- 1Department of Pharmacy, First Affiliated Hospital of Gannan Medical University, Ganzhou, China
- 2School of Pharmacy, Gannan Medical University, Ganzhou, China
- 3Key Laboratory of Biomedical Sensors of Ganzhou, Ganzhou Key Laboratory of Immunotherapeutic Drugs Developing for Childhood Leukemia, Key Laboratory of Biomaterials and Biofabrication in Tissue Engineering of Jiangxi Province, School of Medical and Information Engineering, School of Public Health and Health Management, Oil-Tea in Medical Health Care and Functional Product Development Engineering Research Center in Jiangxi, Gannan Medical University, Ganzhou, China
As a new type of “zero-dimensional” fluorescent carbon nanomaterials, carbon dots (CDs) have some unique optical and chemical properties, they are being explored for a variety of applications in bio-related fields, such as bioimaging, biosensors, and therapy. This review mainly summarizes the recent progress of CDs in bioimaging. The overview of this review can be roughly divided into two categories: (1) In vitro bioimaging based on CDs in different cells and important organelles. (2) The distribution, imaging and application of CDs in mice and zebrafish. In addition, this review also points out the potential advantages and future development directions of CDs for bioimaging, which may promote the development of CDs in the field of bioimaging.
1 Introduction
Biological imaging covers many modalities, including X-ray, B-ultrasound, Computed tomography (CT), Positron emission computed tomography (PET), Magnetic resonance imaging (MRI), and so on (Li et al., 2019; Molkenova et al., 2019; Molkenova et al., 2020; Wen et al., 2020; Mulikova et al., 2021; Olifirenko et al., 2021; Yi et al., 2022). Among them, fluorescence imaging technology plays an important role in bioimaging due to its advantages of high sensitivity, easy observation and simple instrument. At present, many fluorescent material have been used for biological imaging, such as organic small molecules (Wu J. et al., 2021), nanomaterials (Deling Li et al., 2020), and so on. Since carbon dots (CDs) were synthesized in 2004 (Xu et al., 2004), they have received extensive attention in various fields as a new type of fluorescent probe. Because of their multicolor luminescence (Kailasa et al., 2019; Jiao et al., 2020; Ghosh et al., 2021a; Ghosh et al., 2021b), tunable optical properties (Wang et al., 2020a), superior chemical and photostability (Wang et al., 2020b; Rao et al., 2020), low cytotoxicity and excellent biocompatibility (Huang et al., 2020; Kuang et al., 2020; Lin et al., 2021a; Lin et al., 2021b; Mei et al., 2022), CDs are promising candidates bioimaging. Due to the easy functionalization and good biocompatibility of the surface of CDs, they can also be used as an effective tool for visual monitoring of biological processes (Sun et al., 2021; Vedhanayagam et al., 2021; Huang et al., 2022). More importantly, the synthesis method of CDs is simple, environmentally friendly, economical, and energy-saving, and their synthetic raw materials are widely sourced, green and cheap. Therefore, a wide range of CDs preparation methods and sources of raw materials provide opportunities to achieve biological in vitro and in vivo imaging.
In recent years, several reviews have been summarized on applications of CDs for neurological treatment, biosensors, photocatalysis, bioimaging, and so on (Ashrafizadeh et al., 2020; Younis et al., 2020; Kailasa and Koduru, 2022; Wang and Lu, 2022; Ðorđević et al., 2022). At present, the review on the bioimaging of CDs mostly focuses on the in vitro bioimaging of CDs, such as imaging of cancer cells and nerve cells, etc. In this review, we survey the latest research on the application of CDs in the rapidly evolving bioimaging field, especially in vitro bioimaging of important organelles (nucleus, mitochondria) and in vivo bioimaging (mice, zebrafish). This review aims to provide readers with the latest, most exciting and influential research in this field. What’s more, the opportunities and challenges of carbon dots in bioimaging applications in the future are briefly discussed. Eventually, we hope to provide some new ideas for developing bioimaging based on CDs.
2 In Vitro Bioimaging
CDs have shown excellent potential in the field of bioimaging due to the CDs have good dispersibility, the ability to specifically bind to target units, high near-infrared absorption and photoluminescence efficiency, good chemical stability and photostability (Bondon et al., 2020; Lin et al., 2020; Cardoso Dos Santos et al., 2020; Wang et al., 2021). As known, Different types of cells have different structures and morphologies, and different cell membranes or cytoplasm contain different biomarkers, leading to specific responses to foreign nanoparticles. On this basis, CDs with special functions were synthesized and used in biological imaging technology, which also provided the possibility of applying CDs in vivo bioimaging, which hope to promote the development of cell imaging techniques.
2.1 Cell Bioimaging
2.1.1 Cancer Cells Bioimaging
Cancer is one of the greatest challenges facing humanity today, and many people die from cancer every year, early diagnosis of cancer and prevention of malignant tumors are extremely important. Currently, CDs can penetrate a variety of cancer cells, what’s more due to the stable optical properties and excellent biocompatibility of CDs, cancer cells can be detected by fluorescence imaging of CDs (Mchugh et al., 2018). Gudimella et al. synthesis of fluorescent CDs from the citrus peel as a renewable green resource, and then the CDs was conjugated with folic acid (FA-CDs). As shown in Figure 1A, MCF-7 cells treated with FA-CDs had brighter fluorescence emission than MCF-7 cells treated with CDs, indicating that FA-CDs are a remarkable material for cell imaging (Gudimella et al., 2021). Different CDs can specifically recognize cancer cells by interacting with groups on the surface of cancer cells. However, cellular uptake of free CDs lacks selectivity, and the same negative charge as the cell membrane may lead to inefficient cellular internalization. Based on the modification of CDs with the DNA aptamer AS1411 with polyimine (PEI) as the bridge. Kong et al. developed a surface charge inversion nanosystem, using the DNA aptamer AS1411 labeled CDs nanoparticle probe for specifically targeted bioimaging of cancer cells (Figure 1B) (Kong et al., 2020). In addition, Mahani et al. demonstrated the selective targeting and imaging of hepatoma cells by fluorescent CDs molecularly imprinted polymers (CDs-MIPs). The overexpression of monosaccharides on cancer cells can act as targeting molecules. On these cells, CDs-MIP specifically binds to D-glucuronicacid (GlcA) and N-acetylneuraminicacid (NANA), resulting in high-contrast images in cancer cells imaging (Mahani et al., 2021).
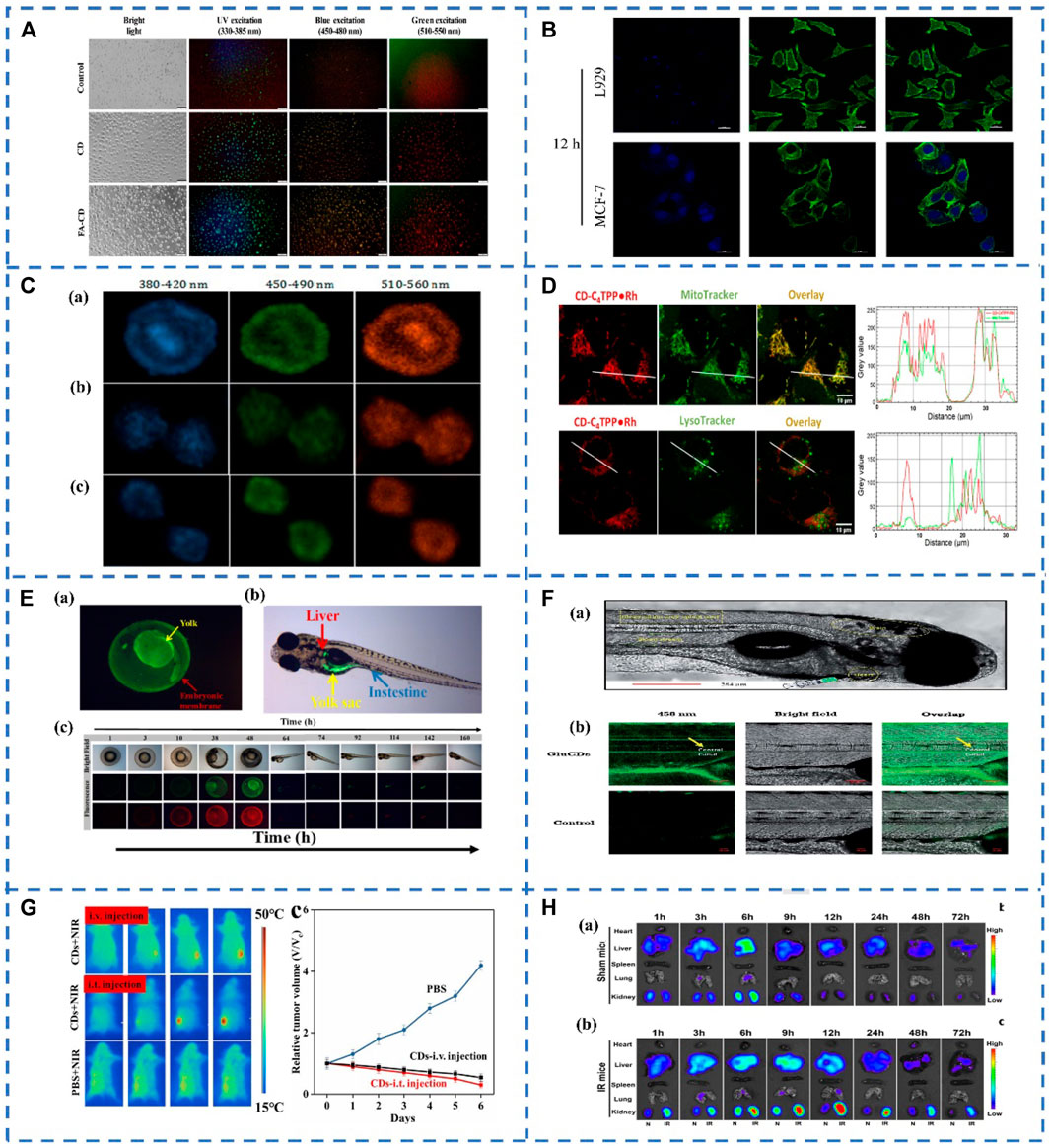
FIGURE 1. (A) Bioimaging of CDs and FA-CDs in cancer cells (Gudimella et al., 2021). (B) Flow cytometry profiles of MCF-7 cells and L929 cells treated with the CDs-PEI-AS1411 for 12 h (Kong et al., 2020). (C) Mitotic cells stained by N-CDs visualizing chromosome aggregation through progression of mitosis’ prophase, metaphase and anaphase, respectively (Zhang et al., 2020). (D) N-CDs internalization in live MDA-MB-231 cells. (Kaminari et al., 2021); (E) (a) Uptake and distribution of WCDs in zebrafish embryos; (b) The distribution of WCDs in zebrafish larvae; (c) Fluorescence microscopy images of uptake of WCDs in zebrafish growth cycle (Zonglin Liu et al., 2021). (F) (a) Confocal images of wild-type zebrafish showing the injection route, heart, blood stream, CNS and observation area (central canal of spinal cord). (b) Accumulation of GluCDs-F in the CNS of zebrafish (Seven et al., 2021). (G) Representative infrared thermal images of tumor-bearing mice with intravenous injection, intratumoral (i.t.) injection of CDs (50 μl, 20 mg/ml) and PBS (50 μl) into the tumor site under 808 nm laser irradiation and time course change in the relative volume after treatment by using CDs and PBS (Li et al., 2019) (H) In vivo bio-distribution of PDA-CDs in both ischemia-reperfusion (IR)-AKI and healthy mice.Representative fluorescence images (excitation: 410 nm, emission: 500 nm) of major organs from shammice (a) and IR-injured mice (b) at different times after PDA-CDs intravenous injection (Gao et al., 2020).
2.1.2 Neural Cells Bioimaging
An in-depth and comprehensive understanding of the nature and function of the nervous system can lead to more effective treatment of brain diseases. Therefore, it is necessary to develop a new brain bioimaging method to visualize the relationship between brain structure, neuronal activity and neurochemistry. Due to their excellent biocompatibility, stable fluorescence properties of CDs can help to overcome current challenges in neuroimaging. Pei et al. used lignin as the starting carbon source to synthesize carbon quantum dots CDs by hydrothermal method. The team explored the imaging of lignin CDs in N27 cells (a rat neural cell line used as a model of dopaminergic neurons). A large accumulation of CDs was observed in the cytoplasm and nucleus, which indicated that CDs have good permeability of cell membrane and other intracellular organelle membranes. It could be applied to biological imaging of brain cells in the future (Pei et al., 2021). Besides. Wu et al. developed a novel two-photon fluorescent probe M9, which consists of graphene oxide (GO), red-emitting CDs, and azobenzene bound to DNA (DNA-Azo). For in situ imaging of miR-9 in living neurons and brain tissue of AD mice. It was found that the M9 fluorescent probe easily entered neuron cells and distributed them in the cytoplasm of neuron cells. These results shed light on understanding the genetic basis of AD and hold great promise for the early diagnosis of cancer and neurological disorders (Wu et al., 2020). In conclusion, due to the penetrability and good biocompatibility of CDs, they can provide a good possibility for developing effective methods for clinical diagnosis and treatment of the central nervous system.
2.2 Organelles Bioimaging
Nowadays, fluorescence imaging technology is becoming more and more mature, and more and more fluorescent probes for biological imaging have been developed, but the bioimaging of subcellular and organelles still needs further efforts to track changes in cell morphology or function. Biomaging of cell nuclei is crucial for revealing nuclear morphology and its role in cell metabolism, growth, differentiation, and inheritance (Zhou et al., 2020). Zhang et al. synthesized nitrogen doped CDs (N-CDs) by using citric acid as raw material and propylene diamine as a passivation agent. Then the N-CDs were used for HeLa cell staining, the results showed that N-CDs were located in the nucleus, showing a multicolor luminescence effect, and there was almost no blue-green-red fluorescence intensity in the cytoplasm (Figure 1C). The N-CDs will be automatically enriched in the nucleus, and the concentration in the cytoplasm is very low, and they could be a new way to track individual cells or visualize processes such as nuclear marker-based mitosis, which may provide new tools for tracking chromatin phase changes during cell cycle changes (Zhang et al., 2020).
Mitochondria are one of the most important subcellular compartments in the cell, the site of ATP production and the center of cellular metabolism. The state of mitochondria is associated with a variety of diseases, grasping the state of mitochondria plays a crucial role in the treatment of various diseases (Anqi Li et al., 2020; Mani et al., 2021). CDs can be a new mitochondrial imaging probe, a series of N-CDs were prepared by Kaminari et al. The N-CDs exhibit subcellular mitochondrial localization and compared to known mitochondrial probes, multi-functionalized N-CDs exhibit superior photostability, stable long-term mitochondrial imaging, and cell compatibility with apoptotic labeling potential (Figure 1D). Their uptake depends on mitochondrial membrane potential and induces their preferential localization in malignant cells, which is expected to serve as a carrier for mitochondria-targeted delivery of anticancer drugs (Kaminari et al., 2021). Guo et al. synthesized fluorescent CDs that could observe cell viability in situ by simple microwave-assisted synthesis. Due to electrostatic interactions, positively charged CDs tend to accumulate in mitochondria with high negative MMPs in healthy cells. When cells are damaged with a concomitant decrease in MMP levels, CDs migrate from the mitochondria to the nucleolus due to their binding affinity to nucleic acids. Once the cellular state is restored, MMP levels are again highly reduced, and the CDs are reversed back to the mitochondria. Therefore, the viability of cells can be easily observed through the different spatiotemporal distribution of CDs in living cells. The discovery of CDs has great potential in the study of cell survival (Guo et al., 2021).
The in vitro bioimaging demonstrate that CDs have broad application prospects in the biomedical field. The CDs can be used as fluorescent probes for cancer cell imaging and organelle targeting for the diagnosis and prevention of some major diseases. However, the current CDs-based fluorescent probes still have some defects, we should focus on developing fluorescent probes with better performance, such as precise targeting ability, high fluorescence quantum yield, high stability, simple surface functionalization, and so on. In addition, the targeting of CDs and the application of in vivo bioimaging should be further investigated in detail.
3 In Vivo Bioimaging
In recent years, in vivo biomedical applications based on fluorescent CDs have made many efforts for future clinical diagnosis and therapy. Yang et al. (2009) are the first to report in vivo imaging of CDs in mice via three injection routes. Since then, more and more animal models have been established to explore the imaging studies of CDs in vivo.
3.1 Biodistribution of CDs in Zebrafish and Mice
CDs play an important role in the medical field. In vivo imaging of CD may have chronic toxicity, low stability, and potential for accumulation. Therefore, the distribution and metabolism of CD in vivo should be assessed. Liu et al. synthesized F.nucleatum-CDs (Fn-CDs) for in vivo imaging in mice. The CDs were injected into mice and detected Fn-CDs in mice at different periods. At different periods, different parts of the mice appeared fluorescence successively. After that, the fluorescence intensity of Fn-CDs gradually decreased, indicating that Fn-CDs may have entered the blood circulation. What’s more, the fluorescence disappeared after 24 h, which manifesting that Fn-CDs can be excreted through digestive system metabolism, making Fn-CDs an excellent candidate for in vivo bioimaging and biosensing (Lijuan Liu et al., 2021). Besides, Liu et al. synthesized high-yield water-soluble CDs (WCDs) by the targeted method with good biocompatibility. The uptake and metabolism of WCDS in vivo were studied by zebrafish. When WCDs were cultured with zebrafish embryos, WCDs mainly existed in the yolk of the zebrafish embryonic stage. In adult zebrafish, green fluorescence is distributed in the intestine, stomach, liver and yolk sac (Figure 1E). By observing the fluorescence imaging of WCDs in zebrafish at different periods, the distribution and metabolism of WCDs in zebrafish can be observed (Zonglin Liu et al., 2021). These research works provide valuable information for the administration of CDs in vivo, monitoring the therapeutic effect, etc. By observing the distribution and metabolism of CDs in vivo, evaluating the toxicity of CDs in vivo, and reducing the potential cellularity of CDs through surface modification.
3.2 Fluorescent Bioimaging-Guided Drug Delivery System
The blood-brain barrier (BBB) is one of the most important factors limiting the development of treatments for neurological diseases and brain cancer. Many drugs cannot directly penetrate the BBB, resulting in very limited drug delivery systems (DDS) for the treatment of central nervous system-related diseases and brain cancer. Therefore, the development of new DDS is very necessary. CDs-mediated DDS has received extensive attention due to its penetration of the blood-brain barrier (Liyanage et al., 2020). A fluorescein carbon dots prepared from glucose (GluCDs-F) was synthesized by Seven et al. They tested the ability of GluCDs-F to cross the blood-brain barrier in zebrafish and rat models. After intravenous administration in rats, GluCDs-F was observed to concentrate in cervical spinal cord gray matter (e.g., ventral horn, dorsal horn, mid-gray) in the central nervous system, consistent with aggregation behavior in neurons. Therefore, GluCDs-F-targeted neurons have great potential as a drug delivery platform in neurodegenerative diseases, traumatic injuries, and central nervous system malignancies (Figure 1F). In addition, to enhance tumor-specific imaging and drug delivery, tumor drug molecule delivery systems can be realized by targeting probe-binding ligands that recognize receptor-like molecules (Seven et al., 2021). Li et al. reported large amino acid mimetic CDs (LAAM-CDs) for selective imaging and drug delivery to tumors, including brain tumors. The LAAM-CDs were used as a DDS utilizes specific carrier transporters that are differentially upregulated in cancer cells. LAAMTC-CDs, a type of LAAM-CDs, which were synthesized by using 1,4,5,8-tetraaminoanthraquinone (TAAQ) and citric acid (CA). Then LAAMTC-CDs were administered intravenously to patients with U87 glioma in mice and analyzed using near-infrared fluorescence imaging at different time points. The accumulation of LAAM TC-CDs in the brain increased over time by fluorescence imaging profiles and peaked at 8–12 h after injection when the fluorescence signal in the brain dominated. Therefore, LAAM-CDs had the potential to translate into clinical applications for imaging and drug delivery in various tumors and diseases of the central nervous system (Shuhua Li et al., 2020).
3.3 Fluorescent Bioimaging Guides the Treatment of Cancer and Kidney Disease
Cancer treatment is one of the biggest challenges facing the medical field today. Li et al. have explored watermelon-derived CDs with secondary near-infrared (NIR-II) emission as in vivo optical fluorescent agents, which in addition to their excellent optical properties, also possess excellent 808 nm laser-induced photothermal properties, photothermal therapy (PTT) for cancer and CD has a good biological function (Figure 1G). These studies verified the potential application of CDs in the treatment of cancer PTT (Li et al., 2019). Due to insufficient light-to-heat conversion, developing an ideal CDs is still difficult, requiring the aid of high-power-density lasers. Therefore, Kim et al. prepared sulfur-doped CDs (S-CDs) with strong near-infrared absorption ability using Japanese camellia as raw material by hydrothermal method. And the lower dose of S-CDs was found to have higher PTT performance (Kim et al., 2021). Acute kidney injury (AKI) is a reactive oxygen species (ROS)-promoted disease with high mortality and morbidity for which there is currently no effective drug treatment. Gao et al. prepared m-phenylenediamine-based CDs (PDA-CDs) with ultra-small-sized glomerular filtration barrier permeability and antioxidant properties, the PDA-CDs exhibited significant ROS scavenging in vitro and in vivo active. Both AKI mice and healthy mice were injected with PDA-CDs intravenously. Then major organs (heart, liver, spleen, lung, and kidney) were taken for fluorescent bioimaging of fluorescence-specific parameters of PDA-CDs. Normal mouse PDA-CDs mainly accumulated in the liver and kidney. In AKI mice, PDA-CDs mainly accumulated in the liver and kidney (Figure 1H), and after injection of 12 h, the accumulation of PDA-CDs in the kidneys of AKI mice reached a peak, which was much longer than that in normal kidneys. Meanwhile, by comparing with the cisplatin-induced AKI model, it was found that PDA-CDs exhibited significant therapeutic effects in both models. This provides an effective drug therapy strategy for ROS-induced AKI with significant clinical translation potential (Gao et al., 2020).
In a short, in vivo bioimaging based on CDs have broad application, the above work validates the ability of CDs to have potent chemotherapeutic effects, which can provide a strategy for the potential clinical application of CDs in image-guided tumor therapy, renal disease treatment and other diseases.
4 Conclusion and Future Prospects
Till now, CDs have already proven to be an intriguing class of nanoparticles, which have made significant achievements in the field of bioimaging due to their excellent fluorescence properties, good biocompatibility, low toxicity, high sensitivity, and easy surface functionalization. This review mainly summarizes recent advances in the field of CDs-based bioimaging in two aspects (Table 1): in vitro bioimaging of important organelles (nucleus, mitochondria) and in vivo bioimaging (mice, zebrafish). Although the CDs have shown many advantages in bioimaging, there are still some challenges: (1) How to synthesize CDs with excellent fluorescent properties by a simple synthesis method and explain the synthesis mechanism? (2) More experimental and theoretical studies should be carried out to elucidate the fluorescent mechanism of the CDs. (3) CDs with red or near-IR fluorescent emission are more suitable for bioimaging in vitro/vivo, it is very important to prepare CDs with red or near-IR fluorescent emission which have high quantum yield and excellent bioimaging performance. (4) With the advancement of bioimaging methods and equipment, efficient tissue penetration and in vivo bioimaging under low background fluorescence will continue to be achieved, which will further promote the development of CDs in bioimaging. We believe that with the continuous development of the field, the practical application of CDs in bioimaging will be greatly improved.
Author Contributions
CH and XL: Defined the focus of the review. YM, YL, MY, and YK: Writing. XY: Editing. WZ: Reviewing. QH and BZ: Writing, reviewing and editing.
Funding
This work has been supported by the National Natural Science Foundation of China (No. 82060599), the Natural Science Foundation of Jiangxi (No. 20202BABL213018), the Science and Technology Project of the Education Department of Jiangxi Province (Nos. GJJ190795, GJJ190775, GJJ190827 and GJJ211517), the Science and Technology Project of Jiangxi Health Committee (No. 202131033), the Research Fund of Gannan Medical University (Nos. ZD201901, YQ202003, YB201905, YB201931 and YB201911), the Open Project of Key Laboratory of Prevention and Treatment of Cardiovascular and Cerebrovascular Diseases, Ministry of Education (Nos. XN201911 and XN202017), the open fund of Fujian Provincial Key Laboratory of Modern Analytical Science and Separation Technology and the Science and Technology (No. K2017-01) and the Plan Post-subsidy Project of Ganzhou (No. 2019-60-174).
Conflict of Interest
The authors declare that the research was conducted in the absence of any commercial or financial relationships that could be construed as a potential conflict of interest.
Publisher’s Note
All claims expressed in this article are solely those of the authors and do not necessarily represent those of their affiliated organizations, or those of the publisher, the editors and the reviewers. Any product that may be evaluated in this article, or claim that may be made by its manufacturer, is not guaranteed or endorsed by the publisher.
References
Anqi Li, A., Gao, M., Jiang, W., Qin, Y., and Gong, G. (2020). Mitochondrial Dynamics in Adult Cardiomyocytes and Heart Diseases. Front. Cell Dev. Biol. 8, 584800. doi:10.3389/fcell.2020.584800
Ashrafizadeh, M., Mohammadinejad, R., Kailasa, S. K., Ahmadi, Z., Afshar, E. G., and Pardakhty, A. (2020). Carbon Dots as Versatile Nanoarchitectures for the Treatment of Neurological Disorders and Their Theranostic Applications: A Review. Adv. Colloid Interface Sci. 278, 102123. doi:10.1016/j.cis.2020.102123
Atchudan, R., Gangadaran, P., Edison, T. N. J. I., Perumal, S., Sundramoorthy, A. K., Vinodh, R., et al. (2022a). Betel Leaf Derived Multicolor Emitting Carbon Dots as a Fluorescent Probe for Imaging Mouse Normal Fibroblast and Human Thyroid Cancer Cells. Phys. E Low-dimensional Syst. Nanostructures 136, 115010. doi:10.1016/j.physe.2021.115010
Atchudan, R., Chandra Kishore, S., Gangadaran, P., Jebakumar Immanuel Edison, T. N., Perumal, S., Rajendran, R. L., et al. (2022b). Tunable Fluorescent Carbon Dots from Biowaste as Fluorescence Ink and Imaging Human Normal and Cancer Cells. Environ. Res. 204, 112365. doi:10.1016/j.envres.2021.112365
Bondon, N., Raehm, L., Charnay, C., Boukherroub, R., and Durand, J.-O. (2020). Nanodiamonds for Bioapplications, Recent Developments. J. Mat. Chem. B 8, 10878–10896. doi:10.1039/d0tb02221g
Cardoso Dos Santos, M., Algar, W. R., Medintz, I. L., and Hildebrandt, N. (2020). Quantum Dots for Förster Resonance Energy Transfer (FRET). TrAC Trends Anal. Chem. 125, 115819. doi:10.1016/j.trac.2020.115819
Chang, D., Zhao, Z., Niu, W., Shi, L., and Yang, Y. (2021). Iron Ion Sensing and In Vitro and In Vivo Imaging Based on Bright Blue-Fluorescent Carbon Dots. Spectrochimica Acta Part A Mol. Biomol. Spectrosc. 260, 119964. doi:10.1016/j.saa.2021.119964
Deling Li, D., Liu, Q., Qi, Q., Shi, H., Hsu, E. C., Chen, W., et al. (2020). Gold Nanoclusters for NIR‐II Fluorescence Imaging of Bones. Small 16, 2003851. doi:10.1002/smll.202003851
Desai, M. L., Jha, S., Basu, H., Singhal, R. K., Park, T.-J., and Kailasa, S. K. (2019). Acid Oxidation of Muskmelon Fruit for the Fabrication of Carbon Dots with Specific Emission Colors for Recognition of Hg2+ Ions and Cell Imaging. ACS Omega 4, 19332–19340. doi:10.1021/acsomega.9b02730
Ðorđević, L., Arcudi, F., Cacioppo, M., and Prato, M. (2022). A Multifunctional Chemical Toolbox to Engineer Carbon Dots for Biomedical and Energy Applications. Nat. Nanotechnol. 17, 112–130. doi:10.1038/s41565-021-01051-7
Gao, J., Liu, Y., Jiang, B., Cao, W., Kan, Y., Chen, W., et al. (2020). Phenylenediamine-based Carbon Nanodots Alleviate Acute Kidney Injury via Preferential Renal Accumulation and Antioxidant Capacity. ACS Appl. Mat. Interfaces 12, 31745–31756. doi:10.1021/acsami.0c05041
Ge, G., Li, L., Chen, M., Wu, X., Yang, Y., Wang, D., et al. (2022). Green Synthesis of Nitrogen-Doped Carbon Dots from Fresh Tea Leaves for Selective Fe3+ Ions Detection and Cellular Imaging. Nanomaterials 12 (6), 986. doi:10.3390/nano12060986
Ghosh, S., Gul, A. R., Park, C. Y., Xu, P., Baek, S. H., Bhamore, J. R., et al. (2021a). Green Synthesis of Carbon Dots from Calotropis Procera Leaves for Trace Level Identification of Isoprothiolane. Microchem. J. 167, 106272. doi:10.1016/j.microc.2021.106272
Ghosh, S., Gul, A. R., Park, C. Y., Kim, M. W., Xu, P., Baek, S. H., et al. (2021b). Facile Synthesis of Carbon Dots from Tagetes erecta as a Precursor for Determination of Chlorpyrifos via Fluorescence Turn-Off and Quinalphos via Fluorescence Turn-On Mechanisms. Chemosphere 279, 130515. doi:10.1016/j.chemosphere.2021.130515
Gudimella, K. K., Appidi, T., Wu, H.-F., Battula, V., Jogdand, A., Rengan, A. K., et al. (2021). Sand Bath Assisted Green Synthesis of Carbon Dots from Citrus Fruit Peels for Free Radical Scavenging and Cell Imaging. Colloids Surfaces B Biointerfaces 197, 111362. doi:10.1016/j.colsurfb.2020.111362
Guo, S., Sun, Y., Li, J., Geng, X., Yang, R., Zhang, X., et al. (2021). Fluorescent Carbon Dots Shuttling between Mitochondria and the Nucleolus for In Situ Visualization of Cell Viability. ACS Appl. Bio Mat. 4, 928–934. doi:10.1021/acsabm.0c01408
Huang, Q., Lin, X., Tong, L., and Tong, Q.-X. (2020). Graphene Quantum Dots/multiwalled Carbon Nanotubes Composite-Based Electrochemical Sensor for Detecting Dopamine Release from Living Cells. ACS Sustain. Chem. Eng. 8, 1644–1650. doi:10.1021/acssuschemeng.9b06623
Huang, Q., Lin, X., Chen, D., and Tong, Q.-X. (2022). Carbon Dots/α-Fe2O3-Fe3O4 Nanocomposite: Efficient Synthesis and Application as a Novel Electrochemical Aptasensor for the Ultrasensitive Determination of Aflatoxin B1. Food Chem. 373, 131415. doi:10.1016/j.foodchem.2021.131415
Jiao, Y., Liu, Y., Meng, Y., Gao, Y., Lu, W., Liu, Y., et al. (2020). Novel Processing for Color-Tunable Luminescence Carbon Dots and Their Advantages in Biological Systems. ACS Sustain. Chem. Eng. 8, 8585–8592. doi:10.1021/acssuschemeng.0c01016
Jin, L., Shao, Y., Chen, X., Wang, X., Wang, Q., Wang, Y., et al. (2022). Dual-Excitation Red-Emissive Carbon Dots Excited by Ultraviolet Light for the Mitochondria-Targetable Imaging and Monitoring of Biological Process in Living Cells. J. Photochem. Photobiol. A: Chem. 425, 113702.
Kailasa, S. K., Ha, S., Baek, S. H., Phan, L. M. T., Kim, S., Kwak, K., et al. (2019). Tuning of Carbon Dots Emission Color for Sensing of Fe3+ Ion and Bioimaging Applications. Mater. Sci. Eng. C 98, 834–842. doi:10.1016/j.msec.2019.01.002
Kailasa, S. K., and Koduru, J. R. (2022). Perspectives of Magnetic Nature Carbon Dots in Analytical Chemistry: From Separation to Detection and Bioimaging. Trends Environ. Anal. Chem. 33, e00153. doi:10.1016/j.teac.2021.e00153
Kaminari, A., Nikoli, E., Athanasopoulos, A., Sakellis, E., Sideratou, Z., and Tsiourvas, D. (2021). Engineering Mitochondriotropic Carbon Dots for Targeting Cancer Cells. Pharmaceuticals 14, 932. doi:10.3390/ph14090932
Kim, D., Jo, G., Chae, Y., Subramani, S., Lee, B. Y., Kim, E. J., et al. (2021). Bioinspired Camellia Japonica Carbon Dots with High Near-Infrared Absorbance for Efficient Photothermal Cancer Therapy. Nanoscale 13, 14426–14434. doi:10.1039/d1nr03999g
Kirbas Cilingir, E., Seven, E. S., Zhou, Y., Walters, B. M., Mintz, K. J., Pandey, R. R., et al. (2021). Metformin Derived Carbon Dots: Highly Biocompatible Fluorescent Nanomaterials as Mitochondrial Targeting and Blood-Brain Barrier Penetrating Biomarkers. J. Colloid Interface Sci. 592, 485–497. doi:10.1016/j.jcis.2021.02.058
Kong, T., Zhou, R., Zhang, Y., Hao, L., Cai, X., and Zhu, B. (2020). AS1411 Aptamer Modified Carbon Dots via Polyethylenimine-Assisted Strategy for Efficient Targeted Cancer Cell Imaging. Cell Prolif. 53, e12713. doi:10.1111/cpr.12713
Kuang, Y., Zhang, J., Xiong, M., Zeng, W., Lin, X., Yi, X., et al. (2020). A Novel Nanosystem Realizing Curcumin Delivery Based on Fe3O4@Carbon Dots Nanocomposite for Alzheimer's Disease Therapy. Front. Bioeng. Biotechnol. 8, 614906. doi:10.3389/fbioe.2020.614906
Li, Y., Bai, G., Zeng, S., and Hao, J. (2019). Theranostic Carbon Dots with Innovative NIR-II Emission for In Vivo Renal-Excreted Optical Imaging and Photothermal Therapy. ACS Appl. Mat. Interfaces 11, 4737–4744. doi:10.1021/acsami.8b14877
Li-ping Li, L.-p., Ren, X.-f., Bai, P.-r., Liu, Y., Xu, W.-y., Xie, J., et al. (2021). Near-infrared Emission Carbon Dots for Bio-Imaging Applications. New Carbon Mater. 36 (3), 632–638. doi:10.1016/s1872-5805(21)60041-0
Lijuan Liu, L., Zhang, S., Zheng, X., Li, H., Chen, Q., Qin, K., et al. (2021). Carbon Dots Derived from Fusobacterium Nucleatum for Intracellular Determination of Fe3+ and Bioimaging Both In Vitro and In Vivo. Anal. Methods 13, 1121–1131. doi:10.1039/d1ay00020a
Lin, H., Yang, L., Zhang, X., Liu, G., Zhuo, S., Chen, J., et al. (2020). Emerging Low‐Dimensional Nanoagents for Bio‐Microimaging. Adv. Funct. Mat. 30, 2003147. doi:10.1002/adfm.202003147
Lin, X., Mei, Y., He, C., Luo, Y., Yang, M., Kuang, Y., et al. (2021a). Electrochemical Biosensing Interface Based on Carbon Dots-Fe3O4 Nanomaterial for the Determination of Escherichia coli O157:H7. Front. Chem. 9, 769648. doi:10.3389/fchem.2021.769648
Lin, X., Xiong, M., Zhang, J., He, C., Ma, X., Zhang, H., et al. (2021b). Carbon Dots Based on Natural Resources: Synthesis and Applications in Sensors. Microchem. J. 160, 105604. doi:10.1016/j.microc.2020.105604
Liu, S., Zhong, Z., Zhang, C., Zhou, Y., Fu, C., and Xu, X. (2022). Targeted Therapy for the Treatment of Gliomas with Multifunctional Orange Emissive Carbon Dots. Nanoscale Adv. 4, 894–903. doi:10.1039/d1na00722j
Liyanage, P. Y., Zhou, Y., Al-Youbi, A. O., Bashammakh, A. S., El-Shahawi, M. S., Vanni, S., et al. (2020). Pediatric Glioblastoma Target-specific Efficient Delivery of Gemcitabine across the Blood-Brain Barrier via Carbon Nitride Dots. Nanoscale 12, 7927–7938. doi:10.1039/d0nr01647k
Mahani, M., Mahmoudi, F., Fassihi, J., Hasani, Z., and Divsar, F. (2021). Carbon Dots-Embedded N-Acetylneuraminic Acid and Glucuronic Acid-Imprinted Polymers for Targeting and Imaging of Cancer Cells. Microchim. Acta 188, 224. doi:10.1007/s00604-021-04876-0
Mani, S., Swargiary, G., Tyagi, S., Singh, M., Jha, N. K., and Singh, K. K. (2021). Nanotherapeutic Approaches to Target Mitochondria in Cancer. Life Sci. 281, 119773. doi:10.1016/j.lfs.2021.119773
Mchugh, K. J., Jing, L., Behrens, A. M., Jayawardena, S., Tang, W., Gao, M., et al. (2018). Biocompatible Semiconductor Quantum Dots as Cancer Imaging Agents. Adv. Mat. 30, 1706356. doi:10.1002/adma.201706356
Mei, Y., He, C., Zeng, W., Luo, Y., Liu, C., Yang, M., et al. (2022). Electrochemical Biosensors for Foodborne Pathogens Detection Based on Carbon Nanomaterials: Recent Advances and Challenges. Food Bioprocess Technol. 15, 498–513. doi:10.1007/s11947-022-02759-7
Mohapatra, D., Pratap, R., Pandey, V., Dubey, P. K., Agrawal, A. K., Parmar, A. S., et al. (2022). Tinospora Cordifolia Leaves Derived Carbon Dots for Cancer Cell Bioimaging, Free Radical Scavenging, and Fe3+ Sensing Applications. J. Fluoresc. 32, 275–292.
Molkenova, A., Shin, Y. C., Kang, M. S., Mulikova, T., Han, D.-W., and Atabaev, T. S. (2019). Gd2O3 Nanoparticles Coated with a Fluorescent Carbon Layer for Potential T1-Weighted Magnetic Resonance and Cells Imaging. Nanosci. Nanotechnol. Lett. 11, 813–817. doi:10.1166/nnl.2019.2973
Molkenova, A., Toleshova, A., Song, S.-J., Kang, M. S., Abduraimova, A., Han, D.-W., et al. (2020). Rapid Synthesis of Nontoxic and Photostable Carbon Nanoparticles for Bioimaging Applications. Mater. Lett. 261, 127012. doi:10.1016/j.matlet.2019.127012
Mulikova, T., Abduraimova, A., Molkenova, A., Em, S., Duisenbayeva, B., Han, D.-W., et al. (2021). Mesoporous Silica Decorated with Gold Nanoparticles as a Promising Nanoprobe for Effective CT X-Ray Attenuation and Potential Drug Delivery. Nano-Structures Nano-Objects 26, 100712. doi:10.1016/j.nanoso.2021.100712
Murali, G., Kwon, B., Kang, H., Modigunta, J. K. R., Park, S., Lee, S., et al. (2022). Hematoporphyrin Photosensitizer-Linked Carbon Quantum Dots for Photodynamic Therapy of Cancer Cells. ACS Appl. Nano Mat. 5, 4376–4385. doi:10.1021/acsanm.2c00443
Naixin Li, N., Lei, F., Xu, D., Li, Y., Liu, J., and Shi, Y. (2021). One-step Synthesis of N, P Co-doped Orange Carbon Quantum Dots with Novel Optical Properties for Bio-Imaging. Opt. Mater. 111, 110618. doi:10.1016/j.optmat.2020.110618
Olifirenko, V., Abduraimova, A., Kang, M. S., Raja, I. S., Duisenbayeva, B., Molkenova, A., et al. (2021). Potential Applicability of Polyethyleneimine PEI-Coated Eu2O3 and Dy2O3 Nanoparticles for Contrast Enhancement in Computed Tomography. Nano Express 2, 10022. doi:10.1088/2632-959x/abe343
Pei, Y., Chang, A.-Y., Liu, X., Wang, H., Zhang, H., Radadia, A., et al. (2021). Nitrogen-doped Carbon Dots from Kraft Lignin Waste with Inorganic Acid Catalyst and Their Brain Cell Imaging Applications. AIChE J. 67, e17132. doi:10.1002/aic.17132
Phukan, K., Sarma, R. R., Dash, S., Devi, R., and Chowdhury, D. (2022). Carbon Dot Based Nucleus Targeted Fluorescence Imaging and Detection of Nuclear Hydrogen Peroxide in Living Cells. Nanoscale Adv. 4, 138–149. doi:10.1039/d1na00617g
Rao, H., Liu, W., He, K., Zhao, S., Lu, Z., Zhang, S., et al. (2020). Smartphone-Based Fluorescence Detection of Al3+ and H2O Based on the Use of Dual-Emission Biomass Carbon Dots. ACS Sustain. Chem. Eng. 8, 8857–8867. doi:10.1021/acssuschemeng.0c03354
Seven, E. S., Seven, Y. B., Zhou, Y., Poudel-Sharma, S., Diaz-Rucco, J. J., Kirbas Cilingir, E., et al. (2021). Crossing the Blood-Brain Barrier with Carbon Dots: Uptake Mechanism and In Vivo Cargo Delivery. Nanoscale Adv. 3, 3942–3953. doi:10.1039/d1na00145k
Shuhua Li, S., Su, W., Wu, H., Yuan, T., Yuan, C., Liu, J., et al. (2020). Targeted Tumour Theranostics in Mice via Carbon Quantum Dots Structurally Mimicking Large Amino Acids. Nat. Biomed. Eng. 4, 704–716. doi:10.1038/s41551-020-0540-y
Sun, L., Liu, Y., Wang, Y., Xu, J., Xiong, Z., Zhao, X., et al. (2021). Nitrogen and Sulfur Co-doped Carbon Dots as Selective and Visual Sensors for Monitoring Cobalt Ions. Opt. Mater. 112, 110787. doi:10.1016/j.optmat.2020.110787
Vedhanayagam, M., Raja, I. S., Molkenova, A., Atabaev, T. S., Sreeram, K. J., and Han, D.-W. (2021). Carbon Dots-Mediated Fluorescent Scaffolds: Recent Trends in Image-Guided Tissue Engineering Applications. Int. J. Mol. Sci. 22, 5378. doi:10.3390/ijms22105378
Wang, B., and Lu, S. (2022). The Light of Carbon Dots: From Mechanism to Applications. Matter 5, 110–149. doi:10.1016/j.matt.2021.10.016
Wang, Y., Hong, F., Yu, L., Xu, H., Liu, G., Dong, X., et al. (2020a). Construction, Energy Transfer, Tunable Multicolor and Luminescence Enhancement of YF3:RE3+(RE=Eu, Tb)/carbon Dots Nanocomposites. J. Luminescence 221, 117072. doi:10.1016/j.jlumin.2020.117072
Wang, Y., Niu, J., Gao, X., and Zhang, Y. (2020b). Synergetic Tuning of Photocatalytic Activity and Photostability of Ag3PO4 via Yttrium Doping, Carbon Quantum Dots and BiVO4 for Atenolol Degradation. Appl. Surf. Sci. 533, 147458. doi:10.1016/j.apsusc.2020.147458
Wang, Y., Xia, K., Wang, L., Wu, M., Sang, X., Wan, K., et al. (2021). Peptide‐Engineered Fluorescent Nanomaterials: Structure Design, Function Tailoring, and Biomedical Applications. Small 17, 2005578. doi:10.1002/smll.202005578
Wen, L., Wen, C., Zhang, F., Wang, K., Yuan, H., and Hu, F. (2020). siRNA and Chemotherapeutic Molecules Entrapped into a Redox-Responsive Platform for Targeted Synergistic Combination Therapy of Glioma. Nanomedicine Nanotechnol. Biol. Med. 28, 102218. doi:10.1016/j.nano.2020.102218
Wu, J., Wang, Q., Dong, X., Xu, M., Yang, J., Yi, X., et al. (2021a). Biocompatible AIEgen/p-Glycoprotein siRNA@reduction-Sensitive Paclitaxel Polymeric Prodrug Nanoparticles for Overcoming Chemotherapy Resistance in Ovarian Cancer. Theranostics 11, 3710–3724. doi:10.7150/thno.53828
Wu, H., Pang, L.-F., Wei, N., Guo, X.-F., and Wang, H. (2021b). Nucleus-targeted N-Doped Carbon Dots via DNA-Binding for Imaging of Hypochlorous in Cells and Zebrafish. Sensors Actuators B Chem. 333, 129626. doi:10.1016/j.snb.2021.129626
Wu, W., Zheng, T., and Tian, Y. (2020). An Enzyme-free Amplification Strategy Based on Two-Photon Fluorescent Carbon Dots for Monitoring miR-9 in Live Neurons and Brain Tissues of Alzheimer's Disease Mice. Chem. Commun. 56, 8083–8086. doi:10.1039/d0cc01971b
Xu, X., Ray, R., Gu, Y., Ploehn, H. J., Gearheart, L., Raker, K., et al. (2004). Electrophoretic Analysis and Purification of Fluorescent Single-Walled Carbon Nanotube Fragments. J. Am. Chem. Soc. 126, 12736–12737. doi:10.1021/ja040082h
Yang, S.-T., Cao, L., Luo, P. G., Lu, F., Wang, X., Wang, H., et al. (2009). Carbon Dots for Optical Imaging In Vivo. J. Am. Chem. Soc. 131, 11308–11309. doi:10.1021/ja904843x
Yi, X., Zeng, W., Wang, C., Chen, Y., Zheng, L., Zhu, X., et al. (2022). A Step-by-step Multiple Stimuli-Responsive Metal-Phenolic Network Prodrug Nanoparticles for Chemotherapy. Nano Res. 15, 1205–1212. doi:10.1007/s12274-021-3626-2
Younis, M. R., He, G., Lin, J., and Huang, P. (2020). Recent Advances on Graphene Quantum Dots for Bioimaging Applications. Front. Chem. 8, 424. doi:10.3389/fchem.2020.00424
Yu, X.-W., Liu, X., Jiang, Y.-W., Li, Y.-H., Gao, G., Zhu, Y.-X., et al. (2022). Rose Bengal-Derived Ultrabright Sulfur-Doped Carbon Dots for Fast Discrimination between Live and Dead Cells. Anal. Chem. 94, 4243–4251. doi:10.1021/acs.analchem.1c04658
Zhang, Y., Zhang, X., Shi, Y., Sun, C., Zhou, N., and Wen, H. (2020). The Synthesis and Functional Study of Multicolor Nitrogen-Doped Carbon Dots for Live Cell Nuclear Imaging. Molecules 25, 306. doi:10.3390/molecules25020306
Zhang, K., Ma, G., Wang, H., Liang, Z., Zhou, L., and Yan, B. (2021). Protamine Assisted Rapid Synthesis of Carbon Dots for Living Nucleolus Imaging and Gene Delivery Applications. J. Mater Sci. 56, 4396–4406. doi:10.1007/s10853-020-05526-1
Zheng, X., Qin, K., He, L., Ding, Y., Luo, Q., Zhang, C., et al. (2021). Novel Fluorescent Nitrogen-Doped Carbon Dots Derived from Panax Notoginseng for Bioimaging and High Selectivity Detection of Cr6+. Analyst 146, 911–919. doi:10.1039/d0an01599g
Zhou, F., Zhang, K., Li, G., Gui, C., Hu, R., Li, S., et al. (2020). Keto-salicylaldehyde Azine: a Kind of Novel Building Block for AIEgens and its Application in Tracking Lipid Droplets. Mat. Chem. Front. 4, 3094–3102. doi:10.1039/d0qm00405g
Zonglin Liu, Z., Li, B., Shi, X., Li, L., Feng, Y., Jia, D., et al. (2021). Target-oriented Synthesis of High Synthetic Yield Carbon Dots with Tailored Surface Functional Groups for Bioimaging of Zebrafish, Flocculation of Heavy Metal Ions and Ethanol Detection. Appl. Surf. Sci. 538, 148118. doi:10.1016/j.apsusc.2020.148118
Keywords: in vitro bioimaging, in vivo bioimaging, fluorescence imaging, nanomedicine, carbon dots
Citation: He C, Lin X, Mei Y, Luo Y, Yang M, Kuang Y, Yi X, Zeng W, Huang Q and Zhong B (2022) Recent Advances in Carbon Dots for In Vitro/Vivo Fluorescent Bioimaging: A Mini-Review. Front. Chem. 10:905475. doi: 10.3389/fchem.2022.905475
Received: 27 March 2022; Accepted: 20 April 2022;
Published: 05 May 2022.
Edited by:
Dong-Wook Han, Pusan National University, South KoreaReviewed by:
Suresh Kumar Kailasa, Sardar Vallabhbhai National Institute of Technology Surat, IndiaCopyright © 2022 He, Lin, Mei, Luo, Yang, Kuang, Yi, Zeng, Huang and Zhong. This is an open-access article distributed under the terms of the Creative Commons Attribution License (CC BY). The use, distribution or reproduction in other forums is permitted, provided the original author(s) and the copyright owner(s) are credited and that the original publication in this journal is cited, in accordance with accepted academic practice. No use, distribution or reproduction is permitted which does not comply with these terms.
*Correspondence: Xiaofeng Lin, TGlueGZAZ211LmVkdS5jbg==; Weijia Zeng, emVuZ3dlaWppYTIwMTlAcXEuY29t; Qitong Huang, aHF0QGdtdS5lZHUuY24=; Bin Zhong, emhvbmdiaW5qb2VAMTYzLmNvbQ==