- 1Inner Mongolia Key Laboratory of Environmental Chemistry, College of Chemistry and Enviromental Science, Inner Mongolia Normal University, Hohhot, China
- 2College of Pharmacy, Inner Mongolia Medical University, Hohhot, China
Based on the aggregation-induced luminescence of glutathione-protected non-noble metal copper nanoparticles (GSH-CuNPs), a fluorescence method for the rapid detection of bismuth (Bi3 +) was developed. The fluorescence intensity of GSH-CuNP solution is good, and the fluorescence can be quenched in the presence of Bi3 +. Based on this principle, a fluorescence mean for the admeasurement of Bi3+was built. The linear range was 0–100 mmol/L, and the detection limit was 10 mmol/L. The method is simple, rapid, and selective and can be used for the qualitative detection of Bi3 +.
Introduction
Due to the serious impact of metal ions on the environment and human health, the detection of metal ions has been a hot research topic. (Brower et al., 1997; Aragay et al., 2011). These species come from anthropological and natural resources and are found in soil, reservoir water, and marine environments (Yoshizaki and Tomida, 2000; Gans et al., 2005; Ghaedi et al., 2009). They accumulate in plants, meat, and other foods (Gill, 1983; Oyaro et al., 2007; Sud et al., 2008). Through these different absorption channels, these ions can gather in the developing brain, destroy the secondary structure of proteins/peptides, and lead to debilitating diseases (Stohs and Bagchi, 1995; Järup, 2003; Sharma and Dietz, 2009). Specifically, the local accumulation of Zn2+ in the brain leads to the rapid induction of the amyloid structure in Alzheimer’s disease (Bush et al., 1994; Lee et al., 2004). Due to the obstinacy of heavy metal ions in the environment and the resistance to conventional filtration methods, heavy metal ion pollution has plagued most developed countries (Fu and Wang, 2011). The harmful effects of heavy metal ions have prompted extensive research on their sensitive detection (Alexander et al., 2000; Ferreira et al., 2004; Korolczuk et al., 2005; Lee et al., 2008; Li et al., 2012). Acute bismuth poisoning is mainly due to oral entry; the patient will have nausea, vomiting, salivation, tongue and throat pain, abdominal pain, diarrhea, black stool with blood, skin and mucous membrane bleeding, headache, spasm, etc. Liver and kidney damage can lead to jaundice, urine protein and tube type, and even acute liver and kidney failure. For those allergic to bismuth salt, fever, rash, acute hemolysis, and exfoliative dermatitis may occur after intramuscular injection. Long-term application of bismuth can cause multiple neuritis, stomatitis, gingival swelling, pigmentation of the oral mucosa, and a black line on the gingiva. The X-ray film of the long bone end of the patient showed a white band, which was similar to that of lead poisoning cases.
As the next generation of materials, nanomaterials have shown great application prospects in many fields, such as catalysis, photonics, environmental detection, biomedical diagnosis, and treatment (Nie et al., 2010; Chen et al., 2012; Lu and Yin, 2012; Schroeder et al., 2012). This prospect comes from the unique properties of these nanomaterials, such as their plasma and quantum confinement effects, which are largely dependent on morphology (such as shape and surface structure) (Xia et al., 2009). Fluorescent metal nanoclusters (Au, Ag, and CuNCs) have attracted much attention due to their ultra-small size, quantum confinement, high photostability, and biocompatibility (Zheng et al., 2004; Burda et al., 2005; Zhu et al., 2008; Xie et al., 2009; Bain et al., 2015; Barman et al., 2016; Jin et al., 2016; Bain et al., 2017; Chakraborty and Pradeep, 2017; Kundu and Patra, 2017; Cao et al. 2014). Water soluble CuNCs were prepared from tannic acid, and the fluorescence quenching induced by the electron transfer mechanism was used to realize the high sensitivity detection of Fe3 +. At present, the detection of metal ions by fluorescent nanoclusters is mostly based on the fluorescence quenching mechanism. Since there are many substances that inhibit fluorescence, fluorescence quenching is a common phenomenon and has a great influence on selectivity or sensitivity (Chen et al., 2016). Fluorescence AgNCs were synthesized with glutathione (GSH) as a protective agent, and S2− quenched the fluorescence of silver nanomaterials to detect S2−. In the work by Feng et al. (2015), branched polyethyleneimine (bpei)-terminated CuNCs were synthesized by using bpei as the template and ascorbic acid as the reducing agent. The results display that the luminous intensity of CuNCs reaches its maximum value at 360 and 430 nm. Even in a high-salt medium, it has great water solubility, high stability, and photostability. The results show that Fe3+can significantly quench the fluorescence of bpei CuNCs by electron transfer, while other common anions and cations have little effect on the luminous intensity of CuNCs. Based on these, an ultrasensitive and super selective Fe3+fluorescence sensor was built and applied for measure of Fe3+ in human urine and water.
In this experiment, Cu nanoparticles were prepared by an easy template method. GSH is used as the protective agent and template of CuNPs, and the synthesized high fluorescence CuNPs can detect bismuth ions. When bismuth ions were added into the system, the fluorescence of Cu nanoparticles was inhibited. Moreover, among the common inorganic metal cations and anions, only Bi3+ can trigger the significant fluorescence quenching effect of copper nanomaterials. The nanomaterial sensor has good linear range, stability, and high selectivity and has great potential in the field of metal ion sensing and detection.
Materials and Methods
Materials
Glutathione (GSH), CuSO4, Cu (NO3)2 3H2O, ZnSO4·7H2O (Analytically Pure, Tianjin FengChuan Chemical Reagent Technology Co., Ltd.), FeCl2·4H2O (Analytical Pure, Beijing Shangle Chemical Plant), Bi(NO3)3·3H2O, NaCl, KCl (Analytical Pure, Tianjin Beilian Fine Chemicals Development Co., Ltd.), Ni (NO3)2·6H2O (Analytical Pure, Beijing 5671 Chemical Plant), NaOH (Analytically Pure, Tianjin Shengao Chemical Reagent Co., Ltd.), MnSO4·H2O (Analytical Reagent, No.4 Chemical Plant, Chaoyang District, Beijing), Cd(NO3)2 (Analytical Pure, Beijing Chemical Plant), NH4Cl (Analytical Pure, Tianjin People’s Chemical Plant), Na2SiO3·9H2O (Analytical Pure, Chemical Plant of Beijing Yizhuang Middle School), NaNO2 (Analytical Reagent, Tianjin Public Private Joint Venture Chemical Reagent Factory No.1), KI (Analytical Reagent, Baoding Chemical Reagent Factory, Hebei Province), Na2SiO3·9H2O (Analytical Pure, Chemical Plant of Beijing Yizhuang Middle School), CoCl2·6H2O (Analysys, Shanghai Public Private Joint Venture China Trade Factory), NaF (Analytical Pure, Beijing Public and Private Chemical Plant), and ultrapure water (about 18.25 m Ω).
Instruments
JEOL-2100f high resolution transmission electron microscope (equipped with xflash, Bruker, Germany), 5030t X-ray spectrometer (Japan Electronics Co., Ltd.), F-4600 fluorescence spectrophotometer (Hitachi high tech company), PHS-3c pH meter (Shanghai Yidian Scientific Instrument Co., Ltd.), d1008 series handheld centrifuge (Beijing Dalong Xingchuang Experimental Instrument Co., Ltd.), zf-7a portable UV detection lamp (Shanghai Guanghao analyzer Co., Ltd.), manual (adjustable and fixed) pipette (Beijing Dalong Xingchuang Experimental Instrument Co., Ltd.), and HJ-4A constant temperature magnetic heating agitator (Jiangsu Jintan Honghua instrument factory).
Synthesis of Copper Nanomaterials
Cu nanoparticles were prepared by blending 0.5 ml 50 × 10−3 M Cu (NO3) 2 ·3H2O with 2.5 ml of 50 × 10−3 M glutathione (GSH) and 2.0 ml of water, adjusting to pH 2.7 with 2 M NaOH, and centrifuging for 20 min to obtain GSH-CuNPs. Finally, it was stored at 4°C for subsequent experiments.
Detection of a Trivalent Bismuth Ion
The prepared GSH-CuNPs were put into a 1.5 ml centrifuge tube at 450 μ L, and then 50 μ L Bi3+ ion solutions with different levels were added. The reaction time was 15 min at room temperature. The fluorescence intensity at 471 nm was measured.
Results and Discussion
Synthesis Principle of GSH-CuNPs
In this test, the synthesis method of GSH-CuNPs is shown in Figure 1. GSH-CuNPs with hyperfluorescence were prepared at room temperature with glutathione as the protective agent and reducing agent. When Bi3+ was added into the GSH-CuNP system, Bi3+ would destroy GSH-CuNPs and cause aggregation of GSH-CuNPs, resulting in fluorescence fading. Therefore, based on the trivalent bismuth ions, we can effectively inhibit the fluorescence of GSH-CuNPs so as to realize the detection of bismuth ions.
Characterization of Copper Nanomaterials
The fluorescence spectra of GSH-CuNPs are shown in Figure 2A. The emission and excitation of GSH-CuNPs are at 475 and 365 nm, respectively. As shown in Figure 2B, Cu nanoparticles have a small UV absorption spectrum, which also proves the successful synthesis of copper nanomaterials. Figure 2C exhibits the TEM image of GSH-CuNPs with small particle size and uniform distribution. Figure 2D exhibits the particle size spreading of GSH-CuNPs. The average diameter of GSH-CuNPs is about 17.0 ± 0.2 nm. These findings suggest that the synthesized GSH-CuNPs have the characteristics of great fluorescence, equality, dispersibility, and granule dimensions, indicating the successful synthesis of hyperfluorescence GSH-CuNPs.
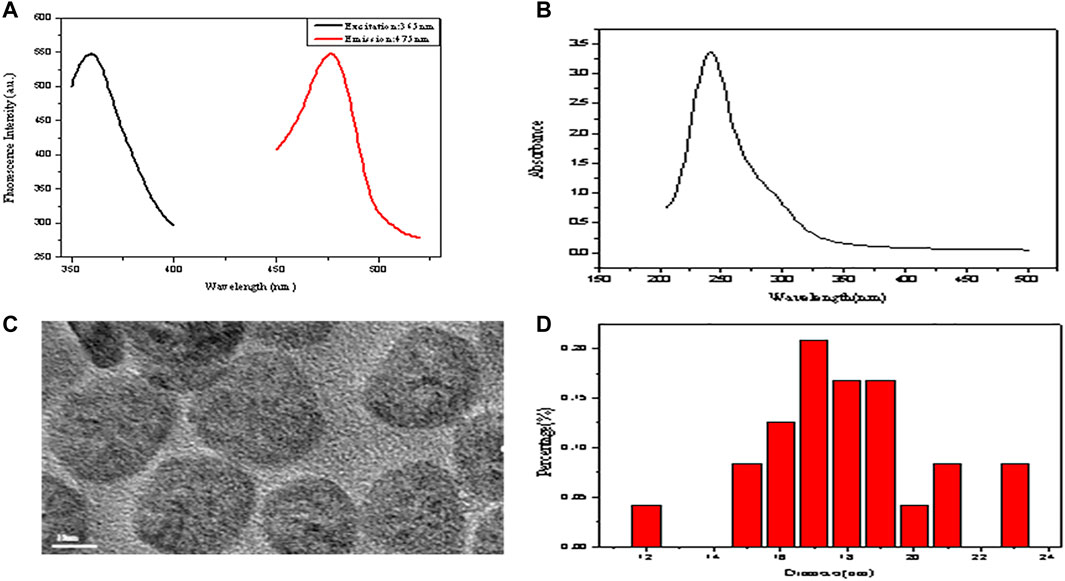
FIGURE 2. (A) Fluorescence spectra of GSH-CuNPs; (B) UV–vis spectra of GSH-CuNPs; (C) TEM image of GSH-CuNPs; (D) size-distribution histogram of GSH-CuNPs.
Stability of Copper Nanomaterials
We study the stability of GSH-CuNPs under different conditions. As revealed in Figure 3A, the fluorescence magnitude of GSH-CuNPs remains unchanged when a sodium chloride solution of different concentrations is added. As exhibited in Figure 3B, when the pH value of CuNPs was adjusted, it was found that the fluorescence magnitude of CuNPs was affected by pH. When pH is 2.7, GSH-CuNPs have a higher fluorescence magnitude. As shown in Figure 3C, the fluorescence intensity of GSH-CuNPs remains unchanged with time. As we can see, compound GSH-CuNPs can be stored for about 45 days at 4°C. The aforementioned data prove that GSH-CuNPs have good storage stability and good optical stability.
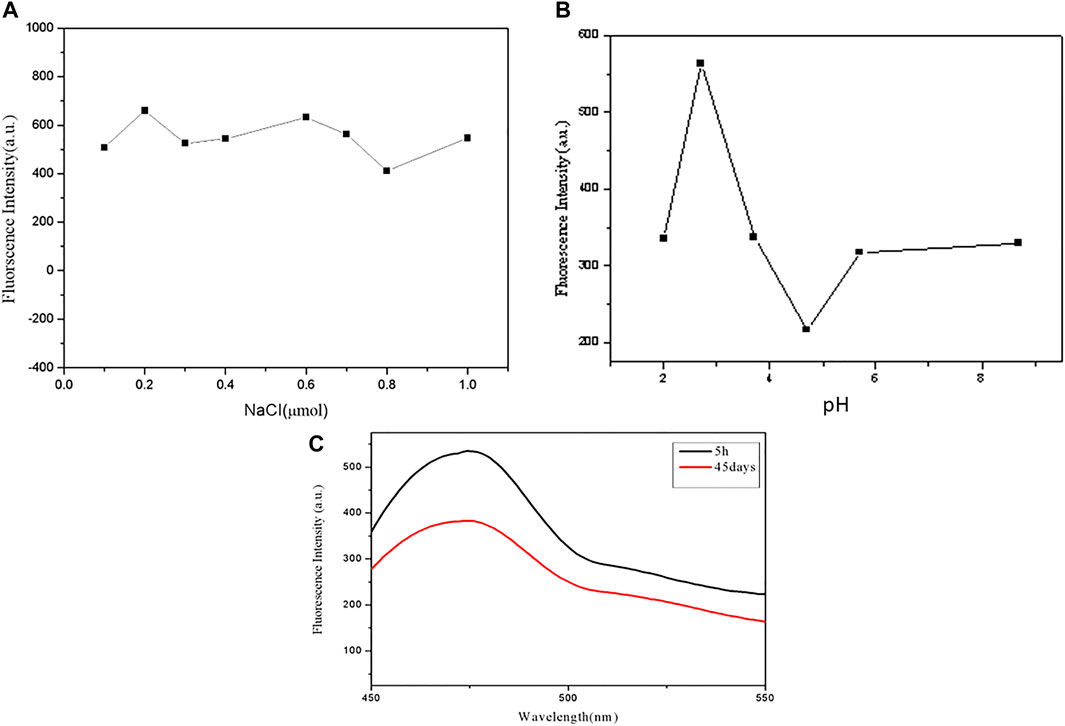
FIGURE 3. (A) Fluorescence magnitude of GSH-CuNPs; (B) effect of pH value on GSH-CuNP fluorescence intensity; (C) GSH-CuNPs can be stored for 1 month at 4°C.
Selectivity and Sensing Properties of Copper Nanomaterials
A series of different cations (such as CO2 +, Mg2+, Cd2 +, K+, Na+, Bi3+, and Fe3+) and anions (such as I- and F-) with identical concentration (100 mmol L−1) were tested. As shown in Figures 4A, B, Bi3+ can quench GSH-CuNPs, and the other ions have little effect on GSH-CuNPs. The results show that the technique has higher selectivity for the gauging of Bi3+.
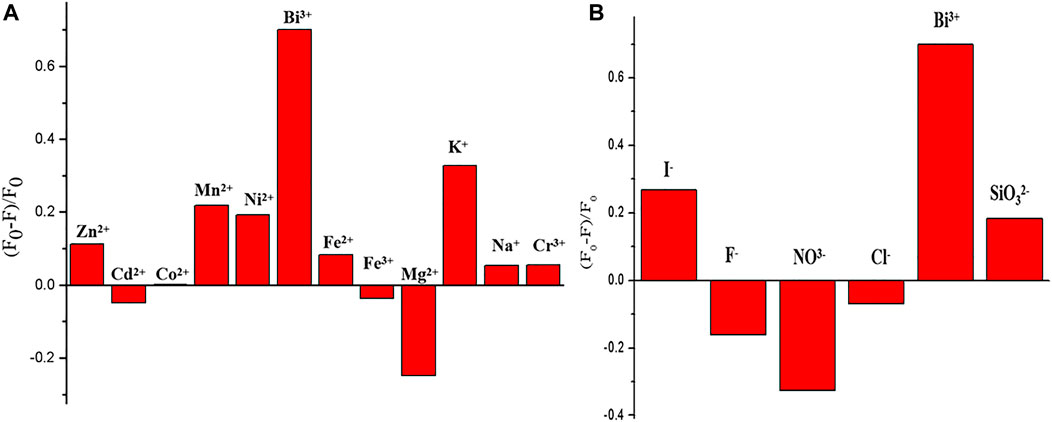
FIGURE 4. Fluorescence intensity of GSH-CuNPs at 475 nm under the existence of various cations (A) and anions (B).
Analysis of Sensing Properties of Copper Nanomaterials
In this trial run, a few bismuth ions with different levels were used for sensor analysis. As revealed in Figure 5A, the fluorescence of GSH-CuNPs was found by inch inhibition with the increase of bismuth ion concentration (0 mmol l−1–100 μ mol L−1). As revealed in Figure 5B, Bi3+ concentration has a good linear relationship in the range of 0 μ mol l−1–100 μ mol L−1 (R = 0.9495), and the detection limit is 10 mmol L−1.
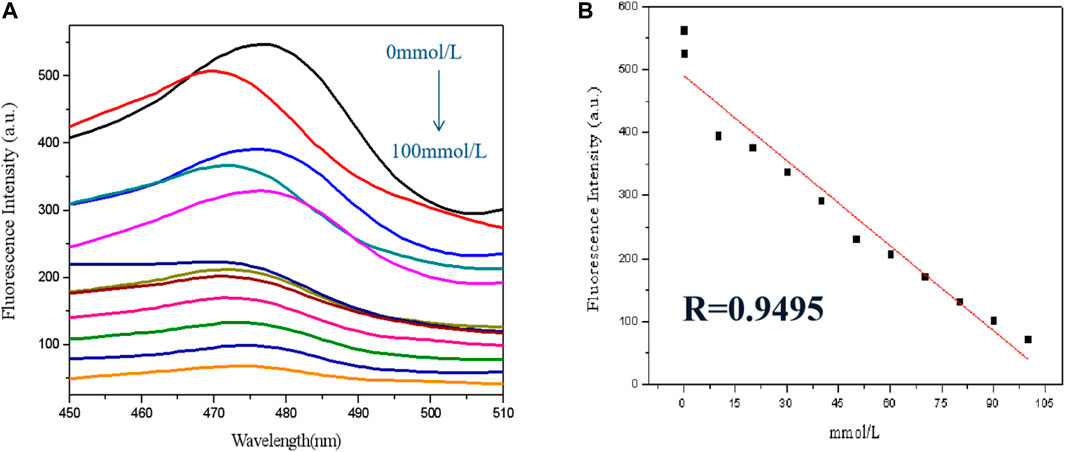
FIGURE 5. (A) GSH-CuNP fluorescence spectra with different concentration of bismuth ions; (B) linear relationship between fluorescence intensity of GSH-CuNPs and concentration of Bi3+.
Conclusion
In view of the fluorescence inhibition mechanization, a fluorescent analysis means for the detection of Bi3+ was built by using GSH-CuNPs as the fluorescence probe and Bi3+ as the quenching agent. With the increase of Bi3+ concentration, the fluorescence of Cu nanoparticles is inhibited in diverse degrees. The results showed that the fluorescence quenching linear relationship was good in the range of 0 μ mol l−1–100 μ mol L−1 (R = 0.9495). The GSH-CuNPs can be used for selective detection of bismuth ions.
Data Availability Statement
The original contributions presented in the study are included in the article/Supplementary Material, further inquiries can be directed to the corresponding authors.
Author Contributions
RW: preparation and characterization of materials and writing of the manuscript. LG: detection of bismuth ions and theoretical guidance. JA: writing and revising of the manuscript.
Funding
This work was supported by the National Natural Science Foundation of China (Grant No. 21864020), the Natural Science Foundation of Inner Mongolia (Grant No. 2018MS02012), the Collaborative Innovation Center for Water Environmental Security of Inner Mongolia Autonomous Region, China, (Grant No. XTCX003), and the Inner Mongolia Innovation Guide Project and Research Project of Higher School, Department of Education of Inner Mongolia Autonomous Region (Grant No. NJZC16047).
Conflict of Interest
The authors declare that the research was conducted in the absence of any commercial or financial relationships that could be construed as a potential conflict of interest.
Publisher’s Note
All claims expressed in this article are solely those of the authors and do not necessarily represent those of their affiliated organizations, or those of the publisher, the editors, and the reviewers. Any product that may be evaluated in this article, or claim that may be made by its manufacturer, is not guaranteed or endorsed by the publisher.
References
Alexander, D. C., Costanzo, M. A., Guzzo, J., Cai, J., Charoensri, N., Diorio, C., et al. (2000). Blazing towards the Next Millennium: Luciferase Fusions to Identify Genes Responsive to Environmental Stress. Water Air Soil Pollut. 123, 81–94. doi:10.1007/978-94-011-4369-1_8
Aragay, G., Pons, J., and Merkoçi, A. (2011). Recent Trends in Macro-, Micro-, and Nanomaterial-Based Tools and Strategies for Heavy-Metal Detection. Chem. Rev. 111, 3433–3458. doi:10.1021/cr100383r
Bain, D., Paramanik, B., and Patra, A. (2017). Silver(I)-Induced Conformation Change of DNA: Gold Nanocluster as a Spectroscopic Probe. J. Phys. Chem. C 121, 4608–4617. doi:10.1021/acs.jpcc.6b10560
Bain, D., Paramanik, B., Sadhu, S., and Patra, A. (2015). A Study into the Role of Surface Capping on Energy Transfer in Metal Cluster-Semiconductor Nanocomposites. Nanoscale 7, 20697–20708. doi:10.1039/c5nr06793f
Barman, M. K., Paramanik, B., Bain, D., et al. (2016). Light Harvesting and White-Light Generation in a Composite of Carbon Dots and Dye-Encapsulated BSA-Protein-Capped Gold Nanoclusters. Chem. Eur. [J] 22, 11699–11705. doi:10.1103/physrevlett.93.077402
Brower, J. B., Ryan, R. L., and Pazirandeh, M. (1997). Comparison of Ion-Exchange Resins and Biosorbents for the Removal of Heavy Metals from Plating Factory Wastewater. Environ. Sci. Technol. 31, 2910–2914. doi:10.1021/cr100383r
Burda, C., Chen, X., Narayanan, R., and El-Sayed, M. A. (2005). Chemistry and Properties of Nanocrystals of Different Shapes. Chem. Rev. 105, 1025–1102. doi:10.1021/cr030063a
Bush, A., Pettingell, W., Multhaup, G., d Paradis, M., Vonsattel, J., Gusella, J., et al. (1994). Rapid Induction of Alzheimer A Beta Amyloid Formation by Zinc. Science 265, 1464–1467. doi:10.1126/science.8073293
Cao, H., Chen, Z., Zheng, H., and Huang, Y. (2014). Copper Nanoclusters as a Highly Sensitive and Selective Fluorescence Sensor for Ferric Ions in Serum and Living Cells by Imaging. Biosens. Bioelectron. 62, 189–195. doi:10.1016/j.bios.2014.06.049
Chakraborty, I., and Pradeep, T. (2017). Atomically Precise Clusters of Noble Metals: Emerging Link between Atoms and Nanoparticles. Chem. Rev. 117, 8208–8271. doi:10.1021/acs.chemrev.6b00769
Chen, D. J., Li, S. X., and Zheng, F. Y. (2016). Water Soluble Sulphur Quantum Dots for Selective Ag+ Sensing Based on the Ion Aggregation-Induced Photoluminescence Enhancement. Biosens. Bioelectron. 8, 632–636. doi:10.1016/j.bios.2014.06.049
Chen, X.-J., Sanchez-Gaytan, B. L., Qian, Z., and Park, S.-J. (2012). Noble Metal Nanoparticles in DNA Detection and Delivery. WIREs Nanomed Nanobiotechnol 4, 273–290. doi:10.1002/wnan.1159
Fan, J. A., Wu, C., Bao, K., Bao, J., Bardhan, R., Halas, N. J., et al. (2010). Self-Assembled Plasmonic Nanoparticle Clusters. Science 328, 1135–1138. doi:10.1126/science.1187949
Feng, J., Ju, Y., Liu, J., Zhang, H., and Chen, X. (2015). Polyethyleneimine-templated Copper Nanoclusters via Ascorbic Acid Reduction Approach as Ferric Ion Sensor. Analytica Chim. Acta 854 (22), 153–160. doi:10.1016/j.aca.2014.11.024
Ferreira, S. L. C., dos Santos, H. C., Costa, A. C. S., and de la Guardia, M. (2004). Procedures of Separation and Pre‐concentration for Molybdenum Determination Using Atomic Spectrometry-A Review. Appl. Spectrosc. Rev. 39, 457–474. doi:10.1081/asr-120037147
Fu, F., and Wang, Q. (2011). Removal of Heavy Metal Ions from Wastewaters: A Review. J. Environ. Manage. 92, 407–418. doi:10.1016/j.jenvman.2010.11.011
Gans, J., Wolinsky, M., and Dunbar, J. (2005). Computational Improvements Reveal Great Bacterial Diversity and High Metal Toxicity in Soil. Science 309, 1387–1390. doi:10.1126/science.1112665
Ghaedi, M., Shokrollahi, A., Niknam, K., et al. (2009). Cloud point Extraction and Flame Atomic Absorption Spectrometric Determination of Cadmium (II), lead (II), Palladium (II) and Silver (I) in Environmental Samples. Hazard. Mater. 168, 1022–1390. doi:10.1126/science.1112665
Gill, P. G. K. (1983). Surface Interaction Model for Tracemetal Toxicity to Fishes: Role of Complexation, pH, and Water Hardness. Environ. Sci. Technology 17, 342–347.
Järup, L. (2003). Hazards of Heavy Metal Contamination. Br. Med. Bull. 68, 167–182. doi:10.1093/bmb/ldg032
Jin, R., Zeng, C., Zhou, M., and Chen, Y. (2016). Atomically Precise Colloidal Metal Nanoclusters and Nanoparticles: Fundamentals and Opportunities. Chem. Rev. 116, 10346–10413. doi:10.1021/acs.chemrev.5b00703
Korolczuk, M., Moroziewicz, A., and Grabarczyk, M. (2005). Determination of Subnanomolar Concentrations of Cobalt by Adsorptive Stripping Voltammetry at a Bismuth Film Electrode. Anal. Bioanal. Chem. 382, 1678–1682. doi:10.1007/s00216-005-3358-2
Kundu, S., and Patra, A. (2017). Nanoscale Strategies for Light Harvesting. Chem. Rev. 117, 712–757. doi:10.1021/acs.chemrev.6b00036
Lee, J.-Y., Friedman, J. E., Angel, I., Kozak, A., and Koh, J.-Y. (2004). The Lipophilic Metal Chelator DP-109 Reduces Amyloid Pathology in Brains of Human β-amyloid Precursor Protein Transgenic Mice. Neurobiol. Aging 25, 1315–1321. doi:10.1016/j.neurobiolaging.2004.01.005
Lee, J. H., Wang, Z., Liu, J., and Lu, Y. (2008). Highly Sensitive and Selective Colorimetric Sensors for Uranyl (UO22+): Development and Comparison of Labeled and Label-free DNAzyme-Gold Nanoparticle Systems. J. Am. Chem. Soc. 130, 14217–14226. doi:10.1021/ja803607z
Li, N., Zhang, L., Chen, Y., Fang, M., Zhang, J., and Wang, H. (2012). Highly Efficient, Irreversible and Selective Ion Exchange Property of Layered Titanate Nanostructures. Adv. Funct. Mater. 22 (4), 835–841. doi:10.1002/adfm.201102272
Lu, Z., and Yin, Y. (2012). Colloidal Nanoparticle Clusters: Functional Materials by Design. Chem. Soc. Rev. 41, 6874–6887. doi:10.1039/c2cs35197h
Nie, Z., Petukhova, A., and Kumacheva, E. (2010). Properties and Emerging Applications of Self-Assembled Structures Made from Inorganic Nanoparticles. Nat. Nanotech 5, 15–25. doi:10.1038/nnano.2009.453
Oyaro, N., Juddy, O., Murago, E., et al. (2007). The Contents of Pb, Cu, Zn and Cd in Meat in Nairobi, Kenya. Int. Food Agric. Environ. 5, 119–121.
Schroeder, A., Heller, D. A., Winslow, M. M., Dahlman, J. E., Pratt, G. W., Langer, R., et al. (2012). Treating Metastatic Cancer with Nanotechnology. Nat. Rev. Cancer 12, 39–50. doi:10.1038/nrc3180
Sharma, S. S., and Dietz, K.-J. (2009). The Relationship between Metal Toxicity and Cellular Redox Imbalance. Trends Plant Sci. 14, 43–50. doi:10.1016/j.tplants.2008.10.007
Stohs, S., and Bagchi, D. (1995). Oxidative Mechanisms in the Toxicity of Metal Ions. Free Radic. Biol. Med. 18, 321–336. doi:10.1016/0891-5849(94)00159-h
Sud, D., Mahajan, G., and Kaur, M. (2008). Agricultural Waste Material as Potential Adsorbent for Sequestering Heavy Metal Ions from Aqueous Solutions - A Review. Bioresour. Technology 99, 6017–6027. doi:10.1016/j.biortech.2007.11.064
Xia, Y., Xiong, Y., Lim, B., and Skrabalak, S. E. (2009). Shape-Controlled Synthesis of Metal Nanocrystals: Simple Chemistry Meets Complex Physics? Angew. Chem. Int. Ed. 48, 60–103. doi:10.1002/anie.200802248
Xie, J., Zheng, Y., and Ying, J. Y. (2009). Protein-Directed Synthesis of Highly Fluorescent Gold Nanoclusters. J. Am. Chem. Soc. 131, 888–889. doi:10.1021/ja806804u
Yoshizaki, S., and Tomida, T. (2000). Principle and Process of Heavy Metal Removal from Sewage Sludge. Environ. Sci. Technol. 34, 1572–1575. doi:10.1021/es990979s
Zheng, J., Zhang, C., and Dickson, R. M. (2004). Highly Fluorescent, Water-Soluble, Size-Tunable Gold Quantum Dots. Phys. Rev. Lett. 93, 077402. doi:10.1103/PhysRevLett.93.077402
Keywords: Bi3+, detection, copper nanomaterials, fluorescence, glutathione
Citation: Wu R, Ai J and Ga L (2022) Synthesis of Fluorescent Copper Nanomaterials and Detection of Bi3+. Front. Chem. 10:899672. doi: 10.3389/fchem.2022.899672
Received: 19 March 2022; Accepted: 31 March 2022;
Published: 25 May 2022.
Edited by:
Muhammad Asif, Wuhan Institute of Technology, ChinaCopyright © 2022 Wu, Ai and Ga. This is an open-access article distributed under the terms of the Creative Commons Attribution License (CC BY). The use, distribution or reproduction in other forums is permitted, provided the original author(s) and the copyright owner(s) are credited and that the original publication in this journal is cited, in accordance with accepted academic practice. No use, distribution or reproduction is permitted which does not comply with these terms.
*Correspondence: Jun Ai, imacaj01@163.com; Lu Ga, 13404832082@163.com