- The Key Laboratory of Bioactive Substances and Resources Utilization of Chinese Herbal Medicine, Ministry of Education, Institute of Medicinal Plant Development, Chinese Academy of Medical Sciences and Peking Union Medical College, Beijing, China
Four novel triterpenoid alkaloids, siragrosvenins A–D (1–4), and two new cucurbitane-type triterpenoids, siragrosvenins E–F (5, 6), together with eight known analogs (7−14), were isolated from the roots of Siraitia grosvenorii. Compounds 1−4 possessed a rare cucurbitane-type triterpenoid scaffold, featuring an extra pyrazine unit via the Strecker reaction in the cucurbitane framework. Compound 5 displayed a 6/6/6/5/6/5-fused polycyclic ring system, with an uncommon fused furan and pyran ring in the side chain. All the structures were characterized by extensive spectroscopic analysis, including HRESIMS, NMR, and X-ray crystallographic data. It is worth noting that the DP4+ analysis method was applied for the first time to determine the absolute configurations of the trihydroxybutyl moiety in the side chain of compounds 1–4. In vitro cytotoxicity screening found that compounds 4, 8, 9, 13, and 14 exhibited remarkable cytotoxic activities against three cell lines with IC50 values ranging from 1.44 to 9.99 μM. Siragrosvenin D shows remarkable cytotoxic activity on MCF-7 cells. As a result, it inhibited the proliferation of MCF-7 cells and reduced their viability via the induction of G2/M phase arrest and significantly induced apoptosis in MCF-7 cells.
Introduction
Triterpenoids are commonly distributed in higher plants and have attracted much attention due to their structural diversities and broad range of bioactivities (Chen et al., 2005). Cucurbitane triterpenoids, as an important part of the triterpenoid family, are famous for their highly oxygenated skeletons, which are obtained initially from the Cucurbitaceae genus. More importantly, members of this group of natural products have been reported for their diverse pharmacological effects, such as anticancer (Garg et al., 2018), anti-inflammatory (Liu et al., 2020), antihyperglycemic (Sun et al., 2018), and antilipidemic activities (Huang et al., 2012; Cai et al., 2015). In addition, cucurbitane triterpeniods possessing a nitrogen-containing heterocycle are rarely reported. The insertion of heteroatoms can often improve the biological activity of chemicals, which has attracted extensive attention of scholars (Pettit et al., 1988; Urban et al., 2007).
Siraitia grosvenorii Swingle (Cucurbitaceae) is a perennial plant growing in the southern part of China, Guangxi province. The roots of S. grosvenorii are traditionally used to treat tongue fat, meningitis sequelae, diarrhea, and rheumatoid arthritis as a folk medicine in China (Qing et al., 2017). However, few investigations were conducted on the isolation and identification of compounds presented in the roots. In order to search for active natural products from this plant, we isolated four uncommon triterpenoid alkaloids (1–4), two new cucurbitane-type triterpenoids (5, 6) and eight known compounds (7–14) (Figure 1). Among them, compounds 1–4 contained a novel cucurbitane-type triterpenoid skeleton with an additional pyrazine unit via a carbon–nitrogen linkage in the structure. Compound 5 showed an unexpected triterpenoid structure with a 6/6/6/5/6/5-fused polycyclic ring system, through aldol condensation. The cytotoxicity of these compounds was evaluated against three human cancer cell lines (MGC-803, MCF-7, and CNE-1) in vitro. Herein, we presented the isolation, structure elucidation, and cytotoxicity of these cucurbitane-type triterpenoids. Furthermore, we also conducted a preliminary investigation on the effects of siragrosvenin D, which could arrest the cell cycle and significantly induce apoptosis in MCF-7 cells.
Materials and Methods
Plant Material
The roots of S. grosvenorii were collected from the Yongfu county, Guangxi province and identified by Prof. Ma Xiaojun (Institute of Medicinal Plant Development, Chinese Academy of Medical Sciences and Peking Union Medical College) in October 2019. The voucher specimen (accession number 2019004) was stored at the herbarium of our institute.
General Experimental Procedures
UV spectra data were recorded on a Thermo Scientific Genesys 10S spectrophotometer (Thermo Scientific, Madison, WI, United States). Infrared spectra were obtained on a Nicolet iS5 FT-IR spectrophotometer (Thermo Scientific, Madison, WI, United States). HRESIMS data were measured on a Thermo Scientific LTQ-Orbitrap XL (Bremen, Germany). Optical rotations were measured with an Anton Paar MCP 200 automatic polarimeter (Anton Paar GmbH, Graz, Austria) in MeOH at 25°C. 1D and 2D NMR spectra data were recorded on a Bruker AV III 600 NMR spectrometer (Rheinstetten, Germany). Column chromatography was performed by using silica gel (100–200, 300–400 mesh, Qingdao Haiyang Chemical Co., Ltd., Qingdao, China) and Sephadex LH-20 (Pharmacia Biotech, Sweden). Thin-layer chromatography (TLC) was performed over precoated silica gel GF254 plates (0.25 mm, Qingdao Haiyang Chemical Co., Ltd., Qingdao, China). Semi-preparative HPLC was carried out on a LC-UV system (Waters 2,549, United States) with a YMC-C18 column (250 × 10 mm, 5 μm, Japan) and Agilent SB-Phenyl (250 × 10 mm, 5 μm, United States), detected by a binary channel UV detector at 210 and 254 nm. All solvents used for HPLC were of HPLC grade obtained from Thermo Fisher.
Extraction and Isolation
The air-dried roots (20.0 kg) of S. grosvenorii were extracted three times by 90% ethanol under reflux. The solvent was then removed under reduced pressure to yield a crude extract (1,300 g), which was then suspended in water (3 × 2000 ml) and partitioned with petroleum ether, dichloromethane, ethyl acetate, and n-butanol. The CH2Cl2 residue (200 g) was chromatographed on silica gel CC using an increasing gradient of CH2Cl2-MeOH (150:1 to 0:1, v/v) to afford seven main fractions (A−G).
Fraction B (30 g) was submitted to a silica gel column by using CH2Cl2-MeOH (120:1 to 0:1 v/v) as an eluent to generate fractions B1–B5. Subsequently, further purification of B2 was separated as four parts over an MCI gel column with a MeOH-H2O gradient system (7:3–0:1 v/v). B2-1 was purified by a Sephadex LH-20 column (4 × 120 cm) to yield compound 7 (10 mg). Compound 1 (3.0 mg, tR 23 min) and compound 2 (8.0 mg, tR 25 min) were isolated from fraction B2-3 (24 mg) via semi-preparative HPLC with 40% MeOH at 2 ml/min. B2-4 (33 mg) was chromatographed by semi-preparative HPLC (CH3CN-H2O, 65:35, v/v) to furnish compound 3 (3.2 mg, tR 15 min), compound 4 (2.4 mg, tR 17 min), and compound 8 (6 mg, tR 22 min). B2-5 was subjected to an additional separation by preparative HPLC (MeOH-H2O, 55:45, v/v, 5 ml/min) to afford three parts, which were further purified by semi-preparative HPLC to yield compound 9 (20 mg, tR 11.1 min), compound 11 (15 mg, tR 12 min), and compound 5 (5 mg, tR 15.3 min).
Fraction B4 (7 g) was applied to separation by the MCI gel column using MeOH–H2O with a stepwise elution gradient (7:3–0:1 v/v) to yield eight parts. The 60% MeOH fraction (B4-3, 2.5 g) was fractionated on silica gel columns to obtain eight fractions. Fraction B4-3-3 (30 mg) was chromatographed by semi-preparative HPLC (MeOH-H2O, 45:55) to furnish compound 10 (4.5 mg, tR 24 min). Fraction B4-4 was subjected to Sephadex LH-20 (4 × 120 cm). We merged the same fractions according to their TLC profiles and then acquired compound 6 (6 mg, tR 19 min), 12 (5 mg, tR 28 min), and 13 (5 mg, tR 30 min) from fraction B4-4 using semi-preparative HPLC (CH3CN -H2O, 70:30). B4-5 was separated by semi-preparative HPLC (20% MeOH–80% H2O) to yield 14 (4 mg, tR 15.5 min).
Compound Characterization
Siragrosvenin A (1): pale-yellow powder; (α)25D +43 (c 0.1, MeOH); UV (MeOH) λmax (log ε) 205 (1.19) and 282 (0.90) nm; IR νmax 3,419, 2,968, 2,928, 1,697, 1,392, 1,370, 1,260, and 1,205 cm−1; 1H NMR (Pyrdine-D5, 600 MHz) and 13C NMR (Pyrdine-D5, 150 MHz) data are shown in Tables 1, 2; HRESIMS (positive mode) m/z 707.3880 (M + Na)+ (calculated for C38H56N2O9Na, 707.3883).
Siragrosvenin B (2): pale-yellow powder; (α)25D +25 (c 0.1, MeOH); UV (MeOH) λmax (log ε) 205 (1.32) and 282 (1.01) nm; IR νmax 3,444, 2,976, 2,942, 1,698, 1,369, 1,254, and 1,022 cm−1; 1H NMR (Pyrdine-D5, 600 MHz) and 13C NMR (Pyrdine-D5, 150 MHz) data are shown in Tables 1, 2; HRESIMS (positive mode) m/z 705.3724 (M + Na)+ (calculated for C38H54N2O9Na, 705.3727).
Siragrosvenin C (3): pale-yellow powder; (α)25D +5 (c 0.1, MeOH); UV (MeOH) λmax (log ε) 207 (2.09) and 282 (1.35) nm; IR νmax 3,420, 2,966, 2,928, 1,697, 1,388, 1,371, 1,261, and 1,024 cm−1 1H NMR (Pyrdine-D5, 600 MHz) and 13C NMR (Pyrdine-D5, 150 MHz) data are shown in Tables 1, 2; HRESIMS (positive mode) m/z 707.3882(M + Na)+ (calculated for C38H56N2O9Na, 707.3883).
Siragrosvenin D (4): pale-yellow powder; (α)25D +9 (c 0.1, MeOH); UV (MeOH) λmax (log ε) 207 (2.35) and 282 (0.90) nm; IR νmax 3,419, 2,967, 2,927, 1,687, 1,372, 1,260, and 1,023 cm−1; 1H NMR (Pyrdine-D5, 600 MHz) and 13C NMR (Pyrdine-D5, 150 MHz) data are shown in Tables 1, 2; HRESIMS (positive mode) m/z 705.3730 (M + Na)+ (calculated for C38H54N2O9Na, 705.3727).
Siragrosvenin E (5): white powder; (α)25D +159 (c 0.1, MeOH); UV (MeOH) λmax (log ε) 207 (2.13) nm; IR νmax 3,419, 2,965, 2,882, 1,688, 1,055, and 1,029 cm−1; 1H NMR (600 MHz, Pyrdine-D5) and 13C NMR (150 MHz, Pyrdine-D5) data are shown in Tables 1, 2; HRESIMS (positive mode) m/z 541.3126 (M + Na)+ (calculated for C30H46O7Na, 541.3141).
Siragrosvenin F (6): white powder; (α)25D +59 (c 0.1, MeOH); UV (MeOH) λmax (log ε) 228 (2.60) nm; IR νmax 3,391, 2,966, 2,882, 1,687, 1,373, 1,068, and 1,027 cm−1; 1H NMR (600 MHz, Pyrdine-D5) and 13C NMR (150 MHz, Pyrdine-D5) data are shown in Tables 1, 2; HRESIMS (positive mode) m/z 543.3301 (M + Na)+ (calculated for C30H48O7Na, 543.3297).
13C NMR Calculations
The 13C NMR spectra were calculated according to the reported methods (Buevich and Elyashberg, 2016). The computational data were fitted in the GraphPad Prism 7. The process is also described in detail in Supplementary Material.
X-Ray Crystallographic Analysis
Colorless needle crystals of compound 5 were crystallized in CH3CN–H2O (5:1) at room temperature. Crystal data: C30H46O7, hexagonal, a = 22.7327 (2) Å, b = 22.7327 (2) Å, c = 9.80650 (10) Å, β = 90°, U = 4,388.81 (9) Å3, T = 99.99 (10), space group P61, Z = 6, μ (Cu Kα) = 1.54184, and Flack parameter = -0.03 (4). A total of 49,416 reflections were measured, 6,100 unique (Rint = 0.0394) which were used in all calculations. The final R1 was 0.0331 (I > 2σ (I)), and the wR2 was 0.0916 (all data). Crystal size: 0.32 × 0.06 × 0.05 mm3.
The crystallographic data have been deposited in the Cambridge Crystallographic Data Center (CCDC), and the CCDC deposition number is CCDC 2151134. These data can be obtained free of charge via http://www.ccdc.cam.ac.uk/conts/retrieving.html.
Cell Viability Assays
The cellular viability of compounds 1–14 was evaluated using the MTT procedure with MGC-803, MCF-7, and CNE-1 cancer cell lines. The cells were cultured in DMEM supplemented with 10% fetal bovine serum and cultured at a density of 3 × 104 cells/mL in a 96-well microtiter plate. After 24 h of incubation, six various concentrations of each agent dissolved in dimethyl sulfoxide (DMSO) were then added in the wells. Each concentration was evaluated three times. After incubation under 5% CO2 at 37°C for 48 h, 20 μL of MTT (5 mg/ml) was added into each well, and the cells were incubated for an additional 4 h. Then, after the liquid was removed, DMSO (200 μL) was placed into the wells. After shaking for 5 min, the absorbance was measured with a microplate reader at 570 nm (SpectraFluor, TECAN, Sunrise, Austria).
Cell Cycle Analysis
The MCF-7 cells were plated in a six-well plate at a density of 3 × 104 cells per well and treated with compound 4 (0, 5, 10, and 20 μM). After 24 h, the cells were fixed in ice-cold ethanol (70%) at 4°C overnight. After the cells were suspended in 0.1% Triton X-100 and 100 μg/ml RNase A, 5 μL PI solution was added and incubated for 30 min (Ueno et al., 2021). Then, the sample was analyzed by a flow cytometer FACS Verse. Modifit LT 4.0 was used to analyze the obtained data.
Apoptosis Analysis
The MCF-7 cells were pre-treated with compound 4 (0, 5, 10, and 20 μM) for 24 h. After washing with PBS, the cells were incubated with 5 μL annexin V in binding buffer for 30 min at room temperature in the dark, followed by 10 μL PI for 5 min. FACS Calibur flow cytometry (Becton Dickinson, United States) was used to detect and analyze the stained cells. The apoptosis rate was reported as the percentage of apoptotic cells to the total number of cells.
Colony Formation Assay
The MCF-7 cells were plated in a six-well plate at a density of 250 cells per well and were treated with different concentrations of compound 4 (0, 0.1, 0.5, 1, 2, and 4 μM) for 14 days. The drugs were removed, and cells were washed twice with PBS. Then, the cells were fixed in methanol for 10 min and stained with 0.1% crystal violet solution for 30 min at room temperature. Finally, PBS was used to wash the cells to visualize the colonies (Ni et al., 2018).
Cell Morphology Observation and AO/EB Staining Assay
The MCF-7 cells were seeded at a density of 2 × 104 cells/well onto 24-well plates and were treated with different concentrations of compound 4 (0, 5, 10, and 20 μM) for 24 h. After discarding the cell culture medium, some cells were added with 500 μL AO/EB staining solution for 5 min in the dark. Subsequently, photographs were taken under a fluorescence microscope.
Statistical Analysis
All data were analyzed by GraphPad Prism version 5.0 and were presented as the mean ± SD in at least three independent experiments. Student’s t-test and one way ANOVA were conducted to evaluate significant distinctions. Values of p < 0.05 were considered as statistically significant.
Results and Discussion
Compound Structure Elucidation
Compound 1 was isolated as a pale-yellow powder. Its molecular formula C38H56N2O9 was indicated by its HRESIMS at m/z 707.3880 (M + Na)+, calculated for C38H56N2O9Na 707.3883, suggesting 12 degrees of unsaturation. The absorption bands at 3,419 and 1,697 cm−1 in the IR spectrum suggested the existence of the hydroxyl and carbonyl groups in compound 1. The characteristic absorption bands at 205 and 280 nm in the UV spectrum provided evidence for the existence of the aromatic group. The 1H NMR spectrum (Table 1) displayed signals attributed to nine methyl groups at δH 1.25, 1.27, 1.46, 1.47, 1.48, 1.50, 1.63, 1.68, and 1.90 (each 3H, s); a set of oxygenated proton signals at δH 4.96 (1H, t), 4.83 (1H, t), 4.52 (1H, m), 4.40 (1H, m), and 4.36 (1H, m) suggested its polyhydroxy property. One olefinic proton at δH 5.92 (1H, m) indicated the presence of double bonds, and one downfield proton at δH 8.78 (1H, s) implied the heterocyclic aromatic ring. The 13C-APT NMR spectrum (Table 1) revealed 38 carbon resonances, including two keto carbonyls at δC 213.7 and 215.4, one ester carbonyl at δC 170.5, and six olefinic carbons and aromatic carbons at δC 155.7, 153.8, 151.4, 144.2, 142.1, and 121.0. The rest of the signals were ascribed to nine methyls, eight methylenes (including one oxygenated carbon at δC 65.5), five methines (including three oxygenated carbons at δC 76.8, 73.5, and 70.7), and six quaternary carbons (including two oxygenated carbons at δC 80.5 and 81.9). All protons are assigned to their corresponding carbons with the help of the HSQC spectrum. In fact, the results after the comparison of these data with the known ones suggested that compound 1 showed the same B–C–D ring and the C-17 side chain structure as 23,24-dihydrocucurbitacin E (Wu et al., 2004), except for the additional four aromatic carbons (δC 155.7, 153.8, 151.4, and 144.2) and a trihydroxybutyl side chain. In the HMBC spectrum, the correlations from δH 8.78 (1H, s) to C-2 (δC 151.4) and C-33 (δC 153.8) implied that the four downfield aromatic carbons formed a closed-loop system. Considering the two N atoms in the molecular formula, an extra pyrazine unit was established in the structure (Seeman et al., 1992). The HMBC correlations (Figure 1) from H3-28, H3-29 to C-3 (δC 155.7) and C-5 and from H-1 to C-2 (δC 151.4) revealed that the pyrazine ring was adjacent to ring A. The presence of 36,37,38-trihydroxybutyl moiety was established by the 1H−1H COZY (Figure 2) correlations of H-36/H-37/H-38 and the key HMBC correlations of H-35 to C-36 and C-37, H-36 to C-35 and C-37, and H-38 to C-36 and C-37 (Wang et al., 2014). Subsequently, the trihydroxybutyl moiety was confirmed to attach to C-33 from the HMBC correlations of H-35 to C-33, C-32, and C-3 and H-32 to C-1, C-2, and C-3. Thus, the planar structure of 1 was established unambiguously.
The relative configuration of 1 was evaluated by its NOESY spectrum (recorded in Pyrdine-d5). The NOESY correlations (Figure 2) from H3-19 to H3-18, H3-19 to H-8, H3-30 to H-10, H3-30 to H-17, and H3-18 to H-16 revealed that H3-18, H3-19, H-8, and H-16 are β-oriented, H3-30 and H-10, and H-17 are α-oriented. We tried to determine the absolute configuration of compound 1 by crystallization but failed. However, the clear comparison of NMR data with cucurbitacin E, whose absolute configuration has been fully determined, indicated that the cucurbitane partial structure at C-8, C-9, C-10, C-13, C-14, C-16, and C-17 of compound 1 was S, R, R, R, S, R, and R, respectively (Wu et al., 2004), which was completely consistent with the biogenic pathway of cucurbitane triterpenes. The relative configuration of the 36,37,38-trihydroxybutyl moiety was determined by J-based NMR. The low-temperature NMR (−4°C, CD3OD) of 1 revealed a large coupling constant between H-36 and H-37 (J = 9.2 Hz) (Wang et al., 2014), indicating an antirelationship between the two protons (Li et al., 2015). In addition, a significant NOESY correlation of H-37/H-35/H-38/H-36 indicated a threo configuration (Figure 3) of H-36 and H-37 (36S, 37S or 36R, 37R). The absolute configuration of this fragment was further determined by DP4 calculations (Marcarino et al., 2021). NMR shielding constants were computed using the GIAO method at the mPW1PW91/6–311 + G** level in the gas phase by the GAUSSIAN09 program (Zanardi et al., 2018). In the application of the DP4+ analysis method, we selected the only partial data of the 36,37,38-trihydroxybutyl moiety to carry out DP4 calculations of the two possible configurations (36S, 37S and 36R, 37R). Based on the calculation results, compound 1a (36S, 37S) showed satisfying linear regression analysis (R2 0.9985) of the experimental data and calculated 13C chemical shifts (Figure 4), indicated better fit by comparison of experimental and calculated NMR data (Table 3), and then was designated as the most promising candidate. Therefore, the structure of compound 1 was assigned as shown in Figure 2.
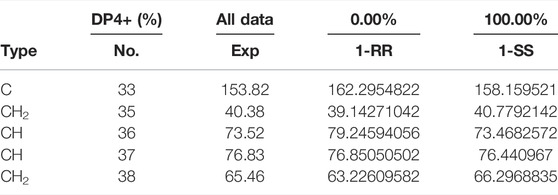
TABLE 3. Comparison of calculated 13C NMR chemical shifts with experimentally observed shifts for 1.
Compound 2, pale-yellow powder, had the molecular formula of C38H54N2O9 based on its HRESIMS data [m/z 705.3724 (M + Na)+, calculated for C38H54N2O9Na 705.3727], suggesting 13 degrees of unsaturation. The 1H NMR and 13C-APT NMR spectra data (Table 1) showed resemblance with those of 1, except that compound 2 possessed an additional double bond at δC 122.8 and 150.2. In HMBC, the appearance of α–β unsaturated ketone at C-22–C-23 was further verified by the cross-peaks of H-23 (δH 7.34, 1H, d, J = 15.8 Hz), H-24 (δH 7.41, 1H, d, J = 15.8 Hz) to C-22 (δC 204.7) and correlations of the signals at H3-26 (δH, 1.55) and H3-27 (δH, 1.52) with the resonances at C-24 (δC 150.2). Additional NOESY correlations of H3-19/H3-18, H3-19/H-8, H3-30/H-10, H3-30/H-17, and H3-18/H-16 indicated that H3-18, H3-19, H-8, and H-16 are β-oriented, while H3-30, H-10, and H-17 are α-oriented. The similar absorption peaks (205 nm, Δε 9.0; 300 nm, Δε 1.2) in the CD spectrum of compound 2 indicated that the absolute configurations of compound 2 was identical with those of 1. Thus, the structure of 2 was established as described.
Compound 3 was isolated as pale-yellow powder and assigned an identical molecular formula of C38H56N2O9 similar to that of 1 based on the HRESIMS ion at m/z 707.3880 (calculated for C38H56N2O9Na 707.3883). Extensive analysis of its NMR data indicated that the structure of 3 was similar to that of 1, except for the downfield shifts of C-33 (Δδ + 0.8) and C-3 (Δδ + 1.6) and upfield shifts of C-32 (Δδ−0.9) and C-2 (Δδ−1.5), which indicated that the location of the trihydroxybutyl group may be different. By the HMBC experiment, the cross-peaks from H-35 (δH 3.79, 3.42) to C-32 (δC143.3), C-33 (δC 154.6), and C-2 (δC 149.9) and from H-33 (δH 8.64, s) to C-3 (δC 157.3) and C-4 (δC 42.9) proved that the fragment of the 36,37,38-trihydroxybutyl unit was linked to C-32. Similar NOESY correlations and identical CD spectra of compounds 3 and 1 suggested their undifferentiated absolute configuration. Thus, the structure of 3 was elucidated as shown.
Compound 4 was obtained as a pale-yellow powder. The molecular formula of 4 was established to be C38H54N2O9 from its HRESIMS data. The NMR spectroscopic data (Table 1) of 4 displayed high similarity to those of 3, except for an additional double bond at the side chain, which indicated that compound 4 was a dehyrdrogenated product of compound 3, as proven by the HMBC experiment. In the HMBC spectrum, the cross peaks from H-23 (δH 7.35, 1H, d, J = 15.8 Hz) and H-24 (δH 7.41, 1H, d, J = 15.8 Hz) to C-22 (δC 204.8) and from H3-26 (δH, 1.55) and H3-27 (δH, 1.52) to C-24 (δC 150.2) supported this deduction. The identical CD spectra and similar optical rotation of compounds 3–4 indicated that they owed the same absolute configuration. Therefore, the structure of compound 4 was determined as depicted and given the trivial name siragrosvenin D.
Compound 5 was isolated as a white amorphous powder, and it has a molecular formula of C30H46O7 as deduced on the basis of (M + Na)+ ion peak at m/z 541.3126 (calculated C30H46O7Na 541.3141) in HRESIMS, which indicated eight degrees of unsaturation. The 1H NMR data (Table 1) displayed six methyl groups (δH 1.22, 1.24, 1.31, 1.34, 1.36, and 1.47, each 3H, s), one olefinic methine [δH 5.69 (1H, m, H-6)], three oxygenated methines [δH 4.09 (1H, overlap, H-2), 3.43 (1H, m, H-3), and 5.00 (1H, m, H-16)], and two methylenes [δH 3.79 (1H, m, H-26a), 3.74 (1H, m, H-26b) and 4.22 (1H, m, H-27a), 4.09 (1H, overlap, H-27b)]. The 13C-APT NMR spectrum revealed 30 carbon signals attributed to one ketone carbon (δC 213.2), one pair of double bonds (δC 142.9 and 119.0), three oxygenated methines (δC 81.8, 71.3, and 70.8), two oxygenated quaternary carbons (δC 72.6 and 110.0), two oxygenated methylenes (δC 71.1 and 64.8), and six methyls (δC 20.2, 21.0, 21.7, 22.6, 25.8, and 29.0). Based on these data, compound 5 was classified as a cucurbitane triterpenoid (Clericuzio et al., 2004). Comparing the results of 1H and 13C NMR spectrum data of compound 5 with those of jinfushanencin F suggested their identical A/B/C/D rings (Li et al., 2016), except for the specific quaternary carbon signal at δC 110.0 in compound 5. Considering the unsaturation of 5, we inferred that there was an extra ring in the side chain. In the 2D NMR spectra, the fragment of C-24–C-25 (-C-27)-C-26 was established in the 1H-1H COZY experiment, while the HMBC correlations from H-27 (δH 4.22, 4.09) and H-24 (δH 2.33, 1.71) to δC 110.0 suggested the presence of a spiro ring as formed by aldol condensation at C-23 (Figure 5). Thus, the planar structure of 5 was elucidated as an uncommon triterpenoid structure, which showed the spiro ring in the side chain.
The relative configuration was elucidated based on the NOESY correlations. Intense correlation of H-2/H-10 and H-3/Me-19 indicated that 2-OH was in β-orientation and 3-OH was in α-orientation. In addition, the cross peaks of H-17/Me-30, H-10/Me-30, H-8/Me-18, and H-8/Me-19 suggested the β-orientation for H-10, Me-30, and H-17 and α-orientation for H-8, Me-18, and Me-19. Fortunately, a suitable crystal of 5 was obtained and the single-crystal X-ray diffraction analysis was performed using Cu–Kα radiation (Figure 6), which established the absolute configuration of 5 to be 2S, 3S, 8S, 9R, 10R, 13R, 14S, 16R, 17R, 20R, and 25S. Consequently, the structure of 5 was named as siragrosvenin E.
Compound 6 was obtained as a white amorphous powder and given the molecular formula of C30H48O7 based on HRESIMS. The 1H NMR spectrum of 6 also showed signals of a typical cucurbitacin triterpenoid with seven methyls at δH 1.27, 1.29, 1.32, 1.39, 1.49 (overlap), and 1.79 and two olefin protons at δH 5.15 (1H, m, H-23) and 6.06 (1H, m H-24). In addition, there were four oxygenated methines at δH 4.12 (1H, overlap, H-2), 3.45 (1H, d, J = 9 Hz, H-3), 4.92 (1H, m, H-16), and 5.15 (1H, m, H-23) and one methylene at δH 4.30 (2H, s, H-26). Its 13C NMR spectra exhibited thirty carbon signals ascribed to seven methyls, five methylenes, four alkene carbon atoms, six oxygenated carbons, and five quaternary carbons (a carbonyl and an oxygenated carbon). The spectroscopic data displayed a resemblance to those of the known compound jinfushanencin F (Li et al., 2016), except for the more 18 amu than jinfushanencin F, which indicated that compound 6 could be a hydrolyzate of jinfushanencin F in the C-16–O-23 moiety. The key HMBC correlations from H-23 (δH 5.15) to C-20 (δC 71.8), C-22 (δC 50.0), C-24 (δC 126.2), and C-25 (δC 138.5) suggested that one hydroxy group was connected to C-23, and HMBC correlations from H-16 (δH 4.92) to C-13 (δC 49.1), C-14 (δC 48.9), C-17 (δC 56.3), and C-20 (δC 71.8) showed that another hydroxy group was attached to C-16 (Figure 5). The relative configurations of 6 were similar to those of compound 5 according to their similar NOESY correlations and NMR data. The ECD spectrum of 6 also showed same cotton effects to those of 5, suggesting identical absolute configuration. However, with rotational freedom in the side chain, it was not possible to definitively assign the C-23 configuration for either epimer. Thus, the structure of 6 was elucidated as depicted, named siragrosvenin F.
In addition, eight known compounds were isolated from the roots of S. grosvenorii. Their structures were identified as 23,24-dihydrocucurbitacin F (7) (Guerrero-Analco et al., 2007), cucurbitacin IIa (8) (Zeng et al., 2021), cucurbitacin Q1 (9) (Add El-Fattah, 1994), jinfushanencin F (10) (Li et al., 2016), siraitic acid A (11) (Si et al., 1999), siraitic acid B (12) (Si et al., 1999), cucurbitacin E (13) (Attard et al., 2005), and 23,24-dihydrocucurbitacin E (14) (Tang et al., 2015).
Proposed Biosynthetic Pathways for the Formation of Compounds 1–4
As far as we know, none of the identified cucurbitane-type pyrazine triterpenoid alkaloids has been reported as natural products so far in the literature. Consequently, we tried to deduce the potential biosynthetic pathway of compound 1 (Figure 7). First, the free amino acids in plants could react with the carbonyl group of hexose sugar. After the Strecker reaction and dehydration reaction, we obtained the amide-type conjugation (A) (Fujii and Kobatake, 1972; Wakamatsu et al., 2019). Simultaneously, 2, 3-oxysqualene was protonated, cyclized, rearranged, and deprotonated under the catalysis of various 2,3-oxidosqualene cyclases (OSCs) to obtain triterpenoid precursors, such as cucurbitenol. Then, cucurbitacin IIa was obtained under the catalysis of various cytochrome oxygenases P450 (CPY450), and the oxidation of its C-2 hydroxyl group will produce the derivative iso-23,24-dihydrocucurbitacin B (B), which could trigger the next chemical reaction. Subsequently, the precursor (B) was further aminated to form the structure C. The formation process of the pyrazines can be explained by the occurrence of Schiff’s base reaction by the degradation of the carbonyl group and amide group (Ganesan, 1996), which was easier to take place in the plants to yield compound 1. Finally, structures 2–4 could be generated by a similar mechanism as that described for structure 1.
Cytotoxicity Evaluation of all Isolates
Further studies were also performed using the MTT viability assay to evaluate the cytotoxicity of compounds 1−14 against MGC-803 (human gastric cancer cells), MCF-7 (human breast cancer cells), and CNE-1 (human nasopharyngeal carcinoma cells), and taxol (diterpene alkaloid) was used as a positive control. As shown in Table 4, compounds 4, 8, 9, 13, and 14 exhibited obvious in vitro cytotoxicity, with IC50 values ranging from 1.44 to 9.99 μM. Cucurbitacin E, with the lowest IC50 values among those compounds, has been investigated extensively for its cytotoxicity toward several cancer cell lines through various underlying molecular mechanisms (Sun et al., 2010; Zhang et al., 2012; Attard and Martinoli, 2015). Through the analysis of the structure–activity relationship, α–β unsaturated ketone as functional groups can significantly enhance the cytotoxicity of cucurbitane-type compounds (Lin et al., 2015). In addition, compound 4 also showed the potential of cytotoxicity with an IC50 of 8.04 μM.
Cytotoxicity Against MCF-7 Cells of Siragrosvenin D (4)
Cucurbitacin E, as the most anticancer potential natural products of the isolated compounds, has been investigated extensively for its cytotoxic activities toward several cancer cell lines through the various underlying molecular mechanisms (Huang et al., 2012; Attard and Martinoli, 2015). Therefore, to investigate the cytotoxicity of the siragrosvenin D, the cells were exposed to different concentrations of this compound. In cell cycle analysis, siragrosvenin D significantly arrested the growth of cells at the G2/M phase, increasing from 12.70% of cells treated with the negative control to 13.44, 36.25, and 41.56% of cells treated with 5, 10, and 20 μM of siragrosvenin D, respectively (Figure 8). These results implied that siragrosvenin D inhibited proliferation of MCF-7 cells via the induction of G2/M phase arrest.
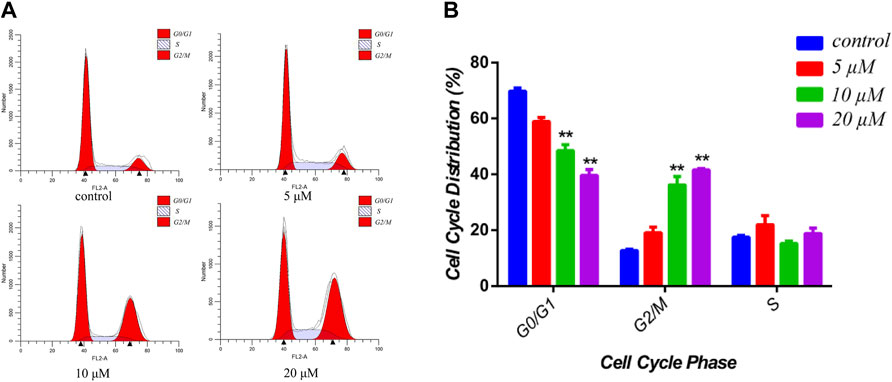
FIGURE 8. Effects of siragrosvenin D on cell cycle distribution in MCF-7 cells. (A) MCF-7 cells were treated by siragrosvenin D (0, 5, 10, and 20 μM) for 24 h and then analyzed by flow cytometry for cell cycle distribution. (B) Data were presented as the mean ± SD of three independent experiments. **p < 0.01 vs. control was considered statistically significant.
In order to study the mechanism of the promoting effect of siragrosvenin D on cell apoptosis, first, we observed the morphology of the cells after being treated with siragrosvenin D (0, 5, 10, and 20 μM). Comparing to negative control, with the increase of the concentration of siragrosvenin D, the number of cells started to decrease, accompanied with contraction and exfoliation (Figure 9). The results of AO/EB staining also demonstrated that siragrosvenin D significantly increased the percentage of apoptotic cells in the treated cells, which showed red fluorescence. Moreover, cell apoptosis was analyzed by flow cytometry. As shown in Figure 10, siragrosvenin D induced apoptosis of MCF-7 cells evidently. The percentage of apoptotic and necrotic cells in the treated cells was increased in a dose-dependent manner compared with that in the control group.
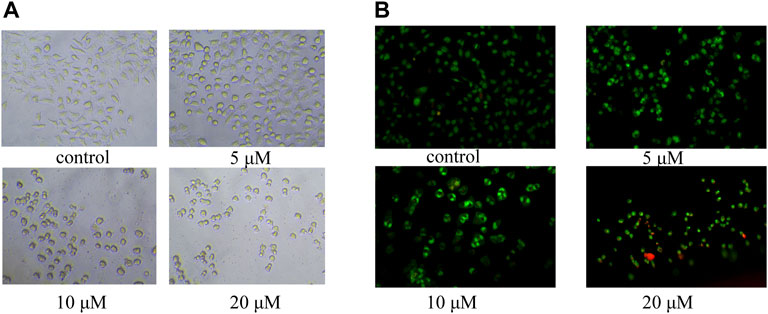
FIGURE 9. Apoptosis induced by siragrosvenin D in MCF-7 cells detected by the fluorescence microscope. (A) Morphology of the cells after being treated with siragrosvenin D (0, 5, 10, and 20 μM) for 24 h. (B) Percentage of apoptotic cells by AO/EB staining after being treated with siragrosvenin D (0, 5, 10, and 20 μM) for 24 h.
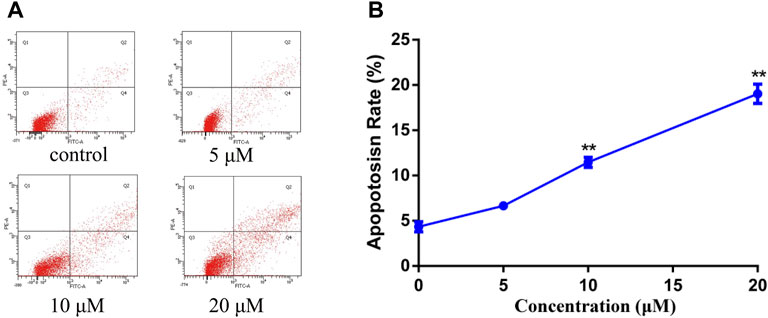
FIGURE 10. Apoptosis induced by siragrosvenin D in MCF-7 cells detected by the annexin V-FITC/PI staining test. MCF-7 cells were treated with siragrosvenin D (0, 5, 10, and 20 μM) for 24 h. (A) Apoptotic rates were determined by annexin V-FITC/PI staining. Apoptosis rate was reported as the percentage of apoptotic cells (AV+/PI− and AV+/PI+) among total cells. (B) Data were presented as the mean ± SD (n = 3). **p < 0.01.
To assess the effect of siragrosvenin D on the proliferation of MCF-7 cells, we treated the cells with different concentrations of siragrosvenin D (0, 5, 10, and 20 μM) and evaluated its cell viability by the MTT assay for 24 and 48 h. It was observed that the growth of MCF-7 cells was suppressed in a dose- and time-dependent manner (Figure 11A). In addition, colony formation assay showed that after 14 days of incubation with siragrosvenin D, the number of colonies in the treated groups was significantly less than that in the control group (Figure 11B).
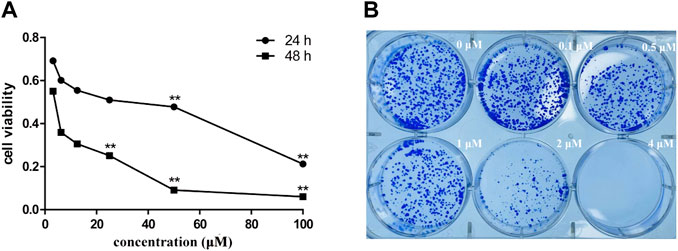
FIGURE 11. Cytotoxicity of siragrosvenin D. (A) MTT assay was performed to assess cell viability in MCF-7 cells treated with different concentrations of siragrosvenin D for 24 and 48 h. (B) Effect of siragrosvenin D on colony formation. All data are presented as mean ± SD. **p < 0.01.
Conclusion
In summary, four novel cucurbitane-type triterpenoid pyrazine alkaloids, siragrosvenins A–D (1–4), along with two new cucurbitacins, siragrosvenins E–F (5–6), were isolated from the roots of S. grosvenorii. Among them, compounds 1–4 contained a novel cucurbitane-type triterpenoid skeleton with an additional pyrazine unit via a carbon–nitrogen linkage in the structure. Compound 5 showed an unexpected triterpenoid structure with a 6/6/6/5/6/5-fused polycyclic ring system, through aldol condensation. Although the pyrazine moiety is ever reported in plants (Li et al., 2006), siragrosvenins A–D (1−4) are the first examples of cucurbitane-type triterpenoid pyrazine alkaloids isolated from the herbs and may provide new chemotypes for the development of novel promising anticancer agents. Siragrosvenin B and D showed more significant cytotoxicity against the tested cell lines, which further confirmed that the presence of the α,β-unsaturated ketone moiety could improve the antitumor activity. A widely accepted mechanism for these compounds was the occurrence of the Michael addition between the α,β-unsaturated ketone fraction and the soft nucleophiles, such as mercaptan and protein sulfhydryl groups, resulting in the inactivation of the SH enzyme or SH coenzyme (Wijeratne et al., 2012).
Furthermore, we also conducted a preliminary investigation on siragrosvenin D, and the results implied that siragrosvenin D inhibited proliferation of MCF-7 cells and reduced their viability via the induction of G2/M phase arrest and significantly induced apoptosis in MCF-7 cells.
Data Availability Statement
The original contributions presented in the study are publicly available. These data can be found here: https://www.ccdc.cam.ac.uk/structures/, 2151134.
Author Contributions
HJW: methodology and writing—original draft. GM: writing—review and editing. HXW: formal analysis. LL: formal analysis. AD: data curation. HL: validation. XH: software. JS: supervision and writing—review and editing. JW: supervision and writing—review and editing.
Funding
This work was financially supported by the National Natural Science Foundation of China (Grant No. 81903920).
Conflict of Interest
The authors declare that the research was conducted in the absence of any commercial or financial relationships that could be construed as a potential conflict of interest.
Publisher’s Note
All claims expressed in this article are solely those of the authors and do not necessarily represent those of their affiliated organizations, or those of the publisher, the editors, and the reviewers. Any product that may be evaluated in this article, or claim that may be made by its manufacturer, is not guaranteed or endorsed by the publisher.
Supplementary Material
The Supplementary Material for this article can be found online at: https://www.frontiersin.org/articles/10.3389/fchem.2022.885487/full#supplementary-material
References
Add El-Fattah, H. (1994). Structure Revision of Cucurbitacin Q1. Phytochemistry 36 (1), 159–161. doi:10.1016/s0031-9422(00)97030-4
Attard, E., Brincat, M. P., and Cuschieri, A. (2005). Immunomodulatory Activity of Cucurbitacin E Isolated from Ecballium Elaterium. Fitoterapia 76 (5), 439–441. doi:10.1016/j.fitote.2005.02.007
Attard, E., and Martinoli, M.-G. (2015). Cucurbitacin E, an Experimental Lead Triterpenoid with Anticancer, Immunomodulatory and Novel Effects against Degenerative Diseases. A Mini-Review. Ctmc 15 (17), 1708–1713. doi:10.2174/1568026615666150427121331
Buevich, A. V., and Elyashberg, M. E. (2016). Synergistic Combination of CASE Algorithms and DFT Chemical Shift Predictions: A Powerful Approach for Structure Elucidation, Verification, and Revision. J. Nat. Prod. 79 (12), 3105–3116. doi:10.1021/acs.jnatprod.6b00799
Cai, Y., Fang, X., He, C., Li, P., Xiao, F., Wang, Y., et al. (2015). Cucurbitacins: A Systematic Review of the Phytochemistry and Anticancer Activity. Am. J. Chin. Med. 43 (7), 1331–1350. doi:10.1142/s0192415x15500755
Chen, J. C., Chiu, M. H., Nie, R. L., Cordell, G. A., and Qiu, S. X. (2005). Cucurbitacins and Cucurbitane Glycosides: Structures and Biological Activities. Nat. Prod. Rep. 22 (3), 386–399. doi:10.1039/b418841c
Clericuzio, M., Mella, M., Vita-Finzi, P., Zema, M., and Vidari, G. (2004). Cucurbitane Triterpenoids from Leucopaxillus Gentianeus. J. Nat. Prod. 67 (11), 1823–1828. doi:10.1021/np049883o
Fujii, S., and Kobatake, H. (1972). Deoxygenations of 2-(d-Arabino-Tetrahydroxybutyl)pyrazine 4-N-Oxide and 1-N-Oxide. J. Org. Chem. 37 (16), 2635–2637. doi:10.1021/jo00981a029
Ganesan, A. (1996). The Dimeric Steroid-Pyrazine Marine Alkaloids: Challenges for Isolation, Synthesis, and Biological Studies. Angew. Chem. Int. Ed. Engl. 35 (6), 611–615. doi:10.1002/anie.199606111
Garg, S., Kaul, S., and Wadhwa, R. (2018). Cucurbitacin B and Cancer Intervention: Chemistry, Biology and Mechanisms (Review). Int. J. Oncol. 52 (1), 19–37. doi:10.3892/ijo.2017.4203
Guerrero-Analco, J., Medina-Campos, O., Brindis, F., Bye, R., Pedraza-Chaverri, J., Navarrete, A., et al. (2007). Antidiabetic Properties of Selected Mexican Copalchis of the Rubiaceae Family. Phytochemistry 68 (15), 2087–2095. doi:10.1016/j.phytochem.2007.05.006
Huang, W.-W., Yang, J.-S., Lin, M.-W., Chen, P.-Y., Chiou, S.-M., Chueh, F.-S., et al. (2012). Cucurbitacin E Induces G2/M Phase Arrest through STAT3/p53/p21 Signaling and Provokes ApoptosisviaFas/CD95 and Mitochondria-dependent Pathways in Human Bladder Cancer T24 Cells. Evidence-Based Complement. Altern. Med. 2012, 1–11. doi:10.1155/2012/952762
Li, J., Zuang, C. L., Tang, H., Sun, P., and Zang, W. (2015). Application of Coupling Constant Based Analysis in Determining the Relative Configuration of Flexible Natural Molecules. J. Int. Pharm. Res. 42 (6), 713–725. doi:10.13220/j.cnki.jipr.2015.06.005
Li, Y.-D., Yi, S.-R., Sun, X.-B., Zhou, X.-Y., Zhang, H.-Y., Wang, Y.-Q., et al. (2016). Bioactive Cucurbitane Triterpenoids from the Tubers of Hemsleya Penxianensis. Phytochemistry Lett. 18, 5–9. doi:10.1016/j.phytochem.2017.12.01410.1016/j.phytol.2016.08.011
Li, Z. L., Li, D. Y., Li, X., Li, N., and Meng, D. L. (2006). A New Alkaloid from the Husk of Xanthoceras Sorbifolia. Yao Xue Xue Bao 41 (12), 1197–1200.
Lin, Z., Guo, Y., Gao, Y., Wang, S., Wang, X., Xie, Z., et al. (2015). Ent-Kaurane Diterpenoids from Chinese Liverworts and Their Antitumor Activities through Michael Addition as Detected In Situ by a Fluorescence Probe. J. Med. Chem. 58 (9), 3944–3956. doi:10.1021/acs.jmedchem.5b00208
Liu, W., Deng, S., Zhou, D., Huang, Y., Li, C., Hao, L., et al. (2020). 3,4-seco-Dammarane Triterpenoid Saponins with Anti-inflammatory Activity Isolated from the Leaves of Cyclocarya Paliurus. J. Agric. Food Chem. 68 (7), 2041–2053. doi:10.1021/acs.jafc.9b06898
Marcarino, M. O., Cicetti, S., Zanardi, M. M., and Sarotti, A. M. (2021). A Critical Review on the Use of DP4+ in the Structural Elucidation of Natural Products: the Good, the Bad and the Ugly. A Practical Guide. Nat. Prod. Rep. 39 (1), 58–76. doi:10.1039/d1np00030f
Ni, Y., Wu, S., Wang, X., Zhu, G., Chen, X., Ding, Y., et al. (2018). Cucurbitacin I Induces Pro‐death Autophagy in A549 Cells via the ERK‐mTOR‐STAT3 Signaling Pathway. J. Cel. Biochem. 119 (7), 6104–6112. doi:10.1002/jcb.26808
Pettit, G. R., Inoue, M., Kamano, Y., Herald, D. L., Arm, C., Dufresne, C., et al. (1988). Antineoplastic Agents. 147. Isolation and Structure of the Powerful Cell Growth Inhibitor Cephalostatin 1. J. Am. Chem. Soc. 110 (6), 2006–2007. doi:10.1021/ja00214a078
Qing, Z. X., Zhao, H., Tang, Q., Mo, C. M., Huang, P., Cheng, P., et al. (2017). Systematic Identification of Flavonols, Flavonol Glycosides, Triterpene and Siraitic Acid Glycosides from Siraitia Grosvenorii Using High-Performance Liquid Chromatography/quadrupole-Time-Of-Flight Mass Spectrometry Combined with a Screening Strategy. J. Pharm. Biomed. Anal. 138, 240–248. doi:10.1016/j.jpba.2017.01.059
Seeman, J. I., Paine, J. B., Secor, H. V., Im, H. S., and Bernstein, E. R. (1992). Supersonic Jet Studies of Alkyl-Substituted Pyrazines and Pyridines. Minimum-Energy Conformations and Torsional Motion. J. Am. Chem. Soc. 114, 5269–5280. doi:10.1021/ja00039a044
Si, J. Y., Chen, D. H., Shen, l. G., and Tu, G. Z. (1999). Studies on the Chemical Constituents of Root of Luohanguo(Siraitia Grosvenori). Acta Pharm. Sin. 34, 918–920.
Sun, C., Zhang, M., Shan, X., Zhou, X., Yang, J., Wang, Y., et al. (2010). Inhibitory Effect of Cucurbitacin E on Pancreatic Cancer Cells Growth via STAT3 Signaling. J. Cancer Res. Clin. Oncol. 136 (4), 603–610. doi:10.1007/s00432-009-0698-x
Sun, Y., Gao, L.-l., Tang, M.-y., Feng, B.-m., Pei, Y.-h., and Yasukawa, K. (2018). Triterpenoids from Euphorbia Maculata and Their Anti-inflammatory Effects. Molecules 23 (9), 2112. doi:10.3390/molecules23092112
Tang, Y., Li, W., Cao, J., Li, W., and Zhao, Y. (2015). Bioassay-guided Isolation and Identification of Cytotoxic Compounds from Bolbostemma Paniculatum. J. Ethnopharmacology 169, 18–23. doi:10.1016/j.jep.2015.04.003
Ueno, M., Kariya, R., Sittithumcharee, G., and Okada, S. (2021). Cucurbitacin B Induces Apoptosis of Primary Effusion Lymphoma via Disruption of Cytoskeletal Organization. Phytomedicine 85, 153545. doi:10.1016/j.phymed.2021.153545
Urban, M., Sarek, J., Kvasnica, M., Tislerova, I., and Hajduch, M. (2007). Triterpenoid Pyrazines and Benzopyrazines with Cytotoxic Activity. J. Nat. Prod. 70 (4), 526–532. doi:10.1021/np060436d
Wakamatsu, J., Stark, T. D., and Hofmann, T. (2019). Antioxidative Maillard Reaction Products Generated in Processed Aged Garlic Extract. J. Agric. Food Chem. 67 (8), 2190–2200. doi:10.1021/acs.jafc.8b06907
Wang, P., Kong, F., Wei, J., Wang, Y., Wang, W., Hong, K., et al. (2014). Alkaloids from the Mangrove-Derived Actinomycete Jishengella Endophytica 161111. Mar. Drugs 12 (1), 477–490. doi:10.3390/md12010477
Wijeratne, E. M. K., Bashyal, B. P., Liu, M. X., Rocha, D. D., Gunaherath, G. M. K. B., U’Ren, J. M., et al. (2012). Geopyxins A-E, Ent-Kaurane Diterpenoids from Endolichenic Fungal Strains Geopyxis Aff. Majalis and Geopyxis Sp. AZ0066: Structure-Activity Relationships of Geopyxins and Their Analogues. J. Nat. Prod. 75 (3), 361–369. doi:10.1021/np200769q
Wu, P.-L., Lin, F.-W., Wu, T.-S., Kuoh, C.-S., Lee, K.-H., and Lee, S.-J. (2004). Cytotoxic and Anti-HIV Principles from the Rhizomes of Begonia Nantoensis. Chem. Pharm. Bull. 52 (3), 345–349. doi:10.1248/cpb.52.345
Zanardi, M. M., Biglione, F. A., Sortino, M. A., and Sarotti, A. M. (2018). General Quantum-Based NMR Method for the Assignment of Absolute Configuration by Single or Double Derivatization: Scope and Limitations. J. Org. Chem. 83 (19), 11839–11849. doi:10.1021/acs.joc.8b01749
Zeng, Y., Wang, J., Huang, Q., Ren, Y., Li, T., Zhang, X., et al. (2021). Cucurbitacin II a: A Review of Phytochemistry and Pharmacology. Phytotherapy Res. 35 (8), 4155–4170. doi:10.1002/ptr.7077
Keywords: Cucurbitaceae, Siraitia grosvenorii, cucurbitane-type triterpenoid, pyrazine, cytotoxicity
Citation: Wang H, Ma G, Wang H, Li L, Dong A, Liu H, Huo X, Si J and Wang J (2022) Novel Triterpenoid Alkaloids With Their Potential Cytotoxic Activity From the Roots of Siraitia grosvenorii. Front. Chem. 10:885487. doi: 10.3389/fchem.2022.885487
Received: 28 February 2022; Accepted: 04 April 2022;
Published: 29 April 2022.
Edited by:
Cheng-Peng Sun, Dalian Medical University, ChinaReviewed by:
Jiang-Jiang Tang, Northwest A&F University, ChinaFeng Qiu, Tianjin University of Traditional Chinese Medicine, China
Copyright © 2022 Wang, Ma, Wang, Li, Dong, Liu, Huo, Si and Wang. This is an open-access article distributed under the terms of the Creative Commons Attribution License (CC BY). The use, distribution or reproduction in other forums is permitted, provided the original author(s) and the copyright owner(s) are credited and that the original publication in this journal is cited, in accordance with accepted academic practice. No use, distribution or reproduction is permitted which does not comply with these terms.
*Correspondence: Jianyong Si, anlzaUBpbXBsYWQuYWMuY24=; Junchi Wang, amN3YW5nQGltcGxhZC5hYy5jbg==
†These authors have contributed equally to this work