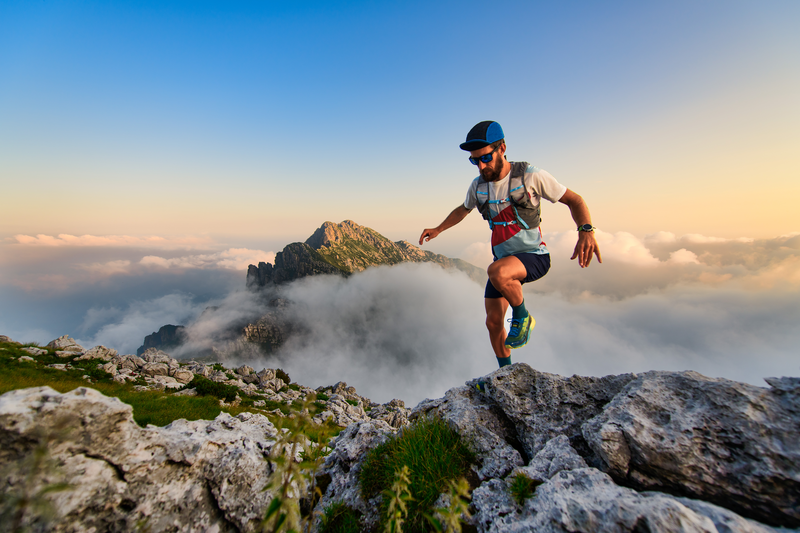
95% of researchers rate our articles as excellent or good
Learn more about the work of our research integrity team to safeguard the quality of each article we publish.
Find out more
REVIEW article
Front. Chem. , 17 March 2022
Sec. Organic Chemistry
Volume 10 - 2022 | https://doi.org/10.3389/fchem.2022.871684
The structural units of amino-/cyano-substituted furazans and furoxans played significant roles in the synthesis of nitrogen-rich energetic compounds. This account focused on the synthetic strategies toward nitrogen-rich energetic compounds through the transformations based on cyanofurazan/furoxan structures, including 3-amino-4-cyanofurazan, 4-amino-3-cyano furoxan, 3,4-dicyanofurazan, and 3,4-dicyanofuroxan. The synthetic strategies toward seven kinds of nitrogen-rich energetic compounds, such as azo (azoxy)-bridged, ether-bridged, methylene-bridged, hybrid furazan/furoxan-tetrazole–based, tandem furoxan–based, hybrid furazan-isofurazan–based, hybrid furoxan-isoxazole–based and fused framework–based energetic compounds were fully reviewed, with the corresponding reaction mechanisms toward the nitrogen-rich aromatic frameworks and examples of using the frameworks to create high energetic substances highlighted and discussed. The energetic properties of typical nitrogen-rich energetic compounds had also been compared and summarized.
Nitrogen-rich aromatic structures are the most essential frameworks for the constructions of nitrogen-rich energetic compounds, which constitute the core component shared by some most powerful explosives and propellants (Singh et al., 2007; Viswanath et al., 2018; Gao et al., 2020; Zhou et al., 2021). Unlike traditional energetic compounds, nitrogen-rich energetic compounds generate environmentally friendly molecular nitrogen as the major end-product of propulsion or explosion and have been the focus of research into energetic materials worldwide (Yin and Shreeve, 2017; Klapötke, 2021). During the past decades, heterocyclic five-membered rings of furazan, furoxan, isofurazan (1,2,4-oxadiazole and 1,3,4-oxadiazole), tetrazole, isoxazole, and their fused derivatives (such as pyridofurazan, triazolofurazan, pyridazinofuroxan etc.) are the most popular choice in the creation of new nitrogen-rich energetic compounds (Tang et al., 2016a; Zhang et al., 2017; Huang et al., 2020; Voronin et al., 2020; Yan et al., 2020; Dalinger et al., 2021; Larin et al., 2021). From the structural point of view, the frameworks based on the combinations of these heterocyclic five-membered rings, especially the ones with furazan, furoxan, tetrazole, or isofurazan units, can achieve more compact structures and higher enthalpy of positive formations, leading to corresponding nitrogen-rich energetic compounds with excellent detonation performances (Jaidann et al., 2010; Swain et al., 2010; Suntsova and Dorofeeva, 2017).
The synthetic pathways to frameworks, or more often their functionalized derivatives, suitable for the various required nitrogen-rich energetic compounds usually depend on key intermediates with functional groups that enable diverse and desired transformations (Agrawal and Hodgson, 2007). Compared with other nitrogen-rich heterocycles, most furazan- and furoxan-based energetic heterocycles demonstrate superior energetic properties and better modifiability, making them as a most successful class of structures for the design and synthesis of energetic materials. From a practical standpoint, cyanofurazan/furoxan has been regarded as one of the most important energetic intermediates for the synthesis of nitrogen-rich energetic compounds comprising furazan and furoxan structures, and these key cyanofurazan/furoxan structures mainly include 3-amino-4-cyanofurazan, 4-amino-3-cyanofuroxan, 3,4-dicyanofurazan, and 3,4-dicyanofuroxan. From a synthetic standpoint, cyano groups in cyanofurazan/furoxan structures enjoy a high degree of transformational diversity and can be further transformed into other heterocyclic five-membered rings such as furoxan, isofurazan, tetrazole, and isoxazole, leading to a series of linear or fused frameworks of nitrogen-rich energetic compounds (Qu et al., 2016; Pagoria et al., 2017; Wu et al., 2017; Zhai et al., 2019a; Johnson et al., 2020). The cyano groups could also be easily turned to highly powerful explosophoric groups of dinitromethyl, trinitromethyl, and fluorodinitromethyl groups (Gu et al., 2018). Moreover, the additional amino groups in cyanofurazan/furoxan structures provide perfect precursors for the synthesis of azo/azoxy moieties (Luo et al., 2010; Li et al., 2014).
The first part of the account focuses on the synthetic methods of 3-amino-4-cyanofurazan, 4-amino-3-cyanofuroxan, 3,4-dicyanofurazan, and 3,4-dicyanofuroxan and the abovementioned key intermediate structures and building blocks. The detailed reaction mechanisms for their preparations were also discussed in this part. It then outlines the recent successful manipulation of these intermediate structures/building blocks for the design and synthesis of corresponding nitrogen-rich energetic compounds. Based on the active cyano and amino groups, the introductions of azo/azoxy moieties, the formations of fused energetic heterocycles, the constructions of liner structures coupled via C–C or C–O bonds by bringing in additional heterocycles (including tetrazole, furazan, furoxan, isoxazole, and isofurazan), and the physicochemical properties and detonation performances of typical energetic compounds were fully reviewed (Figure 1). New structural design concepts and promising synthetic strategies related were also discussed.
The synthetic intermediates, including 3-amino-4-cyanofurazan, 4-amino-3-cyanofuroxan, 3,4-dicyanofurazan, and 3,4-dicyanofuroxan, are the basis and key building blocks for the synthetic work toward numerous nitrogen-rich energetic compounds. In general, the synthesis of these cyano-/amino-substituted furazans and furoxans involves the preparations of specific cyclized precursors and complex functional group transformation mechanism, which also provides important references for the design and synthesis of other cyano-/amino-substituted heterocycles.
Based on malononitrile, an active methylene compound as the starting material, the core furazan framework was formed through nitrosation and oximation reactions followed by dehydration and cyclization. The treatment of the hydroxylamine moiety with lead dioxide in acetic acid solution led to the formation of 3-amino-4-cyanofurazan 1 with a yield of 71% as the major product (Fan et al., 2008; Pagoria et al., 2017). The heavy metal reagents participate in the final elimination process and generate intensive heavy metal residues in the wastewater, causing serious pollution. To avoid the use of heavy metal reagents, an alternative synthetic method of 3-amino-4-cyanofurazan was developed based on 4-aminofurazan-3-formamide 7, which was obtained from N,N-dimethylformamide, a chlorination reagent, and an organic base. 3-amino-4-cyanofurazan was then obtained through a dehydration process (Fershtat et al., 2015). This method was much more environment-friendly and, therefore, more practical for scalable synthesis, providing fast access to large quantities of the target material for industrial production. Similar to the synthetic methods of 3-amino-4-cyanofuroxan, the synthesis of 4-amino-3-cyanofuroxan could also be achieved from malononitrile, but the synthesis pathway is more straightforward. After similar nitrosation and oximation reactions, the treatment of the dioxime moiety with lead dioxide gave the desired 4-amino-3-cyanofuroxan in 42% overall yields. (Luo et al., 2010). The alternative “green” synthesis of 3-amino-4-cyanofuroxan was also developed by dehydration of 4-aminofuroxan-3-formamide 9 under the medium of (CF3CO)2O/Py with a high reaction yield of 84% (Fershtat et al., 2015). More recently, another green and mild access to 3-amino-4-cyanofuroxan was further achieved through the oxidation of 8 by (diacetoxyiodo)benzene (PIDA) (Zhang et al., 2022). (Scheme 1A)
SCHEME 1. (A) Synthesis of 3-amino-4-cyanofurazan and 4-amino-3-cyanofuroxan; (B) Synthesis of 3,4-dicyanofurazan and 3,4-dicyanofuroxan.
Compared with 3-amino-4-cyano–substituted furazan and furoxan, 3,4-dicyano–substituted furazan and furoxan are better substrates for the design and synthesis of symmetrical energetic structures. 3,4-dicyanofurazan was prepared based on furazan-3,4-dicarboxylic acid, a commercially available chemical. The esterification and amination of the carboxyl groups led to furazan-3,4-dicarboxamide 12, and 3,4-dicyanofurazan 3 was obtained under the treatment of trifluoroacetic anhydride (TFAA) as a dehydrating agent with a yield approximated to 100% (Beyerle, 1983; Godovikova et al., 2009; Guo et al., 2018). In contrast, the preparation of 3,4-dicyanofuroxan was based on the commercially available chemical of dichloroglyoxime. The cyanation followed by a cyclization process yielded 3,4-dicyanofuroxan 4 in moderate yield (Grundmann et al., 1975). 3,4-dicyanofuroxan 4 could also be prepared through the nitration of cyanoacetic acid in trifluoroacetic acid medium, but with a poor yield (Parker et al., 1962). By using the dichloromethane solvent instead of trifluoroacetic acid (TFA), a much higher yield was achieved (72%), and the operational safety was also improved (Johnson et al., 2019). (Scheme 1B)
Azo and azoxy groups, which require the formation of two covalent bonds, are a bridge between two identical or different frameworks. Because of the inherent greater endothermicity of the N=N bond, the constructions of azo and azoxy groups are very useful for creating energetic compounds with a high enthalpy of formation and contribute markedly to the overall energetic performance. Compared with monocyclic compounds, azo-bridged bicyclic compounds had more reaction activity sites and could be modified by more substituent groups so as to enrich the diversity of energetic materials (Qu and Babailov, 2018; Kozak et al., 2008; Türker., 2016; Chavez et al., 2000; Liu et al., 2016; Sheremetev et al., 1998). Based on the reaction characteristics of the amino group in 3-amino-4-cyanofurazan 1, the corresponding azo compound 3,3'- dicyano-4,4'-azofurazan 16 (ρ: 1.62 g cm−3, Td: 234°C, D: 7,640 m s−1, P: 21.8 GPa) was obtained by the oxidation of potassium permanganate in acidic medium (Fan et al., 2008). Due to the high reaction activity of the cyano group, energetic groups such as geminal-dinitro (-CH(NO2)2) and triazole ring could be introduced to significantly improve the properties of 16 derivatives. Using 16 as the starting material, a three-dimensional energetic metal organic skeleton potassium 4,4'-bis (dinitromethyl)-3,3'-azofurazanate 19 was obtained by Tang et al. through addition, diazotization, nitration, and reduction reactions (Tang et al., 2016b). It was a promising green explosive with good thermal stability and detonation performances (Td: 229°C, D: 8,138 m s−1, P: 30.1 GPa), an impact sensitivity of 2 J, and a friction sensitivity of 20 N to external stimuli. In addition, triazole energetic compound 21 was synthesized by Qu et al. from 16 through hydrazine addition and cyclization (Qu et al., 2016). (Scheme 2A) The cyclization mechanism is also shown in Scheme 2A: Under alkaline conditions, HBr was eliminated because BrCN attacked the N atom on the hydrazino group; then the lone pair electrons of nitrogen on the amino group attacked the C atom on the cyano group (-CN) to form a C–N bond, and then through proton transfer, a triazole ring structure could be formed. Compared with 16, the thermal stability and detonation performances of 21 were improved to a certain extent (Td: 309°C, D: 8,458 m s−1, P: 26.2 GPa). In order to further improve the detonation performance of energetic compounds, the method of H2O2/H2SO4 oxidation or NaNO2/H2SO4 diazotization–substitution (Zhang et al., 2020) could be used to convert amino groups (-NH2) into nitro groups (-NO2).
SCHEME 2. (A) Synthesis of 19 and 21 and the mechanism of cyclization process via hydrazide and BrCN; (B) Synthesis of 23 and 26 by oxidative coupling.
Based on the oxidative couplings between primary amines and nitroso compounds, a series of azoxy energetic compounds were synthetized by Parakhin et al. using 4-amino-3-cyanofuroxan 2 as starting materials (Parakhin et al., 2017). The azoxy intermediate 22 obtained by reacting with 1,1-dinitro-1-nitroethane further reacted with TMSN3 by [3 + 2] cycloaddition, and tetrazolyl was successfully introduced to obtain a new energetic compound 23. Moreover, the energetic intermediate 25 was obtained from 2 and 2,2-dimethyl-5-nitro-5-nitroso-1,3-dioxane by oxidative coupling using DBI (dibromocyanuric acid) and then hydrolysis. Using 25 as the intermediate, compound 26 could be obtained by the nitration of NO2BF4 introducing into nitrate groups. The dinitromethyl structure could also be obtained through bromination, reduction, and nitration from 26 so as to further improve the energy and density of target energetic compounds (Zhang et al., 2016). (Scheme 2B)
Bridging multiple furazan through ether bonds can significantly enhance the density level, improve oxygen balance, and increase flexibility of the aromatic molecules. Most furazanyl ether compounds exhibit high energy density, high standard enthalpy of formation, high nitrogen content, low melting point and strong plasticity, making them ideal candidates as energetic plasticizers or oxidant components in low signal characteristic propellant (Wang et al., 2012).,
Starting from 3-amino-4-cyanofurazan 1, 3-nitro-4-cyanofurazan 27 was provided under Caro’s acid oxidation conditions (Youssif, 2001). A novel intermolecular etherification was carried out under alkaline conditions with the oxygen bridged compound 3,3-dicyanodifurazan ether FOF-2 obtained in good yield (Fan et al., 2009). (Scheme 3A) With an impact sensitivity of 0%, a friction sensitivity of 0%, and an H50 value greater than 125.9 cm, FOF-2 was regarded as a good candidate of an insensitive high-energy plasticizer and used as an important starting material for the synthesis of other furazan energetic compounds.
SCHEME 3. (A) Synthesis of FOF-2; (B) synthesis of 28 and FOF-13; (C) synthesis of 37 and 38; (D) synthesis of 41 and 42.
3,3’-Bis (tetrazol-5-yl) difurazanyl ether 28 (ρ: 1.87 g cm−3, D: 8,720 m s−1, P: 33.41 GPa) was synthetized by Li et al. from [3 + 2] cycloaddition between FOF-2 and sodium azide in 88% yield. The contained intensive hydrogen bond interactions increased the thermal stability of the target compound substantially (Li et al., 2012). 3.3'-Bis (fluorodinitromethyl) difurazanyl ether (FOF-13) was prepared by Wang et al. from FOF-2 through the five-step reactions of cyano group addition, diazotization, N2O5 nitration, KI reduction, and XeF2 fluorination (Wang et al., 2014). (Scheme 3B) FOF-13 is regarded as a potential energetic plasticizer due to its high density of 1.97 g cm−3, good thermal stability (no obvious thermal decomposition below 270°C), and excellent compatibility with most other components of mixed explosives and solid rocket propellants.
3-Nitro-4-cyanofurazan 27, a substance obtained by the oxidation of 3-amino-4-cyanofurazan 1, was etherified with 3,4-dihydroxyfurazan sodium salt and yielded an oxygen-bridged trifurazan ether intermediate of 33 with a yield of 89% (Sheremetev et al., 1998). A new green primary explosive 3,4-bis(3-dinitromethylfurazan-4-oxy) furazan 37 (ρ: 2.09 g cm−3, D: 8,431 m s−1) was synthesized by Zhai et al. via the four-step reactions of addition, diazotization, nitration and reduction. Compared with the common applied primary explosive of lead azide; 37 was a nontoxic compound and showed stronger initiation ability (Zhai et al., 2015a). In the following year, 3,4-bis(3-fluorodinitromethylfurazan-4-oxy) furazan 38, which showed the characteristics of good thermal stability (Td: 197.8°C), high density (1.88 g cm−3), low melting point (50°C), and high energy level (D: 8,644.5 m s−1, P: 34.0 GPa), was also obtained by Zhai et al. from further fluorination of 37 (Scheme 3C). The newly developed compound could be used as an energetic plasticizer with excellent comprehensive properties (Zhai et al., 2016a).
3,4-Bis (4'-nitrofurazan-3'-yl) furoxan (DNTF) was synthesized from 3-amino-4-cyanofurazan 1 via the four-step reactions of cyano addition, diazotization–chlorination, intermolecular cyclization, and oxidation (Zhou et al., 2011). Bifurazano[3,4-b:3',4'-f] furoxano[3″,4″-d] oxacyclohetpatriene 41 (ρ: 1.866 g cm−3, m.p.: 92°C, D: 8,256 m s−1, H50: 57.5 cm) was obtained by Zhou et al. via intramolecular etherification of DNTF under the basic conditions of Na2CO3 in acetonitrile solution (Zhou et al., 2012). Trifurazanoxyheterocycloheptene 42 (ρ: 1.935 g cm−3, m.p.: 76.5°C, D: 8,646 m s−1, H50: 72.4 cm) was further developed by Wang et al. from the triethylphosphite reduction of 41 with a yield of 83% (Wang et al., 2020). (Scheme 3D) These two cyclic furazan ether energetic compounds exhibited the characteristics of low melting point, low sensitivity, and high energy density and were potential liquid carrier explosive components in melting and casting explosives.
Methylene-bridged compound 43 was developed by Sun et al. from the condensation reaction of 3-amino-4-cyanofurazan and formaldehyde (Sun et al., 2017). N,N’-methylenebis(n-(4-(2H-tetrazol-5-yl) 1,2,5-oxadiazol-3-yl) nitramide) 45, which showed excellent energy performances (D: 9,043 m s−1, P: 35.6 GPa) and acceptable sensitivities (IS:16 J, FS:180 N), was obtained by the nitration and addition reactions. It was noteworthy that the tetrazole group was converted to a carboxyl group when 45 was nitrated with the mixed 100% nitric acid and trifluoroacetic anhydride; the rarely reported method provided a new strategy for the preparations of heterocyclic carboxylic acids (Scheme 4).
Tetrazoles have high nitrogen content and high positive enthalpy of formation and are, therefore, very suitable as building blocks for the development of energetic materials. The hybridization of tetrazoles and furazans/furoxans was an effective strategy to construct a series of energetic compounds with hybrid furazan/furoxan-tetrazole frameworks, which also greatly expand the research and applications of tetrazole-based energetic substances (Kumar et al., 2017).
The cycloaddition reaction between cyano and azido moieties was one of the important methods in the synthesis and construction of tetrazoles, and the mechanism of the transformation is shown in Scheme 5A: under acidic conditions, tetrazole structure was formed from the 1,3-dipole cycloadditions between cyano and azido groups (Fershtat et al., 2015). Using cyanofurazan (furoxan) as the starting material, a series of tetrazole energetic compounds with excellent properties have been prepared.
SCHEME 5. (A) Mechanism of 1,3-dipole cycloaddition reaction toward tetrazoles; (B) Synthesis of 48, 49, and 50 and its energetic ionic salts; (C) synthesis of 52, 53, 54, 60, 61, and 62; (D) synthesis of 68 and its energetic ionic salts.
3-Amino-4-(tetrazol-5-yl) furazan 47 was synthetized by Wang et al. via cycloaddition from 3-amino-4-cyanofurazan 1 with a yield of 90% (Wang et al., 2011). Based on the activity of the amino group and tetrazole group in 47, a series of new energetic compounds were successfully obtained. 3,3-bis(1H-tetrazol-5-yl)-4,4-azofurazan 48 (ρ: 1.69 g cm−3, D: 8,477 m s−1) was prepared by the oxidation of 47 by potassium permanganate under acidic conditions, and 3-nitro-4-(1H-tetrazol-5-yl) furazan 49 (ρ:1.67 g cm−3, D:8,257 m s−1) was prepared by the oxidation of the amino group (Gao et al., 2013). In addition, three nitrogen-rich energetic salts were obtained by using the acidity of tetrazole in 47, which could be potential energetic components in the propellant (Scheme 5B)
Based on the similar transformations, 3,3’-bis (1H-tetrazol-5-yl)-4,4’-azofuroxan 52, 4-nitro-3-(1H-tetrazol-5-yl) furoxan 53, and its energetic salt were prepared from 4-amino-3-cyanofuroxan 2 (Zhai et al., 2018; Liang et al., 2013). The detonation performance for the ammonium salt of 53 (ρ: 1.84 g cm−3, D: 8,919 m s−1, P: 36.2 GPa) was close to that of RDX. As shown in Scheme 5C, using 3,4-dicyanofuroxan 4 as the starting material, two tetrazole frameworks were introduced to obtain 3,4-bis (1H-tetrazol-5-yl) furoxan 54 (ρ: 1.62 g cm−3, D: 7,778 m s−1, P: 23.9 GPa) (Zhai et al., 2015b). From 3,4-dicyanofurazan 3, some tetrazolylfurazans could be obtained from nitrile precursors (Godovikova et al., 2009). After the convention of one cyano group to the fluorodinitromethyl group through cyanoaddition, diazotization, nitration, reduction, and fluorination, the other cyano group was then turned into a tetrazolyl moiety, leading to 3-(1H-tetrazol-5-yl)-4-fluorodinitromethylfuroxan 60 with excellent detonation properties (D: 8,923 m s−1, P: 36.3 GPa) and broad application prospects in solid rocket propellants and explosives (Zhai et al., 2020a).
The cycloadditions of chlorooxime and azide are widely applied in the synthesis of hydroxytetrazole compounds. Starting from 3-amino-4-cyanofurazan 1, 5-(4-amino-1,2,5-oxadiazol-3-yl)-1-hydroxytetrazole 55 was prepared by Zhai et al. through NH2OH addition, diazotization–chlorination, NaN3 substitution, and cycloaddition reaction in an 89% yield (Zhai et al., 2016b). The nitroamino group in 5-(4-nitroamino-1,2,5-oxadiazol-3-yl)-1-hydroxytetrazole 65 was then introduced by Wei et al. through the nitration of 64, based on which the corresponding hydroxylaminium salt 68a and hydrazinium salt 68b were further obtained (Wei et al., 2015a). The two energetic salts showed high density (1.84 g cm−3, 1.74 g cm−3), excellent detonation pressure (38.3 GPa, 32.2 GPa), and detonation velocity (9,323 m s−1, 9,094 m s−1), which were better than those of the high explosive of RDX and insensitive explosive of TATB (Scheme 5D).
Tandem furoxans are significant energetic frameworks which have been widely applied in the development of HDEMs and achieved great success in recent years. Theoretical studies proved that replacing one nitro group with a furoxan moiety could increase the density of compounds by (0.06–0.08) g·m−3 and the corresponding detonation velocity by more than 300 m s−1 (Wang et al., 2021). Therefore, the design and synthesis of C–C bonded trifuroxan compounds was an effective method to obtain energetic compounds with outstanding energetic properties (Zhai, et al., 2019b).
Chlorooxime intermediate 56 was prepared by Zhai et al. from 3,4-dicyanofuroxan 4 via the reaction process of cyano addition and diazotization, and a new type of energetic compound 3,4-bis (3-cyanofuroxan-4-yl) furoxan 69 was then obtained by two cyclization methods (Zhai et al., 2019c; Zhai et al., 2021): 1) under the condition of a weak base, HCl in 56 was removed and transformed into nitrile oxide structure and then furoxan was built by bimolecular dimerization–cyclization reaction for nitrile oxide; 2) the dinitromethyl potassium salt obtained by nitration of 56 was removed from a molecule of nitric acid and NO2+ under the action of NO2BF4 to form a nitrile oxide intermediate, and then the target compound was formed by the dimerization–cyclization of nitrile oxide (Scheme 6A).
SCHEME 6. (A) Synthetic methods and reaction mechanism toward 69; (B) synthesis of 70, 75, and 78; (C) synthesis of 84 and 85.
Compound 69 was also an energetic intermediate with good expansibility, and using the reaction activity of the cyano group, tetrazolyl- (Zhai et al., 2020b) and fluorodinitromethyl-(Zhai et al., 2019c) substituted trifuroxan high-energy compounds could be obtained. Among them, 3,4-bis (3-tetrazolylfuroxan-4-yl) furoxan 70 had a very high heat of formation (1,290.8 kJ mol−1) and good detonation performances (D: 8,621 m s−1, P: 31.5 GPa), while 3,4-bis (3-fluorodinitromethylfuroxan-4-yl) furoxan 75 showed very excellent detonation performances (D: 9,509 m s−1, P: 42.6 GPa), which was close to those of CL-20. It is noteworthy that the synthesis sequence was changed under the same reaction conditions in each step; first, the cyano group in 4 was converted to the fluorodinitromethyl group; then, another cyano group was mainly dimerized and cyclized by a multistep reaction; last, the regional isomer 3,4-bis (4-fluorodinitromethylfuroxan-3-yl) furoxan 78 (D: 9,196 kJ mol−1, P: 38.8 GPa) was obtained in a 51% yield (Scheme 6B). Due to the synergistic effect between fluorodinitromethyl group and the N-oxidation bond of adjacent furoxan, the densities of the two isomers were significantly different (ρ (75): 2.00 g cm−3, ρ (78): 1.91 g cm−3). Therefore, this regiochemical modulation was expected to be an effective strategy to construct high-density energetic materials.
The amino group in 2 is too reactive and needs to be protected when NaNO2/HCl conditions are necessary. After the diazotization of aminooxime, dimerization and cyclization were carried out to provide the tandem furoxan framework. Deprotection reaction could be conducted under acidic conditions in the late stage, and the amino group given could be turned to nitro or azo moieties under oxidative conditions. Using this strategy, 3,4-bis(4’-aminofuroxano-3’) furoxan 83 was successfully synthesized by He et al. from 3-cyano-4-aminofuroxan (He et al., 2018). Under the oxidation of trichloroisocyanuric acid, an intramolecular azo bridge would be formed from 83, and trifuroxan-fused 1,2-diazocine compound 84 (IS: 19 J, FS: 80 N, D: 9,417 m s−1, P: 39.2 GPa) was obtained in a 64% yield, which was expected to be a substitute for RDX and HMX. Under the condition of HOF-CH3CN, 3,4-bis (4’-nitrofuroxano-3’) furoxan 85 (ρ: 1.914 g cm−3, D: 9,503 m s−1, P: 40.8 GPa) was obtained in a 75% yield by the amino group being oxidized to the nitro group, and its high density, positive oxygen balance, and detonation properties were close to those of CL-20 (Scheme 6C).
Although the formation enthalpy of isofurazan (1,2,4-oxadiazole and 1,3,4-oxadiazole) is lower than that of furazan, its stabilities toward external stimuli are much higher (Xue et al., 2019). Therefore, the introduction of isofurazans as desensitizing moieties to high-energy energetic compounds to form conjugate systems was an important strategy for the design and synthesis of low sensitivity and high-energy materials (Xue et al., 2020).
Amidoxime intermediate 6 obtained by cyano addition of 3-amino-4-cyanofurazan could be applied in the synthesis of various 1,2,4-oxadiazole energetic compounds with carboxylic acid derivatives and nitriles. From a mechanical point of view, 1,2,4-oxadiazole was synthetized from amidoxime and ester under alkali catalysis. As shown in Scheme 7A, the oxygen on the amidoxime is negatively ionized and nucleophilic to attack the carbonyl carbon of the ester to form the C–O bond, then 1,2,4-oxadiazole was constructed after the electron was transferred, -OR3 was cleaved, and a molecule of water was removed. As shown in Scheme 7B, using high-resistant organic base 2,4,6-trimethylpyridine as the catalyst, compound 86 was obtained by the reaction process of dehydration and cyclization from 6 and diethyl oxalate, and then 3,3’-bis (3-nitro-1,2,5-oxadiazol-4-yl)-5,5’-bi-1,2,4-oxadiazole 87 was synthetized by Tsyshevsky et al. based on nitration of 86 (Tsyshevsky et al., 2017). LLM-200 showed a theoretical density of 1.94 g cm−3, a detonation velocity of 8,780 m s−1, and a characteristic drop height (H50) of 62 cm, making it a high-energy, insensitive, heat-resistant explosive with good stability and detonation performance equivalent to that of RDX.
SCHEME 7. (A) Cyclization mechanism of amidoxime and ester; (B) synthesis of 87; (C) O-acylation mechanism of amidoximes and anhydride via acid catalysis; (D) synthesis of 89 and 91.
Anhydride was a strong acylating agent, which easily triggered O-acylation with amidoxime catalyzed under acidic or alkaline conditions. As the mechanism shown in Scheme 7C, anhydride was converted to carboxylic acid and carbonyl carbonium ion in acidic conditions, and then C–O bonds were formed by lone pair electrons on amidoxime attacking carbonium ions. The corresponding azo products (89, 91) were obtained by Qu et al. from 6 via O-acylation of acetic anhydride or trifluoroacetic anhydride, cyclization, and oxidation of the KMnO4 / HCl system (Qu et al., 2016). (Scheme 7D)
1,2,4-Oxadiazole framework was normally formed by the condensation and cyclization of amide and amidoxime. 4-amino-3-(5-methyl-1,2,4-oxadiazol-3-yl)furazan 88 was synthesized by Yang et al. using 6 and acetamide as starting materials, and the active amino group on furazan was further applied in the following derivatizations (Yu et al., 2017): 1) N-(4-(5-methyl-1,2,4-oxadiazol-3-yl)-1,2,5-oxadiazol-3-yl)-N-(2,2,2-trinitroethyl) nitramide 93 (ρ: 1.78 g cm−3, D: 8,602 m s−1, P: 32.8 GPa) was obtained through the Mannich reaction of trinitroethanol catalyzed by Lewis acid which introduced the trinitroethyl energetic group, and the N-NO2 moiety was formed after further nitration. 2) The nitramino group was formed by the nitration of 88, and several energetic ionic salts were obtained based on the neutralization nitramino moiety. Among them, hydroxylaminium salt 95a exhibited good thermal stability (Td: 212°C) and detonation performance (D: 8,350 m s−1, P: 27.3 GPa) better than that of TNT (Scheme 8A). In addition, Qu et al. reported that under the catalysis of ether boron trifluoride, 4-(1,2,4-oxadiazol-3-yl)-1,2,5-oxadiazol-3-amine 96 was synthetized from 6 and (EtO)3CH. The azo moiety could also be formed by the oxidative coupling of 96. (Qu et al., 2016). (Scheme 8B)
SCHEME 8. (A) Synthesis of 93, 95 and its energetic ionic salts; (B) synthesis of 96; (C) cyclization mechanism of 1,2,5-oxadiazole from BrCN; (D) synthesis of 100 and 102 .
Using BrCN and amidoxime as starting materials under alkaline conditions, O-acylation could be carried out and then 1,2,4-oxadiazole could be formed by dehydration and cyclization (Scheme 8C). As shown in Scheme 8D, 3-amino-4-(5-amino-1,2,4-oxadiazol-3-yl) furazan 98 was synthesized by Shaposhnikov et al. by the reaction of 6 and BrCN (Shaposhnikov et al., 2002). The activity of the amino group on furazan in 6 was higher than that of 1,2,4-oxadiazole, and 100 (ρ: 2.12 g cm−3, D: 10114 m s−1) could be obtained by the process of coupling and nitration (Qu et al., 2016). 102 (ρ: 1.92 g cm−3, D: 9,240 m s−1) could also be obtained by different reaction processes of nitration and coupling (Wang et al., 2018).
Nitrile oxide was formed after the extrusion of NH3 in amidoxime activated by PTSA-ZnCl2, and 1,2,4-oxadiazole could then be obtained by 1,3-dipole cycloaddition of nitrile oxide and organic nitrile. 3,5-bis(3-amino-furazan-4-yl)-1,2,4-oxadiazole 103 was reported by Shreeve et al. starting from the cyclization reaction of 3-amino-4-cyanofurazan 1 and the amidoxime intermediate 6 in the TsOH/ZnCl2 catalytic system. The nitroamino compound 104 and its energetic ionic salts were obtained by nitration and neutralization, among which the density of ammonium salt 105a was 1.71 g cm−3 and the detonation velocity was 8,603 m s−1 (Wei et al., 2015b). (Scheme 9A)
SCHEME 9. (A) Cyclization mechanism of 1,2,5-oxadiazole from organic nitrile and syntheses of 104 and its energetic ionic salts; (B) synthesis of energetic ionic salt 108a-108i.
The hydrazide intermediate 106 was prepared by the hydrolysis, acylation, and hydrazinolysis from 3-amino-4-cyanofurazan. The 3-amino-4-(2-amino-1,3,4-oxadiazol-5-yl)-1,2,5-oxadiazole 107 was then obtained by the condensation of 106 and BrCN (Tang et al., 2015). Since the amino group of 1,3,4-oxadiazole was inactive, only mononitration products could be obtained by the nitration of 107. A series of energetic ionic salts (108a-108i) were synthesized by further neutralization reaction with a density of 1.61–1.81 g cm−3 and a theoretical detonation velocity of 7,493–8,711 m s −1 (Scheme 9B).
Wingard et al., (2016; Wingard et al., (2017) show that energetic compounds with heterocyclic framework and alkyl nitrate groups had good wetting and plasticizing properties. Compared with tetrazole or triazole, isoxazole was easier to introduce the alkyl nitrate side chain into the N-heterocyclic skeleton. 3,4-bis(5-nitroxymethylisoxazol-3-yl) furoxan 112 (ρ:1.712 g cm−3, m.p.:89.8°C, D:7,374 m s−1), which could be used as a new type of molten explosive, was synthesized by Johnson et al. from 3,4-dicyanofuroxan 4 through cyano-addition, diazotization, cyclization, and nitration (Johnson et al., 2020). (Scheme 10A) The specific cyclization mechanism is shown in Scheme 10B: a metastable coordination –C≡N→O moiety was formed from the chlorooxime group under weak base conditions, in which –C≡N→O and –C+ = NO− are resonance forms reacted with the alkyne moiety to give an isoxazole ring structure through [3 + 2] cycloaddition.The melting point and the initial decomposition temperature of 112 are 89.8 and 193.8°C, respectively. With the detonation performance obviously better than that of TNT and low sensitivity to impact and friction (D: 8,350 m s−1, P: 27.3 GPa, IS: 7.8 J, FS: 240 N), 112 was regarded as a potential substitute for TNT in the formulation of melt-cast explosive.
Due to the unique planar structure of fused rings, delocalization resonance of π electrons, and easier conjugate stacking effect, these compounds showed low mechanical sensitivity and high thermal stability (Zhang et al., 2018). At present, the development of fused ring energetic compounds had well-supplemented and expanded the research scope of high-energy density energetic materials.
Based on the reactions between the amino group (-NH2) and cyano group (-CN) in 3-amino-4-cyanofurazan, a series of furazanopyridine derivatives could be obtained from the reaction of β-dicarbonyl compounds. As shown in Scheme 11A, under alkali catalysis, α-carbon anion attacked cyano carbon to form C–C bond and then the amino group attacked the carbonyl group, forming a fused ring structure after the extrusion of H2O. Accordingly, using 3-amino-4-cyanofurazan 1 as the starting material to react with ethyl 3-oxobutanoate and 5,5-dimethylcyclohexane-1,3-dione, the corresponding pyrido furazan fused ring compounds (113, 114) could be yielded (Vasil et al., 2001). Similarly, using malononitrile as the starting material to react with 3-amino-4-cyanofurazan 1, the cyano group could be introduced into the fused ring system and 2,4-diamino-3- cyanopyridofurazan 116, which was conducive to further energetic derivatization, was obtained by Strizhenko et al. (Strizhenko et al., 2020).
SCHEME 11. (A) Cyclization mechanism of pyridofurazan and synthesis of pyridofurazan intermediates 113, 114, and 116; (B) synthesis of 117 and cyclization mechanism of the furazanopyrimidine framework; (C) synthesis of 119 and cyclization mechanism of the triazolofurazan framework.
Taking 3-amino-4-cyanofurazan 1 and its amidoxime derivative 6 as the starting material, the fused ring structure furazanopyrimidine product 117 was synthetized by Pagoria et al. through high-temperature cyclization (Pagoria et al., 2017). The mechanism is shown in Scheme 11B: the lone pair electrons of the amino group (-NH2) in 6 attacked the carbon on the cyano group of 1 to form C–N bond, and then the lone pair electrons of the amino group attacked the = N–OH moiety to remove a molecule of NH2OH to form a pyrimidine ring structure. A new conjugated large π fused ring compound 119 was synthesized Vydzhak et al. using 1 and 118 (Vydzhak et al., 2020). As shown in Scheme 11C, the primary amino group in 118 attacked the cyano group, and then the lone pair electrons of the secondary amino group attacked the carbon on the cyano group to form C–N bond and eliminated a molecule of NH3 to form a fused ring structure. These novel cyclization reactions were valuable for further designing and synthesis of new energetic materials.
Due to the different reaction activities of the two cyano groups in 3,4-dicyanofuroxan 4, the addition reaction usually occurred at the more reactive cyano group in position 4, and then the cyclization reaction was conducted to obtain the fused ring system. Amidoxime intermediate 55 was obtained via nucleophilic addition of 4 with hydroxylamine, and after the tautomerism of 55, the hydroxyl nucleophilic attacked the carbon of the cyano group at position 3 to complete ring closure, and the fused ring compound 120 was obtained (Boyer and Pillai, 1982). (Scheme 12A) Using 4 and hydrazine as starting materials, furazanopyridine products 122 was obtained by Khisamutdinov et al. through two-step nucleophilic addition reaction (Khisamutdinov et al., 1995). As shown in Scheme 12B, the cyano group at position 3 in the cyclization process was more likely to react with the amino group on the hydrazone to form a stable six-membered ring structure.
SCHEME 12. (A) Synthesis of 120 and its cyclization mechanism; (B) synthesis of 122 and the cyclization mechanism of pyridazinofurazan; (C,D) two cyclization mechanism of fused ring structures; (E) three cyano-substituted fused ring structures.
A fused aromatic structure was formed by the 1,3-dipole cycloaddition between 3,4-dicyanofurazan and cyclic compounds containing double bonds. Two possible reaction mechanisms were involved: the first one is the formations of two molecules of cyanide through reductions (Scheme 12C), while the second one is based on the ring opening cracking which eliminates a molecule of acetonitrile to form cyanide (Scheme 12D). A variety of fused ring compounds (123–125) could be further designed and synthesized accordingly, and energetic groups such as tetrazolyl, hydroxytetrazolyl, dinitromethyl, and fluorodinitromethyl could be introduced to further improve the detonation performances of fused aromatic structures (Shimizu et al., 1985). (Scheme 12E)
Cyano-substituted furazan and furoxan have been proven to be important intermediates for the developments of nitrogen-rich energetic compounds. In recent years, an enormous number of synthetic strategies toward energetic structures related with furazan/furoxan have been achieved based on the cyanofurazans/furoxans, revealing that these synthetic intermediates are still full of opportunities and of great interest to the chemical and material scientists around the world. This account summarized the current synthetic methods of cyanofurazan/furoxan structures, including 3-amino-4-cyanofurazan, 4-amino-3-cyano furoxan, 3,4-dicyanofurazan, and 3,4-dicyanofuroxan. Both the advantages and disadvantages of these synthetic methods were analyzed and compared. Based on the reaction activities of the amino and cyano groups in the cyanofurazan/furoxan, the synthetic strategies toward seven kinds of nitrogen-rich energetic compounds, such as azo (azoxy)-bridged, ether-bridged, methylene-bridged, hybrid furazan/furoxan-tetrazole–based, tandem furoxan–based, hybrid furazan-isofurazan–based, hybrid furoxan-isoxazole–based, and fused framework–based energetic compounds were fully reviewed. Amino groups were normally transformed into azo/azoxy or nitro moieties through oxidative processes or reacted with electrophilic structures through substitution or condensation reactions. Cyano groups showed significant advantages in cyclization processes, leading to the formations of various aromatic heterocycles such as tetrazole, furoxan, isofurazan, isoxazole, and complicated fused frameworks and the mechanisms of framework constructions were also highlighted. Furazan and furoxan structures will certainly continue to trigger increasing research in the future. With the in-depth applications of these cyanofurazan/furoxan intermediates, we believe more advanced energetic materials with excellent detonation performances, high thermal stabilities, good insensitivities to impacts/frictions, and convenient synthesis approaches will be achieved.
LW completed the main content of the article, LZ, WS, and MW assisted with literature research and scheme drawings. JZ and BW designed the main content and scope of the review.
This research was funded by the National Natural Science Foundation of China (Grant Nos. 21805223 and 22175139).
The authors declare that the research was conducted in the absence of any commercial or financial relationships that could be construed as a potential conflict of interest.
All claims expressed in this article are solely those of the authors and do not necessarily represent those of their affiliated organizations, or those of the publisher, the editors, and the reviewers. Any product that may be evaluated in this article, or claim that may be made by its manufacturer, is not guaranteed or endorsed by the publisher.
Agrawal, J. P., and Hodgson, R. D. (2006). Organic Chemistry of Explosives. John Wiley & Sons. doi:10.1002/9780470059364
Beyerle, R. D. (1983). 1,2,5-Oxadiazole-3,4-dicarboxylic-acid Derivatives, Process for Their Production, Their Use and Pharmaceutical Preparations Containing Them. EP0075141A1.
Boyer, J. H., and Pillai, T. P. (1982). Dicyanofuroxan and Hydrazine or Hydroxylamine. HETEROCYCLES 19, 1063. doi:10.3987/r-1982-06-1063
Chavez, D., Hill, L., Hiskey, M., and Kinkead, S. (2000). Preparation and Explosive Properties of Azo- and Azoxy-Furazans. J. Energetic Mater. 18, 219–236. doi:10.1080/07370650008216121
Dalinger, I. L., Shkineva, T. K., Vatsadze, I. A., Kormanov, A. V., Kozeev, A. M., Suponitsky, K. Y., et al. (2021). Novel Energetic CNO Oxidizer: Pernitro-Substituted Pyrazolyl-Furazan Framework. FirePhysChem 1, 83–89. doi:10.1016/j.fpc.2021.04.005
Fan, Y., Wang, B., Lai, W., Lian, P., Jiang, J., Wang, X., et al. (2009). Synthesis, Characterization and Quantum Chemistry Study on 3,3’-dicyanodifurazanyl Ether (FOF-2). Chin. J. Org. Chem. 29, 614–620.
Fan, Y., Wang, B., Luo, Y., Zhou, Y., and Yang, R. (2008). Synthesis and crystal Structure of 3,3'-Dicyano-4,4'-Azofurazan (DCAF). Huaxue Shiji 30, 739–742. doi:10.13822/j.cnki.hxsj.2008.10.005
Fershtat, L. L., Epishina, M. A., Kulikov, A. S., Ovchinnikov, I. V., Ananyev, I. V., and Makhova, N. N. (2015). An Efficient Access to (1H-Tetrazol-5-Yl)furoxan Ammonium Salts via a Two-step Dehydration/[3+2]-Cycloaddition Strategy. Tetrahedron 71, 6764–6775. doi:10.1016/j.tet.2015.07.034
Gao, H., Zhang, Q., and Shreeve, J. n. M. (2020). Fused Heterocycle-Based Energetic Materials (2012-2019). J. Mater. Chem. A. 8, 4193–4216. doi:10.1039/C9TA12704F
Gao, L., Yang, H., Wu, B., Cheng, G., and Lu, C. (2013). New Synthetic Route of Five Furazan Derivatives. Chin. J. Energ. Mater. 21, 226–229. doi:10.3969/j.issn.1006-9941.2013.02.014
Godovikova, T. I., Vorontsova, S. K., Konyushkin, L. D., Firgang, S. I., and Rakitin, O. A. (2009). Synthesis of 5-(1,2,5-Oxadiazol-3-Yl)-1h-Etrazoles from 3-Cyano-1,2,5-Oxadiazoles. Russ. Chem. Bull. 58, 406–409. doi:10.1007/s11172-010-0023-5
Grundmann, C., Nickel, G. W., and Bansal, R. K. (1975). Nitriloxide, XVIII1) Das Tetramere der Knallsäure (Isocyanilsäure) und seine Derivate. Justus Liebigs Ann. Chem. 1975, 1029–1050. doi:10.1002/jlac.197519750602
Gu, H., Ma, Q., Huang, S., Zhang, Z., Zhang, Q., Cheng, G., et al. (2018). Gem-dinitromethyl-substituted Energetic Metal-Organic Framework Based on 1,2,3-triazole from In Situ Controllable Synthesis. Chemistry-An Asian J. 13, 2786–2790. doi:10.1002/asia.201800722
Guo, T., Wang, Z., Tang, W., Wang, W., Bi, F., Wang, B., et al. (2018). A Good Balance between the Energy Density and Sensitivity from Assembly of Bis(dinitromethyl) and Bis(fluorodinitromethyl) with a Single Furazan Ring. J. Anal. Appl. pyrolysis 134, 218–230. doi:10.1016/j.jaap.2018.06.012
He, C., Gao, H., Imler, G. H., Parrish, D. A., and Shreeve, J. n. M. (2018). Boosting Energetic Performance by Trimerizing Furoxan. J. Mater. Chem. A. 6, 9391–9396. doi:10.1039/c8ta02274g
Huang, W., Tang, Y., Imler, G. H., Parrish, D. A., and Shreeve, J. n. M. (2020). Nitrogen-Rich Tetrazolo[1,5-B]pyridazine: Promising Building Block for Advanced Energetic Materials. J. Am. Chem. Soc. 142, 3652–3657. doi:10.1021/jacs.0c00161
Jaidann, M., Roy, S., Abou-Rachid, H., and Lussier, L.-S. (2010). A DFT Theoretical Study of Heats of Formation and Detonation Properties of Nitrogen-Rich Explosives. J. Hazard. Mater. 176, 165–173. doi:10.1016/j.jhazmat.2009.10.132
Johnson, E. C., Bukowski, E. J., Sausa, R. C., and Sabatini, J. J. (2019). Safer and Convenient Synthesis of 3,4-Dicyanofuroxan. Org. Process. Res. Dev. 23, 1275–1279. doi:10.1021/acs.oprd.9b00186
Johnson, E. C., Sabatini, J. J., Chavez, D. E., Wells, L. A., Banning, J. E., Sausa, R. C., et al. (2020). Bis(Nitroxymethylisoxazolyl) Furoxan: A Promising Standalone Melt‐Castable Explosive. ChemPlusChem 85, 237–239. doi:10.1002/cplu.201900710
Khisamutdinov, G. K., Mratkhuzina, T. A., Gabdullin, R. M., Abdrakhmanov, I. S., Smirnov, S. P., Rakitin, O. A., et al. (1995). Synthesis of 4,7-diaminopyridazino [4, 5-c] Furoxan. Russ. Chem. Bull. 44, 1499–1500. doi:10.1007/BF00714440
Klapötke, T. M. (2021). “TKX-50: A Highly Promising Secondary Explosive,” in Materials Research and Applications (Singapore: Springer), 1–91. doi:10.1007/978-981-15-9223-2_1
Kozak, G. D., Vasin, A. Y., and D’yachkova, A. V. (2008). Estimating the Explosion hazard of Aromatic Azo Compounds. Combust Explos Shock Waves 44, 579–582. doi:10.1007/s10573-008-0087-9
Kumar, D., Imler, G. H., Parrish, D. A., and Shreeve, J. n. M. (2017). Aminoacetonitrile as Precursor for Nitrogen Rich Stable and Insensitive Asymmetric N-Methylene-C Linked Tetrazole-Based Energetic Compounds. J. Mater. Chem. A. 5, 16767–16775. doi:10.1039/C7TA05394K
Larin, A. A., Shaferov, A. V., Kulikov, A. S., Pivkina, A. N., Monogarov, K. A., Dmitrienko, A. O., et al. (2021). Design and Synthesis of Nitrogen‐Rich Azo‐Bridged Furoxanylazoles as High‐Performance Energetic Materials. Chem. Eur. J. 27, 14628–14637. doi:10.1002/chem.202101987
Li, H., Wang, B., Yu, Q., Li, Y., and Shang, Y. (2012). Synthesis and Characterization of 3,3’-Bis (Tetrazol -5 -yl )difurazanyl Ether. Chin. J. Energ. Mater. 20, 18–21. doi:10.3969/j.issn.1006-9941.2012.01.005
Li, H., Yu, Q.-Q., Zhao, F.-Q., Wang, B.-Z., and An, T. (2014). Crystal Structure and thermal Behavior of Oxy-Bridged bis(1H-Tetrazol-5-Yl)furazan. J. Anal. Appl. Pyrolysis 110, 333–337. doi:10.1016/j.jaap.2014.09.024
Liang, L., Wang, K., Bian, C., Ling, L., and Zhou, Z. (2013). 4-Nitro-3-(5-tetrazole)furoxan and its Salts: Synthesis, Characterization, and Energetic Properties. Chem. Eur. J. 19, 14902–14910. doi:10.1002/chem.201301042
Liu, Y., Zhang, J., Wang, K., Li, J., Zhang, Q., and Shreeve, J. n. M. (2016). Bis(4-nitraminofurazanyl-3-azoxy)azofurazan and Derivatives: 1,2,5-Oxadiazole Structures and High-Performance Energetic Materials. Angew. Chem. 128, 11720–11723. doi:10.1002/anie.201606378
Luo, Y., Ma, L., Wang, B., Zhou, Y., Huo, H., and Jia, S. (2010). Synthesis and Characterization of 3,3'-Dicyano-4,4'- Azofuroxan. Chin. J. Energ. Mater. 18, 538–540. doi:10.3969/j.issn.1006-9941.2010.05.013
Pagoria, P. F., Zhang, M.-X., Zuckerman, N. B., DeHope, A. J., and Parrish, D. A. (2017). Synthesis and Characterization of Multicyclic Oxadiazoles and 1-hydroxytetrazoles as Energetic Materials. Chem. Heterocycl Comp. 53, 760–778. doi:10.1007/s10593-017-2122-9
Parakhin, V. V., Shlykova, N. I., and Luk´yanov, O. A. (2017). 4-(Nitroalkyl- and Dinitroalkyl-ONN-Azoxy)-3-Cyanofuroxans and Some of Their Derivatives. Russ. Chem. Bull. 66, 320–326. doi:10.1007/s11172-017-1734-7
Parker, C. O., Emmons, W. D., Rolewicz, H. A., and McCallum, K. S. (1962). Chemistry of Dinitroacetonitrile-I. Tetrahedron 17, 79–87. doi:10.1016/s0040-4020(01)99006-4
Qu, Y., and Babailov, S. P. (2018). Azo-linked High-Nitrogen Energetic Materials. J. Mater. Chem. A. 6, 1915–1940. doi:10.1039/C7TA09593G
Qu, Y., Zeng, Q., Wang, J., Ma, Q., Li, H., Li, H., et al. (2016). Furazans with Azo Linkages: Stable CHNO Energetic Materials with High Densities, Highly Energetic Performance, and Low Impact and Friction Sensitivities. Chem. Eur. J. 22, 12527–12532. doi:10.1002/chem.201601901
Shaposhnikov, S. D., Korobov, N. V., Sergievskii, A. V., Pirogov, S. V., Mel'Nikova, S. F., and Tselinskii, I. V. (2002). New Heterocycles with a 3-aminofurazanyl Substituent. Russ. J. Org. Chem. 38, 1351–1355. doi:10.1023/A:1021668216426
Sheremetev, A. B., Kulagina, V. O., Aleksandrova, N. S., Dmitriev, D. E., Strelenko, Y. A., Lebedev, V. P., et al. (1998). Dinitro Trifurazans with Oxy, Azo, and Azoxy Bridges. Propellants, Explosives, Pyrotechnics 23, 142–149. doi:10.1002/(sici)1521-4087(199806)23:3<142::aid-prep142>3.0.co;2-x
Shimizu, T., Hayashi, Y., Taniguchi, T., and Teramura, K. (1985). Reaction of 3,4-disubstituted 1,2,5-oxadiazole 2-oxides with Dipolarophiles: Substituent and Solvent Effect on the Reaction Courses. Tetrahedron 41, 727–738. doi:10.1016/S0040-4020(01)96450-6
Singh, R. P., Gao, H., Meshri, D. T., and Shreeve, J. n. M. (2007). Nitrogen-Rich Heterocycles. High Energ. Density Mater. 125, 35–83. doi:10.1007/430_2006_055
Strizhenko, K. V., Vasil’ev, L. S., Suponitsky, K. Y., and Sheremetev, A. B. (2020). 3-Amino-4-(1-amino-2-cyanovinyl)furazans: Synthesis and Cyclization. Chem. Heterocycl Comp. 56, 1103–1107. doi:10.1007/s10593-020-02781-7
Sun, Q., Shen, C., Li, X., Lin, Q., and Lu, M. (2017). 3D-cube Layer Stacking: a Promising Strategy for High-Performance Insensitive Energetic Materials. Cryst. Growth Des. 17, 6105–6110. doi:10.1021/acs.cgd.7b01264
Suntsova, M. A., and Dorofeeva, O. V. (2017). Prediction of Enthalpies of Sublimation of High-Nitrogen Energetic Compounds: Modified Politzer Model. J. Mol. Graphics Model. 72, 220–228. doi:10.1016/j.jmgm.2017.01.013
Swain, P. K., Singh, H., and Tewari, S. P. (2010). Energetic Ionic Salts Based on Nitrogen-Rich Heterocycles: a Prospective Study. J. Mol. Liquids 151, 87–96. doi:10.1016/j.molliq.2009.11.009
Tang, Y., Gao, H., Mitchell, L. A., Parrish, D. A., and Shreeve, J. n. M. (2016). Syntheses and Promising Properties of Dense Energetic 5,5′‐Dinitramino‐3,3′‐azo‐1,2,4‐oxadiazole and its Salts. Angew. Chem. Int. Ed. 55, 3200–3203. doi:10.1002/anie.201600068
Tang, Y., He, C., Mitchell, L. A., Parrish, D. A., and Shreeve, J. n. M. (2015). Energetic Compounds Consisting of 1,2,5- and 1,3,4-oxadiazole Rings. J. Mater. Chem. A. 3, 23143–23148. doi:10.1039/c5ta06898c
Tang, Y., He, C., Mitchell, L. A., Parrish, D. A., and Shreeve, J. n. M. (2016). Potassium 4,4′‐Bis(dinitromethyl)‐3,3′‐azofurazanate: A Highly Energetic 3D Metal-Organic Framework as a Promising Primary Explosive. Angew. Chem. Int. Ed. 55, 5565–5567. doi:10.1002/anie.201601432
Tsyshevsky, R., Pagoria, P., Zhang, M., Racoveanu, A., Parrish, D. A., Smirnov, A. S., et al. (2017). Comprehensive End-To-End Design of Novel High Energy Density Materials: I. Synthesis and Characterization of Oxadiazole Based Heterocycles. J. Phys. Chem. C 121, 23853–23864. doi:10.1021/acs.jpcc.7b07584
Türker, L. (2016). Azo-bridged Triazoles: green Energetic Materials. Defence Technology 12, 1–15. doi:10.1016/j.dt.2015.11.002
Vasil"ev, L. S., Sheremetev, A. B., Khoa, N. K., Dem"yanets, Z. K., Dmitriev, D. E., and Dorokhov, V. A. (2001). Reactions of Cyanofurazans with β-dicarbonyl Compounds. Russ. Chem. Bull. 50, 1280–1286. doi:10.1002/chin.20021712110.1023/a:1014075327382
Viswanath, D. S., Ghosh, T. K., and Boddu, V. M. (2018). Emerging Energetic Materials: Synthesis, Physicochemical, and Detonation Properties. Dordrecht: Springer. doi:10.1007/978-94-024-1201-7
Voronin, A. A., Fedyanin, I. V., Churakov, A. M., Pivkina, A. N., Muravyev, N. V., Strelenko, Y. A., et al. (2020). 4H-[1,2,3]Triazolo[4,5-c][1,2,5]oxadiazole 5-oxide and its Salts: Promising Multipurpose Energetic Materials. ACS Appl. Energ. Mater. 3, 9401–9407. doi:10.1021/acsaem.0c01769
Vydzhak, R. N., Panchishin, S. Y., and Brovarets, V. S. (2020). Application of Nickel Complexes with 1,3-Dicarbonyl Compounds for Synthesis of Fused 4-Aminopyridine-Based Systems. Russ. J. Gen. Chem. 90, 1439–1446. doi:10.1134/S1070363220080101
Wang, B., Li, H., Li, Y., Lian, P., Zhou, Y., and Wang, X. (2012). Review on Energetic Compounds Based on Furoxanyl Ether. Chin. J. Energ. Mater. 20, 385–390. doi:10.14077/j.issn.1007-7812.2016.06.002
Wang, B., Zhai, L., Lian, P., Li, Y., and Li, H. (2014). A Novel Synthesis of 3,3’-bis (Fluoro-dinitromethyl) Difurazanyl Ether (FOF-13). Chin. J. Energ. Mater. 22, 884–886. doi:10.11943/j.issn.1006-9941.2014.06.033
Wang, B., Zhang, G., Huo, H., Fan, Y., and Fan, X. (2011). Synthesis, Characterization and Thermal Properties of Energetic Compounds Derived from 3-Amino-4-(tetrazol-5-Yl)furazan. Chin. J. Chem. 29, 919–924. doi:10.1002/cjoc.201190189
Wang, Q., Shao, Y., and Lu, M. (2018). C8N12O8: A Promising Insensitive High-Energy-Density Material. Cryst. Growth Des. 18, 6150–6154. doi:10.1021/acs.cgd.8b01016
Wang, X., Chen, S., Lian, P., Luo, Z., Jia, S., and Wang, B. (2020). New Facile Synthesis and Quantum Chemistry Studies of Trifurazanooxacycloheptatriene. J. Chem. Eng. Chin. Universities 34, 848–854.
Wang, Y., Wang, S., Li, Z., Sun, L., Yang, X., and Tang, S. (2021). Synthesis of UiO-66 in Supercritical CO2 and its Application in Dye Adsorption. Ind. Eng. Chem. Res. 60, 771–780. doi:10.1021/acs.iecr.0c04201
Wei, H., He, C., Zhang, J., and Shreeve, J. n. M. (2015). Combination of 1,2,4-oxadiazole and 1,2,5-oxadiazole Moieties for the Generation of High-Performance Energetic Materials. Angew. Chem. Int. Ed. 54, 9367–9371. doi:10.1002/anie.201503532
Wei, H., Zhang, J., He, C., and Shreeve, J. n. M. (2015). Energetic Salts Based on Furazan-Functionalized Tetrazoles: Routes to Boost Energy. Chem. Eur. J. 21, 8607–8612. doi:10.1002/chem.201500513
Wingard, L. A., Guzmán, P. E., Johnson, E. C., Sabatini, J. J., Drake, G. W., and Byrd, E. F. C. (2016). Synthesis of Bis-Isoxazole-Bis-Methylene Dinitrate: a Potential Nitrate Plasticizer and Melt-Castable Energetic Material. ChemPlusChem 82, 195–198. doi:10.1002/cplu.201600470
Wingard, L. A., Johnson, E. C., Guzmán, P. E., Sabatini, J. J., Drake, G. W., Byrd, E. F. C., et al. (20172017). Synthesis of Biisoxazoletetrakis(methyl Nitrate): A Potential Nitrate Plasticizer and Highly Explosive Material. Eur. J. Org. Chem. 2017, 1765–1768. doi:10.1002/ejoc.201700280
Wu, B., Liang, J., Wang, J., and An, C. (2017). Preparation, crystal Structure and thermal Decomposition of a 2D MOF High-Nitrogen (N%= 43.3%) Compound [Cd(H2O)2(AFT)2]n (HAFT= 4-Amino-3-(5-Tetrazolate)-Furazan). Main Group Chem. 16, 67–75. doi:10.3233/MGC-160226
Xue, Q., Bi, F.-q., Zhang, J.-l., Wang, Z.-j., Zhai, L.-j., Huo, H., et al. (2020). A Family of Energetic Materials Based on 1,2,4-oxadiazole and 1,2,5-oxadiazole Backbones with Low Insensitivity and Good Detonation Performance. Front. Chem. 7, 942. doi:10.3389/fchem.2019.00942
Xue, Q., Bi, F., Zhang, J., Zhang, J., Wang, B., and Zhang, S. (2019). Advances in the Synthesis and Properties of the Isofurazan Energetic Compounds. Chin. J. Org. Chem. 39, 1244–1262. doi:10.6023/cjoc20181102910.6023/cjoc201811029
Yan, T., Yang, H., and Cheng, G. (2020). Unsymmetrical Functionalization of Bis-1,2,4-Triazoles Skeleton: Exploring for Promising Energetic Materials. ACS Appl. Energ. Mater. 3, 6492–6500. doi:10.1021/acsaem.0c00724
Yin, P., and Shreeve, J. M. (2017). Nitrogen-Rich Azoles as High Density Energy Materials. Adv. heterocyclic Chem. 121, 89–131. doi:10.1016/bs.aihch.2016.04.004
Youssif, S. (2001). Recent Trends in the Chemistry of Pyridine N-Oxide. ARKIVOC 2001, 242–268. doi:10.3998/ark.5550190.0002.116
Yu, Q., Cheng, G., Ju, X., Lu, C., Lin, Q., and Yang, H. (2017). Compounds Based on 3-Amino-4-(5-Methyl-1,2,4-Oxadiazol-3-Yl)furazan as Insensitive Energetic Materials. New J. Chem. 41, 1202–1211. doi:10.1039/c6nj03333d
Zhai, L., Bi, F., Huo, H., Luo, Y., Li, X., Chen, S., et al. (2019). The Ingenious Synthesis of a Nitro-Free Insensitive High-Energy Material Featuring Face-To-Face and Edge-To-Face π-Interactions. Front. Chem. 7, 559. doi:10.3389/fchem.2019.00559
Zhai, L., Bi, F., Luo, Y., Sun, L., Huo, H., Zhang, J., et al. (2019). Exploring the Highly Dense Energetic Materials via Regiochemical Modulation: a Comparative Study of Two Fluorodinitromethylfunctionalized Herringbone Trifuroxans. Chem. Eng. J. 391, 123573. doi:10.1016/j.cej.2019.123573
Zhai, L., Bi, F., Luo, Y., Wang, N., Zhang, J., and Wang, B. (2019). New Strategy for Enhancing Energetic Properties by Regulating Trifuroxan Configuration: 3,4-Bis(3-Nitrofuroxan-4-Yl)furoxan. Sci. Rep. 9, 1–8. doi:10.1038/s41598-019-39723-z
Zhai, L., Bi, F., Zhang, J., Zhang, J., Li, X., Wang, B., et al. (2020). 3,4-Bis(3-tetrazolylfuroxan-4-yl)furoxan: A Linear C-C Bonded Pentaheterocyclic Energetic Material with High Heat of Formation and Superior Performance. ACS omega 5, 11115–11122. doi:10.1021/acsomega.0c01048
Zhai, L., Chang, P., Xu, C., Zhang, Y., Wang, B., Li, P., et al. (2021). Synthesis, crystal Structure and Properties of 3, 4-bis (3-Cyanofuroxan-4-Yl) Furoxan. Chin. J. Energ. Mater. 29, 694–699.
Zhai, L., Fan, X., Wang, B., Bi, F., Huo, H., Li, Y., et al. (2015). Facile Synthesis and crystal Structure of 3, 4-bis (1H-5-Tetrazolyl) Furoxan. Chin. J. Energ. Mater. 23, 1172–1175. doi:10.11943/j.issn.1006-9941.2015.12.004
Zhai, L., Fan, X., Wang, B., Bi, F., Li, Y., and Zhu, Y. (2015). A green High-Initiation-Power Primary Explosive: Synthesis, 3D Structure and Energetic Properties of Dipotassium 3,4-Bis(3-Dinitromethylfurazan-4-Oxy)furazan. RSC Adv. 5, 57833–57841. doi:10.1039/C5RA09822J
Zhai, L., Fan, X., Wang, B., Huo, H., Li, Y., Bi, F., et al. (2016a). Synthesis and Properties of 5-(3-Amino-1,2,5-Oxadiazol-4-Yl) Tetrazol-1-Ol and its Ammonium and Hydroxylammonium Salts. Chin. J. Energ. Mater. 24, 862–867.
Zhai, L., Fan, X., Wang, B., Lian, P., Zhou, C., Huo, H., et al. (2016b). 3,4-Bis (3-Fluorodinitro-Methylfurazan-4-Oxy) Furazan: a New Thermally Stable Plasticizer with High Energy Density. Chin. J. Energ. Mater. 24, 922–924. doi:10.11943/j.issn.1006-9941.2016.09.018
Zhai, L., Wang, B., Fan, X., Huo, H., Li, Y., Bi, F., et al. (2018). Synthesis and Performance Prediction of 3,3’-bis (5-tetrazole) -4,4’-azofuroxan. Chin. J. Explosives Propellants 41, 21–25. doi:10.14077/j.issn.1007-7812.2018.01.004
Zhai, L., Zhang, J., Wu, M., Huo, H., Bi, F., and Wang, B. (2020). Balancing Good Oxygen Balance and High Heat of Formation by Incorporating of -C(NO2)2F Moiety and Tetrazole into Furoxan Block. J. Mol. Struct. 1222, 128934. doi:10.1016/j.molstruc.2020.128934
Zhang, J., Bi, F., Wang, B., Zhang, J., Zhai, L., Li, Y., et al. (2016). Research Progress on the Synthesis of Energetic Compounds Based on α-polynitromethyl-azoxy Moieties. Huozhayao Xuebao 39, 12–19. doi:10.14077/j.issn.1007-7812.2016.06.002
Zhang, J., Wang, Z., Wang, B., Liang, Y., Pan, G., and Zhang, J. (2018). Fused-ring Nitrogen-Rich Heterocycles as Energetic Material: Maintaining a fine Balance between Performance and Stability. Chin. J. Org. Chem. 26, 983–990. doi:10.1002/cplu.201900710
Zhang, J., Zhou, J., Bi, F., and Wang, B. (2020). Energetic Materials Based on Poly Furazan and Furoxan Structures. Chin. Chem. Lett. 31, 2375–2394. doi:10.1016/j.cclet.2020.01.026
Zhang, Q., Zhao, C., Zhang, X., He, C., and Pang, S. (2022). Oxidation of O-Dioxime by (Diacetoxyiodo)benzene: green and Mild Access to Furoxans. New J. Chem. 46, 1489–1493. doi:10.1039/D1NJ05510K
Zhang, W., Zhang, J., Deng, M., Qi, X., Nie, F., and Zhang, Q. (2017). A Promising High-Energy-Density Material. Nat. Commun. 8, 1–7. doi:10.1038/s41467-017-00286-0
Zhou, J., Zhang, J., Wang, B., Qiu, L., Xu, R., and Sheremetev, A. B. (2021). Recent Synthetic Efforts towards High Energy Density Materials: How to Design High-Performance Energetic Structures? FirePhysChem. doi:10.1016/j.fpc.2021.09.005
Zhou, Y., Wang, B., Li, J., Zhou, C., Hu, L., Chen, Z., et al. (2011). Study on Synthesis, Characterization and Properties of 3,4-bis (4'-Nitrofurazano-3'-Yl) Furoxan. Acta Chim. Sinica 69, 1673–1680.
Keywords: nitrogen-rich energetic compounds, cyanofurazan/furoxan, synthesis, reaction mechanism, energetic property
Citation: Wang L, Zhai L, She W, Wang M, Zhang J and Wang B (2022) Synthetic Strategies Toward Nitrogen-Rich Energetic Compounds Via the Reaction Characteristics of Cyanofurazan/Furoxan. Front. Chem. 10:871684. doi: 10.3389/fchem.2022.871684
Received: 08 February 2022; Accepted: 21 February 2022;
Published: 17 March 2022.
Edited by:
Naohiko Yoshikai, Tohoku University, JapanReviewed by:
Matsubara Ryosuke, Kobe University, JapanCopyright © 2022 Wang, Zhai, She, Wang, Zhang and Wang. This is an open-access article distributed under the terms of the Creative Commons Attribution License (CC BY). The use, distribution or reproduction in other forums is permitted, provided the original author(s) and the copyright owner(s) are credited and that the original publication in this journal is cited, in accordance with accepted academic practice. No use, distribution or reproduction is permitted which does not comply with these terms.
*Correspondence: Junlin Zhang, anVubGluLTExMUAxNjMuY29t; Bozhou Wang, d2J6NjAwQDE2My5jb20=
Disclaimer: All claims expressed in this article are solely those of the authors and do not necessarily represent those of their affiliated organizations, or those of the publisher, the editors and the reviewers. Any product that may be evaluated in this article or claim that may be made by its manufacturer is not guaranteed or endorsed by the publisher.
Research integrity at Frontiers
Learn more about the work of our research integrity team to safeguard the quality of each article we publish.