- 1Institute of Information Technology, Shenzhen Institute of Information Technology, Shenzhen, China
- 2School of Advanced Materials, Peking University Shenzhen Graduate School, Peking University, Shenzhen, China
- 3Division of Physics and Applied Physics, School of Physical and Mathematical Sciences, Nanyang Technological University, Singapore, Singapore
- 4School of Electronics and Information Technology, Sun Yat-sen University, Guangzhou, China
Organic light-emitting diodes (OLEDs) have great potential for display, lighting, and near-infrared (NIR) applications due to their outstanding advantages such as high efficiency, low power consumption, and flexibility. Recently, it has been found that the ultrathin emitting nanolayer technology plays a key role in OLEDs with simplified structures through the undoped fabricated process, and exciplex-forming hosts can enhance the efficiency and stability of OLEDs. However, the elementary structure and mechanism of the energy transfer process of ultrathin emitting nanolayers within interface exciplexes are still unclear. Therefore, it is imminently needed to explore the origin of ultrathin emitting nanolayers and their energy process within exciplexes. Herein, the mechanism of films growing to set ultrathin emitting nanolayers (<1 nm) and their energy transfer process within interface exciplexes are reviewed and researched. The UEML phosphorescence dye plays a key role in determining the lifetime of excitons between exciplex and non-exciplex interfaces. The exciplex between TCTA and Bphen has longer lifetime decay than the non-exciplex between TCTA and TAPC, facilitating exciton harvesting. The findings will be beneficial not only to the further development of OLEDs but also to other related organic optoelectronic technologies.
Introduction
Organic light-emitting diodes (OLEDs) are considered and applied as a feasible technology in high-quality display, solid-state lighting sources (SSLs), and near-infrared (NIR) applications, owing to the excellent advantages including high efficiency, low power consumption, and flexibility. (Baek et al., 2020; Helander et al., 2011; Wang et al., 2011; Wang et al., 2020; Xu et al., 2017; Greiner et al., 2012; Zheng et al., 2013; Xu et al., 2021a). Nevertheless, previous studies usually adopted complicated fabricated processes and device structures of OLEDs, which impede the popularizing of this promising technology. (Zhu et al., 2011; Gao et al., 2020; Yuan et al., 2020; Xu et al., 2021a). Therefore, simplifying the OLEDs is a challenge.
Recently, the ultrathin emitting layer (UEML) structure shows superiority applied in simply fabricating OLEDs without the doping process. (Zhao et al., 2011; Liu et al., 2014a; Liu et al., 2014b; Zhang et al., 2015; Wu et al., 2016; Xu et al., 2016; Xu et al., 2018a; Xu et al., 2018b; Luo et al., 2018; Luo et al., 2019a). To boost the efficiency of OLEDs, an exciplex is applied due to its promoting energy transfer between the host and guest. (Xu et al., 2017a; Li et al., 2019; Li and Liao, 2019; Xu et al., 2019; Xu et al., 2021b; Huang et al., 2021). The other exciton management strategies and energy transfer processes have been developed. Triplet–triplet annihilation (TTA) was proposed as another mechanism for the triplet harvesting process in TTA-dominant exciplex-emitting OLEDs. (Jankus et al., 2013; Kim and Kim, 2019). Furthermore, inspired by exciplex-based OLEDs, the novel concept of long-persistent luminescence has been confirmed by Adachi and co-workers. (Tan et al., 2021). Sandwiching the UEML between the exciplex interface of the hole transporting layer (HTL) and electron transport layer (ETL) not only realizes high luminous efficiency due to improved carrier injection and promoted exciton harvesting, (Xu et al., 2017b) but also restrains efficiency roll-off, (Yao et al., 2019; Zhang et al., 2021a; Xu et al., 2021c) and even extends the working lifetime, owing to good exciton management. (Li and Liao, 2019; Wei et al., 2020; Zhang et al., 2020; Zhang et al., 2021b). In other words, the UEML technology plays an important role in OLEDs with a simplified structure by the undoped fabricated process, while exciplex-forming hosts can help enhance the efficiency and stability of OLEDs.
To date, the elementary structure and mechanism of the energy transfer process of ultrathin emitting nanolayers within interface exciplexes are still unclear. Therefore, it is imminently needed to explore the origin of ultrathin emitting nanolayers and their energy process within exciplexes. In the perspective of growth kinetics of ultrathin organic films (<1 nm), the elementary processes of organic ultrathin growth include nucleation, aggregation, and coalescence of islands. (Winkler and Wandelt, 2018). Between the deposit and substrate, three growth relations are subsistent: 1) non-oriented, 2) texture orientation, and 3) texture and azimuthal orientation. (Sitter et al., 2008). Five modes of crystal growth can be distinguished: Volmer–Weber mode (VW-mode), the Frank–van der Merwe mode (FM-mode), the Stranski–Krastanov mode (SK-mode), the columnar growth mode (CG-mode), and the step flow mode (SF-mode).
Organic islands and discontinuous films are emerged by thin films which grow in sketch diagram modes of the VW-mode, FM-mode, and SK-mode, which play a leading role in the ultrathin organic film exhibited in Figure 1A. (Kaganer et al., 2009). As to the energy transfer process of the interface exciplex with a charge transfer (CT) state, type A and type B of the interface exciplex are classified according to direct contact or not shown in Figure 1B. The type A interface exciplex can transfer energy to the UEML within the type B interface exciplex via the Förster energy transfer. (Schleifenbaum et al., 2014; Becker et al., 2006; Kaur et al., 2020; Cortes and Jacob, 2018; Jones and Bradshaw, 2019; Sanz-Paz et al., 2020). The direct contact (type A) of different organic materials (hole transport material (HTM) and electron transport material (ETM)) could form the exciplex. Not all random combinations of the HTM and ETM can form an exciplex. The formation of the exciplex usually can be confirmed by photoluminescence (PL) measurement of mixing of films of the HTM and ETM to verify the CT state of the HTM and ETM. Generally, the interface exciplex could be composed of an HTM and an ETM, working as the electron donor and the electron acceptor, respectively. The basic working principle of this organic heterojunction attracted lots of research attention. The diffusion mechanism of exciplexes is studied by time-resolved photoluminescence (TRPL) spectroscopy by J.J. Kim. (Kim and Kim, 2020). The amorphous thin films of TADF donor–acceptor (D-A) exciplexes are observed under near-infrared excitation with the maximum distance of ∼6.9 nm for two photon-excited exciplex formations. (Chen et al., 2021). Long-range coupling of electron-hole pairs in spatially separated electron-donating and electron-accepting molecules as long as 10 nm spacer layers is reported, which is similar to type B exhibited in Figure 1B. (Ingram et al., 2014; Ingram et al., 2016; Nakanotani et al., 2016). However, why the interface of the exciplex produces these positive results to the UEML and the origin of the undoped UEML within interface exciplexes is still unexplored. (Sitter et al., 2008). Herein, the origin of ultrathin emitting nanolayers within interface exciplexes or non-exciplexes is reviewed and researched.
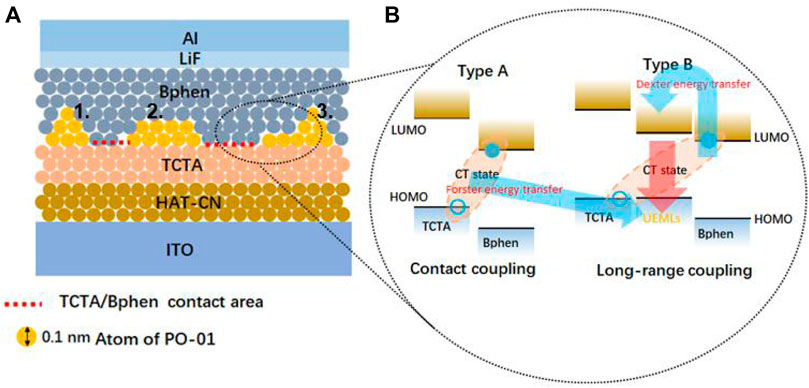
FIGURE 1. (A) Thin films grow sketch diagram modes of ultrathin emitting nanolayers (1. Volmer–Weber, 2. Frank–van der Merwe, and 3. Stranski–Krastanov) and (B) the ultrathin emitting nanolayers and their energy processes within the exciplex (Type A. and Type B.).
Experiment Details
Material and sample preparation: 4,7-Diphenyl-1,10-phenanthroline (Bphen), 4,4′,4″-Tris (carbazol-9-yl)-triphenylamine (TCTA), IridiuM(III) bis(4-phenylthieno [3,2-c]pyridinato-N,C2′)acetylacetonate (PO-01), and Di-[4-(N,N-ditolyl-amino)-phenyl] cyclohexan (TAPC) were purchased from Xi’an Polymer Light Technology Corp and used as received. Acetone and ethanol were consecutively used to clean the quartz substrate with an ultrasonic bath. The quartz substrates were further dried with a N2 flow. After 20 min of ultraviolet light–ozone treatment, later, the samples (A, B, C, and D) were vacuum deposited under 10−5 mbar with corresponding thickness and functional materials showing in Table 1, in order to probe the exciplex/non-exciplex interface and phosphorescence UEMLs within the exciplex/non-exciplex interface. The formation of interface exciplexes has been confirmed between TCTA and Bphen (Zhao et al., 2017) while TCTA and TAPC both working as the HTM cannot form interface exciplexes as a control group. The tested sample was grown on a quartz substrate. In order to accurately control the evaporation of ultrathin films, we adopted physical vapor deposition to observe the film detecting growth rate using quartz monitor crystals and termly calibrated the film thickness.
Photoluminescence measurement: Time-integrated PL measurement was conducted by directing the excitation laser pulses to thin films. The PL was measured at a backscattering angle of 145° by two lenses via an optical fiber coupled by using a spectrometer (Acton, Spectra Pro 2500i) and a charge-coupled device (CCD) (Princeton Instruments, Pixis 400B). TRPL was collected using an Optronis OptoScope streak camera system with an ultimate temporal resolution of 10 ps. The pump pulses were generated from an optical parametric amplifier (Coherent OPerA Solo) pumped by a 1-kHz regenerative amplifier (Coherent Libra, 800 nm, 50 fs). The amplifier was seeded by a mode-locked Ti: sapphire oscillator (Coherent Vitesse, 100 fs, 80 MHz). The excitation wavelength adapted for PL measurement was set to 380 nm. The fluorescence spectrum of the sample was measured at room temperature.
Results and Discussion
Energy transfer processes of UEMLs within the exciplex interface TCTA/Bpehn and the non-exciplex interface TCTA/TAPC are summarized, as shown in Figures 2A, B, respectively, dividing into type A contact coupling with UEMLs and type B long-range coupling without UEMLs. Three consecutive steps of the energy transfer process of UEMLs is included: 1) pumping electrons in the ground state to electrons in the CT state (exciton generation; T0∼100 fs), 2) Förster and Dexter energy transfer to UEMLs (energy transfer; τ1∼100 ps), and 3) luminescence of UEMLs (relaxation luminescence; τ2∼100 ns), with a distinguishing time scale is described with the energy structure of different organic interfaces in Figure 2. (Ingram et al., 2014; Menke and Holmes, 2014; Gould et al., 1994). The energy level diagrams of organic heterojunction interfaces are also exhibited in Figure 2. The test sample of TAPC/Bphen exhibited exciplex emission, which is similar to the results reported in the literature. (Zhao et al., 2017).
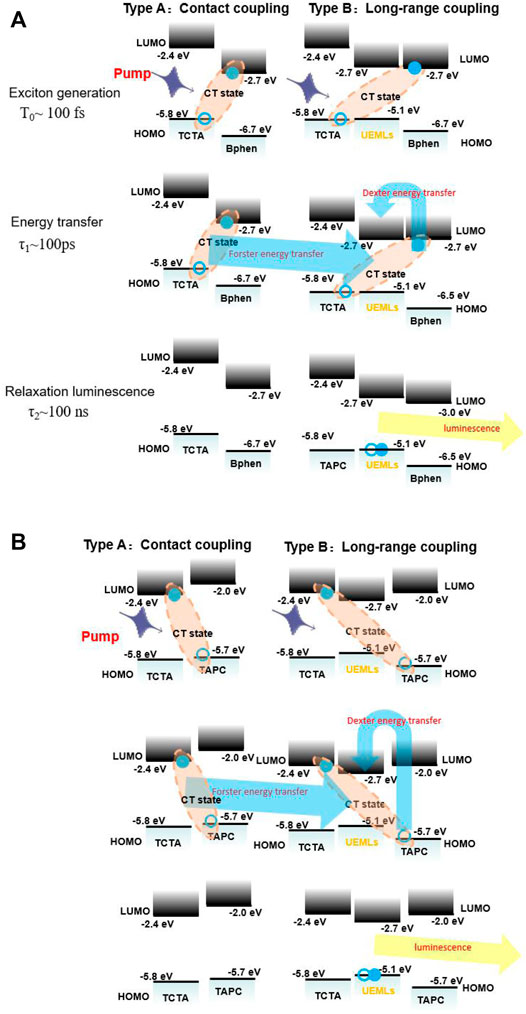
FIGURE 2. Energy transfer process of ultrathin emitting nanolayers within the exciplex interface (A) TCTA/Bphen and the non-exciplex interface (B) TCTA/TAPC.
In general, the relatively intense absorption (Abs.) with a wavelength from 300 to 400 nm is due to the absorption of TAPC, TCTA, and Bphen. The larger band gap of Bphen (∼4 eV) than that of TCTA (∼3.7 eV) strengthens the absorption of samples A and C under 300 nm, compared with the samples B and D, as shown in Figure 3A. There are slight stronger Abs. of sample A, compared with that of sample C due to adding UEML of PO-01. This UEML of PO-01 led to the PL peak with an emission of about 560 nm, implying the energy of exciton transfer from the interface of TAPC and Bphen to UEML, as shown in Figure 3B.
The energy gap (Eg) of exciplexes is generally determined by the energy distinction between the highest occupied molecular orbital (HOMO) of the HTM and the lowest unoccupied molecular orbital (LUMO) of the ETM. (Liu et al., 2013; Liu et al., 2016; Luo et al., 2017; Xiao et al., 2018a). Therefore, the emission peaks of exciplexes can be obtained via the energy distinction. From Figure 2, it is noted that Eg of TCTA and Bpehn (sample C) and Eg of TCTA and TAPC (sample D) are 3.1 and 3.3 eV, corresponding to the wavelength of ∼400 and ∼376 nm, respectively. The strong interface exciplex Abs. of TCTA/Bpehn is observed from 400 to 450 nm in Figure 3A. The wavelength of ∼400 nm (Eg of TCTA/Bpehn) and ∼376 nm (Eg of TCTA/TAPC) are comparatively correlated with the tendency of normalized Abs. (%) of sample C with a blue line and sample D with a red line, as shown in Figure 3A. Obviously, the lifetime decay of samples A and B presents a typical timescale of phosphorescence due to the PL spectra from UEML of PO-01, as shown in Figure 4.
To examine the energy transfer of excitons, transient PL decay behaviors of four samples were explored. The corresponding spectra and fitting data based on I(t) = A1exp (-t/τ1)+A2exp (-t/τ2) are shown in Figure 4 and summarized in Table 2, respectively. It can be seen that TCTA/TAPC and TCTA/Bphen with the similar peak PL emission wavelength at 297 nm showed a comparable lifetime, which is much shorter than samples with PO-01 due to the strong influence of the phosphorescence dye. Furthermore, the PL lifetime of the exciplex between TCTA and Bphen is a little longer than that of the non-exciplex between TCTA and TAPC, facilitating exciton harvesting. Therefore, such findings are believed to provide guidelines to develop high-performance OLEDs and other related optoelectronic devices, particularly for the enhancement of the device performance from the perspective of the innovation of interface engineering. (Xiao et al., 2018b; Xiao et al., 2018c; Luo et al., 2019b).
Conclusion
In summary, we reviewed growth kinetics of ultrathin organic films (<1 nm) and studied the excited phosphorescence properties of an exciplex/non-exciplex interface and phosphorescence UEMLs within the exciplex/non-exciplex interface. As a result, the elementary structure and mechanism of the energy transfer process of ultrathin emitting nanolayers within interface exciplexes have been discussed. The UEML phosphorescence dye plays a key role in determining the lifetime of excitons between exciplex and non-exciplex interfaces. The exciplex between TCTA and Bphen has longer lifetime decay than that of non-exciplex between TCTA and TAPC, facilitating exciton harvesting. Our research may not only help in the understanding and developing of the novel interface exciplex with UEMLs for OLEDs but also be beneficial to the development of other related organic optoelectronic technologies.
Data Availability Statement
The original contributions presented in the study are included in the article/Supplementary Material, further inquiries can be directed to the corresponding author.
Author Contributions
TX conceived of the work. BL fabricated samples. JF tested TRPL; TX and JF tested PL and Abs. spectra; and TX, XW, GL and BL participated in the discussion of the project and writing and modification of the manuscript.
Funding
This work was supported by the Open Project Funding of State Key Laboratory of Surface Physics and Department of Physics, Fudan University, China, (KF2019_13) and the Open Project Funding of Jiangsu Key Laboratory for Carbon-Based Functional Materials and Devices, Soochow University, China. (KJS1908). This work was supported in part by the National Natural Science Foundation of China under Grant 62104265, in part by the Science and Technology Program of Guangdong Province under Grant 2021A0505110009, and in part by the Innovation and Technology Fund under Grant GHP/006/20GD.
Conflict of Interest
The authors declare that the research was conducted in the absence of any commercial or financial relationships that could be construed as a potential conflict of interest.
The reviewer QX declared a past co-authorship with the author BL to the handling editor.
Publisher’s Note
All claims expressed in this article are solely those of the authors and do not necessarily represent those of their affiliated organizations, or those of the publisher, the editors, and the reviewers. Any product that may be evaluated in this article, or claim that may be made by its manufacturer, is not guaranteed or endorsed by the publisher.
Acknowledgments
The authors would like to express their special gratitude to Prof. Tze-Chien SUM, Prof. Hong Meng, and Prof. Xiaoyuan Hou for their support and assistance in the research.
References
Baek, S.-y., Kwak, S.-Y., Kim, S.-T., Hwang, K. Y., Koo, H., Son, W.-J., et al. (2020). Ancillary Ligand Increases the Efficiency of Heteroleptic Ir-Based Triplet Emitters in OLED Devices. Nat. Commun. 11, 2292. doi:10.1038/s41467-020-16091-1
Becker, K., Lupton, J. M., Müller, J., Rogach, A. L., Talapin, D. V., Weller, H., et al. (2006). Electrical Control of Förster Energy Transfer. Nat. Mater 5, 777–781. doi:10.1038/nmat1738
Chen, Z., Dai, C., Zhou, Q., Du, H., Fan, J., Han, S., et al. (2021). Enhanced Two-Photon-Excited Fluorescence from Electron Donor-Acceptor Exciplex. Dyes Pigm. 188, 109249. doi:10.1016/j.dyepig.2021.109249
Cortes, C. L., and Jacob, Z. (2018). Fundamental Figures of merit for Engineering Förster Resonance Energy Transfer. Opt. Express 26, 19371–19387. doi:10.1364/OE.26.019371
Gao, L., Quan, L. N., García de Arquer, F. P., Zhao, Y., Munir, R., Proppe, A., et al. (2020). Efficient Near-Infrared Light-Emitting Diodes Based on Quantum Dots in Layered Perovskite. Nat. Photon. 14, 227–233. doi:10.1038/s41566-020-0635-810.1038/s41566-019-0577-1
Gould, I. R., Young, R. H., Mueller, L. J., and Farid, S. (1994). Mechanisms of Exciplex Formation. Roles of Superexchange, Solvent Polarity, and Driving Force for Electron Transfer. J. Am. Chem. Soc. 116, 8176–8187. doi:10.1021/ja00097a027
Greiner, M. T., Helander, M. G., Tang, W.-M., Wang, Z.-B., Qiu, J., and Lu, Z.-H. (2012). Universal Energy-Level Alignment of Molecules on Metal Oxides. Nat. Mater 11, 76–81. doi:10.1038/nmat3159
Helander, M. G., Wang, Z. B., Qiu, J., Greiner, M. T., Puzzo, D. P., Liu, Z. W., et al. (2011). Chlorinated Indium Tin Oxide Electrodes with High Work Function for Organic Device Compatibility. Science 332, 944–947. doi:10.1126/science.1202992
Huang, T., Song, X., Cai, M., Zhang, D., and Duan, L. (2021). Improving Reverse Intersystem Crossing in Exciplex-Forming Hosts by Introducing Heavy Atom Effect. Mater. Today Energ. 21, 100705. doi:10.1016/j.mtener.2021.100705
Ingram, G. L., Chang, Y.-L., and Lu, Z. H., L. (2014). Probing the Exciton Distribution in Organic Light-Emitting Diodes Using Long-Range Energy Transfer. Can. J. Phys. 92, 845–848. doi:10.1139/cjp-2013-0608
Ingram, G. L., Nguyen, C., and Lu, Z.-H. (2016). Long-Range Energy Transfer and Singlet-Exciton Migration in Working Organic Light-Emitting Diodes. Phys. Rev. Appl. 5, 064002. doi:10.1103/PhysRevApplied.5.064002
Jankus, V., Chiang, C.-J., Dias, F., and Monkman, A. P. (2013). Deep Blue Exciplex Organic Light-Emitting Diodes with Enhanced Efficiency; P-type or E-type Triplet Conversion to Singlet Excitons? Adv. Mater. 25, 1455–1459. doi:10.1002/adma.201203615
Jones, G. A., and Bradshaw, D. S. (2019). Resonance Energy Transfer: From Fundamental Theory to Recent Applications. Front. Phys. 7. doi:10.3389/fphy.2019.00100
Kaganer, V. M., Jenichen, B., Shayduk, R., Braun, W., and Riechert, H. (2009). Kinetic Optimum of Volmer-Weber Growth. Phys. Rev. Lett. 102, 016103. doi:10.1103/PhysRevLett.102.016103
Kaur, A., Kaur, P., and Ahuja, S. (2020). Förster Resonance Energy Transfer (FRET) and Applications Thereof. Anal. Methods 12, 5532–5550. doi:10.1039/D0AY01961E
Kim, H.-B., and Kim, J.-J. (2020). Diffusion Mechanism of Exciplexes in Organic Optoelectronics. Phys. Rev. Appl. 13, 024006. doi:10.1103/PhysRevApplied.13.024006
Kim, H.-B., and Kim, J.-J. (2019). Recent Progress on Exciplex-Emitting OLEDs. J. Inf. Display 20, 105–121. doi:10.1080/15980316.2019.1650838
Li, D., Hu, Y., and Liao, L.-S. (2019). Triplet Exciton Harvesting by Multi-Process Energy Transfer in Fluorescent Organic Light-Emitting Diodes. J. Mater. Chem. C 7, 977–985. doi:10.1039/C8TC05141K
Li, D., and Liao, L.-S. (2019). Highly Efficient Deep-Red Organic Light-Emitting Diodes Using Exciplex-Forming Co-hosts and Thermally Activated Delayed Fluorescence Sensitizers with Extended Lifetime. J. Mater. Chem. C 7, 9531–9536. doi:10.1039/C9TC02834J
Liu, B.-Q., Tao, H., Su, Y.-J., Gao, D.-Y., Lan, L.-F., Zou, J.-H., et al. (2013). Color-Stable, Reduced Efficiency Roll-Off Hybrid White Organic Light Emitting Diodes with Ultra High Brightness. Chin. Phys. B 22, 077303. doi:10.1088/1674-1056/22/7/077303
Liu, B., Nie, H., Zhou, X., Hu, S., Luo, D., Gao, D., et al. (2016). Manipulation of Charge and Exciton Distribution Based on Blue Aggregation-Induced Emission Fluorophors: A Novel Concept to Achieve High-Performance Hybrid White Organic Light-Emitting Diodes. Adv. Funct. Mater. 26, 776–783. doi:10.1002/adfm.201503368
Liu, B., Xu, M., Wang, L., Tao, H., Su, Y., Gao, D., et al. (2014). Very-High Color Rendering Index Hybrid White Organic Light-Emitting Diodes with Double Emitting Nanolayers. Nano-micro Lett. 6, 335–339. doi:10.1007/s40820-014-0006-4
Liu, J., Shi, X., Wu, X., Wang, J., Min, Z., Wang, Y., et al. (2014). Achieving above 30% External Quantum Efficiency for Inverted Phosphorescence Organic Light-Emitting Diodes Based on Ultrathin Emitting Layer. Org. Elect. 15, 2492–2498. doi:10.1016/j.orgel.2014.07.027
Luo, D., Yang, Y., Xiao, Y., Zhao, Y., Yang, Y., and Liu, B. (2017). Regulating Charge and Exciton Distribution in High-Performance Hybrid White Organic Light-Emitting Diodes with N-type Interlayer Switch. Nanomicro Lett. 9, 37–38. doi:10.1007/s40820-017-0138-4
Luo, D., Chen, Q., Gao, Y., Zhang, M., and Liu, B. (2018). Extremely Simplified, High-Performance, and Doping-free White Organic Light-Emitting Diodes Based on a Single Thermally Activated Delayed Fluorescent Emitter. ACS Energ. Lett. 3, 1531–1538. doi:10.1021/acsenergylett.8b00711
Luo, D., Chen, Q., Liu, B., and Qiu, Y. (2019). Emergence of Flexible White Organic Light-Emitting Diodes. Polymers 11, 384. doi:10.3390/polym11020384
Luo, W., Chen, X., Sun, S.-Q., Zhang, Y.-J., Wang, T.-T., Liao, L.-S., et al. (2019). Management of Exciton for Highly-Efficient Hybrid White Organic Light-Emitting Diodes with a Non-doped Blue Emissive Layer. Molecules 24, 4046. doi:10.3390/molecules24224046
Menke, S. M., and Holmes, R. J. (2014). Exciton Diffusion in Organic Photovoltaic Cells. Energy Environ. Sci. 7, 499–512. doi:10.1039/C3EE42444H
Nakanotani, H., Furukawa, T., Morimoto, K., and Adachi, C. (2016). Long-range Coupling of Electron-Hole Pairs in Spatially Separated Organic Donor-Acceptor Layers. Sci. Adv. 2, e1501470. doi:10.1126/sciadv.1501470
Sanz-Paz, M., Wenger, J., van Hulst, N. F., Mivelle, M., and Garcia-Parajo, M. F. (2020). Nanoscale Control of Single Molecule Förster Resonance Energy Transfer by a Scanning Photonic. nanoantenna 9, 4021–4031. doi:10.1515/nanoph-2020-0221
Schleifenbaum, F., Kern, A. M., Konrad, A., and Meixner, A. J. (2014). Dynamic Control of Förster Energy Transfer in a Photonic Environment. Phys. Chem. Chem. Phys. 16, 12812–12817. doi:10.1039/C4CP01306A
Sitter, H., Resel, R., Koller, G., Ramsey, M. G., Andreev, A., and Teichert, C. (2008). “Fundamentals of Organic Film Growth and Characterisation,” in Organic Nanostructures for Next Generation Devices. Editors K. Al-Shamery, H.-G. Rubahn, and H. Sitter (Berlin, Heidelberg: Springer Berlin Heidelberg), 3–19. doi:10.1007/978-3-540-71923-6_1
Tan, S., Jinnai, K., Kabe, R., and Adachi, C. (2021). Long‐Persistent Luminescence from an Exciplex‐Based Organic Light‐Emitting Diode. Adv. Mater. 33, 2008844. doi:10.1002/adma.202008844
Wang, S., Zhang, H., Zhang, B., Xie, Z., and Wong, W.-Y. (2020). Towards High-Power-Efficiency Solution-Processed OLEDs: Material and Device Perspectives. Mater. Sci. Eng. R: Rep. 140, 100547. doi:10.1016/j.mser.2020.100547
Wang, Z. B., Helander, M. G., Qiu, J., Puzzo, D. P., Greiner, M. T., Hudson, Z. M., et al. (2011). Unlocking the Full Potential of Organic Light-Emitting Diodes on Flexible Plastic. Nat. Photon 5, 753–757. doi:10.1038/nphoton.2011.259
Wei, P., Zhang, D., and Duan, L. (2020). Modulation of Förster and Dexter Interactions in Single‐Emissive‐Layer All‐Fluorescent WOLEDs for Improved Efficiency and Extended Lifetime. Adv. Funct. Mater. 30, 1907083. doi:10.1002/adfm.201907083
Winkler, A. (2018). “Kinetics of Ultra-thin Organic Film Growth,” in Encyclopedia of Interfacial Chemistry. Editor K. Wandelt (Oxford: Elsevier), 195–215. doi:10.1016/b978-0-12-409547-2.12894-x
Wu, Z., Luo, J., Sun, N., Zhu, L., Sun, H., Yu, L., et al. (2016). High-Performance Hybrid White Organic Light-Emitting Diodes with Superior Efficiency/Color Rendering Index/Color Stability and Low Efficiency Roll-Off Based on a Blue Thermally Activated Delayed Fluorescent Emitter. Adv. Funct. Mater. 26, 3306–3313. doi:10.1002/adfm.201505602
Xiao, P., Dong, T., Xie, J., Luo, D., Yuan, J., and Liu, B. (2018). Emergence of White Organic Light-Emitting Diodes Based on Thermally Activated Delayed Fluorescence. Appl. Sci. 8, 299–330. doi:10.3390/app8020299
Xiao, P., Huang, J., Yan, D., Luo, D., Yuan, J., Liu, B., et al. (2018). Emergence of Nanoplatelet Light-Emitting Diodes. Materials 11, 1376. doi:10.3390/ma11081376
Xiao, P., Huang, J., Yu, Y., Yuan, J., Luo, D., Liu, B., et al. (2018). Recent Advances of Exciplex-Based White Organic Light-Emitting Diodes. Appl. Sci. 8, 1449. doi:10.3390/app8091449
Xu, T., Liang, X., and Xie, G. (2021a). Solution-Processed Pure Blue Thermally Activated Delayed Fluorescence Emitter Organic Light-Emitting Diodes with Narrowband Emission. Front. Chem. 9, 691172. doi:10.3389/fchem.2021.691172
Xu, T., Xie, G., Huang, T., Liu, H., Cao, X., Tang, Y., et al. (2021b). Solution-processed Multiple Exciplexes via Spirofluorene and S-Triazine Moieties for Red Thermally Activated Delayed Fluorescence Emissive Layer OLEDs. Org. Elect. 96, 106184. doi:10.1016/j.orgel.2021.106184
Xu, T., Yang, M., Liu, J., Wu, X., Murtaza, I., He, G., et al. (2016). Wide Color-Range Tunable and Low Roll-Off Fluorescent Organic Light Emitting Devices Based on Double Undoped Ultrathin Emitters. Org. Elect. 37, 93–99. doi:10.1016/j.orgel.2016.06.014
Xu, T., Yi, R., Zhu, C., and Lin, M. (2021c). Simple-Structured OLEDs Incorporating Undoped Phosphorescent Emitters within Non-exciplex Forming Interfaces: Towards Ultraslow Efficiency Roll-Off and Low Driving Voltage for Indoor R/G/B Illumination. Front. Chem. 8, 630. doi:10.3389/fchem.2020.630687
Xu, T., Zhang, Y.-X., Huang, C.-C., Zhou, J.-G., Fung, M.-K., and Meng, H. (2019). Highly Simplified Blue Phosphorescent Organic Light-Emitting Diodes Incorporating Exciplex-Forming Co-host Assisting Energy Transfer. J. Lumin. 206, 554–559. doi:10.1016/j.jlumin.2018.10.007
Xu, T., Zhang, Y.-X., Murtaza, I., and Meng, H. (2017). P-170: Organic Light-Emitting Diodes Incorporating a Novel Exciplex-Forming Host: A Synergistic Strategy to Design Highly Simplified OLEDs for Application. SID Symp. Dig. Tech. Pap. 48, 1915–1918. doi:10.1002/sdtp.12004
Xu, T., Zhang, Y.-X., Wang, B., Huang, C.-C., Murtaza, I., Meng, H., et al. (2017). Highly Simplified Reddish Orange Phosphorescent Organic Light-Emitting Diodes Incorporating a Novel Carrier- and Exciton-Confining Spiro-Exciplex-Forming Host for Reduced Efficiency Roll-Off. ACS Appl. Mater. Inter. 9, 2701–2710. doi:10.1021/acsami.6b13077
Xu, T., Zhou, J.-G., Fung, M.-K., and Meng, H. (2018). Simplified Efficient Warm white Tandem Organic Light-Emitting Devices by Ultrathin Emitters Using Energy Transfer from Exciplexes. Org. Elect. 63, 369–375. doi:10.1016/j.orgel.2018.09.026
Xu, T., Zhou, J.-G., Huang, C.-C., Zhang, L., Fung, M.-K., Murtaza, I., et al. (2017). Highly Simplified Tandem Organic Light-Emitting Devices Incorporating a Green Phosphorescence Ultrathin Emitter within a Novel Interface Exciplex for High Efficiency. ACS Appl. Mater. Inter. 9, 10955–10962. doi:10.1021/acsami.6b16094
Xu, T., Zhou, J.-G., Meng, H., and Fung, M.-K. (2018). P-164: Energy Transfer from Interface Exciplexes to Ultrathin Emissive Layers: A Path Way to Design Simplified Efficient White Tandem Organic Light-Emitting Diodes for Application. SID Symp. Dig. Tech. Pap. 49, 1779–1781. doi:10.1002/sdtp.12394
Yao, J., Ying, S., Qiao, X., Yang, D., Chen, J., Ahamad, T., et al. (2019). High Efficiency and Low Roll-Off All Fluorescence white Organic Light-Emitting Diodes by the Formation of Interface Exciplex. Org. Elect. 67, 72–78. doi:10.1016/j.orgel.2019.01.011
Yuan, F., Wang, Y.-K., Sharma, G., Dong, Y., Zheng, X., Li, P., et al. (2020). Bright High-Colour-Purity Deep-Blue Carbon Dot Light-Emitting Diodes via Efficient Edge Amination. Nat. Photon. 14, 171–176. doi:10.1038/s41566-019-0557-5
Zhang, C., Zhang, D., Bin, Z., Liu, Z., Zhang, Y., Lee, H., et al. (2021). Color-Tunable All-Fluorescent White Organic Light-Emitting Diodes with a High External Quantum Efficiency over 30% and Extended Device Lifetime. Adv. Mater. 2021, 2103102. doi:10.1002/adma.202103102
Zhang, D., Cai, M., Zhang, Y., Zhang, D., and Duan, L. (2015). Highly Efficient Simplified Single-Emitting-Layer Hybrid WOLEDs with Low Roll-Off and Good Color Stability through Enhanced Förster Energy Transfer. ACS Appl. Mater. Inter. 7, 28693–28700. doi:10.1021/acsami.5b10783
Zhang, D., Song, X., Gillett, A. J., Drummond, B. H., Jones, S. T. E., Li, G., et al. (2020). Efficient and Stable Deep‐Blue Fluorescent Organic Light‐Emitting Diodes Employing a Sensitizer with Fast Triplet Upconversion. Adv. Mater. 32, 1908355. doi:10.1002/adma.201908355
Zhang, T., Yao, J., Zhang, S., Xiao, S., Liu, W., Wu, Z., et al. (2021). Highly Efficient and Low Efficiency Roll-Off Organic Light-Emitting Diodes with Double-Exciplex Forming Co-hosts. J. Mater. Chem. C 9, 6062–6067. doi:10.1039/D0TC05842D
Zhao, B., Zhang, H., Miao, Y., Wang, Z., Gao, L., Wang, H., et al. (2017). Low Turn-On Voltage and Low Roll-Off Rare Earth Europium Complex-Based Organic Light-Emitting Diodes with Exciplex as the Host. J. Mater. Chem. C 5, 12182–12188. doi:10.1039/C7TC03694A
Zhao, Y., Chen, J., and Ma, D. (2011). Realization of High Efficiency orange and white Organic Light Emitting Diodes by Introducing an Ultra-thin Undoped orange Emitting Layer. Appl. Phys. Lett. 99, 163303. doi:10.1063/1.3654150
Zheng, H., Zheng, Y., Liu, N., Ai, N., Wang, Q., Wu, S., et al. (2013). All-solution Processed Polymer Light-Emitting Diode Displays. Nat. Commun. 4, 1971. doi:10.1038/ncomms2971
Keywords: film growth, undoped ultrathin emitting nanolayer, interface exciplexes, photophysics, OLED
Citation: Xu T, Fu J, Wang X, Lu G and Liu B (2022) Understanding the Structure and Energy Transfer Process of Undoped Ultrathin Emitting Nanolayers Within Interface Exciplexes. Front. Chem. 10:887900. doi: 10.3389/fchem.2022.887900
Received: 02 March 2022; Accepted: 21 March 2022;
Published: 12 April 2022.
Edited by:
Dechao Yu, University of Shanghai for Science and Technology, ChinaReviewed by:
Mingjie Li, Hong Kong Polytechnic University, Hong Kong SAR, ChinaQifan Xue, South China University of Technology, China
Hongming Chen, Fuzhou University, China
Copyright © 2022 Xu, Fu, Wang, Lu and Liu. This is an open-access article distributed under the terms of the Creative Commons Attribution License (CC BY). The use, distribution or reproduction in other forums is permitted, provided the original author(s) and the copyright owner(s) are credited and that the original publication in this journal is cited, in accordance with accepted academic practice. No use, distribution or reproduction is permitted which does not comply with these terms.
*Correspondence: Ting Xu, xuting_robin@pku.edu.cn; Xinzhong Wang, xzwang2188@163.com; Baiquan Liu, liubq33@mail.sysu.edu.cn