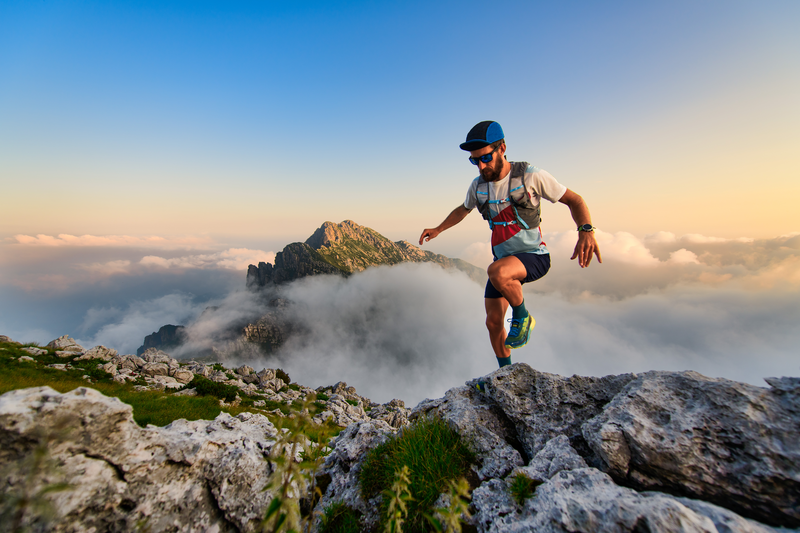
94% of researchers rate our articles as excellent or good
Learn more about the work of our research integrity team to safeguard the quality of each article we publish.
Find out more
MINI REVIEW article
Front. Chem. , 04 March 2022
Sec. Inorganic Chemistry
Volume 10 - 2022 | https://doi.org/10.3389/fchem.2022.865198
This article is part of the Research Topic Stimuli-Responsive Emissive Organic and Metal-Organic Compounds View all 6 articles
Smart luminescent materials, which can respond to the changing of external environment (light, electricity, force, temperature, etc.), have always been one of the research hotspots. Mechanochromism refers to the materials whose emission color or intensity can be altered under the stimulation of external mechanical force. This kind of smart materials have been widely used in data storage, information encryption and sensors due to its simple operation, obvious and rapid response. The introduction of metal atoms in metal-organic compounds brings about fascinating metalophilic interactions and results in more interesting and surprising mechanochromic behaviors. In this mini-review, recent advances in mechanochromism of metal-organic compounds, including mono-, di-, multinuclear metal-organic complexes and metallic clusters are summarized. Varies mechanisms are discussed and some design strategies for metal-organic compounds with mechanochromism are also presented.
Luminescent materials have always been valued by researchers due to their widely use in data storage, optoelectronic devices, biological imaging and other fields (Zhu et al., 2016; Shi et al., 2018; Wang et al., 2021; Jackson et al., 2021; Yin et al., 2021; Min et al., 2022). Metal-organic compounds (MOC), composed of metal ions and organic ligands, are expected to exhibit more interesting photophysical properties. Generally, metal-organic compounds are more conducive in practical application due to their long-lived triplet excited lifetimes and large Stokes shifts. The luminescence color of MOC can be regulated by changing the ligands or by external stimuli, such as light, electricity, temperature, mechanical force, etc (Takeda et al., 2020; Yang M et al., 2020; Yang W et al., 2020). Mechanochromism, a subclass of smart stimuli-responsive materials, refers to the solid materials whose luminescent color or intensity can be changed under the stimulus of mechanical force. Mechanochromic materials have been widely used in data storage, information encryption and sensors due to their simple operation, obvious and fast response (Perruchas et al., 2010; Zhang et al., 2017; Seki et al., 2017; Ito et al., 2008; Song et al., 2018; Li et al., 2019; Zhu et al., 2020). The emission color of mechanochromic materials usually have wide luminous range, covering the entire visible region and even reaching in the near-infrared region (Seki et al., 2017; Zhang et al., 2021; Li et al., 2021). Based on their different phenomenons, mechanochromic materials can be divided into various classes (Figure 1): reversible and irreversible mechanochromism, two-color and multicolor mechanochromism, turn-on and turn-off typed mechanochromism (Yang et al., 2018; Liu et al., 2019; Yang W et al., 2020; Fan et al., 2021; Qian et al., 2021).
To date, most of the reported mechanochromic materials are based on organic molecules (Huang et al., 2019; Jiang et al., 2019; Li et al., 2020; Li and Li, 2020). Traditional organic mechanochromic materials often rely on the change of molecular packing patterns, intermolecular π-π interactions and inermolecular hydrogen-bonding interactions. Although the current research on mechanochromic metal-organic compounds is limited, such mechanochromic materials are still attractive and are expected to exhibit more interesting phenomena due to the metalophilic interactions existed in metal complexes. These mechanochromic phenomenons are thought to result from the crystalline-to-amorphous (CA) phase transformation, single-crystal-to-single-crystal (SCSC) phase transformation or triplet excited states transformation caused by the intermolecular conformational folding or twisting, as well as changes in intermolecular π-π, metalophilic or hydrogen-bonding interactions (Seki et al., 2013; Ito et al., 2013; Seki et al., 2015; Jin et al., 2017; Jin et al., 2018; Li et al., 2019; Pei et al., 2020; Xie et al., 2020). Indeed, an explicit mechanism has not been investigated yet because of the difficulty in characterizing the amorphous phase. Furthermore, the relationship between molecular structures and mechanochromic properties has not been systematically studied, which makes it difficult to find an efficient and simple design strategy for mechanochromic complexes. In this mini-review, a comprehensive summary of recent advances in mechanochromic metal-organic compounds was provided (Figure 2). According to the number of metal atoms in the compounds, we summarized the mono-, di-, and multinuclear complexes, even metallic clusters (Benito et al., 2015; Li et al., 2017; Zhang et al., 2017; Ma, 2019) and metal-organic framework (MOF) (Wei et al., 2016; Wu and Huang, 2018; Jindal et al., 2021) with mechanochromisms, including iridium, platinum, gold, copper and zinc etc. At the same time, the different mechanochromic mechanisms of these complexes were also discussed. Through this review, effective methods for further designing mechanochromic materials based on metal complexes are expected to provide.
Intermolecular interactions play a vital role in mechanochromic materials. Introducing halogen, or oxygen-containing functional groups into ligands is an important way to obtain mechanochromic complexes. Based on this strategy, R. Bryce et al. rationally designed a neutral iridium (III) complex with reversible mechanochromism (Li et al., 2019). The carboxyl and electron-accepting F substituents were incorporated to form intermolecular interactions such as π-π interactions, C-H‧‧‧F and O-H‧‧‧O hydrogen bonds, which played a vital role to achieve the mechanochromism of the iridium (III) complex. Both fourier transform infrared spectroscopy (FTIR) and X-ray photoelectron spectroscopy (XPS) indicated that these intermolecular interactions were broken under grinding. The change of emission color from orange to yellow was resulted from CA transition. From this study, introducing multi-interactions is an effective and facile design strategy to obtain mechanochromic Ir(III) complexes.
Apart from traditional interactions, some infrequent interactions can also promote the formation of loose packing modes. Under mechanical stimulation, the change of interactions lead to the change of stacking modes, resulting in the mechanochromism. Pei and Zhao’ group prepared a series of cationic Ir(III) complexes with significant mechanochromic luminescence (Pei et al., 2020; Zhao et al., 2021). For the first time, the hydroxyl substituents were introduced into Ir(III) complexes to form π-hydrogen bonding. The intermolecular interactions can be easily destroyed by external stimulation, leading to the effective change of luminescent color and showing mechanochromism. Loose packing mode formed by π-hydrogen bonding is critical to establish stimuli responsive phosphorescent materials for Ir(III) complexes.
Compared with the mononuclear complexes, more functional groups can be introduced into the dinuclear metal complexes, and more excellent luminescence properties can be obtained in the dinuclear complexes (Xie et al., 2020). For mechanochromic dinuclear Ir(III) complexes, CA phase transition was still the main mechanism. In recent years, researchers have proposed several specific methods to achieve the stimuli-response properties of iridium complexes. On the one hand, AIE-active units such as tetraphenyl ethylene (TPE) were introduced into the Ir(III) complexes to enhance the luminescent properties in solid states (Li et al., 2020). On the other hand, conformationally flexible Schiff base ligands such as acylhydrazones were introduced into the complexes to enhance intermolecular hydrogen-bonding interactions in the crystals (Jiang et al., 2017a; Jiang et al., 2017b; Li et al., 2020; Xie et al., 2020). In conclusion, both AIE performance and hydrogen-bonding interactions are important for the construction of mechanochromic dinuclear Ir(III) complexes.
The most common mechanism for mechanochromism of complexes was the CA phase transition. Seki et al. reported a number mononuclear Au(I) complexes with mechanochromism, whose emission colors could be red-shifted or blue-shifted (Seki et al., 2018). In 2017, Seki s group prepared a 9-anthryl gold(I) isocyanide complex, which exhibited a bathochromic shift of its emission color from the visible (448 nm) to the infrared (IR) region (710 nm) (Seki et al., 2017). The results of the study indicated that intramolecular ligand-to-ligand charge transfer (3LLCT) was existed in the original crystallic solids of gold(I) complexes, and the aurophilic interactions were formed after grinding, resulting to the formation of metal-metal-to-ligand charge transfer (3MMLCT) states and the extension of the π system, which reduced the energy level of the luminophore and caused red-shifted emission. Notably, this complex possessed the largest red-shifted emission reported so far and provided a facile method to construct infrared light-emitting materials by mechanochromism. On the contrary, some blue-shifted mechanochromic gold(I) complexes were also found. An arylgold (arylisocyanide) complex with hypsochromic mechanochromism was reported in 2018 (Seki et al., 2018). Strong aurophilic interactions were found in the original solids of this complex. However, the weaker aurophilic interactions were detected under the stimulus of mechanical force, which lead to the blue-shifted emission.
A few dinuclear Au(I) complexes with mechanochromism have been reported (Jobbágy et al., 2014a; Jobbágy et al., 2014b; Deák et al., 2015). In 2002, Ito’s group reported the first dinuclear Au(I) complex with reversible red-shift mechanochromic properties (Ito et al., 2008) resulting from CA phase transition. In 2015 and 2016, Chen et al. designed a series dinuclear Au(I) complexes with AIE and mechanochromic properties (Chen et al., 2015; Chen et al., 2016). Similarly, the generation of aurophilic interactions after grinding could turn on or change the luminescence of Au(I) complexes. And the mechanochromism of these complexes were resulted from CA phase transition.
Apart from CA transition, mechano-induced single-crystal-to-single-crystal (SCSC) phase transition was another important mechanism for mechanochromic gold(I) complexes (Ito et al., 2013; Seki et al., 2013; Seki et al., 2015; Jin et al., 2017; Jin et al., 2018). The phase transfer processes could be monitored clearly: The change first happened at the point of contact and then spontaneously spread throughout the entire crystal. Further research indicated that the transition of the intermolecular interactions from C-H‧‧‧π to aurophilic interaction was accompanied by the SCSC phase transition.
The researchers were surprised to find that the neutral-to-ionic (NI) phase transition could contribute to the mechanochromism of Au(I) complexes. In 2015, Baranyai’s group reported a gold(I)-diphosphine complex with reversible mechanochromism (Baranyai et al., 2015). The original neutral mononuclear solid emitted blue luminescence. After mechanical grinding, the neutral mononuclear complex was converted to a ionic dinuclear structure with intramolecular aurophilic interactions, emitting red photoluminescence and showing high contrast mechanochromism.
Both CA and SCSC transitions occured between two phases and caused bicolor mechanochromism. The existence of another metastable state enabled the complexes to exhibit multicolor mechanochromic properties (Zhang Y et al., 2015; Takeda et al., 2018). Wang et al. designed a gold(I)-isocyanide complex demonstrating interesting tricolor mechanochromism feature (Wang et al., 2018). Its emission colors were changed from blue to cyan upon slight grinding, and the cyan light-emitting solids were converted into orange solids after heavy grinding. Interestingly, this orange metastable state could be recovered to the original blue state without any treatment, exhibiting self-recovery tricolor mechanochromism.
The photoluminescence properties of Cu(I) complexes are greatly affected by cuprophilic interaction. However, it is difficult to predict the formation or destruction of Cu-Cu interactions, so it is urgent to develop a simple method to construct mechanochromic copper complexes. Liske’ group investigated some Cu(I) complexes and came up with a new mechanism for mechanochromism (Hupp et al., 2018; Liske et al., 2019). They thought that enhancing the interactions between the cationic metal complexes and suitable counterions could shorten the distance of cation-anion contact and prompt the formation of Cu‧‧‧F interactions, which resulted in the mechanochromic luminescence of Cu(I) complexes. Indeed, this might be a general design strategy worth pursuing.
In the aforementioned Cu(I) complexes, the emission colors could not be returned the original states, showing irreversible mechanochromism. In order to obtain reversible mechanochromic materials based on Cu(I) complexes, flexible and rigid ligands were introduced to prompt the formation of loose stacking modes. Yu et al. reported a steric bis(pyrazol-1-yl)borohydrate-containing Cu(I) complex with reversible mechanochromism (Yu et al., 2021). The study indicated that the pore structure existed in the crystal provided a flexible and loose space, the coordination environment was changed under the stimuli of mechanical force, leading to the distinct mechanochromism.
Similar to other complexes, cuprophilic interaction is important factor for mechanochromic dinuclear Cu(I) complexes. It is crucial to discuss the relationship between structural changes such as Cu-Cu distance and luminescence performance. In 2018, the mechanochromisms of dinuclear Cu(I) complexes bearing N-heterocyclic carbene (NHC) ligands were studied by Lu’s group (Lu et al., 2018). The stimulus-response performance of these complexes came from the CA phase transition. Detailedly, the crystal lattice and the coordination environment of Cu center were destroyed by the stimuli of mechanical force, leading to the shortening of the Cu-Cu distance and the change in emission color. In addition, introducing nitrogenous ligands into the dinuclear Cu(I) complexes was also beneficial to construct mechanochromic Cu(I) complexes. For example, two N-H groups were introduced into the ionic bimetallic Cu(I) complexes to form the N−H∙∙∙O hydrogen bonding with the neighbouring ClO4− anion (Chen et al., 2020). The destruction and recovery of the hydrogen bonding resulted in their reversible mechanochromism.
In recent years, a few trinuclear or tetranuclear Cu(I) complexes with mechanochromic properties resulting from CA phase transition have been reported successively. Xie et al. reported a carbazole-based cyclic trinuclear Cu(I) complex with abnormal pressure-induced phosphorescence enhancement (PIPE) (Xie et al., 2021). The research results indicated that the weak π-acid‧‧‧base interactions existed between the Cu unit and the carbazole group and the Cu‧‧‧Cu interactions played an important role for their turn-on mechanochromism. For tetranuclear Cu(I) complexes, there were many factors that might affect the mechanochromism, such as the change of the Cu‧‧‧Cu distance, π-π interactions and the complex geometry (Benito et al., 2014; Peng et al., 2020; Utrera-Melero et al., 2020; Perruchas, 2021). In 2020, Hu’s group discovered a supersalt-type Cu(I)-thiolate cluster with irreversible mechanochromism for the first time, which was not completely caused by the CA phase transition (Hu et al., 2020). Surprisingly, only the stacking mode was changed and the crystallinity was only destroyed a little. In 2018, a series of luminescent coordination polymers composed of copper(I) iodide were found by Kobayashi’s group (Kobayashi et al., 2018). The switching from thermally activated delayed fluorescence (TADF) to phosphorescene was realized by the stimulus of force, which was caused by the change of excited states from triplet metal-to-ligand and halide-to-ligand charge-transfer (3ML/3HLCT) excited states to cluster-centred (3CC) excited states. In 2017, Xin’s group found a pillared-layered copper(I) MOF, whose mechanochromic performance was resulted from the weak bond contraction of the copper halide layer (Xin et al., 2018). Apart from cuprophilic interaction, the alteration of π‧‧‧π interactions also could give rise to the mechanochromism of Cu MOF (Zhang DX et al., 2015).
Pt (II) complexes have unique photophysical properties due to their multiple excited states, such as LE (local excitation), MLCT (metal-to-ligand charge transfer), LLCT (ligand-to-ligand charge transfer), and ILCT (intraligand charge transfer). These excited states would be changed under the stimuli of force, showing mechanochromism. In 2020, Zhu et al. reported Pt (II) complexes with mechanochromic properties (Zhu et al., 2020). The emission wavelength of original solid powders was at 541 nm, showing orange phosphorescence; a bathochromic red phosphorescence was observed at 639 nm after grinding. Density functional theory (DFT) calculations indicated that the mechanochromic behavior of this complex might be caused by the change in the intermolecular Pt-Pt distance, which resulted to a switching emission state from 3π-π*/3MLCT to 3MLCT. This example proposed a new mechanism of mechanochromic properties and provided a new design strategy to tune phosphorescence by switching triplet excited states.
Zinc is abundant and inexpensive compared with other noble metals, so it is more attractive to design zinc complexes with mechanochromic properties, which would facilitate the development of low-cost sensor materials. In 2018, Song et al. designed a Zn(II) schiff-based complex (Song et al., 2018). The complex displayed high-contrast mechanochromism. XRD, fluorescence microscopy and SEM images demonstrated that the emission-colour transition was caused by the change of molecular packing modes and CA transition.
This paper reviewed the recent advances in mechanochromism of metal-organic compounds such as Ir(III), Au(I), Cu(I), Pt (II) and Zn(II) complexes. Considering the structures of complexes, mono-, di-, multi-nuclear complexes and even clusters, metal-organic frameworks (MOF), and coordination polymers have been summarized according to the type and number of metal atoms. Considering the mechanochromic properties of complexes, various mechanisms were outlined: CA and SCSC phase transitions, NI transition, the pore structure and switching the triplet excited states. By exploring the structure-property relationship of these complexes, several effective design strategies have been proposed: 1) Introduce heteroatom-containing groups to form multiple intermolecular interactions especially the metallophilic interactions; 2) Introduce large flexible groups to form the loose packing modes; 3) Construct the AIE complexes. Despite this, mechanochromic materials still lacked a clear theory because of the difficulty in characterizing the amorphous phase. The current mechanochromic materials suffered from problems that low crystallinity, poor controllability, and low sensitivity. In the future, high-performance mechanochromic materials based on organic-metal compounds that can be used in sensors and anti-counterfeiting fields still need to be further explored. We expect that this paper can provide valuable reference for the development of mechanochromic materials.
Literature research and writing: X-YW, LL; Writing review and editing: X-YW, LL, LS, YH, ZH, ZC; Supervision: ZC. All authors contributed to the article and approved the submitted version.
This study was financially supported by the National Natural Science Foundation of China (nos. 22061018), the Natural Science Foundation of Hebei Province (B 2021106005), the Natural Science Foundation for Distinguished Young Scholars of Jiangxi Province (20212ACB213003), and the Academic and Technical Leader Plan of Jiangxi Provincial Main Disciplines (20212BCJ23004).
The authors declare that the research was conducted in the absence of any commercial or financial relationships that could be construed as a potential conflict of interest.
The reviewer LG declared a shared affiliation, with one of the authors ZC to the handling editor at the time of the review.
All claims expressed in this article are solely those of the authors and do not necessarily represent those of their affiliated organizations, or those of the publisher, the editors and the reviewers. Any product that may be evaluated in this article, or claim that may be made by its manufacturer, is not guaranteed or endorsed by the publisher.
Baranyai, P., Marsi, G., Jobbágy, C., Domján, A., Oláh, L., and Deák, A. (2015). Mechano-induced Reversible Colour and Luminescence Switching of a Gold(I)-diphosphine Complex. Dalton Trans. 44, 13455–13459. doi:10.1039/C5DT01795E
Benito, Q., Le Goff, X. F., Maron, S., Fargues, A., Garcia, A., Martineau, C., et al. (2014). Polymorphic Copper Iodide Clusters: Insights into the Mechanochromic Luminescence Properties. J. Am. Chem. Soc. 136 (32), 11311–11320. doi:10.1021/ja500247b
Benito, Q., Baptiste, B., Polian, A., Delbes, L., Martinelli, L., Gacoin, T., et al. (2015). Pressure Control of Cuprophilic Interactions in a Luminescent Mechanochromic Copper Cluster. Inorg. Chem. 54 (20), 9821–9825. doi:10.1021/acs.inorgchem.5b01546
Chen, Z., Li, Z., Yang, L., Liang, J., Yin, J., Yu, G.-A., et al. (2015). Novel Diisocyano-Based Dinuclear Gold(I) Complexes with Aggregation-Induced Emission and Mechanochromism Characteristics. Dyes Pigm. 121, 170–177. doi:10.1016/j.dyepig.2015.05.021
Chen, Z., Li, Z., Hu, F., Yu, G.-A., Yin, J., and Liu, S. H. (2016). Novel Carbazole-Based Aggregation-Induced Emission-Active Gold(I) Complexes with Various Mechanofluorochromic Behaviors. Dyes Pigm. 125, 169–178. doi:10.1016/j.dyepig.2015.10.038
Chen, X.-W., He, L.-H., Ju, P., Chen, J.-L., Liu, S.-J., and Wen, H.-R. (2020). Mechanochromic Luminescent Materials of Bimetallic Cu(I) Complexes Showing Thermally Activated Delayed Fluorescence. J. Mater. Chem. C 8, 16160–16167. doi:10.1039/D0TC04607H
Deák, A., Jobbágy, C., Marsi, G., Molnár, M., Szakács, Z., and Baranyai, P. (2015). Anion‐, Solvent‐, Temperature‐, and Mechano‐Responsive Photoluminescence in Gold(I) Diphosphine‐Based Dimers. Chem. Eur. J. 21 (32), 11495–11508. doi:10.1002/chem.201501066
Fan, M., Cheng, Y., Fang, B., Lai, L., and Yin, M. (2021). Multicolor Mechanochromism of a Phenothiazine Derivative through Molecular Interaction and Conformational Modulations. Dyes Pigm. 190, 109311. doi:10.1016/j.dyepig.2021.109311
Hu, L., Zheng, A., Kang, Y., Wen, T., and Zhang, J. (2020). A Supersalt-type Copper(I)-thiolate Cluster with Applications for Mechano/thermochromism and the Oxygen Evolution Reaction. Chem. Commun. 56, 3967–3970. doi:10.1039/D0CC00619J
Huang, G., Jiang, Y., Wang, J., Li, Z., Li, B. S., and Tang, B. Z. (2019). The Influence of Intermolecular Interactions and Molecular Packings on Mechanochromism and Mechanoluminescence - a Tetraphenylethylene Derivative Case. J. Mater. Chem. C 7, 12709–12716. doi:10.1039/C9TC04501E
Hupp, B., Nitsch, J., Schmitt, T., Bertermann, R., Edkins, K., Hirsch, F., et al. (2018). Stimulus-triggered Formation of an Anion-Cation Exciplex in Copper(I) Complexes as a Mechanism for Mechanochromic Phosphorescence. Angew. Chem. Int. Ed. 57 (41), 13671–13675. doi:10.1002/anie.201807768
Ito, H., Saito, T., Oshima, N., Kitamura, N., Ishizaka, S., Hinatsu, Y., et al. (2008). Reversible Mechanochromic Luminescence of [(C6F5Au)2(μ-1,4-Diisocyanobenzene)]. J. Am. Chem. Soc. 130, 10044–10045. doi:10.1021/ja8019356
Ito, H., Muromoto, M., Kurenuma, S., Ishizaka, S., Kitamura, N., Sato, H., et al. (2013). Mechanical Stimulation and Solid Seeding Trigger single-crystal-to-single-crystal Molecular Domino Transformations. Nat. Commun. 4, 2009. doi:10.1038/ncomms3009
Jackson, C. T., Jeong, S., Dorlhiac, G. F., and Landry, M. P. (2021). Advances in Engineering Near-Infrared Luminescent Materials. iScience 24, 102156. doi:10.1016/j.isci.2021.102156
Jiang, Y., Li, G., Che, W., Liu, Y., Xu, B., Shan, G., et al. (2017a). A Neutral Dinuclear Ir(III) Complex for Anti-counterfeiting and Data Encryption. Chem. Commun. 53, 3022–3025. doi:10.1039/C7CC00769H
Jiang, Y., Li, G., Zhu, D., Su, Z., and Bryce, M. R. (2017b). An AIE-Active Phosphorescent Ir(III) Complex with Piezochromic Luminescence (PCL) and its Application for Monitoring Volatile Organic Compounds (VOCs). J. Mater. Chem. C 5, 12189–12193. doi:10.1039/C7TC04066K
Jiang, Y., Wang, J., Huang, G., Li, Z., Li, B. S., and Tang, B. Z. (2019). Insight from the Old: Mechanochromism and Mechanoluminescence of Two Amine-Containing Tetraphenylethylene Isomers. J. Mater. Chem. C 7, 11790–11796. doi:10.1039/C9TC04140K
Jin, M., Seki, T., and Ito, H. (2017). Mechano-responsive Luminescence via crystal-to-crystal Phase Transitions between Chiral and Non-chiral Space Groups. J. Am. Chem. Soc. 139 (22), 7452–7455. doi:10.1021/jacs.7b04073
Jin, M., Sumitani, T., Sato, H., Seki, T., and Ito, H. (2018). Mechanical-stimulation-triggered and Solvent-Vapor-Induced Reverse single-crystal-to-single-crystal Phase Transitions with Alterations of the Luminescence Color. J. Am. Chem. Soc. 140 (8), 2875–2879. doi:10.1021/jacs.7b12455
Jindal, S., Anjum, G., Maka, V. K., and Moorthy, J. N. (2021). Mechanoluminescence and Aggregation-Enhanced Emission (AEE) of an In-MOF Based on a 9,9′-Diphenyl-9H-Fluorene Tetraacid Linker. Nanoscale 13, 9668–9677. doi:10.1039/D1NR00898F
Jobbágy, C., Molnár, M., Baranyai, P., and Deák, A. (2014a). Mechanochemical Synthesis of Crystalline and Amorphous Digold(I) Helicates Exhibiting Anion- and Phase-Switchable Luminescence Properties. Dalton Trans. 43, 11807–11810. doi:10.1039/C4DT01214C
Jobbágy, C., Molnár, M., Baranyai, P., Hamza, A., Pálinkás, G., and Deák, A. (2014b). A Stimuli-Responsive Double-Stranded Digold(I) Helicate. CrystEngComm 16, 3192–3202. doi:10.1039/C3CE42474J
Kobayashi, A., Yoshida, Y., Yoshida, M., and Kato, M. (2018). Mechanochromic Switching between Delayed Fluorescence and Phosphorescence of Luminescent Coordination Polymers Composed of Dinuclear Copper(I) Iodide Rhombic Cores. Chem. Eur. J. 24 (55), 14750–14759. doi:10.1002/chem.201802532
Li, Q., and Li, Z. (2020). Molecular Packing: Another Key point for the Performance of Organic and Polymeric Optoelectronic Materials. Acc. Chem. Res. 53 (4), 962–973. doi:10.1021/acs.accounts.0c00060
Li, S.-L., Han, M., Wu, B., Wang, J., Zhang, F.-Q., and Zhang, X.-M. (2017). Observation of Contrary Thermo-Responsive Trend for Single Crystal and Powder Samples in Mechano-, Thermo- and Solvato-Responsive Luminescent Cubane [Ag4I4L4] Cluster. Sci. Rep. 7, 13058. doi:10.1038/s41598-017-11974-8
Li, D., Li, G., Xie, J., Zhu, D., Su, Z., and Bryce, M. R. (2019). Strategic Modification of Ligands for Remarkable Piezochromic Luminescence (PCL) Based on a Neutral Ir(iii) Phosphor. J. Mater. Chem. C 7, 10876–10880. doi:10.1039/C9TC03646F
Li, D., Yang, J., Wang, Y., Li, X., Zhu, D., Fang, M., et al. (2020). Bright Mechanoluminescent Luminogens Even in Daylight through Close Intermolecular Interaction with the Characteristic of Hybridized Local and Charge Transfer (HLCT). J. Mater. Chem. C 8, 10852–10858. doi:10.1039/D0TC01095B
Li, G., Shi, S., Gao, Y., Zhu, D., and Su, Z. (2021). Synthesis, Characterization of Mechanochromic Luminescent-Active Mono-/dinuclear Iridium(III) Complexes with Near-Infrared Emission. J. Organomet. Chem. 931 (1), 121628. doi:10.1016/j.jorganchem.2020.121628
Liske, A., Wallbaum, L., Hölzel, T., Föller, J., Gernert, M., Hupp, B., et al. (2019). Cu-F Interactions between Cationic Linear N-Heterocyclic Carbene Copper(I) Pyridine Complexes and Their Counterions Greatly Enhance Blue Luminescence Efficiency. Inorg. Chem. 58 (9), 5433–5445. doi:10.1021/acs.inorgchem.9b00337
Liu, W., Wang, Y., Yang, J., Li, X., Wang, X., and Ma, L. (2020). The Abnormal Solvatochromism, High-Contrast Mechanochromism and Internal Mechanism of Two AIEE-Active β-diketones. Dyes Pigm. 175, 108149. doi:10.1016/j.dyepig.2019.108149
Lu, T., Wang, J.-Y., Tu, D., Chen, Z.-N., Chen, X.-T., and Xue, Z.-L. (2018). Luminescent Mechanochromic Dinuclear Cu(I) Complexes with Macrocyclic Diamine-Tetracarbene Ligands. Inorg. Chem. 57 (21), 13618–13630. doi:10.1021/acs.inorgchem.8b02217
Ma, X. H. (2019). A Silver Cluster‐Assembled Material Showing Luminescent Mechanochromism and Thermochromism. Chin. J. Chem. 37 (12), 1287–1288. doi:10.1002/cjoc.201900373
Min, X., Yi, F., Han, X.-L., Li, M., Gao, Q., Liang, X., et al. (2022). Targeted Photodynamic Therapy Using a Water-Soluble Aggregation-Induced Emission Photosensitizer Activated by an Acidic Tumor Microenvironment. Chem. Eng. J. 432, 134327. doi:10.1016/j.cej.2021.134327
Pei, Y., Xie, J., Cui, D., Liu, S., Li, G., Zhu, D., et al. (2020). A Mechanochromic Cyclemetalated Cationic Ir(iii) Complex with AIE Activity by Strategic Modification of Ligands. Dalton Trans. 49, 13066–13071. doi:10.1039/D0DT02526G
Peng, D., He, L.-H., Ju, P., Chen, J.-L., Ye, H.-Y., Wang, J.-Y., et al. (2020). Reversible Mechanochromic Luminescence of Tetranuclear Cuprous Complexes. Inorg. Chem. 59 (23), 17213–17223. doi:10.1021/acs.inorgchem.0c02445
Perruchas, S., Le Goff, X. F., Maron, S., Maurin, I., Guillen, F., Garcia, A., et al. (2010). Mechanochromic and Thermochromic Luminescence of a Copper Iodide Cluster. J. Am. Chem. Soc. 132, 10967–10969. doi:10.1021/ja103431d
Perruchas, S. (2021). Molecular Copper Iodide Clusters: a Distinguishing Family of Mechanochromic Luminescent Compounds. Dalton Trans. 50, 12031–12044. doi:10.1039/D1DT01827B
Qian, H., Purwanto, N. S., Ivanoff, D. G., Halmes, A. J., Sottos, N. R., and Moore, J. S. (2021). Fast, Reversible Mechanochromism of Regioisomeric Oxazine Mechanophores: Developing In Situ Responsive Force Probes for Polymeric Materials. Chem 7 (4), 1080–1091. doi:10.1016/j.chempr.2021.02.014
Seki, T., Sakurada, K., and Ito, H. (2013). Controlling Mechano- and Seeding-Triggered Single-Crystal-to-Single-Crystal Phase Transition: Molecular Domino with a Disconnection of Aurophilic Bonds. Angew. Chem. Int. Ed. 52, 12828–12832. doi:10.1002/anie.201307672
Seki, T., Sakurada, K., Muromoto, M., and Ito, H. (2015). Photoinduced single-crystal-to-single-crystal Phase Transition and Photosalient Effect of a Gold(I) Isocyanide Complex with Shortening of Intermolecular Aurophilic Bonds. Chem. Sci. 6 (2), 1491–1497. doi:10.1039/C4SC02676D
Seki, T., Tokodai, N., Omagari, S., Nakanishi, T., Hasegawa, Y., Iwasa, T., et al. (2017). Luminescent Mechanochromic 9-Anthryl Gold(I) Isocyanide Complex with an Emission Maximum at 900 Nm after Mechanical Stimulation. J. Am. Chem. Soc. 139, 6514–6517. doi:10.1021/jacs.7b00587
Seki, T., Kobayashi, K., Mashimo, T., and Ito, H. (2018). A Gold Isocyanide Complex with a Pendant Carboxy Group: Orthogonal Molecular Arrangements and Hypsochromically Shifted Luminescent Mechanochromism. Chem. Commun. 54, 11136–11139. doi:10.1039/C8CC06490C
Shi, C., Zhu, Y., Zhu, G., Shen, X., and Ge, M. (2018). Phototunable Full-Color Emission of Dynamic Luminescent Materials. J. Mater. Chem. C 6, 9552–9560. doi:10.1039/C8TC02955E
Song, X., Yu, H., Yan, X., Zhang, Y., Miao, Y., Ye, K., et al. (2018). A Luminescent Benzothiadiazole-Bridging bis(salicylaldiminato)Zinc(II) Complex with Mechanochromic and Organogelation Properties. Dalton Trans. 47, 6146–6155. doi:10.1039/C8DT00665B
Takeda, Y., Kaihara, T., Okazaki, M., Higginbotham, H., Data, P., Tohnai, N., et al. (2018). Conformationally-flexible and Moderately Electron-Donating Units-Installed D-A-D Triad Enabling Multicolor-Changing Mechanochromic Luminescence, TADF and Room-Temperature Phosphorescence. Chem. Commun. 54, 6847–6850. doi:10.1039/C8CC02365D
Takeda, Y., Data, P., and Minakata, S. (2020). Alchemy of Donor-Acceptor-Donor Multi-Photofunctional Organic Materials: from Construction of Electron-Deficient Azaaromatics to Exploration of Functions. Chem. Commun. 56, 8884–8894. doi:10.1039/D0CC03322G
Utrera-Melero, R., Huitorel, B., Cordier, M., Mevellec, J.-Y., Massuyeau, F., Latouche, C., et al. (2020). Combining Theory and experiment to Get Insight into the Amorphous Phase of Luminescent Mechanochromic Copper Iodide Clusters. Inorg. Chem. 59 (18), 13607–13620. doi:10.1021/acs.inorgchem.0c01967
Wang, X.-Y., Zhang, J., Dong, Y.-B., Zhang, Y., Yin, J., and Liu, S. H. (2018). Different Structures Modulated Mechanochromism and Aggregation-Induced Emission in a Series of Gold(I) Complexes. Dyes Pigm. 156, 74–81. doi:10.1016/j.dyepig.2018.03.062
Wang, Y., Wu, H., Hu, W., and Stoddart, J. F. (2021). Color‐Tunable Supramolecular Luminescent Materials. Adv. Mater., 2105405. doi:10.1002/adma.202105405
Wei, Z.-W., Chen, C.-X., Zheng, S.-P., Wang, H.-P., Fan, Y.-N., Ai, Y.-Y., et al. (2016). Rigidifying Effect of Metal-Organic Frameworks: Protect the Conformation, Packing Mode, and Blue Fluorescence of a Soft Piezofluorochromic Compound under Pressures up to 8 MPa. Inorg. Chem. 55 (15), 7311–7313. doi:10.1021/acs.inorgchem.6b00429
Wu, Z.-F., and Huang, X.-Y. (2018). A Mechanoresponsive Fluorescent Mg-Zn Bimetallic MOF with Luminescent Sensing Properties. ChemistrySelect 3, 4884–4888. doi:10.1002/slct.201800580
Xie, J., Li, D., Duan, Y., Geng, Y., Yang, T., Li, G., et al. (2020). Cationic Dinuclear Ir(III) Complexes Based on Acylhydrazine Ligands: Reversible Piezochromic Luminescence and AIE Behaviours. Dyes Pigm. 172, 107855. doi:10.1016/j.dyepig.2019.107855
Xie, M., Chen, X.-R., Wu, K., Lu, Z., Wang, K., Li, N., et al. (2021). Pressure-induced Phosphorescence Enhancement and Piezochromism of a Carbazole-Based Cyclic Trinuclear Cu(I) Complex. Chem. Sci. 12, 4425–4431. doi:10.1039/D0SC07058K
Xin, B., Sang, J., Gao, Y., Li, G., Shi, Z., and Feng, S. (2018). A Pillared-Layered Copper(I) Halide-Based Metal-Organic Framework Exhibiting Dual Emission, and Piezochromic and Thermochromic Properties with a Large Temperature-dependent Emission Red-Shift. RSC Adv. 8, 1973–1978. doi:10.1039/c7ra11950j
Yang, W., Liu, C., Lu, S., Du, J., Gao, Q., Zhang, R., et al. (2018). AIE-active Smart Cyanostyrene Luminogens: Polymorphism-dependent Multicolor Mechanochromism. J. Mater. Chem. C 6, 290–298. doi:10.1039/C7TC04773H
Yang M, M., Park, I. S., Miyashita, Y., Tanaka, K., and Yasuda, T. (2020). Mechanochromic Delayed Fluorescence Switching in Propeller‐Shaped Carbazole-Isophthalonitrile Luminogens with Stimuli‐Responsive Intramolecular Charge‐Transfer Excited States. Angew. Chem. Int. Ed. 59, 13955–13961. doi:10.1002/anie.202005584
Yang W, W., Yang, Y., Qiu, Y., Cao, X., Huang, Z., Gong, S., et al. (2020). AIE-active Multicolor Tunable Luminogens: Simultaneous Mechanochromism and Acidochromism with High Contrast beyond 100 Nm. Mater. Chem. Front. 4, 2047–2053. doi:10.1039/D0QM00247J
Yin, Y., Chen, Z., Li, R.-H., Yuan, C., Shao, T.-Y., Wang, K., et al. (2021). Ligand-triggered Platinum(II) Metallacycle with Mechanochromic and Vapochromic Responses. Inorg. Chem. 60, 9387–9393. doi:10.1021/acs.inorgchem.1c00233
Yu, X., Li, X., Cai, Z., Sun, L., Wang, C., Rao, H., et al. (2021). Mechanochromic Properties in a Mononuclear Cu(I) Complex without Cuprophilic Interactions. Chem. Commun. 57, 5082–5085. doi:10.1039/D1CC01229K
Zhang DX, D.-X., Zhang, H.-X., Wen, T., Li, D.-S., and Zhang, J. (2015). Mechanochromic Cu(I) boron Imidazolate Frameworks with Low-Dimensional Structures and Reducing Function. Inorg. Chem. Front. 3, 263–267. doi:10.1039/c5qi00155b
Zhang, X., Wang, J.-Y., Qiao, D., and Chen, Z.-N. (2017). Phosphorescent Mechanochromism through the Contraction of Ag12Cu2 Clusters in Tetradecanuclear Copper-Silver Acetylide Complexes. J. Mater. Chem. C 5, 8782–8787. doi:10.1039/C7TC03205F
Zhang, M. X., Yang, X., Zhang, K., Yin, J., and Liu, S. H. (2021). Osmaindenes: Synthesis and Reversible Mechanochromism Characteristics. Chem. Eur. J. 27 (59), 14645–14652. doi:10.1002/chem.202102180
Zhang Y, Y., Wang, K., Zhuang, G., Xie, Z., Zhang, C., Cao, F., et al. (2015). Multicolored-fluorescence Switching of ICT-type Organic Solids with clear Color Difference: Mechanically Controlled Excited State. Chem. Eur. J. 21, 2474–2479. doi:10.1002/chem.201405348
Zhao, K.-Y., Song, W.-L., Yang, Y., Duan, Y.-C., Li, G.-F., Shan, G.-G., et al. (2021). Synthesis of Novel Tetrazol-Pyridine Based Cationic Ir(III) Complexes with Efficient AIE Characteristic and Significant Mechanochromic Luminescence Behavior. Dyes Pigm. 184, 108817. doi:10.1016/j.dyepig.2020.108817
Zhu, X., Su, Q., Feng, W., and Li, F. (2016). Anti-stokes Shift Luminescent Materials for Bio-Applications. Chem. Soc. Rev. 46, 1025–1039. doi:10.1039/C6CS00415F
Keywords: mechanochromism, complex, metalophilic interactions, mechanism, phase transition
Citation: Wang X-Y, Lv L, Sun L, Hou Y, Hou Z and Chen Z (2022) Recent Advances in Mechanochromism of Metal-Organic Compounds. Front. Chem. 10:865198. doi: 10.3389/fchem.2022.865198
Received: 29 January 2022; Accepted: 21 February 2022;
Published: 04 March 2022.
Edited by:
Feng Luo, East China University of Technology, ChinaReviewed by:
Vedichi Madhu, Karunya Institute of Technology and Sciences, IndiaCopyright © 2022 Wang, Lv, Sun, Hou, Hou and Chen. This is an open-access article distributed under the terms of the Creative Commons Attribution License (CC BY). The use, distribution or reproduction in other forums is permitted, provided the original author(s) and the copyright owner(s) are credited and that the original publication in this journal is cited, in accordance with accepted academic practice. No use, distribution or reproduction is permitted which does not comply with these terms.
*Correspondence: Xiao-Yan Wang, d2FuZ3hpYW95YW42MjlAMTYzLmNvbQ==; Zhao Chen, Y2hlbnpoYW82NjZAMTI2LmNvbQ==
†These authors have contributed equally to this work
Disclaimer: All claims expressed in this article are solely those of the authors and do not necessarily represent those of their affiliated organizations, or those of the publisher, the editors and the reviewers. Any product that may be evaluated in this article or claim that may be made by its manufacturer is not guaranteed or endorsed by the publisher.
Research integrity at Frontiers
Learn more about the work of our research integrity team to safeguard the quality of each article we publish.