorescence Signals. Anal. Chem. 91, 2932–2938. doi:10.1021/acs.analchem.8b05116
Tang, D., Chen, X., Kang, R., and Kroemer, G. (2021). Ferroptosis: Molecular Mechanisms and Health Implications. Cell Res. 31, 107–125. doi:10.1038/s41422-020-00441-1
Torti, S. V., and Torti, F. M. (2013). Iron and Cancer: More Ore to Be Mined. Nat. Rev. Cancer 13, 342–355. doi:10.1038/nrc3495
Wang, L., Liu, J., Zhang, H., and Guo, W. (2021). Discrimination between Cancerous and Normal Cells/tissues Enabled by a Near-Infrared Fluorescent HClO Probe. Sensors Actuators B Chem. 334, 129602. doi:10.1016/j.snb.2021.129602
Wang, Z., Zhang, Q., Liu, J., Sui, R., Li, Y., Li, Y., et al. (2019). A Twist Six-Membered Rhodamine-Based Fluorescent Probe for Hypochlorite Detection in Water and Lysosomes of Living Cells. Anal. Chim. Acta 1082, 116–125. doi:10.1016/j.aca.2019.07.046
Wu, K.-J., Polack, A., and Dalla-Favera, R. (1999). Coordinated Regulation of Iron-Controlling Genes, H-Ferritin and IRP2 , by C-MYC. Science 283, 676–679. doi:10.1126/science.283.5402.676
Yan, H.-f., Zou, T., Tuo, Q.-z., Xu, S., Li, H., Belaidi, A. A., et al. (2021). Ferroptosis: Mechanisms and Links with Diseases. Sig Transduct. Target Ther. 6, 49. doi:10.1038/s41392-020-00428-9
Yang, X., Liu, J., Xie, P., Han, X., Zhang, D., Ye, Y., et al. (2021). Visualization of Biothiols and HClO in Cancer Therapy via a Multi-Responsive Fluorescent Probe. Sensors Actuators B Chem. 347, 130620. doi:10.1016/j.snb.2021.130620
Yin, H., Xu, L., and Porter, N. A. (2011). Free Radical Lipid Peroxidation: Mechanisms and Analysis. Chem. Rev. 111, 5944–5972. doi:10.1021/cr200084z
Zhang, H.-C., Tian, D.-H., Zheng, Y.-L., Dai, F., and Zhou, B. (2021). Designing an ESIPT-Based Fluorescent Probe for Imaging of Hydrogen Peroxide during the Ferroptosis Process. Spectrochimica Acta Part A Mol. Biomol. Spectrosc. 248, 119264. doi:10.1016/j.saa.2020.119264
Zhang, X., Zhao, W., Li, B., Li, W., Zhang, C., Hou, X., et al. (2018). Ratiometric Fluorescent Probes for Capturing Endogenous Hypochlorous Acid in the Lungs of Mice. Chem. Sci. 9, 8207–8212. doi:10.1039/c8sc03226b
Zhang, M.-M., Ma, Y.-H., Li, P., Jia, Y., and Han, K.-L. (2020a). Detection of Atherosclerosis-Related Hypochlorous Acid Produced in Foam Cells with a Localized Endoplasmic Reticulum Probe. Chem. Commun. 56, 2610–2613. doi:10.1039/D0CC00090F
Zhang, W., Wang, H., Li, F., Chen, Y., Kwok, R. T. K., Huang, Y., et al. (2020b). A Ratiometric Fluorescent Probe Based on AIEgen for Detecting HClO in Living Cells. Chem. Commun. 56, 14613–14616. doi:10.1039/D0CC06582J
Zhao, J., Gao, Q., Zhang, F., Sun, W., and Bai, Y. (2016). Two Colorimetric Fluorescent Probes for Detection Fe3+: Synthesis, Characterization and Theoretical Calculations. J. Luminescence 180, 278–286. doi:10.1016/j.jlumin.2016.07.037
Keywords: fluorescent probe, ClO−, ratio fluorescence, mitochondria, ferroptosis
Citation: Feng B, Wang K, Wang Z, Niu H, Wang G, Chen Y and Zhang H (2022) Mitochondrial-Targeted Ratiometric Fluorescent Probe to Monitor ClO− Induced by Ferroptosis in Living Cells. Front. Chem. 10:909670. doi: 10.3389/fchem.2022.909670
Received: 31 March 2022; Accepted: 02 May 2022;
Published: 09 June 2022.
Edited by:
Tingting Zheng, East China Normal University, ChinaReviewed by:
Lin Yuan, Hunan University, ChinaDuanping Sun, Guangdong Pharmaceutical University, China
Copyright © 2022 Feng, Wang, Wang, Niu, Wang, Chen and Zhang. This is an open-access article distributed under the terms of the Creative Commons Attribution License (CC BY). The use, distribution or reproduction in other forums is permitted, provided the original author(s) and the copyright owner(s) are credited and that the original publication in this journal is cited, in accordance with accepted academic practice. No use, distribution or reproduction is permitted which does not comply with these terms.
*Correspondence: Hua Zhang, emhhbmdodWExMTA2QDE2My5jb20=