- 1School of Chemical and Environmental Engineering, Anhui Polytechnic University, Wuhu, China
- 2Institutes of Physical Science and Information Technology, Key Laboratory of Structure and Functional Regulation of Hybrid Materials of Ministry of Education, Anhui University, Hefei, China
- 3Key Laboratory of Advanced Structural Materials of Ministry of Education, College of Material Science and Engineering, College of Chemistry and Life Science, Advanced Institute of Materials Science, Changchun University of Technology, Changchun, China
Most pyridine-imine Ni(II) and Pd(II) catalysts tend to yield low-molecular-weight polyethylene and ethylene-based copolymers in olefin insertion polymerization, as the unilateral axial steric structure of such complexes often cannot provide effective shielding of the metal center. In this study, we synthesized a series of hybrid “semi-sandwich” and “sandwich” type pyridine-imine Ni(II) complexes by incorporating diarylmethyl or dibenzosuberyl groups onto 8-aryl-naphthyl motif. The as-prepared Ni(II) complexes afforded highly branched polyethylene with high molecular weights (level of 105 g/mol), and moderate activities (level of 105 g/(molh)) in ethylene polymerization. Most interestingly, compared to “semi-sandwich” Ni(II) complexes bearing (2-diarylmethyl-8-aryl)naphthyl units, the “full-sandwich” counterpart containing (2-dibenzosuberyl-8-aryl)naphthyl motif was able to produce higher-molecular-weight polyethylene with higher branching density. In addition, the effect of remote non-conjugated electronic substituents in diarylmethyl groups of the Ni(II) system was also observed in ethylene polymerization.
Introduction
As known, the [N, N] bidentate α-diimine Ni(II) and Pd(II) complexes bearing double-sided axial steric structures represent a mainstream catalytic system, which tend to yield high-molecular-weight polyethylene and ethylene-polar monomer copolymers. (Dai et al., 2016a; Gong et al., 2019a; Guo et al., 2018; Meinhard et al., 2007; Rhinehart et al., 2013; Dai et al., 2015; Xia et al., 2020; Zhao et al., 2021; Wang et al., 2020a; Zhang et al., 2013; Gong et al., 2019b; Liao et al., 2019; Zhong et al., 2017a; Zhong et al., 2017b; Zhong et al., 2019; Abedini et al., 2021; Kanai et al., 2019; Zhang et al., 2020; Ma et al., 2021; Li et al., 2021a; Allen et al., 2015). In contrast, pyridine-imine Ni(II) and Pd(II) catalysts often give rise to low-molecular-weight oligomers because the only unilateral axial steric hindrance rising from the imine motif hardly shields the metal well in most cases. (Dai et al., 2016b). Consequently, strategies that are effective in suppressing the chain transfer to bring forth high-molecular-weight products in α-diimine systems are often not applicable to the pyridine-imine systems. For example, by using the bulky diarylmethyl anilines, the α-diimine Ni(II) and Pd(II) catalysts can generate high-molecular-weight and even ultra-high-molecular-weight polyethylenes. (Rhinehart et al., 2013; Dai et al., 2015; Zhao et al., 2021; Gong et al., 2019b). However, the pyridine-imine system derived from same diarylmethyl anilines provides only branched ethylene oligomers. (Chen et al., 2018; Li et al., 2021b; Yan et al., 2021; Wang et al., 2020b; Guo et al., 2019). In fact, since Laine et al. reported that the first example of pyridine-imine nickel-catalyzed ethylene polymerization yielded low-molecular-weight branched polyethylene, (Laine et al., 1999), many attempts, including the steric tuning of the o-aryl substituents, modifying the pyridine backbone and adjusting ligand electronic effect have been made to improve this situation, but no visible improvement was achieved (Chart 1A). (Bianchini et al., 2010; Laine et al., 2000; Meneghetti et al., 1999; Huang et al., 2016; Sun et al., 2015a; Huang et al., 2015; Sun et al., 2015b; Yue et al., 2014; Sun et al., 2012; Chen et al., 2016) Recently, we simultaneously integrated 8-aryl-naphthyl and dibenzhydryl substituents into the pyridine-imine system, making the resultant complexes able to effectively suppress chain transfer in the ethylene polymerization, thus yielding high molecular weight polyethylene (Mn well above 100 kg/mol) (Chart 1B). (Dai et al., 2016b) More recently, the dibenzosuberyl groups were also employed in the pyridine-imine system to enhance the polyethylene molecular weight (Mn up to 124 kg/mol) via a rotation-restricted strategy (Chart 1C). (Peng et al., 2021; Li and Dai, 2021) In contrast, the pyridine-imine consisting of N-terphenyl structure failed to retard chain transfer, thus only hyperbranched ethylene oligomers and ethylene-methyl acrylate (MA) co-oligomers can be obtained (Chart 1D). (Fan et al., 2021; Yan et al., 2022; Fan et al., 2022) In addition, the effectiveness of a single dibenzosuberyl group in unsymmetrical iminopyridyl Ni(II) and Pd(II) catalysts in retarding the chain transfer was also demonstrated (Chart 1E). (Ge et al., 2021) In this study, the dibenzosuberyl and 8-aryl-naphthyl units are integrated into the pyridine-imine nickel catalyst at the same time and the resulting hybrid “sandwich” catalyst is capable of catalyzing ethylene polymerization to yield highly branched polyethylene with high molecular weights (Chart 1F).
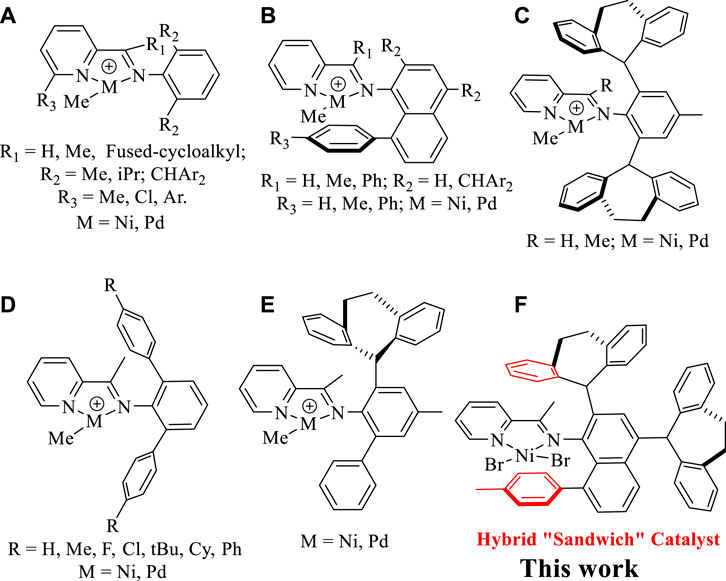
CHART 1. Modifications of pyridine-imine Ni(II) and Pd(II) catalysts (A–E), and our current work (F).
Results and Discussion
Synthesis and Characterization of Pyridine-Imine Nickel and Palladium Complexes
Ligands L1-L5 were synthesized according to the previous reports. (Li and Dai, 2021). Treating these ligands with 1.0 equiv. of [NiBr2(DME)] (DME = Dimethoxyethane) in dichloromethane at ambient temperature yielded Ni(II) complexes Ni1-Ni5 in excellent yields (81–94%) (Scheme 1). The purity and identity of Ni1-Ni5 were examined by elemental analysis and MALDI-TOF MS (Supplementary Figures S3–7). Similarly, the Pd(II) complex Pd5 was synthesized by exposing the ligand L5 to [PdClMe(COD)] (COD = 1, 5-cyclooctadiene) in dichloromethane at ambient temperature (Scheme 1). The obtained Pd(II) complex was verified by 1H and 13C NMR (Supplementary Figures S1, 2), ESI-MS (Supplementary Figure S8), and elemental analysis. The single crystal Pd5 was obtained by layering its CH2Cl2 solution with diethyl ether at ambient temperature (Figure 1). The complex Pd5 displays an approximate planar square geometry at the Pd(II) center, and the 4-methylphenyl group and phenyl ring of dibenzosuberyl substituent lie nearly parallel to the five-membered chelate ring and effectively block the axial coordination site of the Pd(II) complex, which is responsible for the retardation of the undesired chain transfer. Here, we also provide the buried volume diagram of Pd5 complex analyzed by SambVca 2.0 program (Figure 2). (Falivene et al., 2015) As expected, the complex Pd5 possessed crowded environment around the palladium center with the percent buried volume of 51.0%. This type of bulky substituents helps to suppress chain transfer during polymerization to obtain high molecular weight polymers. (Deng et al., 1997; Talarico et al., 2004).
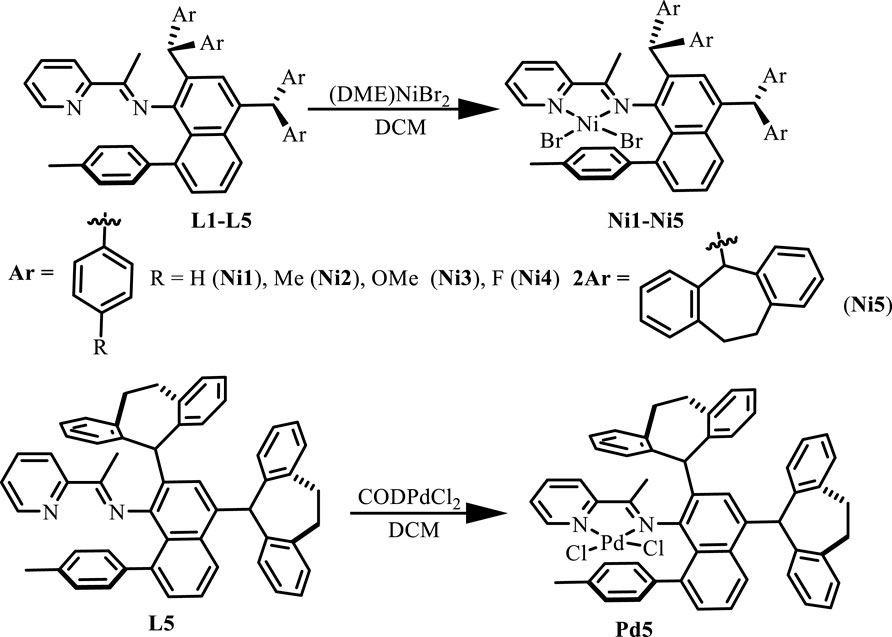
SCHEME 1. Synthesis of “semi-sandwich” and “sandwich” type pyridine-imine Ni(II) (Ni1-Ni5) and Pd(II) (Pd5) complexes.
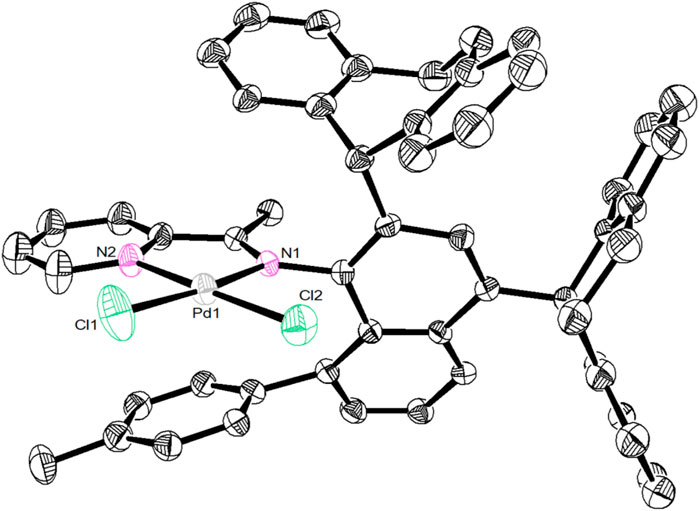
FIGURE 1. Solid-state molecular structure of Pd5 (2150684) at the 30% probability level. All solvent molecules and hydrogen atoms are omitted for better clarity.
Ethylene Polymerization
Upon in-situ activation with 200 equivalents of Et2AlCl, all the Ni(II) complexes exhibited moderate activities (level of 105 g mol−1·h−1) and yielded high molecular weight (level of 100 kg/mol) polyethylene with high branching densities (57-90/1000 C) and low melting points (-6–53°C) in ethylene polymerization (Table 1; Figures 3, 4). The polymerization activities of these Ni(II) complexes remained almost unchanged with the increase of temperature (Figure 3A). As the ethylene pressure decreased (from 6 to 3 atm and 1 atm), both the polymerization activity and the molecular weight of the resulting polyethylene declined, and the decrease in polymerization activity is more pronounced (Table 1, entries 1 vs 17-18). Similar to the reported nickel-catalyzed ethylene polymerization systems (Zhang et al., 2013), chain termination is mainly based on the pathway of synergistic transfer of polymer chains to monomers. Amidst these five nickel complexes, Ni4 containing electron withdrawing fluorine and Ni5 composed of dibenzosuberyl substituent exhibited relatively higher catalytic activity than the others. Probably, a weaker interaction between metal and fluorinated aryl group for Ni4 and the stronger catalyst thermal stability originating from the proper aryl orientation for Ni5 may contribute to the better catalytic activities. As opposed to the fact that the pyridine-imine catalysts usually generate low-molecular-weight polyethylene or copolymers in ethylene (co)polymerization due to the unilateral axial steric structure of the pyridine-imine ligand, all these Ni(II) complexes in our case yielded polyethylene with high molecular weight (level of 100 kg/mol), one or two orders of magnitude higher than those obtained in most reported pyridine-imine systems (Figure 5). (Laine et al., 1999; Meneghetti et al., 1999; Laine et al., 2000; Bianchini et al., 2010; Sun et al., 2012; Yue et al., 2014; Sun et al., 2015a; Sun et al., 2015b; Huang et al., 2015; Chen et al., 2016; Huang et al., 2016; Guo et al., 2019; Wang et al., 2020b; Fan et al., 2021; Li and Dai, 2021; Peng et al., 2021; Yan et al., 2022) This is mainly attributed to the synergistic effect of 8-arylnaphthyl and diarylmethyl groups, which form a sandwich-like structure that can effectively retard chain transfer during polymerization. In particular, Ni5 is capable of generating the highest molecular weight of polyethylene among these catalysts (Figure 2B). An explanation is that the ring structure in the dibenzosuberyl substituent that drives the aryl group closer to the axial position of the metal center. This allows the conversion of the catalyst structure from a semi-sandwich to a full-sandwich structure (Figure 1). It is worth noting that Ni3 also produced higher molecular weight polyethylene than other catalysts of the same type (Ni1, Ni2 and Ni4). This may be due to the interaction of methoxy with the co-catalyst (Et2AlCl) to form a greater axial steric hindrance, which can more effectively retard the chain transfer during polymerization process (Figure 6).
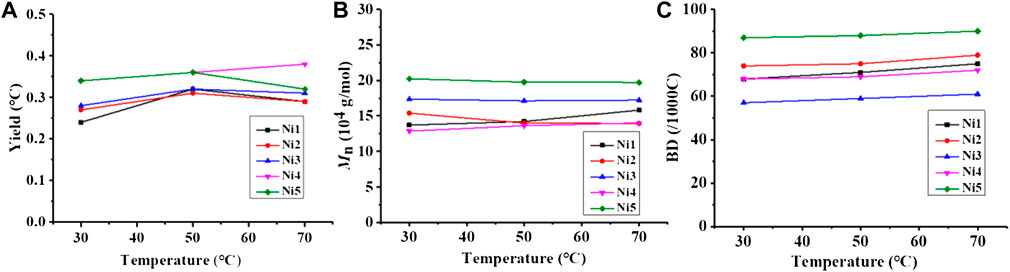
FIGURE 3. Comparisons on yield (A), molecular weight (B), and branching density (C) of polyethylene yielded with catalysts Ni1-Ni5 at 30–70°C.
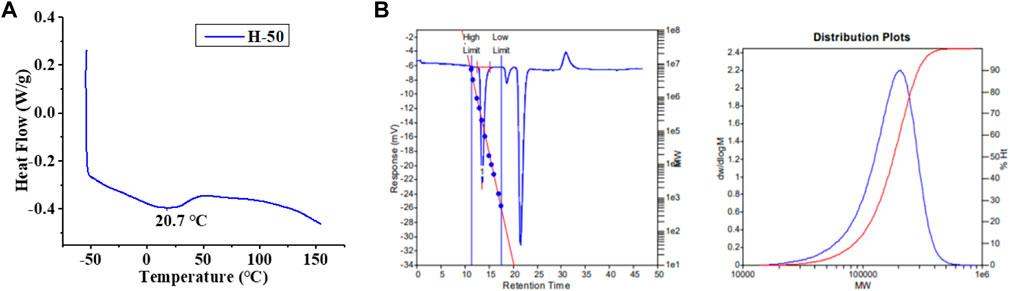
FIGURE 4. DSC (A) and GPC (B) of the branched polyethylene obtained by using Ni1 at 50°C (Table 1, entry 2).
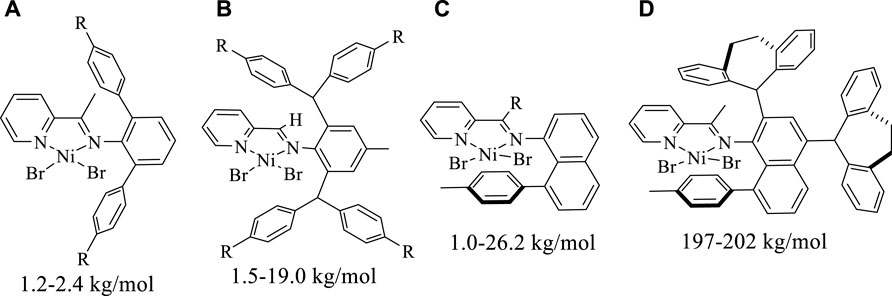
FIGURE 5. Comparisons on molecular weights of polyethylene yielded with previously reported nickel catalysts (A–C) and Ni5 (D).
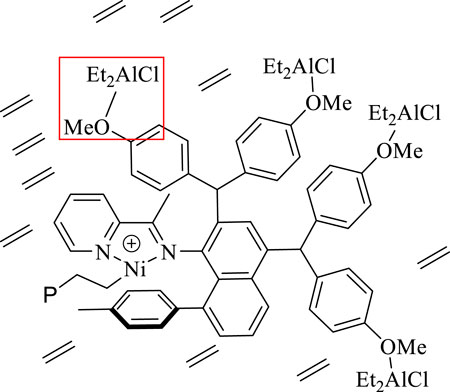
FIGURE 6. The interaction of OMe with the co-catalyst (Et2AlCl) to form a greater axial steric hindrance.
In addition, Ni5 yielded the polyethylene with the highest branching density and lowest melting point while Ni3 produced the polyethylene with the lowest branching density and highest melting point (Figure 2C). As elaborated in previous reports, the sandwich structure of Ni5 facilitates chain walking processes, thus yielding highly branched polyethylene. (Zhang et al., 2013). By contrast, the interaction of methoxy with the co-catalyst (Et2AlCl) in Ni3 forms a large axial steric hindrance that may disfavor chain walking. The proximity interaction of the co-catalyst with β-H may also be one factor contributing to lowered branching density. (Ma et al., 2021; Li et al., 2020). The microstructure of a representative polyethylene product (Table 1, entry 15) was revealed by 13C NMR analysis (Figure 7). (Randall, 1989; Galland et al., 1999; Cotts et al., 2000) The 13C NMR analysis suggests that the obtained polyethylene consists of methyl branches, ethyl branches, n-propyl branches and long chain branches formation with chain walking mechanism (Scheme 2). Among them, methyl branches and long chain branches account for the majority of all the branches. This indicates that Ni5 with a sandwich structure is capable of generating polyethylene with randomly branches distribution in which methyl and long chain branching dominate, further demonstrating its strong chain walking ability. Further comparison with the 13C NMR analysis of the polyethylene yielded with Ni1 and Ni3 at 70°C, the hybrid “sandwich” structure of Ni5 facilitates access to a higher percentage of long chain branching (Supplementary Table S1, Supplementary Figure S12).
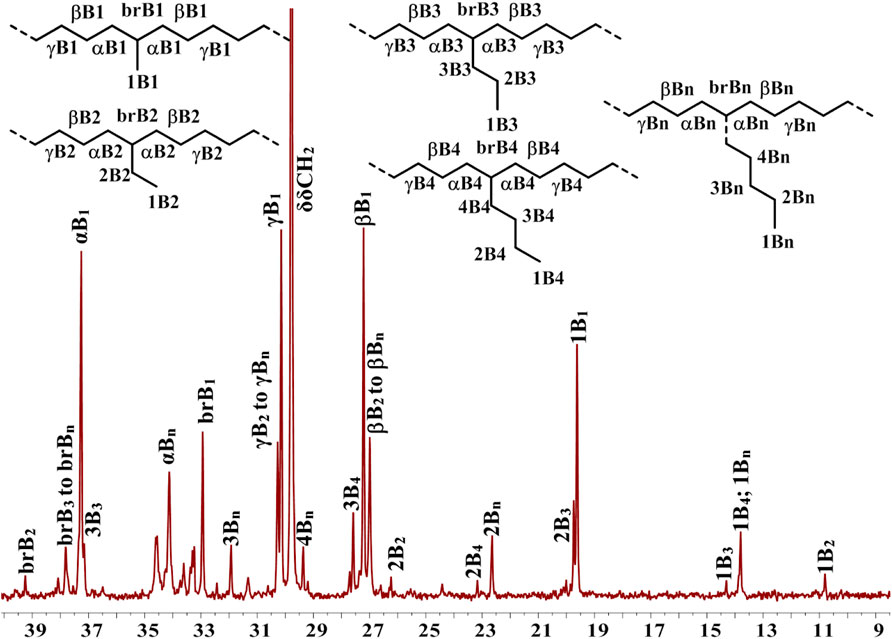
FIGURE 7. Detailed analysis of 13C NMR spectrum of branched polyethylene obtained by using Ni5 at 70°C (Table 1, entry 15). Assignments are numbered according to ref. 49-51. Branches are labeled as xBy, where y is the branch length and x is the carbon, starting from the methyl end with 1. The methine groups for the different branch lengths are labeled with brBy.
Conclusion
In summary, a series of “semi-sandwich” and “sandwich” type pyridine-imine Ni(II) complexes bearing diarylmethyl or dibenzosuberyl groups and 8-aryl-naphthyl substituent were synthesized and characterized. The Ni(II) complexes exhibited moderate activities (level of 105 g/(molh)) and generated highly branched (57-90/1000 C) polyethylene with high molecular weights (level of 105 g/mol) in ethylene polymerization. Moreover, the “full-sandwich” Ni(II) complex containing 8-arylnaphthyl and dibenzosuberyl substituents yielded higher molecular weight polyethylene with higher branching density than those from “semi-sandwich” Ni(II) complexes bearing 8-arylnaphthyl and diarylmethyl groups. In addition, the remote non-conjugated electronic substituents in diarylmethyl groups of the Ni(II) system also have an effect on the ethylene polymerization.
Data Availability Statement
The original contributions presented in the study are included in the article/Supplementary Material, further inquiries can be directed to the corresponding authors.
Author Contributions
SD: Conceptualization, Data curation, Formal analysis, Funding acquisition, Investigation, Resources, Validation, Supervision, Writing—original draft, Writing—review and editing. YC: Resources, Validation, Supervision, Writing—review and editing JG: Resources, Validation, Supervision, Writing— review and editing YW: Investigation QC: Formal analysis, Investigation, Methodology YG: Formal analysis, Investigation, Methodology.
Funding
This work was supported by Natural Science Foundation of Anhui Province (2108085Y06), Anhui Provincial Key Laboratory Open Project Foundation (LCECSC-01), and Jilin Province Science and Technology Department Project (No. 20210101070JC).
Conflict of Interest
The authors declare that the research was conducted in the absence of any commercial or financial relationships that could be construed as a potential conflict of interest.
Publisher’s Note
All claims expressed in this article are solely those of the authors and do not necessarily represent those of their affiliated organizations, or those of the publisher, the editors and the reviewers. Any product that may be evaluated in this article, or claim that may be made by its manufacturer, is not guaranteed or endorsed by the publisher.
Supplementary Material
The Supplementary Material for this article can be found online at: https://www.frontiersin.org/articles/10.3389/fchem.2022.886888/full#supplementary-material
References
Abedini, A., Jamjah, R., and Arabi, H. (2021). Parallel Reactions in Polymerization of Ethylene/methyl Methacrylate by Late-Transition-Metal Catalysts (α-Diimine Nickel). Iran. Polym. J. 30, 843–848. doi:10.1007/s13726-021-00936-7
Allen, K. E., Campos, J., Daugulis, O., and Brookhart, M. (2015). Living Polymerization of Ethylene and Copolymerization of Ethylene/Methyl Acrylate Using “Sandwich” Diimine Palladium Catalysts. ACS Catal. 5, 456–464. doi:10.1021/cs5016029
Bianchini, C., Giambastiani, G., Luconi, L., and Meli, A. (2010). Olefin Oligomerization, Homopolymerization and Copolymerization by Late Transition Metals Supported by (Imino)pyridine Ligands. Coord. Chem. Rev. 254, 431–455. doi:10.1016/j.ccr.2009.07.013
Chen, X.-L., Gao, J., Liao, H., Gao, H.-Y., and Wu, Q. (2018). Synthesis, Characterization, and Catalytic Ethylene Oligomerization of Pyridine-Imine Palladium Complexes. Chin. J. Polym. Sci. 36, 176–184. doi:10.1007/s10118-018-2052-8
Chen, Z., Allen, K. E., White, P. S., Daugulis, O., and Brookhart, M. (2016). Synthesis of Branched Polyethylene with "Half-Sandwich" Pyridine-Imine Nickel Complexes. Organometallics 35, 1756–1760. doi:10.1021/acs.organomet.6b00165
Cotts, P. M., Guan, Z., McCord, E., and McLain, S. (2000). Novel Branching Topology in Polyethylenes as Revealed by Light Scattering and 13C NMR. Macromolecules 33, 6945–6952. doi:10.1021/ma000926r
Dai, S., Sui, X., and Chen, C. (2015). Highly Robust Palladium(II) α-Diimine Catalysts for Slow-Chain-Walking Polymerization of Ethylene and Copolymerization with Methyl Acrylate. Angew. Chem. 127, 10086–10091. doi:10.1002/ange.201503708
Dai, S., Sui, X., and Chen, C. (2016). Synthesis of High Molecular Weight Polyethylene Using Iminopyridyl Nickel Catalysts. Chem. Commun. 52, 9113–9116. doi:10.1039/c6cc00457a
Dai, S., Zhou, S., Zhang, W., and Chen, C. (2016). Systematic Investigations of Ligand Steric Effects on α-Diimine Palladium Catalyzed Olefin Polymerization and Copolymerization. Macromolecules 49, 8855–8862. doi:10.1021/acs.macromol.6b02104
Deng, L., Woo, T. K., Cavallo, L., Margl, P. M., and Ziegler, T. (1997). The Role of Bulky Substituents in Brookhart-type Ni(II) Diimine Catalyzed Olefin Polymerization: A Combined Density Functional Theory and Molecular Mechanics Study. J. Am. Chem. Soc. 119, 6177–6186. doi:10.1021/ja970226a
Falivene, L., Cavallo, L., and Talarico, G. (2015). Buried Volume Analysis for Propene Polymerization Catalysis Promoted by Group 4 Metals: A Tool for Molecular Mass Prediction. ACS Catal. 5, 6815–6822. doi:10.1021/acscatal.5b01363
Fan, H., Chang, G., Bi, H., Gui, X., Wang, H., Xu, G., et al. (2021). Facile Synthesis of Hyperbranched Ethylene Oligomers and Ethylene/Methyl Acrylate Co-oligomers with Different Microscopic Chain Architectures. ACS Polym. Au 2, 88–96. doi:10.1021/acspolymersau.1c00039
Fan, H., Xu, G., Wang, H., and Dai, S. (2022). Direct Synthesis of Hyperbranched Ethene Oligomers and Ethene‐ MA Co‐oligomers Using Iminopyridyl Systems with Weak Neighboring Group Interactions. J. Polym. Sci. doi:10.1002/pol.20220047
Galland, G. B., De Souza, R. F., Mauler, R. S., and Nunes, F. F. (1999). 13C NMR Determination of the Composition of Linear Low-Density Polyethylene Obtained with [η3-Methallyl-Nickel-diimine]PF6 Complex. Macromolecules 32, 1620–1625. doi:10.1021/ma981669h
Ge, Y., Li, S., Wang, H., and Dai, S. (2021). Synthesis of Branched Polyethylene and Ethylene-MA Copolymers Using Unsymmetrical Iminopyridyl Nickel and Palladium Complexes. Organometallics 40, 3033–3041. doi:10.1021/acs.organomet.1c00388
Gong, Y., Li, S., Gong, Q., Zhang, S., Liu, B., and Dai, S. (2019). Systematic Investigations of Ligand Steric Effects on α-Diimine Nickel Catalyzed Olefin Polymerization and Copolymerization. Organometallics 38, 2919–2926. doi:10.1021/acs.organomet.9b00267
Gong, Y., Li, S., Tan, C., Kong, W., Xu, G., Zhang, S., et al. (2019). π–π Interaction Effect in Insertion Polymerization with α-diimine Palladium Systems. J. Cat. 378, 4–191. doi:10.1016/j.jcat.2019.08.034
Guo, L., Li, S., Ji, M., Sun, W., Liu, W., Li, G., et al. (2019). Monoligated vs Bisligated Effect in Iminopyridyl Nickel Catalyzed Ethylene Polymerization. Organometallics 38, 2800–2806. doi:10.1021/acs.organomet.9b00325
Guo, L., Lian, K., Kong, W., Xu, S., Jiang, G., and Dai, S. (2018). Synthesis of Various Branched Ultra-high-molecular-weight Polyethylenes Using Sterically Hindered Acenaphthene-Based α-Diimine Ni(II) Catalysts. Organometallics 37, 2442–2449. doi:10.1021/acs.organomet.8b00275
Huang, C., Zhang, Y., Liang, T., Zhao, Z., Hu, X., and Sun, W.-H. (2016). Rigid Geometry 8-Arylimino-7,7-Dimethyl-5,6-Dihydroquinolyl Nickel Bromides: Single-Site Active Species towards Ethylene Polymerization. New J. Chem. 40, 9329–9336. doi:10.1039/c6nj02464e
Huang, F., Sun, Z., Du, S., Yue, E., Ba, J., Hu, X., et al. (2015). Ring-tension Adjusted Ethylene Polymerization by Aryliminocycloheptapyridyl Nickel Complexes. Dalton Trans. 44, 14281–14292. doi:10.1039/c5dt01831e
Kanai, Y., Foro, S., and Plenio, H. (2019). Bispentiptycenyl-Diimine-Nickel Complexes for Ethene Polymerization and Copolymerization with Polar Monomers. Organometallics 38, 544–551. doi:10.1021/acs.organomet.8b00836
Laine, T. V., Lappalainen, K., Liimatta, J., Aitola, E., Löfgren, B., and Leskelä, M. (1999). Polymerization of Ethylene with New Diimine Complexes of Late Transition Metals. Macromol. Rapid Commun. 20, 487–491. doi:10.1002/(sici)1521-3927(19990901)20:9<487::aid-marc487>3.0.co;2-g
Laine, T. V., Piironen, U., Lappalainen, K., Klinga, M., Aitola, E., and Leskelä, M. (2000). Pyridinylimine-based Nickel(II) and Palladium(II) Complexes: Preparation, Structural Characterization and Use as Alkene Polymerization Catalysts. J. Organomet. Chem. 606, 112–124. doi:10.1016/s0022-328x(00)00291-6
Li, S., and Dai, S. (2021). Highly Efficient Incorporation of Polar Comonomers in Copolymerizations with Ethylene Using Iminopyridyl Palladium System. J. Catal. 393, 51–59. doi:10.1016/j.jcat.2020.11.015
Li, S., Lu, Z., Fan, W., and Dai, S. (2021). Efficient Incorporation of a Polar Comonomer for Direct Synthesis of Hyperbranched Polar Functional Ethylene Oligomers. New J. Chem. 45, 4024–4031. doi:10.1039/d0nj05857b
Li, S., Xu, G., and Dai, S. (2020). A Remote Nonconjugated Electron Effect in Insertion Polymerization with α-diimine Nickel and Palladium Species. Polym. Chem. 11, 2692–2699. doi:10.1039/d0py00218f
Li, S., Zhao, Y., and Dai, S. (2021). Synthesis of Polyethylene Thermoplastic Elastomer by Using Robust α‐diimine Ni( II ) Catalysts with Abundant T Bu Substituents. J. Polym. Sci. 59, 638–645. doi:10.1002/pol.20210017
Liao, Y., Zhang, Y., Cui, L., Mu, H., and Jian, Z. (2019). Pentiptycenyl Substituents in Insertion Polymerization with α-Diimine Nickel and Palladium Species. Organometallics 38, 2075–2083. doi:10.1021/acs.organomet.9b00106
Ma, X., Zhang, Y., and Jian, Z. (2021). Tunable Branching and Living Character in Ethylene Polymerization Using "polyethylene Glycol Sandwich" α-diimine Nickel Catalysts. Polym. Chem. 12, 1236–1243. doi:10.1039/d0py01689f
Meinhard, D., Wegner, M., Kipiani, G., Hearley, A., Reuter, P., Fischer, S., et al. (2007). New Nickel(II) Diimine Complexes and the Control of Polyethylene Microstructure by Catalyst Design. J. Am. Chem. Soc. 129, 9182–9191. doi:10.1021/ja070224i
Meneghetti, S. P., Lutz, P. J., and Kress, J. (1999). Oligomerization of Olefins Catalyzed by New Cationic Palladium(II) Complexes Containing an Unsymmetrical α-Diimine Ligand. Organometallics 18, 2734–2737. doi:10.1021/om990165k
Peng, H., Li, S., Li, G., Dai, S., Ji, M., Liu, Z., et al. (2021). Rotation-restricted Strategy to Synthesize High Molecular Weight Polyethylene Using Iminopyridyl Nickel and Palladium Catalyst. Appl. Organomet. Chem. 35, e6140. doi:10.1002/aoc.6140
Randall, J. C. (1989). A Review of High Resolution Liquid13carbon Nuclear Magnetic Resonance Characterizations of Ethylene-Based Polymers. J. Macromol. Sci. Part C Polym. Rev. 29, 201–317. doi:10.1080/07366578908055172
Rhinehart, J. L., Brown, L. A., and Long, B. K. (2013). A Robust Ni(II) α-Diimine Catalyst for High Temperature Ethylene Polymerization. J. Am. Chem. Soc. 135, 16316–16319. doi:10.1021/ja408905t
Sun, W.-H., Song, S., Li, B., Redshaw, C., Hao, X., Li, Y.-S., et al. (2012). Ethylene Polymerization by 2-iminopyridylnickel Halide Complexes: Synthesis, Characterization and Catalytic Influence of the Benzhydryl Group. Dalton Trans. 41, 11999–12010. doi:10.1039/c2dt30989k
Sun, Z., Huang, F., Qu, M., Yue, E., Oleynik, I. V., Oleynik, I. I., et al. (2015). Targeting Polyethylene Waxes: 9-(2-Cycloalkylphenylimino)-5,6,7,8-Tetrahydrocycloheptapyridylnickel Halides and Their Use as Catalysts for Ethylene Polymerization. RSC Adv. 5, 77913–77921. doi:10.1039/c5ra15806k
Sun, Z., Yue, E., Qu, M., Oleynik, I. V., Oleynik, I. I., Li, K., et al. (2015). 8-(2-Cycloalkylphenylimino)-5,6,7-trihydro-quinolylnickel Halides: Polymerizing Ethylene to Highly Branched and Lower Molecular Weight Polyethylenes. Inorg. Chem. Front. 2, 223–227. doi:10.1039/c4qi00162a
Talarico, G., Busico, V., and Cavallo, L. (2004). "Living" Propene Polymerization with Bis(phenoxyimine) Group 4 Metal Catalysts: New Strategies and Old Concepts. Organometallics 23, 5989–5993. doi:10.1021/om049296y
Wang, C., Zhang, Y., Mu, H., and Jian, Z. (2020). Systematic Studies on Dibenzhydryl and Pentiptycenyl Substituted Pyridine-Imine Nickel(II) Mediated Ethylene Polymerization. Dalton Trans. 49, 4824–4833. doi:10.1039/d0dt00505c
Wang, X., Dong, B., Yang, Q., Liu, H., Zhang, C., and Zhang, X. (2020). α-Diimine Nickel Complexes Bearing Axially Bulky Terphenyl and Equatorially Bulky Dibenzobarrelene Groups: Synthesis, Characterization and Olefin Polymerization Studies. Polym. Chem. 11, 6783–6793. doi:10.1039/d0py01152e
Xia, J., Zhang, Y., Kou, S., and Jian, Z. (2020). A Concerted Double-Layer Steric Strategy Enables an Ultra-highly Active Nickel Catalyst to Access Ultrahigh Molecular Weight Polyethylenes. J. Catal. 390, 30–36. doi:10.1016/j.jcat.2020.07.017
Yan, Z., Bi, H., Ding, B., Wang, H., Xu, G., and Dai, S. (2022). A Rigid-Flexible Double-Layer Steric Strategy for Ethylene (Co)oligomerization with Pyridine-Imine Ni(II) and Pd(II) Complexes. New J. Chem. doi:10.1039/D2NJ00183G
Yan, Z., Li, S., and Dai, S. (2021). Synthesis and Characterization of Hyperbranched Polar Functionalized Olefin Oligomers. Chin. J. Synth. Chem. 29, 1033–1044.
Yue, E., Xing, Q., Zhang, L., Shi, Q., Cao, X.-P., Wang, L., et al. (2014). Synthesis and Characterization of 2-(2-benzhydrylnaphthyliminomethyl)pyridylnickel Halides: Formation of Branched Polyethylene. Dalton Trans. 43, 3339–3346. doi:10.1039/c3dt53205d
Zhang, D., Nadres, E. T., Brookhart, M., and Daugulis, O. (2013). Synthesis of Highly Branched Polyethylene Using "Sandwich" (8-P-Tolyl Naphthyl α-diimine)Nickel(II) Catalysts. Organometallics 32, 5136–5143. doi:10.1021/om400704h
Zhang, Y., Wang, C., Mecking, S., and Jian, Z. (2020). Ultrahigh Branching of Main‐Chain‐Functionalized Polyethylenes by Inverted Insertion Selectivity. Angew. Chem. Int. Ed. 59, 14296–14302. doi:10.1002/anie.202004763
Zhao, Y., Li, S., Fan, W., and Dai, S. (2021). Reversion of the Chain Walking Ability of α-diimine Nickel and Palladium Catalysts with Bulky Diarylmethyl Substituents. J. Organomet. Chem. 932, 121649. doi:10.1016/j.jorganchem.2020.121649
Zhong, L., Du, C., Liao, G., Liao, H., Zheng, H., Wu, Q., et al. (2019). Effects of Backbone Substituent and Intra-ligand Hydrogen Bonding Interaction on Ethylene Polymerizations with α-diimine Nickel Catalysts. J. Catal. 375, 113–123. doi:10.1016/j.jcat.2019.05.026
Zhong, L., Li, G., Liang, G., Gao, H., and Wu, Q. (2017). Enhancing Thermal Stability and Living Fashion in α-Diimine-Nickel-Catalyzed (Co)polymerization of Ethylene and Polar Monomer by Increasing the Steric Bulk of Ligand Backbone. Macromolecules 50, 2675–2682. doi:10.1021/acs.macromol.7b00121
Keywords: hybrid “sandwich”, Ni(II) and Pd(II) catalysts, highly branched, high-molecular-weight, pyridine-imine
Citation: Ge Y, Cai Q, Wang Y, Gao J, Chi Y and Dai S (2022) Synthesis of High-Molecular-Weight Branched Polyethylene Using a Hybrid “Sandwich” Pyridine-Imine Ni(II) Catalyst. Front. Chem. 10:886888. doi: 10.3389/fchem.2022.886888
Received: 01 March 2022; Accepted: 19 April 2022;
Published: 04 May 2022.
Edited by:
Nikhil Kumar Singha, Indian Institute of Technology Kharagpur, IndiaReviewed by:
Naeimeh Bahri-Laleh, Iran Polymer and Petrochemical Institute, IranGiovanni Talarico, University of Naples Federico II, Italy
Copyright © 2022 Ge, Cai, Wang, Gao, Chi and Dai. This is an open-access article distributed under the terms of the Creative Commons Attribution License (CC BY). The use, distribution or reproduction in other forums is permitted, provided the original author(s) and the copyright owner(s) are credited and that the original publication in this journal is cited, in accordance with accepted academic practice. No use, distribution or reproduction is permitted which does not comply with these terms.
*Correspondence: Jiangang Gao, Z2FvamlhbmdhbmdAYWhwdS5lZHUuY24=; Yue Chi, eXVlY2hpQGNjdXQuZWR1LmNu; Shengyu Dai, ZGFpeXVAdXN0Yy5lZHUuY24=
†ORCID: Shengyu Dai orcid.org/0000-0003-4110-7691
‡These authors have contributed equally to this work