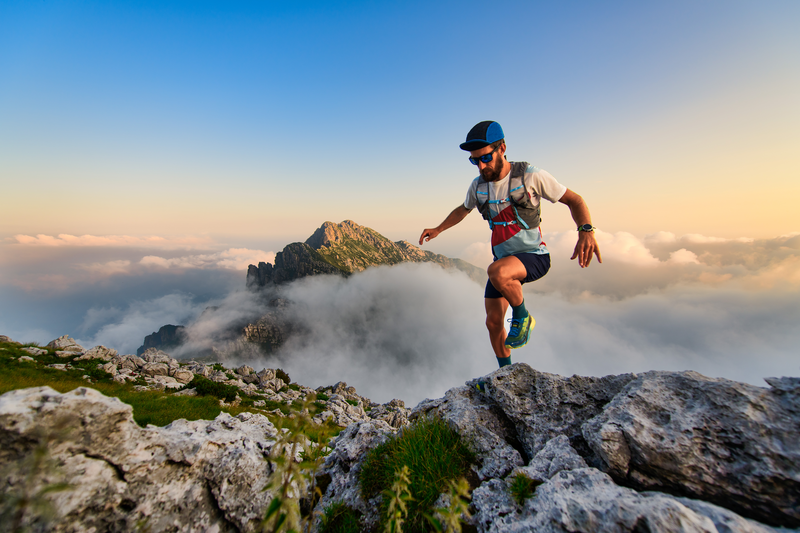
94% of researchers rate our articles as excellent or good
Learn more about the work of our research integrity team to safeguard the quality of each article we publish.
Find out more
MINI REVIEW article
Front. Chem. , 14 April 2022
Sec. Organic Chemistry
Volume 10 - 2022 | https://doi.org/10.3389/fchem.2022.883866
This article is part of the Research Topic Fluorine-18: Radiochemistry and Target-Specific PET Molecular Imaging Application View all 5 articles
Positron emission tomography (PET) is an important technique for the early diagnosis of disease. Due to the specific physical and chemical properties of Fluorine-18, this important isotope is widely used in PET for labelling and molecular imaging, and its introduction into medicine molecules could produce PET tracers. Developing with the development of organic synthetic methodologies, the introduction of Fluorine-18 into drug molecules efficiently and rapidly under mild conditions, and the formation of C-18F chemical bonds, has become one of the leading topics in both organic synthetic chemistry and radiochemistry. In this mini-review, we review a series of recent advances in the organic synthesis of C-18F bonds (2015–2021), including non-catalytic radiofluorinations via good leaving functional groups, transition metal-catalyzed radiofluorinations, and photo- or electro-catalytic synthetic radiofluorinations. As a result of the remarkable advancements in this field, organic synthetic methods for forming C-18F bonds are expected to continue growing.
Positron emission tomography (PET) is a non-invasive technology for radionuclide imaging, and it is sensitive to and informative of biological processes in vivo. A significant advantage of PET technology is that it is non-invasive, and that this powerful tool has been used not only for the diagnosis of various cancers (Phelps, 2000) but also the study of plant science (McKay et al., 1988; Kang et al., 2009; Niu et al., 2020), bacterial infections (Northrup et al., 2019; Welling et al., 2019) and even the subsurface microbial processes of soils (Kinsella et al., 2012).
PET applications in the previously mentioned areas require appropriate positron-emitting radioisotopes and appropriate compounds labeled with the radioisotopes. Internally used positron-emitting radioisotopes should have a long half-life (t1/2), low-energy positron emission, and flexible chemistry properties. Fluorine-18 [18F] is a positron-emitting radioisotope of fluorine, considered as an important source of positrons. The mass of 18F is 18.0009380 (6) u and its half-life is 109.771 (20) minutes. Furthermore, this radioisotope has low positron energy (0.64 MeV) and high efficiency by positron emission (97%) (Qaim, 2017). In medicinal chemistry, fluorine atoms are considered as bioisosteres of hydrogen atoms (Patani and LaVoie, 1996). In summary, all specific properties of Fluorine-18 previously mentioned fit perfectly for the requirements of its applications in PET (Qaim, 2017). Various [18F]-labelled compounds are investigated as PET tracers, including amino acids and their derivatives, peptides, saccharides, small medicinal molecules, and so on (shown in Figure 1). Introducing Fluorine-18 atoms into these molecules remains intractable and challenging, especially molecules with complex structures and various functional groups. Therefore, effective and convenient radiofluorination reactions for the synthesis of PET tracers are required. The reactions should be performed at a late stage of synthesis to reduce the unproductive radioactive decay before injections with radiochemical yields (RCYs) > 5% to support meaningful PET imaging (Lee et al., 2011). The radiotracers should be obtained in sterile solutions suitable for injection. [18F] is generated by a cyclotron in the form of [18F]fluorine gas ([18F]F2) via 18О(p,n)18F or 20Ne (d,α)18F reactions, or obtained from proton bombardment of 18O–enriched water in the form of [18F]fluoride via 18О(p,n)18F reactions. Recent research mainly focused on using [18F]fluorides as fluorine donors, which is more convenient to handle than extremely dangerous [18F]F2. Because the C-F bonds are restricted to enzyme catalysis in vivo, the formation of the C-F bond has been widely studied as a strategy for radiofluorination of PET tracers (Preshlock et al., 2016).
FIGURE 1. Some examples of [18F]-labelled PET tracers. RCC, radiochemical conversion; EDG, electron-donating group; DG, directing group.
In this mini-review, we summarize recent advances from 2015 to 2021 in synthetic methodologies to form C-[18F] bonds. The following sections will include three main topics: 1) radiofluorination of substrates with good leaving groups, 2) transition metals catalyzed radiofluorination, 3) Photo- and electro-catalytic methods for radiofluorination. Hopefully, this mini-review will shed light on new methods for developing radiofluorination methods in the future.
Although fluorination via the SNAr mechanism to form C-F bonds appears to be the easiest fluorination method in the absence of catalysts, Balz-Schiemann reactions (Nozaki and Tanaka, 1967) and Wallach reactions (Kovac et al., 2013) demonstrated low radiofluorination conversion factors (RCYs). Additionally, the requirement for the aromatic precursors in these reactions to have an electron-withdrawing group in the ortho or para position to the leaving group restricts the substrate scope, which means that the development of new precursors is a priority for non-catalytic radiofluorination. It was reported in 2012 that a new radiofluorination strategy to form C-F bonds using diphenyl group instead of dimethyl group (Maeda et al., 1987) was developed, which expanded the substrates scope and maintained high RCYs (Table 1, #1) (Mu et al., 2012). Applying the same strategy, Årstad et al. developed a practical method for aromatic radiofluorination of drug-like molecules, which showed high labelling efficiency (Table 1, #2) (Sander et al., 2015). Consequently, in 2018, they reported dibenzothiophene sulfonium salt as leaving groups under mild conditions (Table 1, #3) (Gendron et al., 2018). Besides sulfonium salts, Pike and colleagues discovered diaryl sulfoxides with electron-withdrawing groups could also serve as precursors and yield high RCYs (Table 1, #4) (Chun et al., 2013).
The radiofluorination reactions to other leaving groups have also been investigated. N-arylsydnones are important precursors that provide excellent RCYs, in which sydnone is an excellent activated group that could not stabilize an incoming negative charge through resonance delocalization. This was reported by Murphy’s group in 2017 (Table 1, #5) (Narayanam et al., 2017). DABCO was studied as another novel leaving group by Bernd Neumaier’s group in 2021 (Humpert et al., 2021). 5-iodo-2-[18F]fluoropyridine can be synthesized from 2-DABCO-5-iodopyridine precursor. This precursor can be prepared from commercially available 5-iodo-2-hydroxypyridine, according to the procedure reported by (Richard et al., 2020). The [18F]-labelled molecules were isolated by reversed-phase solid-phase extraction (RP SPE) strategy, with high RCYs of 77 ± 7% (n > 10) and radiochemical purity (>99%), and was further conjugated with thiol, using XantPhos Pd G3 as a catalyst with the RCYs varying from 10% to >90% (Table 1, #6). The author successfully applied the Pd-catalyzed S-arylation protocol to several short peptides under mild aqueous conditions with rapid kinetics. However, its unstable RCYs depending on the structures of peptides need to be overcome.
Meanwhile, diaryliodonium salts are a range of essential precursors in radiofluorinations reactions. In 1995, Pike and Aighirhio reported the first example of diaryliodonium salts precursors giving high RCYs, revealing that electron-deficient arenes were easier to be labelled (Table 1, #7) (Pike and Aigbirhio, 1995). In 2007, Coenen et al. developed a new precursor, aryl (2-thienyl) iodonium salts, and found this method could introduce [18F] to electron-rich arenes with high regiospecificity (Table 1, #8) (Ross et al., 2007). In 2010, Lee, Pike, and co-workers found that the reactions of [18F]fluoride ions with unsymmetrical diaryliodonium salts complied with the Curtin-Hammett principle (Chun et al., 2010). This mechanism was proved by crystal structures of diaryliodonium fluorides in 2017 (Lee et al., 2017).
Aryl iodonium ylides can also be used as precursors of radiofluorination reactions. In 2014, Ermert et al. applied aryl iodonium ylides with Meldrum’s acid auxiliaries in the radiofluorination of arenes (Cardinale et al., 2014). In the same year, Liang et al. designed spirocyclic iodonium ylides as precursors, and they proved that spirocyclic iodonium ylides were able to radiofluorinate non-activated and sterically hindered arenes and tolerate a range of functional groups with high RCYs and regioselectivity (Table 1, #9) (Rotstein et al., 2014; Liang et al., 2019). The same group found a better auxiliary SPIAd with superior precursor stability under radiofluorination conditions, and confirmed that iodonium (III) ylides performed better than diaryliodonium (III) salts by DFT calculations in 2016 (Rotstein et al., 2016). And in 2017, Riss et al. suggested the addition of PPh3 and N2 protection could further increase fluorination yields and reaction rates of the method (Jakobsson et al., 2017).
Since the -SCF3 functional group has a very high lipophilicity (π = 1.44), the introduction of Ar-SCF218F structure into medicinal molecules can dramatically affect their cell-membrane permeability (Xu et al., 2015). Therefore, the radiofluorination of this structure has been a research focus. Zheng et al. (2015) reported a new method to introduce the 18F-trifluoromethylthiol group in the presence of [18F]-fluoride, S8, and difluoromethylene phosphobetaine (PDFA), which resulted in formation of the 18F-labeled SCF3 anion in situ from difluorocarbene (Table 1, #10). (Carbonnel et al., 2017) used [18F]-fluoroform and disulfides to prepare 18F-labelled aryl-SCF3 with high RCYs, and they found that this method could be used for diphenyl diselenides (Table 1, #11).
Recent research has shown that sulfuryl fluorides can also be used for radiofluorination. In 2021, Zheng et al. (2021) reported the synthesis of aryl [18F]fluorosulfates via sulfur fluoride exchange (SuFEx) click reactions (Table 1, #12). This method show excellent RCYs and high molar activity under mild conditions only within 30 s.
Some different approaches based on the direct formation of the C-CF218F bond of arenes and heteroarenes under copper-mediated/catalyzed conditions have been reported by Gouverneur, Riss, Vugts, Jubault and Labar before 2015 (Huiban et al., 2013; Ivashkin et al., 2014; Ruhl et al., 2014). Copper catalysts have been widely used to mediate or catalyze aromatic fluorination reactions with aryl boronates, aryl boronic acids, (mesityl)-(aryl)iodonium salts, and arylstannanes since 2014 (Tredwell et al., 2014; Guibbal et al., 2020; Mossine et al., 2020). In 2015, Sanford and Scott’s group developed an inexpensive copper salt, Copper (II) trifluoromethanesulfonate (Cu(OTf)2), providing 8–73% RCCs in the radiofluorination of aryl, heteroaryl, and vinyl boronic acid precursors 1 for the first time (Mossine et al., 2015). This method presented good functional group tolerance and water tolerance (Figure 2, Reaction 1). Still utilizing Cu(OTf)2 as catalyst, Makaravage et al. (2016) developed a copper-mediated 18F- fluorination method of arylstannanes 2 with high specific activity. This method was the first practical nucleophilic 18F-fluorination to stannanes and was compatible with both electron-rich and electron-deficient arene substrates (Figure 2, Reaction 2). Notably, as the residual metal levels were below the allowed limits set by ICH guidelines and the protocol was readily automated, it showed a great potential in medicinal PET applications.
Mesityl-aryl iodonium salt was also applied as the precursors in the copper-mediated 18F- fluorination to produce 18F-arenes under mild conditions before 2015 (Ichiishi et al., 2014). In 2017, Sanford and co-workers took their work further (McCammant et al., 2017). Generated in situ from stable and easily available C−H starting materials, the unstable electron-rich diaryliodonium salts intermediates were obtained and subsequently converted to Ar-18F to perform C-H bond activation (Figure 2, Reaction 3). This method tolerates a wide range of electron-rich arenes. Yet, protic functional groups were not compatible and thus pre-protection is required. Notably, the lower selectivity of the method to heterocycles would probably limit its applications.
In 2020, the same group disclosed a new 18F- fluorination method of aryl halides with directing groups (DG) at the ortho position (Sharninghausen et al., 2020). The reaction utilized copper N-heterocyclic carbene complexes 3 as mediators. The N-heterocyclic carbene stabilized Cu(I)− 18F complexes, which could accelerate the key step of aryl-bromide bond activation (Figure 2, Reaction 4).
In 2016, by introducing copper catalyst, Zheng et al. (2017) found that ɑ-bromocarbonyl compounds 4 could also be converted to ɑ-[18F]SCF3 carbonyl derivatives (Figure 2, Reaction 5). This strategy broadened the substrate scope of their former investigations (Table 1, #10).
A series of radiofluorination reactions mediated by Ag had already been reported by Gouverneur’s group before 2015 (Teare et al., 2010; Stenhagen et al., 2013). In 2015, they used AgOTf and [18F]KF/K222 to radiofluorinate aryl -OCF3, -SCF3, and -OCHF2 structures 5 at room temperature to 60°C in 20 min (Figure 2, Reaction 6) (Khotavivattana et al., 2015). Additionally, the protocol accepts aryl cores containing alkyl, ethers, esters, aryl, halogens, and unprotected amines. According to the author, the order of reactivity towards 18F-fluoride is: ArOCHFCl > ArCF2Br ≈ ArCHFCl > ArSCF2Br > ArOCF2Br. Later, in 2019 Shen’s and Gouverneur’s groups extended the protocol to the synthesis of heteroarenes started from commercially available aryl-Bpin (boronic acid pinacol ester) substrates 6 (Figure 2, Reaction 7) (Wu et al., 2019).
Gouverneur’s group synthesized 18F-labeled aryl-CF3 and aryl-CHF2 structures from aryl-CF2CO2H and aryl-CHFCO2H with AgNO3 in 2013 (Mizuta et al., 2013). They described a protocol for obtaining 18F-labeled aryl-CF3 products 7 using AgOTf and [18F]KF/K222 in 2016 (Verhoog et al., 2016), in which AgOTf improved the RCCs of various substrates tremendously. This method has been extended to aryl-CHF2, for which no [18F] labelling methods are available. They succeeded in applying aryl-CHFCl 8 as precursors (Figure 2, Reaction 8) under mild conditions.
A nickel-mediated protocol for aryl-[18F] labeling was presented by Hooker’s and Ritter’s group in 2012 (Lee et al., 2012). They developed and applied their nickel-mediated protocol in 2016, compound 9 being utilized to produce [Ni]-Ar 10 in pyridine from aryl boronic acids and esters (Figure 3, Reaction 1). This method was successfully applied to the synthesis of [18F]5-fluorouracil, a PET tracer for clinical research in oncology for the first time. However, the isolated yield of the entire synthetic route needs to be improved (Hoover et al., 2016).
Ritter’s group investigated ruthenium complexes as transition metal catalysts for the radiofluorination of phenols 11 for the first time in 2017 (Figure 3, Reaction 2) (Beyzavi et al., 2017). Their work enriched the tools for 18F-labeling of electron-rich aryl systems. The method is suitable for heterocyclic compounds as well as a wide variety of functional groups. According to the report, several basic amines performed well, and the reaction show good tolerance to moisture and air. The authors suggested, however, that heterocycles might interfere with the formation of efficient ruthenium complexes, which might be improved through optimization of ligand structures.
MN3PU 12, which targets leucine repeat kinase 2 (LRRK2), is of great significance in the investigation of Parkinson’s disease. However, 12 is temperature-sensitive and thermally decomposes in polar, aprotic solvents (e.g., DMF and DMSO), thus causing undesirable byproducts under the general conditions of radiofluorination. Schaffer et al. (Malik et al., 2020) demonstrated that by using chromium (II) chloride and titanocene dichloride as Lewis acids, it was possible to achieve non-decay corrected radiochemical yields (ndc RCYs) for [18F]MN3PU up to 41 ± 1% and 37 ± 0.7%, respectively (Figure 3, Reaction 3).
Using TiO2 nanoparticles as the catalyst (Sergeev et al., 2015) radiofluorinated aromatic, aliphatic, and cycloaliphatic substrates with a maximum RCC in the presence of up to 25% water (Figure 3, Reaction 4). The tosyl-fallypride 13 was selected as the model compound and was reacted in a mixture of acetonitrile and thexyl alcohol at 110°C for 7 min to yield [18F]fallypride [18F]14, a highly specific radio-probe used in PET imaging of the brain. Interestingly, the authors noticed that some [18F]fluoride was trapped on the catalyst and could not be removed. Furthermore, they determined the optimal reaction conditions and investigated a range of substrates with high RCCs above 70%. Additionally, the group performed full production runs in order to illustrate the overall RCY of isolated [18F]Fallypride as an injectable product. Despite the hypothesised mechanism, more research on its mechanism and substrate scope is required.
The introduction of 18F into organic compounds via Photo- and Electro-catalytic methods under mind conditions is a very attractive topic (Bui and Kim, 2021; Hernández-Valdés and Sadeghi, 2021). Using cationic organic dye 15 as a photoredox catalyst, Nicewicz and colleagues developed a series of photoredox catalytic methods to form C-18F bonds. In 2019, they reported a direct arene C-H radiofluorination catalyzed by this cationic organic dye system under 450 nm laser (Chen et al., 2019) (Figure 4, Reaction 1). According to their work, due to steric effect, 18F-labeling of methoxy aryl substituents at the para position is preferred unless the para position is occupied by another functional group. In addition to direct arene C-H radiofluorination, nucleophilic aromatic substitution was investigated subsequently. They have also applied this catalyst to the aromatic halides and their derivatives, with leaving groups such as F, Cl, Br, I, OTf, NO2 (Chen et al., 2021) and OR (R = para-chlorophenyl) (Tay et al., 2020) (Figure 4, Reaction 2). This method did not perform well when X was the iodine substituent, and the RCYs of reactions varied from non-detected to 79.7%. For the radiofluorination on aliphatic derivatives, Sodium decatungstate (Na4W10O32) has been investigated as a photoredox catalyst. Britton and colleagues demonstrated that this catalyst can radiofluorinate the unprotected branched aliphatic amino acids at the branched positions under mild conditions. In their report, amino acids including leucine, homoleucine and β-amino-homoleucine displayed RCYs of 23.3 ± 3.3%, 27.9 ± 3.3% and 29.8 ± 0.7% repectively, while valine and isoleucine showed unsatisfactory RCYs (<6.4%) (Nodwell et al., 2017) (Figure 4, Reaction 3). Later, considering that peptides are often ideal ligands for diagnostic molecular imaging, the same group also utilized this method to synthesize the 18F-labelled peptides containing leucine residues, including [18F]FAfLGEA-NH2 (a ligand for cancer-specific receptor EGFRvIII) [18F]ZJ-43 (a NAAG peptidase inhibitor) and the analogue of [18F]ZJ-43 (Yuan et al., 2018) (Figure 4, Reaction 4). Their results indicated that their method had great potential in the synthesis of PET tracers and chemical biology. In 2020, Doyle and coworkers reported a photocatalytic nucleophilic radiofluorination of redox-active esters in presence of Ir(F-ppy)3 under the irradiation of a 34W blue LED (Webb et al., 2020) (Figure 4, Reaction 5). Their protocol provided a new strategy for the radiofluorination of bioactive molecules.
Research on 18F-labeling by electrosynthesis provides a new direction for investigations into radiofluorination (Waldmann et al., 2017; Hernández-Valdés and Sadeghi, 2021). Professor Sadeghi’s group studied the electrochemical fluorination of di-tert-butyl-(4-tert-butyl-1,2-phenylene)-dicarbonate (He et al., 2015) and methyl (phenylthio)acetate (Balandeh et al., 2017; Balandeh et al., 2018). These studies led to the invention of an automated apparatus for 18F-fluorination of organic molecules 16 (Figure 4, Reaction 6) by his group (Waldmann et al., 2017), reported to achieve the highest RCY of 17.9%. According to the report, the reaction voltage was set to 1.95V vs. a silver pseudo-reference electrode. One of the highlights of these reactions were their high radiochemical purities (>99%). However, the drawback of the apparatus is the high proportion of the starting activity that is unreacted (48%) and remaining in the system (38%), which needs further improvement.
Introducing C-18F bonds into organic compounds with high efficiency, rapidity, and simplicity under mild conditions has always been a topic of great interest in medicinal chemistry and organic synthesis chemistry, and this topic still presents various opportunities and challenges. When forming C-18F bonds for PET tracers, radiochemists must take functional group tolerance into account. The above summary shows that non-catalytic radiofluorination, transition metal catalysts, photo- and electrocatalytic strategies, and using good leaving functional groups have all been well developed in the last 5 years. In the meantime, late-stage radio-modification of drug-like molecules and PET tracers is still a great prospect, especially rapid and highly selective direct radiofluorination. Throughout the next five to 10 years, it will be exciting to see how this field develops to achieve direct and late-stage radiofluorination of drug-like molecules and PET tracking molecules with C-18F bonds.
ZL, YS, and TL organized and wrote the manuscript together. All authors approved this manuscript.
Funding provided by the National Natural Science Foundation of China (Grants No. 22171141, 22193010, and 22193014). Supports from State Key Laboratory of Elementoorganic Chemistry, College of Chemistry at Nankai University, the Haihe Laboratory of Sustainable Chemical Transformations at Nankai University and Key Laboratory of Organofluorine Chemistry at Shanghai Institute of Organic Chemistry, Chinese Academy of Sciences.
The authors declare that the research was conducted in the absence of any commercial or financial relationships that could be construed as a potential conflict of interest.
All claims expressed in this article are solely those of the authors and do not necessarily represent those of their affiliated organizations, or those of the publisher, the editors and the reviewers. Any product that may be evaluated in this article, or claim that may be made by its manufacturer, is not guaranteed or endorsed by the publisher.
Balandeh, M., Rios, A., Allison, N., Shirazi, D., Gomez, A., Rambaran, L., et al. (2018). Electrochemical Flash Fluorination and Radiofluorination. ChemElectroChem 5 (22), 3353–3356. doi:10.1002/celc.201800973
Balandeh, M., Waldmann, C., Shirazi, D., Gomez, A., Rios, A., Allison, N., et al. (2017). Electrochemical Fluorination and Radiofluorination of Methyl(phenylthio)acetate Using Tetrabutylammonium Fluoride (TBAF). J. Electrochem. Soc. 164 (9), G99–G103. doi:10.1149/2.0941709jes
Beyzavi, H., Mandal, D., Strebl, M. G., Neumann, C. N., D’Amato, E. M., Chen, J., et al. (2017). 18F-Deoxyfluorination of Phenols via Ru π-Complexes. ACS Cent. Sci. 3 (9), 944–948. doi:10.1021/acscentsci.7b00195
Bui, T. T., and Kim, H. K. (2021). Recent Advances in Photo‐mediated Radiofluorination. Chem. Asian J. 16 (16), 2155–2167. doi:10.1002/asia.202100399
Carbonnel, E., Besset, T., Poisson, T., Labar, D., Pannecoucke, X., and Jubault, P. (2017). 18F-Fluoroform: a 18F-Trifluoromethylating Agent for the Synthesis of SCF218F-Aromatic Derivatives. Chem. Commun. 53 (42), 5706–5709. doi:10.1039/c7cc02652h
Cardinale, J., Ermert, J., Humpert, S., and Coenen, H. H. (2014). Iodonium Ylides for One-step, No-Carrier-Added Radiofluorination of Electron Rich Arenes, Exemplified with 4-(([18F]fluorophenoxy)-Phenylmethyl)piperidine NET and SERT Ligands. RSC Adv. 4 (33), 17293–17299. doi:10.1039/c4ra00674g
Chen, W., Huang, Z., Tay, N. E. S., Giglio, B., Wang, M., Wang, H., et al. (2019). Direct Arene C-H Fluorination with 18 F − via Organic Photoredox Catalysis. Science 364 (6446), 1170–1174. doi:10.1126/science.aav7019
Chen, W., Wang, H., Tay, N. E. S., Pistritto, V. A., Li, K.-P., Zhang, T., et al. (2021). Arene Radiofluorination Enabled by Photoredox-Mediated Halide Interconversion. Nat. Chem. 14, 216–223. doi:10.1038/s41557-021-00835-7
Chun, J.-H., Lu, S., Lee, Y.-S., and Pike, V. W. (2010). Fast and High-Yield Microreactor Syntheses of Ortho-Substituted [18F]Fluoroarenes from Reactions of [18F]Fluoride Ion with Diaryliodonium Salts. J. Org. Chem. 75 (10), 3332–3338. doi:10.1021/jo100361d
Chun, J.-H., Morse, C. L., Chin, F. T., and Pike, V. W. (2013). No-carrier-added [18F]fluoroarenes from the Radiofluorination of Diaryl Sulfoxides. Chem. Commun. 49 (21), 2151–2153. doi:10.1039/c3cc37795d
Gendron, T., Sander, K., Cybulska, K., Benhamou, L., Sin, P. K. B., Khan, A., et al. (2018). Ring-Closing Synthesis of Dibenzothiophene Sulfonium Salts and Their Use as Leaving Groups for Aromatic 18F-Fluorination. J. Am. Chem. Soc. 140 (35), 11125–11132. doi:10.1021/jacs.8b06730
Gouverneur, V., Verhoog, S., Pfeifer, L., Khotavivattana, T., Calderwood, S., Collier, T., et al. (2016). Silver-Mediated 18F-Labeling of Aryl-CF3 and Aryl-CHF2 with 18F-Fluoride. Synlett 27 (01), 25–28. doi:10.1055/s-0035-1560592
Guibbal, F., Isenegger, P. G., Wilson, T. C., Pacelli, A., Mahaut, D., Sap, J. B. I., et al. (2020). Manual and Automated Cu-Mediated Radiosynthesis of the PARP Inhibitor [18F]olaparib. Nat. Protoc. 15 (4), 1525–1541. doi:10.1038/s41596-020-0295-7
He, Q., Alfeazi, I., and Sadeghi, S. (2015). No-carrier-added Electrochemical Nucleophilic Radiofluorination of Aromatics. J. Radioanal. Nucl. Chem. 303 (1), 1037–1040. doi:10.1007/s10967-014-3604-1
Hernández‐Valdés, D., and Sadeghi, S. (2021). Electrochemical Radiofluorination of Small Molecules: New Advances. Chem. Rec. 21 (9), 2397–2410. doi:10.1002/tcr.202100086
Hoover, A. J., Lazari, M., Ren, H., Narayanam, M. K., Murphy, J. M., Van Dam, R. M., et al. (2016). A Transmetalation Reaction Enables the Synthesis of [18F]5-Fluorouracil from [18F]Fluoride for Human PET Imaging. Organometallics 35 (7), 1008–1014. doi:10.1021/acs.organomet.6b00059
Huiban, M., Tredwell, M., Mizuta, S., Wan, Z., Zhang, X., Collier, T. L., et al. (2013). A Broadly Applicable [18F]trifluoromethylation of Aryl and Heteroaryl Iodides for PET Imaging. Nat. Chem 5 (11), 941–944. doi:10.1038/nchem.1756
Humpert, S., Omrane, M. A., Urusova, E. A., Gremer, L., Willbold, D., Endepols, H., et al. (2021). Rapid 18F-Labeling via Pd-Catalyzed S-Arylation in Aqueous Medium. Chem. Commun. 57 (29), 3547–3550. doi:10.1039/D1CC00745A
Ichiishi, N., Brooks, A. F., Topczewski, J. J., Rodnick, M. E., Sanford, M. S., and Scott, P. J. H. (2014). Copper-catalyzed [18F]fluorination of (Mesityl)(aryl)iodonium Salts. Org. Lett. 16 (12), 3224–3227. doi:10.1021/ol501243g
Ivashkin, P., Lemonnier, G., Cousin, J., Grégoire, V., Labar, D., Jubault, P., et al. (2014). [18F]CuCF3: A [18F]Trifluoromethylating Agent for Arylboronic Acids and Aryl Iodides. Chem. Eur. J. 20 (31), 9514–9518. doi:10.1002/chem.201403630
Jakobsson, J. E., Grønnevik, G., and Riss, P. J. (2017). Organocatalyst-assisted Ar-18F Bond Formation: a Universal Procedure for Direct Aromatic Radiofluorination. Chem. Commun. 53 (96), 12906–12909. doi:10.1039/c7cc07211b
Kang, D.-J., Nakanishi, T. M., Kume, T., and Ishii, R. (2009). Determination of the Rate of 18F-Labeled Water Movement to the Leaf and its Association with Water Relations in Acid Soil-Tolerant rice Varieties. J. Crop Sci. Biotechnol. 12 (4), 261–265. doi:10.1007/s12892-009-0122-8
Khotavivattana, T., Verhoog, S., Tredwell, M., Pfeifer, L., Calderwood, S., Wheelhouse, K., et al. (2015). 18 F‐Labeling of Aryl‐SCF 3 , ‐OCF 3 and ‐OCHF 2 with [ 18 F]Fluoride. Angew. Chem. Int. Ed. 54 (34), 9991–9995. doi:10.1002/anie.201504665
Kinsella, K., Schlyer, D. J., Fowler, J. S., Martinez, R. J., and Sobecky, P. A. (2012). Evaluation of Positron Emission Tomography as a Method to Visualize Subsurface Microbial Processes. J. Hazard. Mater. 213-214, 498–501. doi:10.1016/j.jhazmat.2012.01.037
Kovac, M., Mavel, S., and Anderluh, M. (2013). 18F-Labeled Aryl-Tracers through Direct Introduction of [18F]fluoride into Electron-Rich Arenes. Coc 17, 2921–2935. doi:10.2174/13852728113179990022
Lee, E., Hooker, J. M., and Ritter, T. (2012). Nickel-Mediated Oxidative Fluorination for PET with Aqueous [18F] Fluoride. J. Am. Chem. Soc. 134 (42), 17456–17458. doi:10.1021/ja3084797
Lee, E., Kamlet, A. S., Powers, D. C., Neumann, C. N., Boursalian, G. B., Furuya, T., et al. (2011). A Fluoride-Derived Electrophilic Late-Stage Fluorination Reagent for PET Imaging. Science 334 (6056), 639–642. doi:10.1126/science.1212625
Lee, Y.-S., Chun, J.-H., Hodošček, M., and Pike, V. W. (2017). Crystal Structures of Diaryliodonium Fluorides and Their Implications for Fluorination Mechanisms. Chem. Eur. J. 23 (18), 4353–4363. doi:10.1002/chem.201604803
Liang, S. H., Wang, L., Stephenson, N. A., Rotstein, B. H., and Vasdev, N. (2019). Facile 18F Labeling of Non-activated Arenes via a Spirocyclic Iodonium(III) Ylide Method and its Application in the Synthesis of the mGluR5 PET Radiopharmaceutical [18F]FPEB. Nat. Protoc. 14 (5), 1530–1545. doi:10.1038/s41596-019-0149-3
Maeda, M., Fukumura, T., and Kojima, M. (1987). The Dimethylsulfonium Moiety as a Leaving Group in Aromatic Radiofluorination Using Tetra-N-Butylammonium [18F]fluoride. International Journal of Radiation Applications and Instrumentation. Part A. Appl. Radiat. Isot. 38 (4), 307–310. doi:10.1016/0883-2889(87)90046-3
Makaravage, K. J., Brooks, A. F., Mossine, A. V., Sanford, M. S., and Scott, P. J. H. (2016). Copper-Mediated Radiofluorination of Arylstannanes with [18F]KF. Org. Lett. 18 (20), 5440–5443. doi:10.1021/acs.orglett.6b02911
Malik, N., Bendre, S., Schirrmacher, R., and Schaffer, P. (2020). Lewis Acid-Facilitated Radiofluorination of MN3PU: A LRRK2 Radiotracer. Molecules 25 (20), 4710. doi:10.3390/molecules25204710
McCammant, M. S., Thompson, S., Brooks, A. F., Krska, S. W., Scott, P. J. H., and Sanford, M. S. (2017). Cu-Mediated C-H 18F-Fluorination of Electron-Rich (Hetero)arenes. Org. Lett. 19 (14), 3939–3942. doi:10.1021/acs.orglett.7b01902
McKay, R. M. L., Palmer, G. R., Ma, X. P., Layzell, D. B., and McKee, B. T. A. (1988). The Use of Positron Emission Tomography for Studies of Long-Distance Transport in Plants: Uptake and Transport of 18F. Plant Cel Environ. 11 (9), 851–861. doi:10.1111/j.1365-3040.1988.tb01911.x
Mizuta, S., Stenhagen, I. S. R., O’Duill, M., Wolstenhulme, J., Kirjavainen, A. K., Forsback, S. J., et al. (2013). Catalytic Decarboxylative Fluorination for the Synthesis of Tri- and Difluoromethyl Arenes. Org. Lett. 15 (11), 2648–2651. doi:10.1021/ol4009377
Mossine, A. V., Brooks, A. F., Makaravage, K. J., Miller, J. M., Ichiishi, N., Sanford, M. S., et al. (2015). Synthesis of [18F]Arenes via the Copper-Mediated [18F]Fluorination of Boronic Acids. Org. Lett. 17 (23), 5780–5783. doi:10.1021/acs.orglett.5b02875
Mossine, A. V., Tanzey, S. S., Brooks, A. F., Makaravage, K. J., Ichiishi, N., Miller, J. M., et al. (2020). Synthesis of High-Molar-Activity [18F]6-Fluoro-L-DOPA Suitable for Human Use via Cu-Mediated Fluorination of a BPin Precursor. Nat. Protoc. 15 (5), 1742–1759. doi:10.1038/s41596-020-0305-9
Mu, L., Fischer, C. R., Holland, J. P., Becaud, J., Schubiger, P. A., Schibli, R., et al. (2012). 18F-Radiolabeling of Aromatic Compounds Using Triarylsulfonium Salts. Eur. J. Org. Chem. 2012 (5), 889–892. doi:10.1002/ejoc.201101730
Narayanam, M. K., Ma, G., Champagne, P. A., Houk, K. N., and Murphy, J. M. (2017). Synthesis of [18F]Fluoroarenes by Nucleophilic Radiofluorination ofN-Arylsydnones. Angew. Chem. Int. Ed. 56 (42), 13006–13010. doi:10.1002/anie.201707274
Niu, H. l., Peng, C. y., Zhu, X. d., Dong, Y. y., Li, Y. y., Tang, L. l., et al. (2020). Positron‐emitting Tracer Imaging of Fluoride Transport and Distribution in tea Plant. J. Sci. Food Agric. 100 (8), 3554–3559. doi:10.1002/jsfa.10367
Nodwell, M. B., Yang, H., Čolović, M., Yuan, Z., Merkens, H., Martin, R. E., et al. (2017). 18F-Fluorination of Unactivated C-H Bonds in Branched Aliphatic Amino Acids: Direct Synthesis of Oncological Positron Emission Tomography Imaging Agents. J. Am. Chem. Soc. 139 (10), 3595–3598. doi:10.1021/jacs.6b11533
Northrup, J. D., Mach, R. H., and Sellmyer, M. A. (2019). Radiochemical Approaches to Imaging Bacterial Infections: Intracellular versus Extracellular Targets. Ijms 20 (22), 5808. doi:10.3390/ijms20225808
Nozaki, T., and Tanaka, Y. (1967). The Preparation of F18-Labelled Aryl Fluorides. The International Journal of Appl. Radiat. Isot. 18 (2), 111–119. doi:10.1016/0020-708X(67)90040-3
Patani, G. A., and LaVoie, E. J. (1996). Bioisosterism: A Rational Approach in Drug Design. Chem. Rev. 96 (8), 3147–3176. doi:10.1021/cr950066q
Phelps, M. E. (2000). Positron Emission Tomography Provides Molecular Imaging of Biological Processes. Proc. Natl. Acad. Sci. U.S.A. 97 (16), 9226–9233. doi:10.1073/pnas.97.16.9226
Pike, V. W., and Aigbirhio, F. I. (1995). Reactions of Cyclotron-Produced [18F]fluoride with Diaryliodonium Salts-A Novel Single-step Route to No-Carrier-Added [18]fluoroarenes. J. Chem. Soc. Chem. Commun. (21), 2215–2216. doi:10.1039/C39950002215
Preshlock, S., Tredwell, M., and Gouverneur, V. (2016). 18F-Labeling of Arenes and Heteroarenes for Applications in Positron Emission Tomography. Chem. Rev. 116 (2), 719–766. doi:10.1021/acs.chemrev.5b00493
Qaim, S. M. (2017). Nuclear Data for Production and Medical Application of Radionuclides: Present Status and Future Needs. Nucl. Med. Biol. 44, 31–49. doi:10.1016/j.nucmedbio.2016.08.016
Richard, M., Specklin, S., Roche, M., Hinnen, F., and Kuhnast, B. (2020). Original Synthesis of Radiolabeling Precursors for Batch and on Resin One-Step/late-Stage Radiofluorination of Peptides. Chem. Commun. 56 (16), 2507–2510. doi:10.1039/C9CC09434B
Ross, T. L., Ermert, J., Hocke, C., and Coenen, H. H. (2007). Nucleophilic 18F-Fluorination of Heteroaromatic Iodonium Salts with No-Carrier-Added [18F]Fluoride. J. Am. Chem. Soc. 129 (25), 8018–8025. doi:10.1021/ja066850h
Rotstein, B. H., Stephenson, N. A., Vasdev, N., and Liang, S. H. (2014). Spirocyclic Hypervalent Iodine(III)-mediated Radiofluorination of Non-activated and Hindered Aromatics. Nat. Commun. 5, 4365. doi:10.1038/ncomms5365
Rotstein, B. H., Wang, L., Liu, R. Y., Patteson, J., Kwan, E. E., Vasdev, N., et al. (2016). Mechanistic Studies and Radiofluorination of Structurally Diverse Pharmaceuticals with Spirocyclic Iodonium(III) Ylides. Chem. Sci. 7 (7), 4407–4417. doi:10.1039/C6SC00197A
Rühl, T., Rafique, W., Lien, V. T., and Riss, P. J. (2014). Cu(i)-mediated 18F-Trifluoromethylation of Arenes: Rapid Synthesis of 18F-Labeled Trifluoromethyl Arenes. Chem. Commun. 50 (45), 6056–6059. doi:10.1039/c4cc01641f
Sander, K., Gendron, T., Yiannaki, E., Cybulska, K., Kalber, T. L., Lythgoe, M. F., et al. (2015). Sulfonium Salts as Leaving Groups for Aromatic Labelling of Drug-like Small Molecules with Fluorine-18. Sci. Rep. 5, 9941. doi:10.1038/srep09941
Sergeev, M. E., Morgia, F., Lazari, M., Wang, C., and van Dam, R. M. (2015). Titania-Catalyzed Radiofluorination of Tosylated Precursors in Highly Aqueous Medium. J. Am. Chem. Soc. 137 (17), 5686–5694. doi:10.1021/jacs.5b02659
Sharninghausen, L. S., Brooks, A. F., Winton, W. P., Makaravage, K. J., Scott, P. J. H., and Sanford, M. S. (2020). NHC-copper Mediated Ligand-Directed Radiofluorination of Aryl Halides. J. Am. Chem. Soc. 142 (16), 7362–7367. doi:10.1021/jacs.0c02637
Stenhagen, I. S. R., Kirjavainen, A. K., Forsback, S. J., Jørgensen, C. G., Robins, E. G., Luthra, S. K., et al. (2013). [18F]Fluorination of an Arylboronic Ester Using [18F]selectfluor Bis(triflate): Application to 6-[18F]fluoro-L-DOPA. Chem. Commun. 49 (14), 1386–1388. doi:10.1039/C2CC38646A
Tay, N. E. S., Chen, W., Levens, A., Pistritto, V. A., Huang, Z., Wu, Z., et al. (2020). 19F- and 18F-Arene Deoxyfluorination via Organic Photoredox-Catalysed Polarity-Reversed Nucleophilic Aromatic Substitution. Nat. Catal. 3 (9), 734–742. doi:10.1038/s41929-020-0495-0
Teare, H., Robins, E. G., Kirjavainen, A., Forsback, S., Sandford, G., Solin, O., et al. (2010). Radiosynthesis and Evaluation of [18F]Selectfluor Bis(triflate). Angew. Chem. Int. Edition 49 (38), 6821–6824. doi:10.1002/anie.201002310
Tredwell, M., Preshlock, S. M., Taylor, N. J., Gruber, S., Huiban, M., Passchier, J., et al. (2014). A General Copper-Mediated Nucleophilic18F Fluorination of Arenes. Angew. Chem. Int. Ed. 53 (30), 7751–7755. doi:10.1002/anie.201404436
Waldmann, C. M., Lebedev, A., Allison, N., and Sadeghi, S. (2017). An Automated Synthesizer for Electrochemical 18F-Fluorination of Organic Compounds. Appl. Radiat. Isot. 127, 245–252. doi:10.1016/j.apradiso.2017.06.028
Webb, E. W., Park, J. B., Cole, E. L., Donnelly, D. J., Bonacorsi, S. J., Ewing, W. R., et al. (2020). Nucleophilic (Radio)Fluorination of Redox-Active Esters via Radical-Polar Crossover Enabled by Photoredox Catalysis. J. Am. Chem. Soc. 142 (20), 9493–9500. doi:10.1021/jacs.0c03125
Welling, M. M., Hensbergen, A. W., Bunschoten, A., Velders, A. H., Roestenberg, M., and van Leeuwen, F. W. B. (2019). An Update on Radiotracer Development for Molecular Imaging of Bacterial Infections. Clin. Transl Imaging 7 (2), 105–124. doi:10.1007/s40336-019-00317-4
Wu, J., Zhao, Q., Wilson, T. C., Verhoog, S., Lu, L., Gouverneur, V., et al. (2019). Synthesis and Reactivity of α‐Cumyl Bromodifluoromethanesulfenate: Application to the Radiosynthesis of [ 18 F]ArylSCF 3. Angew. Chem. Int. Ed. 58 (8), 2413–2417. doi:10.1002/anie.201813708
Xu, X.-H., Matsuzaki, K., and Shibata, N. (2015). Synthetic Methods for Compounds Having CF3-S Units on Carbon by Trifluoromethylation, Trifluoromethylthiolation, Triflylation, and Related Reactions. Chem. Rev. 115 (2), 731–764. doi:10.1021/cr500193b
Yuan, Z., Nodwell, M. B., Yang, H., Malik, N., Merkens, H., Bénard, F., et al. (2018). Site-Selective, Late-Stage C−H 18 F-Fluorination on Unprotected Peptides for Positron Emission Tomography Imaging. Angew. Chem. Int. Ed. 57 (39), 12733–12736. doi:10.1002/anie.201806966
Zheng, J., Cheng, R., Lin, J.-H., Yu, D.-H., Ma, L., Jia, L., et al. (2017). An Unconventional Mechanistic Insight into SCF3Formation from Difluorocarbene: Preparation of18F-Labeled α-SCF3Carbonyl Compounds. Angew. Chem. Int. Ed. 56 (12), 3196–3200. doi:10.1002/anie.201611761
Zheng, J., Wang, L., Lin, J. H., Xiao, J. C., and Liang, S. H. (2015). Difluorocarbene‐Derived Trifluoromethylthiolation and [ 18 F]Trifluoromethylthiolation of Aliphatic Electrophiles. Angew. Chem. Int. Ed. 54 (45), 13236–13240. doi:10.1002/anie.201505446
Keywords: radiofluorination, organic synthetic methodologies, leaving functional groups, transition metal catalysis, photocatalysis, electrocatalysis
Citation: Liu Z, Sun Y and Liu T (2022) Recent Advances in Synthetic Methodologies to Form C-18F Bonds. Front. Chem. 10:883866. doi: 10.3389/fchem.2022.883866
Received: 25 February 2022; Accepted: 14 March 2022;
Published: 14 April 2022.
Edited by:
Zonghua Luo, ShanghaiTech University, ChinaReviewed by:
Adam Rosenberg, Vanderbilt University, United StatesCopyright © 2022 Liu, Sun and Liu. This is an open-access article distributed under the terms of the Creative Commons Attribution License (CC BY). The use, distribution or reproduction in other forums is permitted, provided the original author(s) and the copyright owner(s) are credited and that the original publication in this journal is cited, in accordance with accepted academic practice. No use, distribution or reproduction is permitted which does not comply with these terms.
*Correspondence: Tianfei Liu, dGlhbmZlaWxpdUBuYW5rYWkuZWR1LmNu
Disclaimer: All claims expressed in this article are solely those of the authors and do not necessarily represent those of their affiliated organizations, or those of the publisher, the editors and the reviewers. Any product that may be evaluated in this article or claim that may be made by its manufacturer is not guaranteed or endorsed by the publisher.
Research integrity at Frontiers
Learn more about the work of our research integrity team to safeguard the quality of each article we publish.