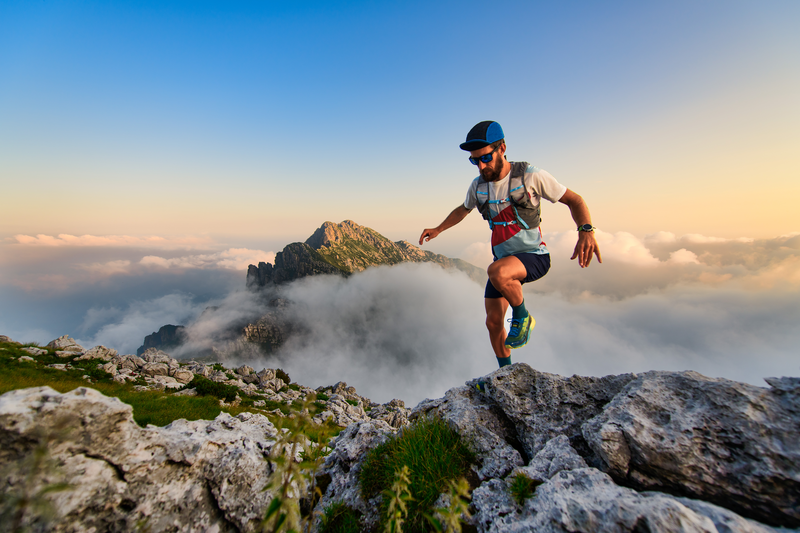
94% of researchers rate our articles as excellent or good
Learn more about the work of our research integrity team to safeguard the quality of each article we publish.
Find out more
REVIEW article
Front. Chem. , 08 March 2022
Sec. Inorganic Chemistry
Volume 10 - 2022 | https://doi.org/10.3389/fchem.2022.840657
This article is part of the Research Topic Emerging Polyoxometalates with Biological, Biomedical, and Health Applications View all 5 articles
Polyoxometalates (POMs) are a class of metal oxide complexes with a large structural diversity. Effective control of the final chemical and physical properties of POMs could be provided by fine-tuning chemical modifications, such as the inclusion of other metals or non-metal ions. In addition, the nature and type of the counterion can also impact POM properties, like solubility. Besides, POMs may combine with carbon materials as graphene oxide, reduced graphene oxide or carbon nanotubes to enhance electronic conductivity, with noble metal nanoparticles to increase catalytic and functional sites, be introduced into metal-organic frameworks to increase surface area and expose more active sites, and embedded into conducting polymers. The possibility to design POMs to match properties adequate for specific sensing applications turns them into highly desirable chemicals for sensor sensitive layers. This review intends to provide an overview of POM structures used in sensors (electrochemical, optical, and piezoelectric), highlighting their main functional features. Furthermore, this review aims to summarize the reported applications of POMs in sensors for detecting and determining analytes in different matrices, many of them with biochemical and clinical relevance, along with analytical figures of merit and main virtues and problems of such devices. Special emphasis is given to the stability of POMs sensitive layers, detection limits, selectivity, the pH working range and throughput.
Polyoxometalates (POMs) are negatively charged polyoxoanions of general formula [MmOy] n–, where M represents the metal centre surrounded by oxygen atoms (O). They are typically composed of transition metal ions in their highest oxidation state (e.g. M = VV, MoVI, WVI, TaV, NbV), bridged by oxo ligands (O2–), to form closed 3-dimensional frameworks (Pope and Müller, 1991). Other elements, mainly heteroatoms, acting as coordination centres, usually labelled as X, can be part of the POM framework [XxMmOy]n− (Pope and Müller, 1991; Hutin et al., 2013). The growing interest in POMs is focused on two main features: 1) the structural diversity due to the coordination flexibility in their metal-oxo structures, and 2) the vast number of elements of the periodic table that can be incorporated inside POM clusters, leading to an overwhelming diversity of molecular structures, of various shapes and sizes, with a diverse range of physical and chemical properties.
Briefly, the history of POMs started in 1783, when Scheele (Long et al., 2010) studied reduced molybdenum salts and discovered what are now known to be the first examples of Molybdenum Blues, followed by the ammonium phosphomolybdate, a yellow precipitate containing the anion [PMo12O40]3−, discovered by Berzelius in 1826 (Hutin et al., 2013). However, only in 1864, with the discovering of the tungstosilicic acids and their salts, now known as [H4SiW12O40]·xH2O, the analytical composition of the 12:1 heteropoly species were precisely determined by Galissard de Marignac (Marignac, 1864). By 1908, around 750 POMs were known. Yet, it was only in the early 30s that J.F. Keggin, using powder X-ray diffraction measurements, revealed the structure of the phosphotungstic acid H3 [PW12O40)].29H2O, which carry his name and is known as the Keggin structure (Keggin, 1934).
In 1991, Pope and Müller (Pope and Müller, 1991) summarised the key features of POMs, highlighting the structural diversity due to the coordination flexibility in their metal-oxo structures and their ability to be functionalized by incorporating virtually any metal from the periodic table. This paper led to an impressively increase in POM’s popularity, reaching an average of 500 publications/year in 2010 (Hutin et al., 2013). Nevertheless, with the advances in material science and nanotechnology, POMs are still seen as promising unique chemical species that could turn very special molecules into very useful materials, as evidenced by the average of around 1,000 articles in each of the last 3 years. Figure 1 shows the evolution in the number of POMs publications (Web of Science, August 2021).
In general, POMs are divided into three main classes:
i) Isopolyoxoanions are POMs of the general formula [MmOy]n–containing only one type of high-valent group V or VI transition metal (M) ion, which is called the addenda atom, and oxygen (O). Commonly, those are much more unstable than their heteropolyoxoanions counterparts (Long et al., 2010).
ii) Heteropolyoxoanions are POMs of the general formula [XxMmOy]n–, containing a high atomic proportion of one type of transition metal atom (M) and a much smaller proportion of the other types of atoms (X), called heteroatoms. X is usually a p or d block element, such as B, S, P or Co. The heteroatom can be either primary, whenever it is essential to the POM structure, or secondary, whenever it fills external vacancies of lacunary structures and can be used, for instance, to link the POM structures to form larger aggregates. Lacunary structures are obtained by the selective removal of one or more metal ions that can be occupied. This strategy of occupying the lacunes with other metal or non-metal atoms is commonly used to modify the structure and properties of POMs. A vast number of POMs derivatives with fascinating architectures have been reported over the years through the self-assembly of purely inorganic building blocks and/or the bridging functions of metal ions and organic ligands (e.g., transition metal-inserted POMs, Ln-substituted POMs, heterometallic POMs, and organic ligand modified POMs) (Wang et al., 2020a).
iii) The third class are the Mo-blue and Mo-brown reduced POM clusters, which are easily recognized by their giant nanosized polymolybdates, such as {Mo154} “big-wheel” or the {Mo132} “big ball” clusters (Hutin et al., 2013).
POMs are seen as the largest non-biologically derived molecules structurally characterized, and these building blocks can be used to construct the systems represented as observed in Figure 2 [adapted from Bijelic et al. (2019)]. Although POMs exhibit a vast diversity in size and structure, the majority of POMs can be identified as one of the four distinct structural families: Lindqvist, Anderson, Keggin, and Well-Dawson type clusters, highlighted in Figure 2 with a yellow box. Such structures are dominant in the field because of their high reproducibility and can be formed by several different types of addenda metal atoms. The Lindqvist structure is the smallest of the four POM types and is adopted by isopolyoxometalates of formula [M6O19]n–. It consists of an octahedral arrangement of six octahedra. Each octahedron, consisting of a metal ion with its coordination sphere, shares four edges with four neighbouring octahedra. The Keggin structure, the most popular structure for heteropolyoxometalates, has a general formula of [XM12O40]n–, or XM12, with tetrahedrally coordinated heteroatoms and four trimetallic groups arranged around a central tetrahedron. The Anderson-Evans structure, also commonly designated as Anderson structure, is the smallest of the common heteropolyoxoanions, incorporating a single heteroatom, X, with the formula [XM6O24]n–, or XM6, with six edge-sharing octahedra arranged into a planar hexagon around the central heteroatom, X. The Well-Dawson structure, commonly known as Dawson structure, is a heteropolyoxometalate with the general formula [X2M18O62]n–, or X2M18. The structure can be seen as a connection of two Keggin units, each of them lacking a {M3O13} unit and connected by a shared corner.
FIGURE 2. Overview of various archetypal POM anions. (A) Keggin, (B) Wells–Dawson, (C) Anderson, (D) Lindqvist, (E) decavanadate, (F) sandwich Keggin, (G) double Keggin, (H) heptamolybdate, (I) α- and (J) γ-octamolybdate, (K) Preyssler, (L) Strandberg, and (M) Krebs-type structure. Blue polyhedra are {MO6} (M = any addenda atom), light green polyhedra {XOn} (X = heteroatom), light green spheres sodium, light blue polyhedra {WO6}, light cyan polyhedra {MoO6}, gray polyhedra {VO6}, purple polyhedra and spheres {YOn} and Y (Y = second heteroatom), orange polyhedra {PO4}, red spheres oxygen. The most common polyoxometalates archetypes are highlighted with a yellow box. Adapted from Bijelic et al. (2019).
The synthesis of POMs is easy to carry out. Basically, all that is needed is an acidic solution containing the relevant metal oxide anions. The reaction either takes place in a single step and is said to be a “one-pot” synthesis or in a small number of multiple steps, using common and relatively inexpensive reagents. Still, a few parameters should be considered in POMs synthesis, namely, the concentration/type of metal oxide anion, pH, ionic strength, heteroatom type/concentration, presence of additional ligands, the reducing agent, the temperature of the reaction, and the process (e.g., microwave, hydrothermal, refluxing) (Long et al., 2010).
POM properties, including molecular composition, size, shape, charge density and redox potentials, are easily tailored by defining the synthesis parameters. Besides, POMs can be rendered soluble in nearly any media, from H2O to hydrocarbons, by properly choosing counter-cations.
One remarkable feature of POMs is their ability to be functionalized by incorporating practically any metal ion from the periodic table. Not many inorganic materials achieve such chemical and structural diversity. Although using pristine POMs can have several disadvantages for specific applications, such as poor conductivity, low specific surface area, leaching, degradation, aggregation, and solubility in aqueous solutions, their functionalizing flexibility can be used to improve the materials. It is possible to obtain highly redox-active materials able to undergo complex electron transfer, making POMs highly desirable functional materials for a myriad of applications.
A very effective way to obtain POMs with specific properties is to form hybrid composite materials by loading POMs onto different supports. The most interesting POM-based composite materials recently reviewed (Miras et al., 2014; Ji et al., 2015a; Herrmann et al., 2015; Wang et al., 2020a; Khalilpour et al., 2021) include POMs-nanocarbon composites that enhance electronic conductivity, POMs-metal composites that increase catalytic and functional sites, POMs-conductive polymers composites that increase conductivity and develop flexible and easily processable materials, and POMs-metal-organic frameworks (POMOFs) composites that increase surface area, expose more active sites and improve stability. Lately, the synergetic effect observed on POM-based multi-material composites (usually triple-materials) has gained increased attention. The combination of the respective advantages of different materials can sometimes endow the composite with unexpectedly improved properties where each material works synergistically, giving superior performances.
POMs are often anchored or immobilized onto substrates. POMs can be attached to substrate surfaces by covalent, electrostatic, or supramolecular bonds and can be present in 3D matrices, on nanostructures, or on flat surfaces. The five main strategies for attaching POM or POM-based materials to a substrate (Cherevan et al., 2020) are dip-coating, Layer-by-Layer (LbL) process, electrochemical deposition, solvothermal deposition and drop-casting, and are briefly described below.
i) Dip-coating is the most simple, easy, and straightforward method, where the substrate is immersed in a solution containing the POM. It has the disadvantages of being prone to leaching, non-homogeneous distribution on the substrate surface, and lack of reproducibility.
ii) The Layer by layer (LbL) process consists of alternate adsorption of opposite charges layers, held in place by electrostatic and Van der Walls forces. This process is known for its simplicity, thickness controlled by adjusting the number of deposited layers, high stability and mechanical strength, and very uniform morphology. Though, depending on the intended thickness, it could be time-consuming.
iii) Electrochemical deposition is limited to conductive substrates and is performed under controlled potential or current, with POMs being deposited on the anodes due to their negative charge, forming monolayers or multilayers. In addition, it enables the obtention of direct electrochemical information about the deposition process.
iv) The Solvothermal deposition occurs in a closed system and requires high temperature and pressure to anchor POM on the substrates. It has the advantage of enabling highly condensed, insoluble lattices while preventing or decreasing leaching and avoiding reversible deposition. However, it is impossible to observe the reaction process (“black box”), and the harsh conditions could lead to structural re-arrangements of POMs.
v) Drop-casting is an easy and fast immobilization method, where POMs are dispersed in a suitable solvent which is then dropped onto a flat surface, followed by evaporation of the solution, forming a thin solid film. This technique is frequently used to modify electrode surfaces for electrocatalysis. Unfortunately, it is not easy to get a uniform coating with a controlled thickness.
The unique versatility in size, thermal stability, multiple and fast redox reactions, photochemical response redox, and magnetic properties are some of the physical and chemical properties that make POMs promising candidates for a wide range of applications. By far, the most popular application of POM-hybrid materials is as catalysts due to their super acidity and excellent structural stability undergoing multi-electron redox cycles (Katsoulis, 1998; Sadakane and Steckhan, 1998; Ren et al., 2015; Patel et al., 2016).
POM anions are also versatile inorganic building blocks for the construction of solid functional materials (Miras et al., 2012). Due to the unique properties of POM hybrid systems, combining redox-active POMs as electron storage sites with nanostructured carbon conducting materials with a high surface, they find applications in the energy field, such as energy storage, energy conversion, and fuel cells (Chen et al., 2015a; Ji et al., 2015a; Herrmann et al., 2015).
POMs also exhibit many ideal properties for use in biological and medical disciplines. They could be extremely small (sub 5 nm), therefore liable to be cleared by the renal system, exhibit low toxicity and are stable in biological media. A great variety of roles have been reported to POMs in combination with natural polymer molecules (proteins, peptides, and amino acids) by taking advantage of the different characteristics of both moieties (Arefian et al., 2017; Bijelic and Rompel, 2018). Additionally, POMs have been gaining relevance in medicine due to their application as antiviral, antibacterial, and antitumor agents and as radiosensitizers in cancer therapy (Bijelic et al., 2019; Guedes et al., 2020; Aureliano et al., 2021).
POMs are also ideal for substrate sensing, and this review aims to present and discuss functionalized POM sensors, explore their applications, and assess their feasibility and contribution to the sensors field.
A POM-based sensor can be defined as an analytical device comprising an immobilized layer of POM on a transducer (Ammam, 2013), as depicted in Figure 3. While POM will be responsible for sensor sensitivity and selectivity, the transducer will be an electrical device responsible for converting one form of energy into another, handling different types of energies such as mechanical, electrical, light, chemical, thermal, acoustic, electromagnetic, etc. After POM immobilization, it will recognize and interact with the analyte. The physical and chemical changes induced by the analyte onto the immobilized POM will be then transformed into an electrical signal, amplified, and converted by the signal processing equipment into a readout signal (Ammam, 2013) (schematically presented in Figure 3). In general, sensitivity depends on the success in POM immobilization and on the deposited POM activity towards a specific analyte (Veríssimo et al., 2010; Gamelas et al., 2018). Selectivity deals with the POM’s preference towards the analyte regarding other species in the sample matrix. The limit of detection (LOD) is the smallest quantity that can be reliably detected. Despite the existence of several quantitative definitions, it is based on the detection of a signal over noise (usually a concentration corresponding to a signal 3 times the noise is accepted) (Veríssimo et al., 2020a). The linear working range of a sensor is the range of concentrations going from the lowest concentrations that can be reliably quantified, the quantification limit (LOQ), and the concentration at which the signal dependence to concentration is no longer linear. Stability deals with the degree to which sensor characteristics remain constant over time. The final goal of any sensor is to reliable detect or quantify an analyte in real samples and, therefore, adequate sensitivity must be assured (Veríssimo et al., 2020b). Sensors are an indispensable tool for our lives, whether detecting a highly toxic metal in lake waters, detecting food frauds, and assuring its safety, or providing clinical tests and detecting cancer at early stages. They can provide security, save lives and improve quality of life.
FIGURE 3. Overview of the sensor composition: from polyoxometalate analyte recognition layer to the transducer and measured signal.
POM-based sensors will be divided and discussed according to their transducer principle: electrochemical, optical and piezoelectric (mass). Figure 4 schematically shows one possible arrangement of a POM-based device for each transducer.
FIGURE 4. Schematic overview of the most common operation principle of a POM-based electrochemical sensor (A), POM-based fibre optic sensor (B) and POM-based mass sensor (C).
Electrochemical sensors extract information about the analyte from the measurement of some electrical parameters. They can be categorized according to the measured electrical parameter: potential (potentiometric sensors), current (amperometric sensors), and resistance or conductance (conductimetric sensors, namely chemiresistors and semiconductor metal oxide sensors).
Albeit conductometric/chemiresistive sensors have the advantage of low-cost fabrication, only a few articles were found reporting chemiresistors or conductimetric POM-based sensors. A resistive humidity sensor based on a Keggin H3PMo12O40- polypyrrole nanocomposite was reported by Miao et al. (2018). To prepare the humidity sensing material by co-electrodeposition, the polyoxoanion of the phosphomolybdic acid was chosen as the anionic building block and the protonated polypyrrole (PPy) was selected as the cationic building block because of its relatively large size and hydrophobicity. The optimized resistive humidity sensor showed a rapid response and recovery time (1.9/1.1 s at a 98% RH level, respectively), a sensing range of 11–98% RH, excellent durability, and repeatability with little hysteresis, superior to commercial thermosetting polyester. Other POMs have also been used to enhance gas sensing, such as the Keggin H5PMo10V2O40 that was proposed to mediate the key Pt (II)-Pt (IV) oxidation while itself being regenerated by O2, for detection of methane in chemiresistive sensors (Bezdek et al., 2021), the cyanometalate-functionalized POM (C4H10ON)23 [HN(CH2CH2OH)3]10H2 [FeIII(CN)6 (α2-P2W17O61CoII)4]·27H2O to improve photoconductivity and gas sensing performances for formaldehyde and methylbenzene in SnO2-based gas sensors (Wang et al., 2017), and the Keggin H4SiW12O40, used as a dopant for detecting a series of chemical vapours in polyaniline (PANI) nanotubes (Gao et al., 2007). Also, Amman et al. (Ammam and Easton, 2011a) reported a POM hybrid compound, the [K4(Py)2(P2Mo18O62)], for detection of NOx, a generic term for nitric oxide NO and nitrogen dioxide (NO2), both toxic gases that can be harmful to health in various ways. Starting with a Dawson K6P2Mo18O62. nH2O, which was used as an oxidizing agent to polymerize pyrrole (Py) in the presence of the mild reducing agent potassium iodide (KI), they accomplished to generate a hybrid material with low Py content. The resulting semiconducting composite illustrated a selective and sensitive response to NOx gases when exposed to various gases and extended linearity up to 5,500 ppm.
It must be emphasized that most POM-based electrochemical sensors found in the literature are amperometric. The standard electrochemical cell consists of a working electrode, a counter electrode, and a reference electrode connected to a potentiostat that controls the working electrode potential and measures the current. The working electrode is the one on which the reaction of interest occurs, the oxidation or reduction of species. Therefore, the correct choice of the working electrode is vital for a successful application. Cost, electrical conductivity, chemical stability, activity towards the analyte, and wide potential range are the most important prerequisites that should be considered in choosing the electrode.
The high redox activity of POMs makes them ideal for the electrocatalytic transfer of electrons to or from a substrate while retaining their structural integrity. However, the application of ordinary POMs in chemically modified electrodes (CMEs) is not straightforward. Ordinary POMs-based CMEs present low stability due to POM’s high solubility in aqueous solutions. Practical applications of POMs for the preparation of CMEs depend on the successful immobilization of these compounds. The solution lies in fabricating organic-inorganic hybrid materials, allying the insolubility of organic compounds with the excellent catalytic properties of inorganic POMs.
Hydrogen peroxide (H2O2) is an oxidant widely used in food and pharmaceutical proceedings as a sterilizing agent and released from several industrial processes. In addition, H2O2 is the product of the reactions catalysed by many oxidases, which is fundamental in food, pharmaceuticals, and environmental analysis. H2O2 can be detected electrochemically, and its concentration can be readily monitored and used to measure the amount of a particular biological species (Ammam and Easton, 2012). To date, a significant number of enzyme-based electrochemical sensors have been developed to detect H2O2. However, the enzymatic biosensors are limited by the poor stability, high cost, complicated immobilization procedure, and critical operational conditions inherent to the nature of enzymes. Nevertheless, enzyme-free electrochemical sensors for H2O2 have gained special attention and have become a current trend. POM-based electrochemical sensors for H2O2 have been reported as appealing non-enzymatic alternatives due to their fast response and high sensitivities, achieved by exploring the synergetic effect of hybrid materials enhancing electrocatalytic activity and stability. Table 1 summarizes the electrochemical sensors for H2O2 found in the literature for the last 2 decades, using electrodes modified with POMs. Table 1 provides information about the POM-hybrid material used to modify the electrode (POM-hybrid@electrode), the archetypal of POM anions, the working pH, the limit of detection, the stability of the sensor and if the sensor was applied to real samples (more details can be found in Supplementary Table S1 in Supplementary Material). In Table 1, notice the work of Guo et al. (2015a), who reported a good example of a POM-modified electrode for H2O2 consisting of a nanocomposite film containing the Dawson K6P2W18O62 (P2W18), carbon nanotubes (CNTs), and Au nanoparticles (AuNPs), abbreviated as P2W18/CNTs/AuNPs, applied on an indium tin oxide (ITO) electrode (listed in Table 1 as P2W18/CNTs/AuNPs@ITO). The sensor showed a good linear range (1–98 mM), an excellent detection limit (52 nM), and a response time to H2O2 of less than 1s. Another good example of a non-enzymatic H2O2 sensor was reported by Berbéc et al. (Berbeć et al., 2018), and this one was based on a Keggin PMo12/AuNPs/reduced graphene oxide (rGO) over a glassy carbon electrode (GCE) and can be found in Table 1 under the designation of PMo12/AuNPs/rGO@GCE. Comparing the figures of merit of H2O2 analytical determination in Supplementary Table S1 with the two electrodes, it could be concluded that linear range and detection limits were similar, but in what concerns to sensitivity (listed in Supplementary Table S1, the Berbéc electrode was superior (sensitivity of 596.1 μA mM−1 cm−2 and 740.8 μA mM−1 cm−2, for Guo (Guo et al., 2015a) and Berbéc (Berbeć et al., 2018) electrodes, respectively) due to the rGO layer on the AuNPs/GC electrode, which significantly improved the sensitivity of AuNPs to H2O2. Nevertheless, both modified electrodes showed that the combination of carbon materials and AuNPs was responsible for significant enhancement of the sensor’s performance. Despite those figures of merit, those electrodes have a limitation related to the usual POMs instability at neutral pH, which constitutes a major drawback as they are restricted to work in acidic media, and therefore cannot be used in physiological systems.
Salimi et al. (2009) reported another type of POM-based hybrid composite that showed not only better performance but extended the pH working range a bit further. The new material was a combination of the Keggin SiMO12, single-walled carbon nanotubes (SWCNTs), and a cationic copper complex resulted in a three-component hybrid composite SWCNTs/SiMo12/[Cu(bpy)2]2+. Due to electrostatic attraction between anions and cations, the stability of adsorbed heteropolyanion and Cu-complex increased at somewhat higher pH values (pH < 7). Even so, authors performed the amperometric detection of H2O2 at pH 1, where the system (as represented in Table 1 SWCNTs/SiMo12/[Cu(bpy)2]2+@GCE) was able to detect nanomolar concentrations (1 nM), with values comparable or even better than other electrodes modified with Cu2+−complexes reported in the literature (Salimi et al., 2009). In addition, this system showed a linear range of 10 nM–18 mM, stability for 30 days, and proved to be successful towards bromate reduction (reported ahead in Table 3). Although less sensible (at μM level), other authors (Ammam and Easton, 2012; Zhang et al., 2015; Li et al., 2016; Zhang et al., 2019a; Liu et al., 2020a; Zhu et al., 2021; Cui et al., 2020; Wang et al., 2018a) achieved to improve electrochemical properties and stability at high pH (physiological level) by pairing POM anions (Keggin, Dawson, crown-shape and Preyssler) with organic or organometallic compounds (Ammam and Easton, 2012; Zhu et al., 2021), with carbon materials decorated with metal nanoparticles (Zhang et al., 2015; Li et al., 2016; Zhang et al., 2019a) or nitrogen (Liu et al., 2020a), and with metal-organic frameworks (MOFs) (Cui et al., 2020; Wang et al., 2018a), that enhanced POM stability at less acidic pH. It is worth mentioning that for the application of POM complexes in an aqueous solution, a thorough insight into the solution chemistry is essential in order to understand the reaction mechanism. The recent work from Gumerova and Rompel (Gumerova and Rompel, 2020) summarizes the species that are present in isopoly- and heteropolyvanadates, -niobates, -molybdates and -tungstates aqueous solutions and covers their stability and transformations, presenting ion distribution diagrams over a wide pH range. These diagrams showed the POM species that are in equilibrium at the given pH value and could help researchers to design the POM according to desired target. Nevertheless, among the sensors for H2O2 represented in Table 1, working at physiological levels (between pH 7.0 and 7.4), the one exhibiting the best performance was a modified glassy carbon rotating disk electrode (GCRDE) with a POM combined with a Nitrogen-doped mesoporous carbon (N-MPC) (Liu et al., 2020a). This [Mo-oxo]n/N-MPC@GCRDE (as listed in Table 1) modified electrode showed a good sensitivity of 2.2 mA mM−1 cm−2, a detection limit of 0.23 μM, a wide linear range from 50 μM to 5 mM, a response time of 2s, and excellent stability along 2 months. In addition, it was unaffected by many common contaminants. Besides, it is worth noting that among these sensors for H2O2 operating at physiological levels, the sensor based on K5 [Ru (bpy)3]-PW18@GCE, a combination of a Dawson type POM and an organometallic moiety, allowed the determination of H2O2 either by reduction or oxidation, displaying an attractive and rare bifunctional catalytic property.
Nitrite is commonly used as a food preservative and a fertilizing agent. It is widely present in the soil, water, food, and physiological systems and plays a role in the global nitrogen cycle. However, it is highly toxic because it interacts with amines to form carcinogenic nitrosamines in the stomach, prone to cause gastric cancer. Excessive nitrite in food products, including vegetables, drinking water, and beverages, is a severe threat to human health and has become a global issue, with the European Community stipulating guideline limits of 0.1 mg L−1 (∼2.2 mM) for drinking water (The European Parliament and the Council of the European Union, 2020). Analytical techniques used to detect nitrites were often spectrophotometry or spectrofluorimetry, and sometimes a chromatographic or electrophoresis separation is necessary. These techniques require somewhat bulky instrumentation, tedious and time-consuming sample pre-treatment, expensive reagents, or organic and toxic solvents. POM-based electrochemical sensors have been successfully used for nitrite detection due to their low cost, easy operation, and high sensitivity. Table 2 summarizes the POMs modified electrodes for NO2− found in the literature. Table 2 reveals detailed information about the POM-hybrid material used to modify the electrode (POM-hybrid@electrode), the POM archetype, the working pH, the limit of detection, the sensor lifetime, and the matrix where the sensor was tested (more details can be found in Supplementary Table S2 in Supplementary Material). It is noteworthy that the best performance was reported by Zuo et al. (2016), using an electrochemical sensor for nitrite based on a Keggin-type POM, H6 [PMo9V3O40] (PMo9V3), a poly (3,4-ethylenedioxythiophene) (PEDOT) and gold nanoparticles (AuNPs) fabricated by a combination of electrodeposition with self-assembly approach. Due to the synergistic contributions of POMs and PEDOT/AuNPs, the composite film electrode exhibited increased electrocatalytic activity towards the oxidation of nitrite and a faster transfer rate than the single-component film. The PEI/PMo9V3/PEDOT/AuNPs@GCE sensor (as listed in Table 2) showed a wide linear range (2.5 × 10−9–1.43 × 10−3 M) and a low detection limit of 1 nM, which are much better than most of the reported nitrite sensors (Zuo et al., 2016). Besides, the sensor presented a fast response time of 0.6 s and very good stability for long-term applications. Acceptable recoveries were obtained in a variety of samples (tap water, mineral water, apple juice, milk, and yoghurt) when spiking them with nitrite standards. Although many of the reported sensors were not tested in real samples, most of the nitrite Keggin or Dawson type POM-based sensors in contact with standards presented good stability and showed sensitivity and selectivity adequate to quantify nitrite at the established guideline values for drinking water.
Bromate is generally found in drinking water as a by-product of ozone disinfection, and it is widely used as a food additive for the maturation of flour and the production of fish paste and fermented beverages. Yet, bromate is a carcinogen. Iodine is an essential micronutrient, which is a crucial part of the thyroid hormones that play an essential role in the development of brain function and cell growth. Potassium iodate has been extensively used for the iodination of commercial table salts as a source of iodine. Deficiency or excess of iodine can cause serious health problems. These are examples of the importance of some of the ionic species (non-metallic oxides) listed in Table 3, and for which several electrochemical sensors based on POMs have been designed. Table 3 also includes information about the POM-hybrid materials used to modify the electrodes (POM-hybrid@electrode), the POM archetype and the figures of merit obtained with those electrodes when analysing standard solutions (more details can be found in Supplementary Table S3 in Supplementary Material). A small number of the reported studies include analysis of real samples. Again, the synergetic effect of the different combinations of POMs, carbon materials, organic compounds, ionic liquids, and metals allowed to improve the limits of detection of the CMEs.
The bifunctional sensor previously reported for H2O2 by Salimi et al. (2009), that used a combination of a Keggin SiMo12, SWCNTs, and a cationic copper complex (listed as SWCNTs/SiMo12/[Cu(bpy)2]2+@GCE in Table 3) did also present an excellent electrocatalytic activity towards the reduction of BrO3− at lower over-potential, due to the copper-complex that catalyses the reduction of bromate. Linear range, detection limit, and stability were 10–200 nM, 1.1 nM, and 30 days, respectively. Even so, no interference or validation studies with real samples were reported.
Sharifi et al. (2021) reported a modified GCE with a tetra-component nanocomposite consisting of a [1,10-(1,4-butanediyl) dipyridinium] ionic liquid (bdpy), the Keggin-type SiW11O39Ni(H2O) (abbreviated as SiW11Ni), and Phosphorus-doped electrochemically reduced graphene oxide (P-rGO), by electrodeposition technique for iodate determination. The presence of bdpy provided an additional advantage for increasing the loading of POM, improving the stability of the nanocomposite due to the strong electrostatic attraction between SiW11Ni and positively charged bdpy. The (bdpy)SiW11Ni/P-rGO@GCE sensor, as listed in Table 3, showed very good stability (1 month), good repeatability (200 cycles), and reproducibility. Furthermore, the modified electrode showed improved analytical figures of merit, such as low limit of detection (0.47 nM), high sensitivity (28.1 μA mM−1), good selectivity, and a wide linear range (10–1,600 μM−1), compared to other CMEs. It was validated by measuring IO3− in mineral and tap water and in a commercial edible iodized salt, proving that it could be efficiently applied to quantifying trace-level IO3− in real samples.
It is noteworthy to highlight another POM-based sensor listed in Table 3: a plastic electrode (PE) decorated with a tetrabutylammonium (TBA) derivative salt of octamolybdate, [N(C4H9)4]4Mo8O26, reported as MO8@PE and used for PO4− detection (Figueredo et al., 2021). This POM-based sensor, specially designed with TBA to be only soluble in organic solvents, achieved a remarkably LOD of 6.1 nM, better than the conventional analytical approaches reported by the authors. Plus, the standard spectrophotometric method used for PO43- detection takes 1 h for colour to develop. In contrast, this single-use MO8@PE sensor takes less than 5 min to show a result, and it was successfully applied in saline and seawater samples, being an affordable alternative for phosphate determination.
The excellent biocompatibility of POMs makes them extremely valuable in electrochemical biosensors, and POM-composite materials have been reported as highly successful electrocatalysts for the oxidation of biomolecules and bio-related species. Table 4 shows a list of POM-based electrodes used for a series of biomolecules, including the respective information about the POM-hybrid materials used to modify the electrodes (POM-hybrid@electrode), the POM archetype, and the figures of merit obtained with those electrodes when analysing standard solutions (more details can be found in Supplementary Table S4 in Supplementary Material). Among those biomolecules reported in Table 4, dopamine (DA) is a naturally occurring catecholamine that plays a very important role as a neurotransmitter in the mammalian central nervous system and plays a central role in Parkinson’s disease. For a healthy individual, the DA level lies in the range of 0.01–10 μM (Thakur et al., 2018). The easy electro-oxidation of DA turns electrochemical methods attractive. However, the coexistence of uric acid (UA) and a high concentration of ascorbic acid (AA) in the extracellular fluids of the central nervous system can cause significant interference because of its oxidation potentials which are close to that of DA on bare electrodes, resulting in poor selectivity. Accordingly, surface modification of electrodes with suitable electrocatalysts had been used to improve both the sensitivity and the selectivity of DA detection over AA and UA. Thakur et al. (2018) developed a novel POM-based sensor for highly selective and ultra-sensitive detection of DA, using a sandwich POM Na12 [WCo3(H2O)2(CoW9O34)2] (abbreviated as Co5POM) and poly (vinylimidazolium) cation [PVIM+] in combination with nitrogen-doped carbon nanotubes (N-CNTs), listed in Table 4 as PVIM−Co5POM/N-CNTs@GE. This combination provides the synergy between PVIM−POM catalyst and N-CNTs as conductive support, which enhances the electron transport at the electrode/electrolyte interface. Besides, it eliminates the interference of AA at physiological pH (7.4). The novel PVIM−Co5POM/N-CNTs composite achieved high selectivity and sensitivity, low detection limit (500 pM), and a wide linear working range of 0.0005–600 μM, even in the presence of a higher concentration of AA (500 μM), being one of the best catalysts reported so far for the selective electrochemical detection of DA. In an attempt to explore this sensor for practical applications, the PVIM−Co5POM/N-CNTs composite was analysed for the detection of DA in real sample using commercially available DA hydrochloride injections (40 mg ml−1) by standard addition method, and the recovery of the sample was in the range of 95–102%, which demonstrated the applicability of the composite for real-time analysis. The stability of the PVIM−Co5POM/N-CNTs composite was demonstrated during 100 cycles. Additionally, Zhou et al. (2013) reported a Dawson-type POM [P2W16V2O62]8- decorated by Au-Pd alloy NPs, applied onto ITO electrodes, by LbL self-assembly technique, used to simultaneously determine AA and DA at pH 7 (listed in Table 4 as P2W16V2/Au-PdNPs@ITO). AA is also an important biomolecule present in the mammalian brain, and it is a vital component in the human diet, used for the prevention and treatment of some diseases (common cold, mental illness, infertility, cancer, and AIDS). The sensing composite film exhibited high electrocatalytic activity towards the oxidation of AA and DA by decreasing the oxidation over-potentials and remarkably increasing the peak currents, attributed to the combining effect of P2W16V2 and Au-Pd in the composite film. DA and AA detection limits were 0.83 and 0.43 μM, respectively, and the sensor showed high selectivity and sensitivity. The sensor could be used to determine in real samples. However, the highest sensibility for AA was achieved by Ammam and Easton (Ammam and Easton, 2011b) with a hybrid material based on a 1-butyl-3-methylimidazolium tetrafluoroborate ionic liquid [(BMIM)(BF4)] and the Dawson-type ion [P2Mo18O62]6−, immobilized on glassy carbon electrode (GCE). The resulting AA sensor, [BMIM]6-P2Mo18@GCE as listed in Table 4, presented a significant sensitivity of ∼63 nA/μM to AA, a fast response time (<9 s), low detection limit (<0.1 μM), high selectivity towards endogenous interferences such as uric acid, acetaminophen and DA, a linear range from 0.1 μM to at least 22 mM, and was stable for at least 2 weeks. In addition, this AA sensor operated in a pH range from 0 to at least 7, which was attributed first to the presence of the ionic liquid cation in the hybrid material, and second to the porous morphology of the deposited film, which allowed a facile charge equilibrium within the film.
Glucose amperometric biosensors, based on the immobilization of the glucose oxidase enzyme (GOx) on POM-hybrid composites, have been reported in the literature (Turdean et al., 2002; Turdean and Popescu, 2012; Yang et al., 2016a; Boussema et al., 2018; Xu et al., 2017), and are listed in Table 4. However, the best sensor performance for glucose detection was achieved by Ayranci et al. (2019) with a non-enzymatic electrochemical sensor. The Keggin-type K7 [CoIIICoII(H2O)W11O39].15H2O, abbreviated as Co2W11, composed of unique mixed-valence Co(III) and Co(II) structures, was confined in a matrix of multi-walled carbon nanotubes (MWCNTs) on graphite electrodes (GEs) (listed in Table 4 as Co2W11/MWCNTs@GE). The proposed non-enzymatic sensor showed a wide linear range, from 0.1 to 10.0 mM of glucose. Besides, it exhibited a low detection limit of 1.21 μM, a fast response time of 6 s, high sensitivity (256.4 μA mM−1 cm−2), and good stability (5 weeks). These good results were explained by the authors with the improvement of electroactive surface area and the synergistic electrocatalytic activity resulting from the combination of Co-POM and MWCNTs.
Another successful non-enzymatic electrochemical sensor was reported by Thakur et al. (2019) for cholesterol. Cholesterol is an essential lipid of the human body and remains one of the most frequently analysed in clinical practice due to its association with various cardiovascular and brain disorders. The normal level of total cholesterol in blood serum is approx. 200 mg dl−1 and values higher than 240 mg dl−1 are responsible for damage of the arteries and diseases such as arteriosclerosis, heart diseases, hypertension, and cerebral thrombosis. Thakur (Thakur et al., 2019) proposed a non-enzymatic electrochemical sensor for cholesterol based on a sandwich POM [WCo3(H2O)2-(CoW9O34)2]12- (Co5POM) combined with poly (vinylbutylimidazolium) [PVIM+], which acted as a conductive matrix, simultaneously balancing the high negative charge (−12) of the POM. After, the PVIM–Co5POM conjugate was supported on nitrogen-rich mesoporous carbon (N-MPC) materials to enhance the activity. The modified graphite electrode (GE), listed in Table 4 as PVIM-Co5POM/N-MPC@GE, demonstrated high selectivity and sensitivity for cholesterol, with a wide detection range from 1 fM to 5 mM and a response time around 5 s. The linear response for cholesterol ranged from 1 fM to 200 nM, and sensitivity was 210 μA mM−1 cm−2. Moreover, interferent species, such as glucose, UA, and AA, showed no significant effect on cholesterol sensing. The sensor was applied for the quantitative analysis of cholesterol in human blood serum at physiological pH.
All these successful examples of non-enzymatic sensors for important biological species proved that the drawback of enzyme-based sensors, such as restricted immobilization and easy inactivation, can be overcome, and POM-hybrid materials may be precursors for producing non-enzymatic electrode materials in the coming years.
Furthermore, POM-based aptasensors, meaning biosensors that use aptamers as recognition elements, have been developed. Jia et al. (2020) reported a novel nanohybrid of polyoxometalate-derived MoS2 nanosheets (PMO12-MoS2 NSs) tightly and vertically grown over ß-FeOOH nanorods (NRs) that were exploited as platforms to immobilize the complementary DNA (cDNA) strands of microRNA-21 (miRNA) for further detection. Compared with other sensing systems referred by Jia et al. (2020), the PMO12-MoS2/β-FeOOH@Au modified electrode (as listed in Table 4) had superior sensing performance toward miRNA-21 with an incredible detection limit of 0.11 fM, a broad linear range from 1 fM to 5 nM, high selectivity, good stability (15 days), excellent reproducibility, and acceptable feasibility. Bao et al. (2020a) also reported a photoelectrochemical sensor based on the matrix FeOOH/Bi2S3/AuNPs and using the hedgehog-shape{Mo368} cluster as an electron donor for the ultrasensitive detection of cardiac troponin I (cTnI). Combined {Mo368}/FeOOH/Bi2S3/AuNPs with the specific recognition of antigen and antibody, a novel sensor based on a modified ITO, and listed in Table 4 as {Mo368}/FeOOH/Bi2S3/AuNPs@ITO, was constructed, showing a wide detection range of 1.00 pg ml−1–100 ng ml−1 and a low detection limit (0.76 pg ml−1). In general, the content of cTnI in normal human serum is below 0.2 ng ml−1, but direct damage to the myocardium occurs when the concentration of cTnI is higher than 2.0 ng ml−1. Therefore, the new sensor was able to detect cTnI at the early stages of cardiovascular disease. Despite the complex preparation procedures, these new strategies can open new routes for biosensing in clinical diagnosis by detecting other targets for which suitable probes (biomarkers) need to be anchored. Table 4 list POM-based electrochemical sensors for other biomolecules such as creatinine, bilirubin, xanthine, L-tyrosine, and L-tryptophan, among others. These sensors have in common to operate in the nM or pM range, and most of them were evaluated for stability and interferents and applied to real sample analysis, with reliable results, showing to be valuable alternatives to more costly and sophisticated analytical techniques.
Table 5 summarises the POM-based composite electrochemical sensors developed for medicines, pesticides, and toxic contaminants, listing the details about the POM-hybrid material used to modify the electrode (POM-hybrid@electrode), the POM archetype, working pH, the limit of detection, the stability of the sensor and information about tests with real samples (more details can be found in Supplementary Table S5 in Supplementary Material). Highlights go to an ultrasensitive electrochemical sensor for the selective measurement of trace ceftizoxime (CFX), proposed by Rouhani and Soleymanpour (Rouhani and Soleymanpour, 2021), using a thin film of Preyssler nanocapsules (PNCs) on pencil graphite electrode (PGE) surface modified with reduced graphene oxide (rGO). Under the optimized conditions, the PNCs/rGO@PGE sensor, as listed in Table 5, presents a wide linear concentration range, from 1.0 × 10−11 to 3.0 × 10−8 M, and an excellent detection limit of 1.8 pM. The outstanding electrochemical performance of the PNCs/rGO@PGE sensor was related to the synergistic influence of PNCs/r-GO/PGE thin film, which enhanced efficiency in drug encapsulation, stability, and effective surface area of the electrode for the CFX oxidation. The novel sensor showed better sensitivity than the earlier reported techniques for the CFX measurement, and it was successfully used to determine the trace amounts of CFX in pharmaceutical formulations and blood serum with suitable recoveries.
POM-based electrochemical sensors have been reported using molecularly imprinted polymers (MIPs) to attain the selectivity required to determine hazardous compounds. Besides the MIP, they have been combined with carbon materials and metal nanoparticles to overcome the restrictions in the conductivity of POMs. A MIP is a polymer that has been synthesized using a molecular imprinting technique with a mould molecule, leaving cavities in the polymer matrix with an affinity for that chosen mould molecule. POM hybrid materials combined with MIPs, mostly Keggin-type, have been reported for sildenafil (Rouhani and Soleymanpour, 2020), simazine (Ertan et al., 2016), ochratoxin A (Yola et al., 2016), and γ-lindane (Pelin Böke et al., 2020), and are listed in Table 5, all presenting excellent detection limits (10−11 M) and wide linear ranges. The sensors have been tested for common interferents and validated by testing their application in real sample analysis. Emphasis goes to POM/MIP-based electrochemical sensors for diazinon and citrinin, which proved to be able to determine these targets in food samples. Diazinon (DIA) is an organophosphorus pesticide and is considered very risky and harmful because of its noxious nature. Their recognition at ultra-trace levels in environmental samples and foodstuff is a serious analytical challenge. Medetalibeyoğlu et al. (Medetalibeyoğlu et al., 2020) reported the use of gold nanoparticles (AuNPs) incorporated Keggin-type POM/two-dimensional hexagonal boron nitride (2D-hBN) nanosheets and molecularly imprinted polymer (MIP) for the electrochemical detection of DIA molecule in fruit juice samples. The modified GCE electrode, listed as MIP/AuNPs/PW12/2D-hBN@GCE in Table 5, showed high selectivity and stability, and reliability for DIA detection. The linearity range and detection limit were 1.00 × 10−11 -1.00 × 10−8 M and 3.0 × 10−12 M, respectively, and sensitivity was better than other reported sensors (Medetalibeyoğlu et al., 2020). Another ultra-sensitive POM/MIP-based electrochemical sensor was reported for Citrinin (CIT). CIT is a toxic mutagenic and carcinogenic secondary metabolite of fungi, resistant to decomposition, and it is found in diverse food samples such as cheese, barley, red yeast rice, and apples. Atar et al. (2016) reported a molecular imprinted voltammetric sensor for CIT based on GCE modified with platinum nanoparticles (PtNPs), involving the Keggin polyoxometalate H3PW12O40 functionalized with reduced graphene oxide (rGO). The developed system, listed in Table 5 as MIP/PtNPs/PW12/rGO@GCE, showed a performance comparable to other CIT-imprinted sensors and conventional analytical methods. It is ultra-sensitive, with a detection limit of 2.0 × 10−13 M, rapid, easy, shows very good stability (45 days), and it might be preferred to other published methods.
Heavy metals have been early identified as primary environmental contaminants due to their non-biodegradability, bioaccumulation, and toxicity. Because of their harmful effects above the permissible limits, it is necessary to measure the concentration of these heavy metals to preserve the environment and health of individuals. Traditional methods for metal analysis include UV-Vis absorption spectrometry, surface-enhanced Raman spectrometry (SERS), atomic absorption spectrometry (AAS), atomic fluorescence spectrometry (AFS), ion chromatography (IC), inductively coupled plasma mass spectrometry (ICP-MS), and inductively coupled plasma optical emission spectrometry (ICP-OES). However, these techniques require expensive instruments, costly operations, and well-skilled operators to perform the multi-step sample preparation and complex analytical procedures, which are unsuitable for on-site and timely measurements necessary to monitor transient phenomena.
Hexavalent chromium (VI) is one of the most toxic heavy metal ions with high solubility in water. It has gained wide attention due to its high poisonousness and mutagenic-carcinogenic effects on human health. Therefore, the Cr(VI)-containing compounds were listed as human carcinogens by International Agency for Research on Cancer (IARC), and the World Health Organization (WHO) has established a maximum permissible concentration of total Cr(VI) in drinking water and industrial water of 0.05 and 0.5 ppm, respectively. POM-based electrochemical sensors were reported as alternatives for metal analysis, especially for chromium (VI), and are summarized in Table 6. Table 6 includes the respective information about the POM-hybrid materials used to modify the electrodes (POM-hybrid@electrode), the POM archetype, and the figures of merit obtained with those electrodes when analysing standard solutions (more details can be found in Supplementary Table S6 in Supplementary Material). Recently, Niu et al. (2021) reported the synthesis of two hourglass-type phosphomolybdate hybrids with different heterometallic centres, achieving an efficient electrochemical detection of ultra-trace Cr(VI) in wide pH ranges of 0–5. The reported sensors, {P4Mo6}/Cu/Mn/BBTZ@GCE (1) and {P4Mo6}/Na/Mn/BBTZ@GCE (2), at pH 0 displayed prominent sensitivities of 111.08 μA μM− 1 and 119.87 μA M−1, along with ultra-low detection limits towards Cr(VI) of 1.59 nM (0.17 ppb) and 2.91 nM (0.30 ppb), respectively, which fully satisfy the WHO standards for drinking water. The activity origin of both hybrids for impressive electrochemical behaviours was originated from the synergistic effect between reduced {P4Mo6} cluster and heterometallic centres at the molecular level. In the pH range of 1–5, good sensitivities and low detection limits (<25 nM) were also achieved by both sensors. Moreover, both were insensitive to common interferences and very stabile.
Cadmium (Cd) and lead (Pb) are used extensively in industry and are carcinogenic agents. Their accumulation in the human body can cause serious harm to internal organs such as lungs, kidneys, liver, bones, and central nervous system. Dianat et al. (Dianat et al., 2019) reported a novel sensitive L-cysteine Keggin tungstophosphate-modified polycrystalline gold electrode, listed in Table 6 as PW12/Cys@Au, developed for the electrochemical detection of Cd2+ and Pb2+ in trace amounts. The (Cys)PW12 hybrid compound was selected to fabricate inorganic self-assembled monolayers (SAMs) on the Au surface, which benefited from high stability due to the strong Au–S interaction. The modified electrode showed a wide linear range (0.01–0.2 μM) for both analytes, excellent reproducibility, high sensitivity, and reasonable detection limit (9.0 and 4.0 nM for Cd2+ and Pb2+, respectively), comparable to the other electrochemical techniques or modified gold electrodes (Dianat et al., 2019). The PW12/Cys@Au sensor was employed for Cd2+ or Pb2+ determination in industrial wastewater samples.
Optical sensors are a broad class of devices detecting light and producing an electrical output. The principle of an optical sensor is based on shifts in the characteristic optical signal of an optical platform, resulting from interactions with analyte molecules which are used for quantitative or qualitative measurements. Most optical sensors are based on light absorption. Reflectance measurements may be made in opaque mediums that interact with the analyte giving rise to a colour change, while scattering is the phenomenon observed when the direction and or frequency of light is changed upon interaction. Surface-enhanced Raman scattering and luminescence, including fluorescence and phosphorescence, are good scattering examples. Fluorescence deserves special emphasis due to its superior selectivity and sensitivity compared to the more common absorption phenomena. Chemiluminescence allows detecting an analyte after a chemical reaction yielding an electronically excited species that emit when returning to the ground state. Surface plasmon resonance is based on the increase of the intensity of the evanescent wave by the collective oscillation of the free-electron plasma at an insulator/metal surface after the adsorption of the analyte. Often, the angle of incidence of light is changed, and the intensity of reflected light is being measured while molecules are attaching the chemically modified surface.
POMs emerge as great promising species for absorption spectrophotometry, as accepting electrons gives rise to coloured mixed-valence state species while retaining their structural integrity. Table 7 lists the reported POMs-based absorption optical sensors, detailing the POM or the POM-hybrid composite, the POM archetype, the chromogenic substrate or reagents used, the working pH, the detection limit of the sensor, the sensor stability, and its application to real samples (more details can be found in Supplementary Table S7 in Supplementary Material).
“Heteropoly blues” are POMs of early transition metals [Mo(VI), W(VI) and V(V)] that exhibit a characteristic deep-blue colour after their reduction and have been reported for naked-eye colorimetric sensing. An example was the UV dosimeter indicator for solar water disinfection systems based on a Keggin [PW12O40]3- in the presence of a sacrificial electron donor, such as glycerol, that allowed the identification of the point at which microbiologically contaminated water was solar-disinfected. It had advantages over other reported methods, such as the POM-based indicator’s ability to recover colour overnight and its reusability (Lawrie et al., 2015). Another example was the reduction of the Keggin phosphomolybdic acid [H3 (PMo12O40)] by lactic acid (LA) in the presence of different UV radiations. Reducing PMo12 to varying extents allowed the development of a skin-specific personalized UV dosimeter for spectrally selective colorimetric differentiation of UVA, UVB, and UVC by the naked eye (Zou et al., 2018). In addition, L-cysteine-doped tungstosilicate (represented in Table 7 as Lcys-SiW12) microtubes have been used to detect ammoniac gas by a colour change from light purple to dark blue (Shen et al., 2012). The result was confirmed by the new absorption bands that appear at 500 and 750 nm, attributed to the d–d transition and the W(V)-W(VI) intervalence-charge transfer that occurred in the doped tungstosilicate microtubes. Plus, by doping the heteropolyoxometalate with the amino acid L-cysteine (C3H7NO2S) containing sulphydryl groups, which are essential in biological processes, the biocompatibility of the POM microtubes was improved.
“Heteropoly blues” have also been reported as inorganic building blocks for fabricating organic-inorganic hybrids to mimic peroxidase, followed by colour sensing. Peroxidase is an enzyme that can catalyse the transfer of two electrons from a substrate to hydrogen peroxide to generate water and an oxidized substrate, and it is widely used in biochemistry applications. The peroxidase-like activity of POMs has been reported to catalyse 3,3,5,5-tetramethylbenzidine (TMB) to its oxidized form, which has a blue colour and could be detected using UV–vis spectroscopy or be seen with the naked eye. Based on H2O2 detection in the TMB system, biomolecules that could generate H2O2 from their reaction with oxidases, such as glucose (Wang et al., 2012; Liu et al., 2012), dopamine, and ractopamine (Duan et al., 2018), had been indirectly detected through colorimetric assays, as listed in Table 7. In addition, by combining the synergetic effects of the peroxide-like activity of different phosphovanado-molybdate PVnMo12−nO40(3+n)- (n = 1–3) and folic acid (FA), Ji et al. (2015b) developed folate-functionalized hybrids (listed as FA-PVnMo12-n in Table 7), successfully used as an indicator for colorimetric immunoassay of the cancer cells, where the FA enhanced the biocompatibility and improved the target to tumour cells of FA-PMoVn hybrids. The authors also demonstrated that without the synergistic effect of FA, the phosphovanado-molybdates could not target the tumour cells for detection. Later, this work led to the development of a simple FA-PMo4V8 system that showed excellent peroxidase-like activity, which was employed to colorimetric detection of sarcosine, a possible biomarker in urine and blood that indicates the malignancy of prostate cancer cells (Mbage et al., 2020). Also, the combination of peroxide-like activity of POMs with metal-organic frameworks (POMOFs) has been reported for bioenzyme free colorimetric sensing. The colorimetric sensor was conceived based on a POMOF, the [Ni4(Trz)6(H2O)2][SiW12O40].4H2O (Trz:1,2,4-triazole), and polydiallyldimethylammonium (PDDA) chloride functionalized reduced graphene oxide (PDDA-rGO). In the nanocomposite, listed as Ni4(Trz)/SiW12/PDDA-rGO (Tong et al., 2020) in Table 7, PDDA acted as a bridging agent to loading rGO nanosheet on the surface of Keggin [SiW12O40]4- which offered excellent catalytic activities under extreme conditions (pH value 2.5), due to the nature and synergies from POMs, MOFs, and PDDA-rGOs. This bi-functional nanocomposite allowed the successful establishment of a platform for colorimetric sensing of H2O2 and citric acid (CA), with higher sensitivity (1–60 μM), fast response (10 min), and lower detection limit (2.07 μM) to CA than all other materials reported by the authors (Tong et al., 2020).
An exceptional ratiometric photoacoustic imaging (PAI) nanoprobe for glutathione (GSH), which plays important roles in a variety of diseases and cellular functions, was successfully achieved by Tang et al. (2019) through the self-assembly of croconaine (CR) dye and molybdenum-based polyoxometalate cluster into uniform nanoparticles, represented in Table 7 as Mo-based POM/CR. The authors discovered that the CR dye could be reduced specifically by GSH, showing a distinct GSH concentration-dependent decrease in the absorbance at 700 nm. In contrast, the Mo-based POM clusters were reduced by the GSH, increasing their absorbance at 866 nm due to the GSH-activated Mo(VI) to Mo(V) conversion. Thus, the photoacoustic (PA) signal ratio of CR-POM at these two wavelengths (PA866/PA700) was much higher than most existing ratiometric PAI probes. Furthermore, the relatively low LOD (0.51 mM) and the linear range up to 14 mM, revealed the capability of Mo-based POM/CR for GSH quantification, which covered exactly the range of the GSH concentration in vivo (0.5–10 mM), which was highly competent for noninvasive quantification of GSH in vivo.
However, not only molecules with biological relevance have been successfully detected by POM-based colorimetric sensors. High toxic metals and food contaminants have also deserved the researcher’s attention. A metal-oxo cluster, (n-Bu4N)2 [Mo5NaO13(OCH3)4(NO)], organically-derivatized from a monolacunary Lindqvist (ML)-type polyoxomolybdate, listed as MLPOM in Table 7, was reported to specifically react with Hg2+ in methanol, displaying a colour change from purple to brown within seconds, after mixing, with a detection limit of 0.05 μM, which was below the guideline value of Hg2+ for contaminated sewage from mercury industries (Chen et al., 2015b). By comparing the structure of polyoxomolybdate, before and after reaction, the colour change was revealed to be caused by the structural transformation of MLPOM accelerated by Hg2+. Additionally, the developed POM-based colorimetric sensor showed a remarkably high selectivity over other environmentally relevant metal ions, such as Fe2+, Fe3+, Cr3+, Zn2+, Pb2+, Ni2+, Ag+, Al3+, Mn2+, Cd2+, Ca2+, Co2+, and Cu2+.
Furthermore, a Keggin POM-based optical sensor for ZnCl2.2H2O, working both in solution as in solid-state, based on [Himi]4 [SiMo12O40] (imi = imidazole), was recently reported by Sabarinathan et al. (2021), where the Molybdenum blue (reduced Mo5+) appeared only in the presence of ZnCl2.2H2O which confirmed the involvement of water molecules in the reduction mechanism. This POM-based sensor, listed as imi-SiMo12 in Table 7, achieved a much lower LOD (0.15 μM) than results published in the literature and a superior selectivity over metal chloride solutions of 15 different metals and other salts of Zn2+. Besides, the imidazole ring possesses potent antimicrobial activity against multiple pathogenic microbes, and in particular against Staphylococcus aureus.
An optical fibre sensor, based on a cladding stripped tip coated with a Keggin-type [(C4H9)4N]4H [PMo10V2O40], specially designed to be insoluble in water, incorporated into a plasticized polyvinylchloride (PVC) membrane containing o-nitrophenyl octyl ether (NPOE), was reported for formaldehyde detection in milk (Veríssimo et al., 2020a). The UV–Vis spectrum of the POM-coating membrane, listed in Table 7 as PMo12V2/PVC/NPOE, changed with formaldehyde. The LOD for formaldehyde determined with the optical sensor was 0.2 mg L−1, similar to the value of the conventional acetylacetone spectrophotometric method, though the limit of quantification (LOQ) was slightly lower for the spectrophotometric method, 0.5 mg L−1 and 0.6 mg L−1, respectively. In addition, the described methodology has the advantage of not requiring a heating step, one of the disadvantages of the conventional acetylacetone spectrophotometric method, which prevents its use in the field.
Fluorescence readouts are particularly interesting for very sensitive sensing applications. The changes in the intensity of light emitted at longer wavelengths than the excitation can be quantitatively related to the concentration of the analyte. Rare-earth (RE)-based materials are often used to manufacture fluorescence (FL) chemosensors because RE ions with rich electron energy levels show outstanding luminous properties under light excitation. Therefore, the combination of RE and POMs, that provide numberless oxo-active sites to capture RE ions, appears as the ideal combination.
Table 8 summarizes the Lanthanide (Ln)-based materials (Ln-POMs-based fluorescence sensors) reported so far in the literature. Table 8 includes the POM archetype, the detection limit, the operation mode of the sensors and the matrix where sensors were tested (more details can be found in Supplementary Table S8 in Supplementary Material). Among Ln-POMs reported probes in Table 8, the Lindqvist europium decatungstate Na9 [EuW10O36].32H2O (EuW10) was the most prevalent due to Eu fascinating property of changing colour and fluorescence dependence on its valency and coordination environment. However, as pure EuW10 exhibited only weak photoluminescence in water, due to luminescence quenching of water molecules, quenching in solution must be prevented by self-assembly of Eu-POM with organic materials, such as polymers, metal-organic frameworks (MOFs) and surfactants.
Self-assembly of Eu-POM with polymers was reported by Wang et al. (2010). A highly transparent flexible self-supporting decatungsteuropate thin film, listed in Table 8 as EuW10/agarose, was fabricated by a facile hydrogel casting technique. The strong interactions between agarose and EuW10 by hydrogen bonds at the hydroxyl sites and the densely packed 3D network structure of agarose in the gel contributed to the homogenous distribution of EuW10 and to the good mechanical properties of the nanocomposite films. When excited with UV-light, the thin-films of EuW10/agarose displayed a strong red emission of Eu3+ that can be reversibly modulated, quenched by HCl gas, and recovered by NH3 gas, behaving as a luminescent switch. Also a TbW10/agarose composite thin film reported more recently by Bin et al. (Wang et al., 2019a), showed the same behaviour in the presence of HCl and NH3 gases, with the green luminescent thin-film sensor presenting a detection limit of 0.2731 mmol L−1 for HCl, showing that other Ln-POM (Dutta and Sarkar, 2016), besides Eu-POMs, could be used for sensing.
The self-assembly of Eu-POMs with 3D coordination networks (MOFs) also appears as a promising approach due to their crystalline nature, permanent porosity, chemical tunability, and robustness, offering an advantageous unique platform for the development of solid-state luminescent materials. Thus, POMs incorporated in the cavities of a metal-organic framework (POM/MOFs) have been used to prevent luminescence quenching. Salomon et al. (2018) reported the introduction of the luminescent EuW10 into the cavities of highly porous zirconium luminescent MOF UiO-67, combining dual-luminescent properties of EuW10/UiO-67, as listed in Table 8. The hybrid material proved to be a solid-state luminescent sensor for amino acids. Enhancement of the EuW10/UiO-67 luminescence is observed in the presence of amino acids globally following the increase of the amino-acid pKa. Due to the strong quenching effect of Fe3+ (KSV 2667 M−1), the EuW10/UiO-67 proved to be also a reusable sensor for Fe3+ in an aqueous solution, with an estimated LOD of 37 µM. Recently, the same group reported the encapsulation of the EuW10 into a mesoporous MOF, a Tb-TATB, built of terbium tetranuclear units connected by TATB ligands (H3TATB = triazine-1,3,5-tribenzoic acid). The dual-luminescent EuW10/Tb-TATB composite (as listed in Table 8) behaved like a highly sensitive luminescent thermometer in the physiological domain and gave rise to a new family of hybrid dual-emitting LnPOM/LnMOF materials (Viravaux et al., 2021).
An example of surfactant-encapsulated polyoxometalates was reported by Hui et al. (Zhang et al., 2006), where the luminescent polyoxometaloeuropate EuW10 was connected through electrostatic interaction with the multi-functional surfactant, trans-10-[4-(4′-pyridylvinylene)-phenyl] oxydecyldodecyldimethyl-ammonium bromide (PyC10C12N). The combined composite, listed as EuW10/PyC10C12N in Table 8, worked as a luminescent logic gate with dual output, operated by light and zinc ion as inputs. Another example was reported by Panpan et al. (Sun et al., 2019), where the polyoxometaloeuropate was used to develop a sensitive, selective off-luminescence chemical sensor, listed as EuW10/[C14-2-C14im] Br2 in Table 8, for the label-free detection of Cr3+ and MnO4− in aqueous solution, with low detection limits of 0.926 and 1.70 μM, respectively, and a wide pH application range. The introduction of [C14-2-C14im]Br2 did increase the luminescence effect, and the strongest luminescence was observed for EuW10/[C14-2-C14im]Br2, which was 32 times that of pure EuW10.
Although the luminescent EuW10 dominate in the Ln-POM based fluorescence optical sensors group, other luminescent Eu-POMs have been reported (Table 8). Two systems, a penta-EuIII sandwiched Dawson-type selenotungstate (Zhang et al., 2020a) [H2N(CH3)2]10H3{SeO4Eu5(H2O)8 [Se2W14O52]2}·40H2O, represented in Table 8 as EuSe3W14, and a polyhydroxycarboxylic acid ligand bridged multi-EuIII-incorporating tellurotungstate (Zhang et al., 2020b) K14H10 [Eu4(H2O)4W6(H2glu)4O12(B-α-TeW9O33)4]·60H2O (H6glu = D-gluconic acid), represented in Table 8 as EuTeW9, were reported as sensors to detect Cu2+ ions in aqueous solution. Both systems exhibited high fluorescence signals and good selectivity for detecting Cu2+ ions in an aqueous solution (Zhang et al., 2020a; Zhang et al., 2020b). The Eu-tellurotungstate showed the best performance due to the hexagonal packing of the tetrameric polyoxoanions, providing excellent porous channels, which greatly increased the specific surface area of the whole framework and fluorescence sensing. This is the most sensitive POM-based fluorescence sensor for detecting Cu2+ ions in an aqueous solution reported so far (LOD 8.82 × 10−6 mM). Furthermore, the same sensors could be used in Cu2+−quenching systems. These “off-on” fluorescence sensors were used to detect Cysteine (Cys) in an aqueous solution with similar LODs (2.17 × 10−4 and 1.75 × 10−4 mM, with EuSe3W14 and EuTeW9, respectively).
Besides Ln-substituted POMs, other luminescent hybrid POMs have been reported by binding chromophore species to POMs, which are summarized in Table 9, along with the POM archetype, their target analyte and the matrix where they were tested, the operation mode of the sensor and the detection limit (LOD) (more details can be found in Supplementary Table S9 in Supplementary Material).
Carraro et al. (2012) reported a bis-lacunary Keggin polyoxotungstate [γ-SiW10O36]8– as a molecular nanosurface where the dansyl chromophore was anchored with a tweezer-type arrangement, which acted as a selective fluorescence sensor for Cu2+ and Pb2+ ions, in quenching and enhancing mode, respectively. Hong et al. (Liu et al., 2015) reported the use of Norfloxacin, a known fluorescence medicine, to produce a Norfloxacin-derivative functionalized octamolybdate, (dNF)2 [γ-Mo8O26(dNF)2].10H2O, where dNF stands for decarboxylated norfloxacin. The combination, listed as SiW10/dansyl in Table 9, showed to be an acid-base switch system, as both the addition of acid or base modulated its fluorescence. Another simple ionic association of a photoluminescent compound, the [IrIII(ppy)2 (bpy)]+ complex incorporating 2-phenyl- pyridine (ppy) and 2,20-bipyridine diimine (bpy), with an octamolybdate result in a strong modulation of its emission wavelength in the solid-state, varying from green to yellow, orange, orange-red, and red, by changing the nature of the POM and the design of the frameworks. The resulting hybrid materials, listed as Mo8/[IrIII(ppy)2 (bpy)]+ in Table 9, turned to be an efficient selective chemosensor for VOC detection (Bolle et al., 2016).
Furthermore, Tian et al. (Tian et al., 2019) reported the synergetic combination of organic species such as benzoic acid (BA), thiamine (TH), and 3-(4-hydroxyphenyl)propionic acid (HPPA), with the Keggin-type polyoxotungstate intrinsic peroxidase Na10 [α-SiW9O34] decomposing H2O2 into ▪OH radicals, which converted weakly fluorescent substrates to strongly fluorescent substrates, under basic pH conditions. Recently, S-/N-containing ligands, such as 2,2′-dimethyl-4,4′-bithiazole (Ying et al., 2019; Wang et al., 2020b) and 2,5-bis(4-pyridyl)thiazolo [5,4-d] thiazole (Mou et al., 2020), and N-containing ligands, such as 2,2′-bipyridyl (bpy) and 4-(3-imidazol-1-yl-ethyl)-4H-[1,3,4]triazole (MET) (Zhang et al., 2021a) were used to modify a series of different archetype POM compounds, in order to build fluorescence sensors for Hg2+. All compounds showed selective response to Hg2+, explained by the preferred interaction between the soft acidic Hg2+ ions and sulphur (soft base). In addition, all hybrid POMs proved to be multi-functional materials, showing not only photocatalytic activity for degradation of dyes (Ying et al., 2019; Wang et al., 2020b; Mou et al., 2020; Zhang et al., 2021a) but also redox properties, which makes them probe to act as electrochemical sensors for NO2− (Ying et al., 2019; Mou et al., 2020; Wang et al., 2020b; Zhang et al., 2021a), for H2O2 (Zhang et al., 2021), and Cr(VI) (Wang et al., 2020b).
Hybrid metal-POMs showing fluorescence properties and applied to sensing have also been reported. The terminal and bridging oxygen atoms on the surface of POMs not only can act as versatile proton acceptors and donors but can also coordinate with other metal ions. Mukul et al. (Raizada et al., 2017) synthesized a decavanadate hybrid material with 4-picoline N-oxide (Pyno) and triethylamine (NEt3), the {Cu(Pyno)4}{NEt3H}2 [H2V10O28] cluster, denoted in Table 9 as V10O28-Cu-pyno-NEt, in which the metal ion linkers belong to distinct coordination complexes with peripheral organic ligands. This water-soluble inorganic-hybrid compound was investigated as the first aqueous-phase sensor for picric acid and Pd2+, with a low detection limit of 0.18 and 0.80 ppb, respectively, within WHO/US EPA prescribed limit for palladium. Another example was recently reported by Qian et al. (Li et al., 2021), where the Anderson type (NH4)3 [H6Fe(III)Mo6O24] (FeMo6), working as an oxidase-mimic nanoenzyme, exhibited the ability to catalytic oxidase of o-phenylenediamine (OPD), 2,2′-azino-bis(3-ethylbenzthiazoline-6-sulfonic acid) (ABTs), and 3,3′,5,5′-tetramethylbenzidine (TMB). The proposed sensor based on two consecutive “turn on” fluorescence was developed for DA by employing the FeMo6-OPD system, and the linear range was from 1 to 100 μM with the detection limit 0.0227 μM. In addition, by loading the FeMo6-OPD system with 10% of reduced graphene oxide (rGO), listed in Table 9 as FeMo6/rGO, the authors increased the oxidase-mimic activity of FeMo6, with an enhancement of the detection limit to 0.012 μM.
While several POM-based fluorescence sensors and POM-based absorbance optical sensors for UV-Vis spectroscopy could be found in the literature, only one paper reported the use of a POM in a reduced graphene oxide (rGO)/Ag film as a surface-enhanced Raman scattering probe for the selective detection of trace formaldehyde in the presence of other aldehydes (Zhang et al., 2017a). The use of the Keggin H3PW12O40 (PW12) as a photoreduction agent helped to improve the reduction degree of the GO. Compared with surface-enhanced Raman scattering probes prepared with the PW12/rGO film and the PW12/Ag film, the PW12/rGO/Ag film displayed a higher sensitivity and the detection limit for formaldehyde reached 1.0 × 10−8 M.
Piezoelectric crystals, like quartz, vibrate with the application of an oscillating electric potential. The acoustic wave propagates on the bulk of the crystal, but a change of mass at the surface of the crystal changes the frequency of oscillation. Other mass-sensitive sensors are based on the launch of a surface acoustic wave from a transmitter consisting of interdigitated electrodes that travel on the surface of the piezoelectric material to another interdigitated set of electrodes, the receiver. These surface acoustic wave devices operate at much higher frequencies but are not as popular as the bulk acoustic wave devices (BAW), also known as quartz crystal microbalances (QCM) when used as gravimetric sensors.
QCM is a powerful technique to study the dynamics of adsorption processes. It was used to monitor organic-inorganic hybrid films growth by recording the quartz crystal’s frequency decrease during each adsorption cycle. QCM sensors were used to study the adsorption of Keggin phosphotungstic acid POM onto a copolymer-coated QCM as a function of time at several pH conditions (Raj et al., 2015). Also, the organic-inorganic hybrid polyoxometalate (NBu4)3 [PW11O39{(SiC6H4NH2)2O}], carrying two amine functions, allowed the construction of an ordered array of amine groups on the sensor surface for benzo [a]pirene detection. The ordered surface enabled better accessibility of the immobilized molecules compared with a reference layer built from an amine-terminated self-assembled monolayer on gold and, consequently, a significant increase in biosensor sensitivity (Mercier et al., 2015). However, papers that use a QCM coated with a POM (POM@QCM), where the POM is the recognition element, are very scarce. Veríssimo et al. (Veríssimo et al., 2017) reported the use of an acoustic wave sensor coated with a sensitive layer of a Keggin-type decamolybdodivanadophosphate (α-[PMo10V2O40]5-), that has been previously reported as an effective redox catalyst for volatile organic compound (VOCs) oxidation (Gamelas et al., 2012). This PMo10V2@QCM sensor was used to detect 5-hydroxymethylfurfural (HMF), a potentially mutagenic, carcinogenic and genotoxic compound, an excellent indicator of honey ageing, poor storage conditions, excessive heat-treatment, or possible adulteration with other sugars or syrups. Sensor lifetime was at least 6 weeks without sensitivity loss, and the quantification limit was well below the legislation threshold of 11.4 μg g−1 for HMF in honey. Another POM@QCM sensor was also published by Veríssimo et al. (2018), where the sensitive coating was a POM salt specially tailored to be insoluble in water. The Keggin-type polyoxotungstate, with tetrabutylammonium (TBA) as counter-cation, [(C4H9)4N]4 [PW11MnIII(H2O)O39], was used as the sensitive membrane of the piezoelectric quartz crystal for acetaldehyde quantification in cider. Results were not statistically different from those obtained with Gas Chromatography-Flame ionization Detection (GC-FID), and LOD and LOQ were similar. The sensor was stable for at least 8 weeks.
Looking at the vast list of applications summarized in this review, a first conclusion emerges that POM-based composites used for sensing applications are mainly based on Keggin [(XM12O40)n−]- and Wells-Dawson [(X2M18O62)n−]-type structures, with Keggin-type being responsible for more than 60% of the listed POMs in Table 1, Table 2, Table 3, Table 4, Table 5, Table 6, Table 7, Table 8, and Table 9. Listed POMs were combined with the most diverse materials, such as metals, polymers, carbon-based materials, and porous framework materials, such as metal-organic frameworks, zeolites and molecular imprinted polymers. Figure 5 schematically summarizes POM hybrid structures used for sensing. These POMs’ modifications enhanced redox, conductive and catalytic properties, included chromophores to enhance optical signals, changed material shape or built cavities with particular geometries and chemical functional groups. Besides, the careful choice of the counter-ion imparts the desired insolubility, preventing the leaching of the sensor sensitive layer.
POM-based electrochemical sensors provided low-cost and straightforward systems competing with expensive and sophisticated technologies, with detection limits for most applications as low as micromolar, with some special applications reaching pM and fM. The additions of POMs to solid electrodes is a result of their multielectron redox properties, giving rise to fast and sensitive responses. POM-hybrid materials used for CMEs were the most diverse. The highlight goes to those combined with nanocarbon materials or metal nanoparticles, which undoubtedly enhanced stability and improved electrochemical performance. Furthermore, the immobilization of specific DNA strands, or the combination with molecularly imprinted polymers, largely influenced the sensor’s sensitivity and selectivity.
POM-based optical sensors were reported for various targets, from metals to biomolecules. Concerning the POM-based absorption optical sensors, which were mainly Keggin-type structures, the standing out goes to the “heteropoly blues”, which acted as inorganic building blocks and were used to fabricate organic-inorganic hybrids to mimic peroxidase with success. Regarding the POM-based fluorescence sensors, those with Eu-POM, have recently been in the spotlight, due to their ability to act as efficient photoswitches or very selective fluorescent probes. Anyway, it is worth highlighting the bifunctionality of most optical sensors based on POM, an asset that allows the sensing of multiple analytes.
POM-based mass sensors, although less explored, also have a say in the world of POM functionalized sensors. Besides sensing for quantitative analysis, these piezoelectric sensors could be used for adsorption studies with great effectiveness. Furthermore, one of the advantages of QCM detection lays on their applicability both in gaseous and liquid media, which may spark new interest in this line of research in the coming years.
This overview of the literature concerning functionalized POM sensors revealed that, in general, the analytical properties of the proposed sensors are significantly better than others previously reported, based on other types of compounds. The design of POM-hybrid materials conceived having in mind the final target, and considering the most appropriate transducer for each application, allowed the development of POM-based sensors with extremely low limits of detection (pM and fM) in line with more sophisticated and expensive analytical techniques. Still, researchers do not always address critical issues such as selectivity, nor do they validate sensors by applying them to real samples.
It is expected that, in the future, POM hybrid materials contribution to the sensing area will increase, with different organic-inorganic hybrid materials providing different coordination modes to construct more specific structures, which will potentially exhibit enhanced performances. Besides, it can be envisaged that POM-based sensors future trends will rely on multi-functional nanomaterials with multi-stimuli responsive materials, contributing to a new era of smart sensors. The possibility to interrogate a POM platform in multiple ways, combining different transducers is an interesting possibility, still waiting for the pioneers.
MV wrote the manuscript, MG added a few paragraphs detailing or introducing some ideas, and both MG and DE critically discussed and revised the paper. All authors have read and agreed with the final version of the paper.
Authors are grateful to FCT/MCTES for the financial support of CESAM (UIDP/50017/2020 + UIDB/50017/2020 + LA/P/0094/2020) and CICECO-Aveiro Institute of Materials (UIDB/50011/2020 + UIDP/50011/2020 + LA/P/0006/2020) through national funds. MV is funded by national funds (OE), through FCT—Fundação para a Ciência e a Tecnologia, I.P., in the scope of the framework contract foreseen in the numbers 4, 5 and 6 of the article 23, of the Decree-Law 57/2016, of August 29, changed by Law 57/2017, of July 19.
The authors declare that the research was conducted in the absence of any commercial or financial relationships that could be construed as a potential conflict of interest.
All claims expressed in this article are solely those of the authors and do not necessarily represent those of their affiliated organizations, or those of the publisher, the editors and the reviewers. Any product that may be evaluated in this article, or claim that may be made by its manufacturer, is not guaranteed or endorsed by the publisher.
The Supplementary Material for this article can be found online at: https://www.frontiersin.org/articles/10.3389/fchem.2022.840657/full#supplementary-material
Ammam, M., and Easton, E. B. (2011). Advanced NOx Gas Sensing Based on Novel Hybrid Organic-Inorganic Semiconducting Nanomaterial Formed between Pyrrole and Dawson Type Polyoxoanion [P2Mo18O62]6−. J. Mater. Chem. 21, 7886–7891. doi:10.1039/c1jm11244a
Ammam, M., and Easton, E. B. (2012). Novel Organic-Inorganic Hybrid Material Based on tris(2,2′-bipyridyl)Dichlororuthenium(II) Hexahydrate and Dawson-type Tungstophosphate K7[H4PW18O62]·18H2O as a Bifuctional Hydrogen Peroxide Electrocatalyst for Biosensors. Sensors Actuators B: Chem. 161, 520–527. doi:10.1016/j.snb.2011.10.070
Ammam, M., and Easton, E. B. (2011). Selective Determination of Ascorbic Acid with a Novel Hybrid Material Based 1-Butyl-3-Methylimidazolium Tetrafluoroborate Ionic Liquid and the Dawson Type Ion [P2Mo18O62]6− Immobilized on Glassy Carbon. Electrochimica Acta 56, 2847–2855. doi:10.1016/j.electacta.2010.12.072
Ammam, M. (2013). Polyoxometalates: Formation, Structures, Principal Properties, Main Deposition Methods and Application in Sensing. J. Mater. Chem. A. 1, 6291–6312. doi:10.1039/c3ta01663c
Arefian, M., Mirzaei, M., Eshtiagh-hosseini, H., and Frontera, A. (2017). A Survey of the Different Roles of Polyoxometalates in Their Interaction with Amino Acids, Peptides and Proteins. Dalton Trans. 46, 6812–6829. doi:10.1039/c7dt00894e
Atar, N., Yola, M. L., and Eren, T. (2016). Sensitive Determination of Citrinin Based on Molecular Imprinted Electrochemical Sensor. Appl. Surf. Sci. 362, 315–322. doi:10.1016/j.apsusc.2015.11.222
Aureliano, M., Gumerova, N. I., Sciortino, G., Garribba, E., Rompel, A., and Crans, D. C. (2021). Polyoxovanadates with Emerging Biomedical Activities. Coord. Chem. Rev. 447, 214143. doi:10.1016/j.ccr.2021.214143
Ayranci, R., Torlak, Y., and Ak, M. (2019). Non-Enzymatic Electrochemical Detection of Glucose by Mixed-Valence Cobalt Containing Keggin Polyoxometalate/Multi-Walled Carbon Nanotube Composite. J. Electrochem. Soc. 166, B205–B211. doi:10.1149/2.0581904jes
Ayranci, R., Torlak, Y., Soganci, T., and Ak, M. (2018). Trilacunary Keggin Type Polyoxometalate-Conducting Polymer Composites for Amperometric Glucose Detection. J. Electrochem. Soc. 165, B638–B643. doi:10.1149/2.1061813jes
Babakhanian, A., Kaki, S., Ahmadi, M., Ehzari, H., and Pashabadi, A. (2014). Development of α-polyoxometalate-polypyrrole-Au Nanoparticles Modified Sensor Applied for Detection of Folic Acid. Biosens. Bioelectron. 60, 185–190. doi:10.1016/j.bios.2014.03.058
Bao, C., Liu, X., Shao, X., Ren, X., Zhang, Y., Sun, X., et al. (2020). Cardiac Troponin I Photoelectrochemical Sensor: {Mo368} as Electrode Donor for Bi2S3 and Au Co-sensitized FeOOH Composite. Biosens. Bioelectron. 157, 112157. doi:10.1016/j.bios.2020.112157
Bao, Y., Li, Z., Wang, H., Li, N., Pan, Q., Li, J., et al. (2020). Electrochemical Reduction-Assisted In Situ Fabrication of a Graphene/Au Nanoparticles@polyoxometalate Nanohybrid Film: High-Performance Electrochemical Detection for Uric Acid. Langmuir 36, 7365–7374. doi:10.1021/acs.langmuir.0c00893
Berbeć, S., Żołądek, S., Jabłońska, A., and Pałys, B. (2018). Sensors Actuators, B Chem. 258, 745–756.
Bezdek, M. J., Luo, S.-X. L., Ku, K. H., and Swager, T. M. (2021). A Chemiresistive Methane Sensor. Proc. Natl. Acad. Sci. USA 118, e2022515118–6. doi:10.1073/pnas.2022515118
Bijelic, A., Aureliano, M., and Rompel, A. (2019). Polyoxometalates as Potential Next‐Generation Metallodrugs in the Combat against Cancer. Angew. Chem. Int. Ed. 58, 2980–2999. doi:10.1002/anie.201803868
Bijelic, A., and Rompel, A. (2018). Polyoxometalates: More Than a Phasing Tool in Protein Crystallography. ChemTexts 4, 1–27. doi:10.1007/s40828-018-0064-1
Bin, W., Xiaohong, W., Limei, D., Liang, X., Peng, Z., Jinpeng, J., et al. (2019). Chem. J. Chin. Univ. 40, 676–684.
Bolle, P., Serier-Brault, H., Génois, R., Faulques, E., Boulmier, A., Oms, O., et al. (2016). Drastic Solid-State Luminescence Color Tuning of an Archetypal Ir(iii) Complex Using Polyoxometalates and its Application as a Vapoluminescence Chemosensor. J. Mater. Chem. C 4, 11392–11395. doi:10.1039/c6tc04341k
Boussema, F., Gross, A. J., Hmida, F., Ayed, B., Majdoub, H., Cosnier, S., et al. (2018). Dawson-type Polyoxometalate Nanoclusters Confined in a Carbon Nanotube Matrix as Efficient Redox Mediators for Enzymatic Glucose Biofuel Cell Anodes and Glucose Biosensors. Biosens. Bioelectron. 109, 20–26. doi:10.1016/j.bios.2018.02.060
Boussema, F., Haddad, R., Ghandour, Y., Belkhiria, M. S., Holzinger, M., Maaref, A., et al. (2016). Polyoxometalate [PMo11O39]7−/carbon Nanocomposites for Sensitive Amperometric Detection of Nitrite. Electrochimica Acta 222, 402–408. doi:10.1016/j.electacta.2016.10.192
Cao, F., Guo, S., Ma, H., and Gong, J. (2012). ITO Electrode Modified by α-K6[P2W18O62] Hybrid Nanofibers for Nitrite Determination. Electroanalysis 24, 418–424. doi:10.1002/elan.201100613
Cao, L., Sun, H., Li, J., and Lu, L. (2011). An Enhanced Electrochemical Platform Based on Graphene-Polyoxometalate Nanomaterials for Sensitive Determination of Diphenolic Compounds. Anal. Methods 3, 1587–1594. doi:10.1039/c1ay05121k
Carraro, M., Modugno, G., Fiorani, G., MacCato, C., Sartorel, A., and Bonchio, M. (2012). Organic-Inorganic Molecular Nano-Sensors: A Bis-Dansylated Tweezer-like Fluoroionophore Integrating a Polyoxometalate Core. Eur. J. Org. Chem. 2012, 281–289. doi:10.1002/ejoc.201101122
Chen, C., Song, Y., and Wang, L. (2009). Electrochemical Behavior and its Electrocatalytic Properties of Chemically Modified Electrode with Keggin-type [SiNi(H2O)W11O39]6−. Electrochimica Acta 54, 1607–1611. doi:10.1016/j.electacta.2008.09.044
Chen, J.-J., Symes, M. D., Fan, S.-C., Zheng, M.-S., Miras, H. N., Dong, Q.-F., et al. (2015). High-Performance Polyoxometalate-Based Cathode Materials for Rechargeable Lithium-Ion Batteries. Adv. Mater. 27, 4649–4654. doi:10.1002/adma.201501088
Chen, K., She, S., Zhang, J., Bayaguud, A., and Wei, Y. (2015). Label-free Colorimetric Detection of Mercury via Hg2+ Ions-Accelerated Structural Transformation of Nanoscale Metal-Oxo Clusters. Sci. Rep. 5, 1–9. doi:10.1038/srep16316
Cherevan, A. S., Nandan, S. P., Roger, I., Liu, R., Streb, C., and Eder, D. (2020). Polyoxometalates on Functional Substrates: Concepts, Synergies, and Future Perspectives. Adv. Sci. 7, 1903511. doi:10.1002/advs.201903511
Cui, L., Fu, Y., Liu, L., Jiang, J., Ding, Y., and Chen, L. (2021). Alkali Metal-Lanthanide Co-encapsulated 19-Tungsto-2-Selenate Derivative and its Electrochemical Detection of Uric Acid. Inorg. Chem. Commun. 130, 108734. doi:10.1016/j.inoche.2021.108734
Cui, L., Yu, K., Lv, J., Guo, C., and Zhou, B. (2020). A 3D POMOF Based on a {AsW12} Cluster and a Ag-MOF with Interpenetrating Channels for Large-Capacity Aqueous Asymmetric Supercapacitors and Highly Selective Biosensors for the Detection of Hydrogen Peroxide. J. Mater. Chem. A. 8, 22918–22928. doi:10.1039/d0ta08759a
Dehnavi, A., and Soleymanpour, A. (2020). Highly Sensitive Voltammetric Electrode for the Trace Measurement of Methyldopa Based on a Pencil Graphite Modified with Phosphomolibdate/graphene Oxide. Microchemical J. 157, 104969. doi:10.1016/j.microc.2020.104969
Dianat, S., Hatefi-Mehrjardi, A., Mahmoodzadeh, K., and Kakhki, S. (2019). Electrocatalytic Determination of Cd2+ and Pb2+ Using an L-Cysteine Tungstophosphate Self-Assembled Monolayer on a Polycrystalline Gold Electrode. New J. Chem. 43, 14417–14425. doi:10.1039/c9nj03459e
Dong, P., Li, N., Zhao, H., Cui, M., Zhang, C., Han, H., et al. (2019). POMs as Active Center for Sensitively Electrochemical Detection of Bisphenol A and Acetaminophen. Chem. Res. Chin. Univ. 35, 592–597. doi:10.1007/s40242-019-8370-8
Duan, X., Bai, Z., Shao, X., Xu, J., Yan, N., Shi, J., et al. (2018). Fabrication of Metal-Substituted Polyoxometalates for Colorimetric Detection of Dopamine and Ractopamine. Materials 11, 674. doi:10.3390/ma11050674
Dutta, T., and Sarkar, S. (2016). Nanocarbon-{[Na10(PrW10O36)]2·130H2O} Composite to Detect Toxic Food Coloring Dyes at Nanolevel. Appl. Nanosci. 6, 1191–1197. doi:10.1007/s13204-016-0529-8
Ensafi, A. A., Gorgabi-Khorzoughi, M., Rezaei, B., and Jafari-Asl, M. (2017). Electrochemical Behavior of Polyoxometalates Decorated on Poly Diallyl Dimethyl Ammonium Chloride-MWCNTs: A Highly Selective Electrochemical Sensor for Determination of Guanine and Adenine. J. Taiwan Inst. Chem. Eng. 78, 56–64. doi:10.1016/j.jtice.2017.06.001
Ertan, B., Eren, T., Ermiş, İ., Saral, H., Atar, N., and Yola, M. L. (2016). Sensitive Analysis of Simazine Based on Platinum Nanoparticles on Polyoxometalate/multi-Walled Carbon Nanotubes. J. Colloid Interf. Sci. 470, 14–21. doi:10.1016/j.jcis.2016.02.036
Feizy, S., and Haghighi, B. (2019). A Survey on the Effect of Ionic Liquid on Electrochemical Behavior and Electrocatalytic Activity of a Phosphomolybdic Acid-Ionic Liquid-MWCNT-Modified Glassy Carbon Electrode. J. Solid State. Electrochem. 23, 1339–1350. doi:10.1007/s10008-019-04228-2
Fernandes, D. M., Nunes, M., Bachiller-Baeza, B., Rodríguez-Ramos, I., Guerrero-Ruiz, A., Delerue-Matos, C., et al. (2017). PMo11V@N-CNT Electrochemical Properties and its Application as Electrochemical Sensor for Determination of Acetaminophen. J. Solid State. Electrochem. 21, 1059–1068. doi:10.1007/s10008-016-3463-5
Figueredo, F., Girolametti, F., Aneggi, E., Lekka, M., Annibaldi, A., and Susmel, S. (2021). Plastic Electrode Decorated with Polyhedral Anion Tetrabutylammonium Octamolybdate [N(C4H9)4]4 Mo8O26 for nM Phosphate Electrochemical Detection. Analytica Chim. Acta 1161, 338469. doi:10.1016/j.aca.2021.338469
Fu, Z., Gao, W., Yu, T., and Bi, L. (2019). Study of Bi-directional Detection for Ascorbic Acid and Sodium Nitrite Based on Eu-Containing Luminescent Polyoxometalate. Talanta 195, 463–471. doi:10.1016/j.talanta.2018.11.091
Gamelas, J. A. F., Evtyugina, M. G., Portugal, I., and Evtuguin, D. V. (2012). New Polyoxometalate-Functionalized Cellulosic Fibre/silica Hybrids for Environmental Applications. RSC Adv. 2, 831–839. doi:10.1039/c1ra00371b
Gamelas, S., Gomes, A., Moura, N., Faustino, M., Cavaleiro, J., Lodeiro, C., et al. (2018). N-confused Porphyrin Immobilized on Solid Supports: Synthesis and Metal Ions Sensing Efficacy. Molecules 23, 867–882. doi:10.3390/molecules23040867
Gao, J., Gong, L., Fan, X., Yu, K., Zheng, Z., and Zhou, B. (2020). {P2W18O62}-Encapsulated Potassium-Ion Nanotubes Intercalated in Copper Biimidazole Frameworks for Supercapacitors and Hydrogen Peroxide Sensing. ACS Appl. Nano Mater. 3, 1497–1507. doi:10.1021/acsanm.9b02312
Gao, J., Yang, T., Wang, X., He, Q., He, P., Jia, L., et al. (2020). Spherical Phosphomolybdic Acid Immobilized on Graphene Oxide Nanosheets as an Efficient Electrochemical Sensor for Detection of Diphenylamine. Microchemical J. 158, 105158. doi:10.1016/j.microc.2020.105158
Gao, Y., Yao, S., Gong, J., and Qu, L. (2007). Preparation of Polyaniline Nanotubes via "Thin Glass Tubes Template" Approach and its Gas Response. Macromol. Rapid Commun. 28, 286–291. doi:10.1002/marc.200600672
Gong, L.-G., Qi, X.-X., Yu, K., Gao, J.-Q., Zhou, B.-B., and Yang, G.-Y. (2020). Covalent Conductive Polymer Chain and Organic Ligand Ethylenediamine Modified MXene-Like-{AlW12O40} Compounds for Fully Symmetric Supercapacitors, Electrochemical Sensors and Photocatalysis Mechanisms. J. Mater. Chem. A. 8, 5709–5720. doi:10.1039/c9ta14103k
Guedes, G., Wang, S., Santos, H. A., and Sousa, F. L. (2020). Polyoxometalate Composites in Cancer Therapy and Diagnostics. Eur. J. Inorg. Chem. 2020, 2121–2132. doi:10.1002/ejic.202000066
Gumerova, N. I., and Rompel, A. (2020). Polyoxometalates in Solution: Speciation under Spotlight. Chem. Soc. Rev. 49, 7568–7601. doi:10.1039/d0cs00392a
Guo, S., Xu, L., Xu, B., Sun, Z., and Wang, L. (2015). A Ternary Nanocomposite Electrode of Polyoxometalate/carbon Nanotubes/gold Nanoparticles for Electrochemical Detection of Hydrogen Peroxide. Analyst 140, 820–826. doi:10.1039/c4an01734j
Guo, W., Cao, X., Liu, Y., Tong, X., and Qu, X. (2014). Electrochemical-Reduction-Assisted Fabrication of a Polyoxometalate/Graphene Composite Film Electrode and its Electrocatalytic Performance. J. Electrochem. Soc. 161, B248–B255. doi:10.1149/2.0101412jes
Guo, W., Ma, J., Cao, X., Tong, X., Liu, F., and Liu, Y. (2020). A Silicomolybdate/graphene/poly(3,4-Ethylenedioxythiophene) Composite Film as a Sensor for Sensitive Determination of Persulfate. Int. J. Electrochem. Sci. 15, 915–928. doi:10.20964/2020.01.84
Guo, W., Tong, X., and Liu, S. (2015). Polyoxometalate/chitosan-electrochemically Reduced Graphene Oxide as Effective Mediating Systems for Electrocatalytic Reduction of Persulfate. Electrochimica Acta 173, 540–550. doi:10.1016/j.electacta.2015.05.097
Haghighi, B., Hamidi, H., and Gorton, L. (2010). Formation of a Robust and Stable Film Comprising Ionic Liquid and Polyoxometalate on Glassy Carbon Electrode Modified with Multiwalled Carbon Nanotubes: Toward Sensitive and Fast Detection of Hydrogen Peroxide and Iodate. Electrochimica Acta 55, 4750–4757. doi:10.1016/j.electacta.2010.03.041
Hamidi, H., Shams, E., Yadollahi, B., and Esfahani, F. K. (2009). Fabrication of Carbon Paste Electrode Containing [PFeW11O39]4− Polyoxoanion Supported on Modified Amorphous Silica Gel and its Electrocatalytic Activity for H2O2 Reduction. Electrochimica Acta 54, 3495–3500. doi:10.1016/j.electacta.2008.12.063
Han, H., Sha, J., Liu, C., Wang, Y., Dong, C., Li, M., et al. (2021). Polyoxometalate-based Catenane as Sensing Material for Electrochemical Detection of Dopamine. J. Coord. Chem. 74, 1781–1793. doi:10.1080/00958972.2021.1944120
Hao, Y., Feng, S., Liu, Y., Xu, J., Ma, Y., and Wang, J. (2017). Electrochemical Sensor Based on Indium Tin Oxide Glass Modified with Poly(Ethyleneimine)/Phosphomolybdic Acid Composite Multilayers. Electroanalysis 29, 1188–1196. doi:10.1002/elan.201600672
Hatami, E., Ashraf, N., and Arbab-Zavar, M. H. (2021). Construction of β-Cyclodextrin-phosphomolybdate Grafted Polypyrrole Composite: Application as a Disposable Electrochemical Sensor for Detection of Propylparaben. Microchemical J. 168, 106451. doi:10.1016/j.microc.2021.106451
Herrmann, S., Ritchie, C., and Streb, C. (2015). Polyoxometalate - Conductive Polymer Composites for Energy Conversion, Energy Storage and Nanostructured Sensors. Dalton Trans. 44, 7092–7104. doi:10.1039/c4dt03763d
Ho, W. H., Chen, T.-Y., Otake, K.-i., Chen, Y.-C., Wang, Y.-S., Li, J.-H., et al. (2020). Polyoxometalate Adsorbed in a Metal-Organic Framework for Electrocatalytic Dopamine Oxidation. Chem. Commun. 56, 11763–11766. doi:10.1039/d0cc04904b
Hutin, M., Rosnes, M. H., Long, D.-L., and Cronin, L. (2013). Polyoxometalates: Synthesis and Structure - from Building Blocks to Emergent Materials. Elsevier 2, 241–269. doi:10.1016/b978-0-08-097774-4.00210-2
Ji, H., Zhu, L., Liang, D., Liu, Y., Cai, L., Zhang, S., et al. (2009). Use of a 12-Molybdovanadate(V) Modified Ionic Liquid Carbon Paste Electrode as a Bifunctional Electrochemical Sensor. Electrochimica Acta 54, 7429–7434. doi:10.1016/j.electacta.2009.07.076
Ji, Y., Huang, L., Hu, J., Streb, C., and Song, Y.-F. (2015). Polyoxometalate-functionalized Nanocarbon Materials for Energy Conversion, Energy Storage and Sensor Systems. Energy Environ. Sci. 8, 776–789. doi:10.1039/c4ee03749a
Ji, Y., Xu, J., Chen, X., Han, L., Wang, X., Chai, F., et al. (2015). Inorganic-bimolecular Hybrids Based on Polyoxometalates: Intrinsic Oxidase Catalytic Activity and Their Application to Cancer Immunoassay. Sensors Actuators B: Chem. 208, 497–504. doi:10.1016/j.snb.2014.11.058
Jia, Q., Huang, S., Hu, M., Song, Y., Wang, M., Zhang, Z., et al. (2020). Polyoxometalate-derived MoS2 Nanosheets Embedded Around Iron-Hydroxide Nanorods as the Platform for Sensitively Determining miRNA-21. Sensors Actuators B: Chem. 323, 128647. doi:10.1016/j.snb.2020.128647
Jiang, J., Liu, L., Liu, G., Wang, D., Zhang, Y., Chen, L., et al. (2020). Organic-Inorganic Hybrid Cerium-Encapsulated Selenotungstate Including Three Building Blocks and its Electrochemical Detection of Dopamine and Paracetamol. Inorg. Chem. 59, 15355–15364. doi:10.1021/acs.inorgchem.0c02318
Jiao, J., Zuo, J., Pang, H., Tan, L., Chen, T., and Ma, H. (2018). A Dopamine Electrochemical Sensor Based on Pd-Pt alloy Nanoparticles Decorated Polyoxometalate and Multiwalled Carbon Nanotubes. J. Electroanalytical Chem. 827, 103–111. doi:10.1016/j.jelechem.2018.09.014
Kakhki, S., and Shams, E. (2013). A New Bifunctional Electrochemical Sensor for Oxidation of Cysteine and Reduction of Iodate. J. Electroanalytical Chem. 704, 249–254. doi:10.1016/j.jelechem.2013.01.017
Kakhki, S., Shams, E., and Barsan, M. M. (2013). Electrocatalytic Oxidation of Cysteine at a CoSalophen/n-(butyl)4SiW12O40Carbon Paste Electrode. Electroanalysis 25, 2100–2108. doi:10.1002/elan.201300235
Kang, L., Ma, H., Yu, Y., Pang, H., Song, Y., and Zhang, D. (2013). Study on Amperometric Sensing Performance of a crown-shaped Phosphotungstate-Based Multilayer Film. Sensors Actuators B: Chem. 177, 270–278. doi:10.1016/j.snb.2012.10.126
Karimi-Maleh, H., Karimi, F., Malekmohammadi, S., Zakariae, N., Esmaeili, R., Rostamnia, S., et al. (2020). An Amplified Voltammetric Sensor Based on Platinum Nanoparticle/polyoxometalate/two-Dimensional Hexagonal boron Nitride Nanosheets Composite and Ionic Liquid for Determination of N-Hydroxysuccinimide in Water Samples. J. Mol. Liquids 310, 113185. doi:10.1016/j.molliq.2020.113185
Karimi-Takallo, A., Dianat, S., and Hatefi-Mehrjardi, A. (2021). Fabrication and Electrochemical Study of K(1,1′- (1,4 Butanediyl)dipyridinium)2[PW11O39Co(H2O)]/MWCNTs-COOH Nanohybrid Immobilized on Glassy Carbon for Electrocatalytic Detection of Nitrite. J. Electroanalytical Chem. 886, 115139. doi:10.1016/j.jelechem.2021.115139
Katsoulis, D. E. (1998). A Survey of Applications of Polyoxometalates. Chem. Rev. 98, 359–388. doi:10.1021/cr960398a
Khalilpour, H., Shafiee, P., Darbandi, A., Yusuf, M., Mahmoudi, S., Moazzami Goudarzi, Z., et al. (2021). Application of Polyoxometalate-Based Composites for Sensor Systems: A Review. jcc 3, 129–139. doi:10.52547/jcc.3.2.6
Lawrie, K., Mills, A., Figueredo-Fernández, M., Gutiérrez-Alfaro, S., Manzano, M., and Saladin, M. (2015). UV Dosimetry for Solar Water Disinfection (SODIS) Carried Out in Different Plastic Bottles and Bags. Sensors Actuators B: Chem. 208, 608–615. doi:10.1016/j.snb.2014.11.031
Li, B., Zhang, T., Wang, H., Zhao, X., Li, F., Liu, M., et al. (2016). Polyoxometalates-mediated Facile Synthesis of Pt Nanoparticles Anchored on an Ordered Mesoporous Carbon for Electrochemical Applications. RSC Adv. 6, 93469–93475. doi:10.1039/c6ra19995j
Li, H., Gong, P., Jiang, J., Li, Y., Pang, J., Chen, L., et al. (2019). Organic-inorganic Hybrids Assembled from Plenary Keggin-type Germanotungstate Units and 3d-4f Heterometal Clusters. Dalton Trans. 48, 3730–3742. doi:10.1039/c9dt00312f
Li, Q., Tian, A., Chen, C., Jiao, T., Wang, T., Zhu, S., et al. (2021). Anderson Polyoxometalates with Intrinsic Oxidase-Mimic Activity for "turn on" Fluorescence Sensing of Dopamine. Anal. Bioanal. Chem. 413, 4255–4265. doi:10.1007/s00216-021-03376-7
Li, S., Ma, H., O’Halloran, K. P., Pang, H., Ji, H., and Zhou, C. (2013). Enhancing Characteristics of a Composite Film by Combination of Vanadium-Substituted Molybdophosphate and Platinum Nanoparticles for an Electrochemical Sensor. Electrochimica Acta 108, 717–726. doi:10.1016/j.electacta.2013.07.033
Li, Y., Yang, X., Yang, F., Wang, Y., Zheng, P., and Liu, X. (2012). Effective Immobilization of Ru(bpy)32+ by Functional Composite Phosphomolybdic Acid Anion on an Electrode Surface for Solid-State Electrochemiluminescene to Sensitive Determination of NADH. Electrochimica Acta 66, 188–192. doi:10.1016/j.electacta.2012.01.087
Li, Z., Chen, J., Pan, D., Tao, W., Nie, L., and Yao, S. (2006). A Sensitive Amperometric Bromate Sensor Based on Multi-Walled Carbon Nanotubes/phosphomolybdic Acid Composite Film. Electrochimica Acta 51, 4255–4261. doi:10.1016/j.electacta.2005.12.004
Liang, Y., He, P., Ma, Y., Zhou, Y., Pei, C., and Li, X. (2009). A Novel Bacterial Cellulose-Based Carbon Paste Electrode and its Polyoxometalate-Modified Properties. Electrochemistry Commun. 11, 1018–1021. doi:10.1016/j.elecom.2009.03.001
Liu, C., Xu, M., Tan, Z., Li, S., Wang, Y., Wang, Y., et al. (2020). Assembly of Wells-Dawson Polyoxometalate Based Crystal Compound for Uric Acid Electrochemical Detection. Z. Anorg. Allg. Chem. 646, 489–494. doi:10.1002/zaac.202000167
Liu, H., Chai, D.-F., Zou, Y.-L., Zhou, S.-J., Wang, W., Shen, D.-F., et al. (2015). Norfloxacin-derivative Functionalized Octamolybdate: Unusual Carbonyl Coordination and Acidity Sensitive Luminescence. RSC Adv. 5, 40688–40691. doi:10.1039/c5ra04108b
Liu, H., Lv, Y., Li, S., Yang, F., Liu, S., Wang, C., et al. (2017). A Solar Ultraviolet Sensor Based on Fluorescent Polyoxometalate and Viologen. J. Mater. Chem. C 5, 9383–9388. doi:10.1039/c7tc01263b
Liu, L., Jiang, J., Liu, X., Liu, G., Wang, D., Chen, L., et al. (2020). First Series of Mixed (PIII, SeIV)-Heteroatomoriented Rare-Earth-Embedded Polyoxotungstates Containing Distinct Building Blocks. Inorg. Chem. Front. 7, 4640–4651. doi:10.1039/d0qi01031f
Liu, Q.-Q., Wang, X.-L., Lin, H.-Y., Chang, Z.-H., Zhang, Y.-C., Tian, Y., et al. (2021). Two New Polyoxometalate-Based Metal-Organic Complexes for the Detection of Trace Cr(vi) and Their Capacitor Performance. Dalton Trans. 50, 9450–9456. doi:10.1039/d1dt01247a
Liu, R., Luo, Y., Zheng, Y., Zhang, G., and Streb, C. (2020). Polyoxometalate-like Sub-nanometer Molybdenum(vi)-Oxo Clusters for Sensitive, Selective and Stable H2O2 Sensing. Chem. Commun. 56, 9465–9468. doi:10.1039/d0cc03758c
Liu, S., Tian, J., Wang, L., Zhang, Y., Luo, Y., Li, H., et al. (2012). Fast and Sensitive Colorimetric Detection of H2O2 and Glucose: A Strategy Based on Polyoxometalate Clusters. Chempluschem 77, 541–544. doi:10.1002/cplu.201200051
Long, D.-L., Tsunashima, R., and Cronin, L. (2010). Polyoxometalates: Building Blocks for Functional Nanoscale Systems. Angew. Chem. Int. Ed. 49, 1736–1758. doi:10.1002/anie.200902483
Ma, H., Shi, S., Zhang, Z., Pang, H., and Zhang, Y. (2010). An Thin Film of the Di-decatungstosilicate with a tetra-Ruthenium(IV)-oxo Core and its Electrochemical Properties. J. Electroanalytical Chem. 648, 128–133. doi:10.1016/j.jelechem.2010.08.003
Ma, H., Zhang, Z., Pang, H., Li, S., Chen, Y., and Zhang, W. (2012). Fabrication and Electrochemical Sensing Property of a Composite Film Based on a Polyoxometalate and Palladium Nanoparticles. Electrochimica Acta 69, 379–383. doi:10.1016/j.electacta.2012.03.017
Ma, Z., Qiu, Y., Yang, H., Huang, Y., Liu, J., Lu, Y., et al. (2015). Effective Synergistic Effect of Dipeptide-Polyoxometalate-Graphene Oxide Ternary Hybrid Materials on Peroxidase-like Mimics with Enhanced Performance. ACS Appl. Mater. Inter. 7, 22036–22045. doi:10.1021/acsami.5b07046
Mbage, B., Li, Y., Si, H., Zhang, X., Li, Y., Wang, X., et al. (2020). Fabrication of Folate Functionalized Polyoxometalate Nanoparticle to Simultaneously Detect H2O2 and Sarcosine in Colorimetry. Sensors Actuators B: Chem. 304, 127429–9. doi:10.1016/j.snb.2019.127429
Medetalibeyoğlu, H., Beytur, M., Manap, S., Karaman, C., Kardaş, F., Akyıldırım, O., et al. (2020). ECS J. Solid State. Sci. Technol. 9, 101006.
Mercier, D., Ben Haddada, M., Huebner, M., Knopp, D., Niessner, R., Salmain, M., et al. (2015). Polyoxometalate Nanostructured Gold Surfaces for Sensitive Biosensing of Benzo[a]pyrene. Sensors Actuators B: Chem. 209, 770–774. doi:10.1016/j.snb.2014.12.015
Miao, J., Chen, Y., Li, Y., Cheng, J., Wu, Q., Ng, K. W., et al. (2018). Proton Conducting Polyoxometalate/Polypyrrole Films and Their Humidity Sensing Performance. ACS Appl. Nano Mater. 1, 564–571. doi:10.1021/acsanm.7b00072
Miras, H. N., Vilà-Nadal, L., and Cronin, L. (2014). Polyoxometalate Based Open-Frameworks (POM-OFs). Chem. Soc. Rev. 43, 5679–5699. doi:10.1039/c4cs00097h
Miras, H. N., Yan, J., Long, D.-L., and Cronin, L. (2012). Engineering Polyoxometalates with Emergent Properties. Chem. Soc. Rev. 41, 7403–7430. doi:10.1039/c2cs35190k
Mou, H.-c., Ying, J., Tian, A.-x., Cui, H.-t., and Wang, X.-l. (2020). Four Keggin-Based Compounds Constructed by a Series of Pyridine Derivatives: Synthesis, and Electrochemical, Photocatalytic and Fluorescence Sensing Properties. New J. Chem. 44, 15122–15130. doi:10.1039/d0nj00103a
Mou, H., Liu, G., Jia, X., Tian, A., Yang, M., Fu, Y., et al. (2019). Three Keggin-Templated Compounds Constructed by Flexible Ligands: Syntheses and Electrochemical Properties. J. Coord. Chem. 72, 2968–2981. doi:10.1080/00958972.2019.1683828
Niu, J.-Q., An, W.-T., Zhang, X.-J., Ma, Y.-Y., and Han, Z.-G. (2021). Ultra-trace Determination of Hexavalent Chromium in a Wide pH Range Triggered by Heterometallic Cu-Mn Centers Modified Reduced Phosphomolybdate Hybrids. Chem. Eng. J. 418, 129408. doi:10.1016/j.cej.2021.129408
Oghli, A. H., and Soleymanpour, A. (2020). Polyoxometalate/reduced Graphene Oxide Modified Pencil Graphite Sensor for the Electrochemical Trace Determination of Paroxetine in Biological and Pharmaceutical media. Mater. Sci. Eng. C 108, 110407. doi:10.1016/j.msec.2019.110407
Ortiz, M., Debela, A. M., Svobodova, M., Thorimbert, S., Lesage, D., Cole, R. B., et al. (2017). PCR Incorporation of Polyoxometalate Modified Deoxynucleotide Triphosphates and Their Application in Molecular Electrochemical Sensing of Yersinia pestis. Chem. Eur. J. 23, 10597–10603. doi:10.1002/chem.201701295
Patel, A., Narkhede, N., Singh, S., and Pathan, S. (2016). Keggin-type Lacunary and Transition Metal Substituted Polyoxometalates as Heterogeneous Catalysts: A Recent Progress. Catal. Rev. 58, 337–370. doi:10.1080/01614940.2016.1171606
Pelin Böke, C., Karaman, O., Medetalibeyoglu, H., Karaman, C., Atar, N., and Lütfi Yola, M. (2020). A New Approach for Electrochemical Detection of Organochlorine Compound Lindane: Development of Molecular Imprinting Polymer with Polyoxometalate/carbon Nitride Nanotubes Composite and Validation. Microchemical J. 157, 105012. doi:10.1016/j.microc.2020.105012
Pope, M. T., and Müller, A. (1991). Polyoxometalate Chemistry: An Old Field with New Dimensions in Several Disciplines. Angew. Chem. Int. Ed. Engl. 30, 34–48. doi:10.1002/anie.199100341
Qi, L., Wu, W., Kang, Q., Hu, Q., and Yu, L. (2020). Detection of Organophosphorus Pesticides with Liquid Crystals Supported on the Surface Deposited with Polyoxometalate-Based Acetylcholinesterase-Responsive Supramolecular Spheres. Food Chem. 320, 126683. doi:10.1016/j.foodchem.2020.126683
Qian, J., Wang, K., Jin, Y., Yang, X., Jiang, L., Yan, Y., et al. (2014). Polyoxometalate@magnetic Graphene as Versatile Immobilization Matrix of Ru(bpy)32+ for Sensitive Magneto-Controlled Electrochemiluminescence Sensor and its Application in Biosensing. Biosens. Bioelectron. 57, 149–156. doi:10.1016/j.bios.2014.02.005
Raizada, M., Sama, F., Ashafaq, M., Shahid, M., Ahmad, M., and Siddiqi, Z. A. (2017). New Hybrid Polyoxovanadate-Cu Complex with V⋯H Interactions and Dual Aqueous-phase Sensing Properties for Picric Acid and Pd2+: X-ray Analysis, Magnetic and Theoretical Studies, and Mechanistic Insights into the Hybrid's Sensing Capabilities. J. Mater. Chem. C 5, 9315–9330. doi:10.1039/c7tc03172f
Raj, G., Swalus, C., Delcroix, M., Devillers, M., Dupont-Gillain, C., and Gaigneaux, E. M. (2015). In Situ quartz crystal Microbalance Monitoring of the Adsorption of Polyoxometalate on a Polyampholyte Polymer Matrix. J. Colloid Interf. Sci. 445, 24–30. doi:10.1016/j.jcis.2014.12.035
Ren, Y., Wang, M., Chen, X., Yue, B., and He, H. (2015). Heterogeneous Catalysis of Polyoxometalate Based Organic-Inorganic Hybrids. Materials 8, 1545–1567. doi:10.3390/ma8041545
Ross, N., and Civilized Nqakala, N. (2020). Electrochemical Determination of Hydrogen Peroxide by a Nonenzymatic Catalytically Enhanced Silver-Iron (III) Oxide/Polyoxometalate/Reduced Graphene Oxide Modified Glassy Carbon Electrode. Anal. Lett. 53, 2445–2464. doi:10.1080/00032719.2020.1745223
Rouhani, M., and Soleymanpour, A. (2020). Molecularly Imprinted Sol-Gel Electrochemical Sensor for Sildenafil Based on a Pencil Graphite Electrode Modified by Preyssler Heteropolyacid/gold Nanoparticles/MWCNT Nanocomposite. Microchim. Acta 187, 512. doi:10.1007/s00604-020-04482-6
Rouhani, M., and Soleymanpour, A. (2021). Ultrasensitive Electrochemical Determination of Trace Ceftizoxime Using a Thin Film of Preyssler Nanocapsules on Pencil Graphite Electrode Surface Modified with Reduced Graphene Oxide. Microchemical J. 165, 106160. doi:10.1016/j.microc.2021.106160
Sabarinathan, C., Karthikeyan, M., Murugappan, R. M., Anthony, S. P., Shankar, B., Parthasarathy, K., et al. (2021). Polyoxometalate Based Ionic crystal: Dual Applications in Selective Colorimetric Sensor for Hydrated ZnCl2 and Antimicrobial Activity. New J. Chem. 45, 5576–5588. doi:10.1039/d1nj00138h
Sadakane, M., and Steckhan, E. (1998). Electrochemical Properties of Polyoxometalates as Electrocatalysts. Chem. Rev. 98, 219–238. doi:10.1021/cr960403a
Sahraoui, Y., Sbartai, A., Chaliaa, S., Maaref, A., Haddad, A., and Jaffrezic-Renault, N. (2015). A Nitrite Electrochemical Sensor Based on Boron-Doped Diamond Planar Electrochemical Microcells Modified with a Monolacunary Silicotungstate Polyoxoanion. Electroanalysis 27, 1359–1367. doi:10.1002/elan.201400682
Salimi, A., Korani, A., Hallaj, R., Khoshnavazi, R., and Hadadzadeh, H. (2009). Immobilization of [Cu(bpy)2]Br2 Complex onto a Glassy Carbon Electrode Modified with α-SiMo12O404− and Single Walled Carbon Nanotubes: Application to Nanomolar Detection of Hydrogen Peroxide and Bromate. Analytica Chim. Acta 635, 63–70. doi:10.1016/j.aca.2009.01.007
Salomon, W., Dolbecq, A., Roch-Marchal, C., Paille, G., Dessapt, R., Mialane, P., et al. (2018). A Multifunctional Dual-Luminescent Polyoxometalate@Metal-Organic Framework EuW10@UiO-67 Composite as Chemical Probe and Temperature Sensor. Front. Chem. 6, 1–8. doi:10.3389/fchem.2018.00425
Sharifi, M., Dianat, S., and Hosseinian, A. (2021). Electrochemical Investigation and Amperometry Determination Iodate Based on Ionic liquid/polyoxotungstate/P-Doped Electrochemically Reduced Graphene Oxide Multi-Component Nanocomposite Modified Glassy Carbon Electrode. RSC Adv. 11, 8993–9007. doi:10.1039/d1ra00845e
Shen, Y., Peng, J., Zhang, H., Meng, C., and Zhang, F. (2012). Preparation and Application of L-Cysteine-Doped Keggin Polyoxometalate Microtubes. J. Solid State. Chem. 185, 225–228. doi:10.1016/j.jssc.2011.10.048
Shi, S., Duan, J., Jiang, J., Chen, L., and Zhao, J. (2019). A Novel Inorganic-Organic Hybrid 3d-4f Heterometallic Germanotungstate Based on Saturated Keggin-type [α-GeW12O40]4− Polyanion. Inorg. Chem. Commun. 108, 107542. doi:10.1016/j.inoche.2019.107542
Song, W., Liu, Y., Lu, N., Xu, H., and Sun, C. (2000). Application of the Sol-Gel Technique to Polyoxometalates: towards a New Chemically Modified Electrode. Electrochimica Acta 45, 1639–1644. doi:10.1016/s0013-4686(99)00326-6
Song, Y., Xu, M., Li, Z., He, L., Hu, M., He, L., et al. (2020). Ultrasensitive Detection of Bisphenol A under Diverse Environments with an Electrochemical Aptasensor Based on Multicomponent AgMo Heteronanostructure. Sensors Actuators B: Chem. 321, 128527. doi:10.1016/j.snb.2020.128527
Suma, B. P., Adarakatti, P. S., Kempahanumakkagari, S. K., and Malingappa, P. (2019). A New polyoxometalate/rGO/Pani Composite Modified Electrode for Electrochemical Sensing of Nitrite and its Application to Food and Environmental Samples. Mater. Chem. Phys. 229, 269–278. doi:10.1016/j.matchemphys.2019.02.087
Sun, P., Zhang, S., Xiang, Z., Zhao, T., Sun, D., Zhang, G., et al. (2019). Photoluminescent Sensing Vesicle Platform Self-Assembled by Polyoxometalate and Ionic-liquid-type Imidazolium Gemini Surfactants for the Detection of Cr3+ and MnO4− Ions. J. Colloid Interf. Sci. 547, 60–68. doi:10.1016/j.jcis.2019.03.085
Tang, L., Yu, F., Tang, B., Yang, Z., Fan, W., Zhang, M., et al. (2019). Tumor Microenvironment-Activated Ultrasensitive Nanoprobes for Specific Detection of Intratumoral Glutathione by Ratiometric Photoacoustic Imaging. ACS Appl. Mater. Inter. 11, 27558–27567. doi:10.1021/acsami.9b08100
Thakur, N., Das Adhikary, S., Kumar, M., Mehta, D., Padhan, A. K., Mandal, D., et al. (2018). Ultrasensitive and Highly Selective Electrochemical Detection of Dopamine Using Poly(ionic Liquids)-Cobalt Polyoxometalate/CNT Composite. ACS Omega 3, 2966–2973. doi:10.1021/acsomega.7b02049
Thakur, N., Kumar, M., Das Adhikary, S., Mandal, D., and Nagaiah, T. C. (2019). PVIM-Co5POM/MNC Composite as a Flexible Electrode for the Ultrasensitive and Highly Selective Non-enzymatic Electrochemical Detection of Cholesterol. Chem. Commun. 55, 5021–5024. doi:10.1039/c9cc01534e
The European Parliament and the Council of the European Union (2020). Off. J. Eur. Communities 2019, 35.
Tian, A., Han, Z., Peng, J., Zhai, J., and Zhao, Y. (2007). Inorganic-organic Microporous Solid of Wells-Dawson Type Polyoxometalate: Synthesis, Characterization, and Electrochemical Properties. Z. Anorg. Allg. Chem. 633, 495–503. doi:10.1002/zaac.200600320
Tian, A., Liu, J., Li, T., Tian, Y., Liu, G., and Ying, J. (2018). Amperometric Sensing and Photocatalytic Properties under Sunlight Irradiation of a Series of Keggin-AgI Compounds through Tuning Single and Mixed Ligands. CrystEngComm 20, 2940–2951. doi:10.1039/c7ce02214j
Tian, A., Yang, M., Ni, H., Sun, N., Yang, Y., Fu, Y., et al. (2018). Use of Symmetrical and Pendant Pyrazole Derivatives for the Construction of Two Polyoxometalate-Based Complexes as Electrochemical Sensors. Transit. Met. Chem. 43, 621–633. doi:10.1007/s11243-018-0250-4
Tian, R., Zhang, B., Zhao, M., Zou, H., Zhang, C., Qi, Y., et al. (2019). Fluorometric Enhancement of the Detection of H2O2 Using Different Organic Substrates and a Peroxidase-Mimicking Polyoxometalate. RSC Adv. 9, 12209–12217. doi:10.1039/c9ra00505f
Tong, Z., Xu, M., Li, Q., Liu, C., Wang, Y., and Sha, J. (2020). Polyelectrolyte-functionalized Reduced Graphene Oxide Wrapped Helical POMOF Nanocomposites for Bioenzyme-free Colorimetric Biosensing. Talanta 220, 121373. doi:10.1016/j.talanta.2020.121373
Trammell, S. A., Shriver-Lake, L. C., and Dressick, W. J. (2017). Statistical Evaluation of an Electrochemical Probe for the Detection of Chlorate. Sensors Actuators B: Chem. 239, 951–961. doi:10.1016/j.snb.2016.08.087
Turdean, G. L., Curulli, A., Catalin Popescu, I., Rosu, C., and Palleschi, G. (2002). Electropolymerized Architecture Entrapping a Trilacunary Keggin-type Polyoxometalate for Assembling a Glucose Biosensor. Electroanalysis 14, 1550–1556. doi:10.1002/1521-4109(200211)14:22<1550:aid-elan1550>3.0.co;2-6
Turdean, G., and Popescu, I. C. (2012). Self-assembled Architecture Based on Triiron-Substituted Polyoxomolybdate Anion and Positively Charged Polymer. J. Solid State. Electrochem. 16, 681–687. doi:10.1007/s10008-011-1385-9
Veríssimo, M. I. S., Almodôvar, V. A. S., Tomé, A. C., and Gomes, M. T. S. R. (2020). Fluorescent Optrode for Proteins Based on a Diketopyrrolopyrrole Derivative: Practical Application to Total Protein Determination in Urine. Opt. Laser Technol. 130, 106364. doi:10.1016/j.optlastec.2020.106364
Veríssimo, M. I. S., Gamelas, J. A. F., Evtuguin, D. V., and Gomes, M. T. S. R. (2017). Determination of 5-hydroxymethylfurfural in Honey, Using Headspace-solid-phase Microextraction Coupled with a Polyoxometalate-Coated Piezoelectric Quartz crystal. Food Chem. 220, 420–426. doi:10.1016/j.foodchem.2016.09.204
Veríssimo, M. I. S., Gamelas, J. A. F., Fernandes, A. J. S., Evtuguin, D. V., and Gomes, M. T. S. R. (2020). A New Formaldehyde Optical Sensor: Detecting Milk Adulteration. Food Chem. 318, 126461. doi:10.1016/j.foodchem.2020.126461
Veríssimo, M. I. S., Gamelas, J. A. F., Simões, M. M. Q., Evtuguin, D. V., and Gomes, M. T. S. R. (2018). Quantifying Acetaldehyde in Cider Using a Mn(III)-substituted Polyoxotungstate Coated Acoustic Wave Sensor. Sensors Actuators B: Chem. 255, 2608–2613. doi:10.1016/j.snb.2017.09.068
Veríssimo, M. I. S., Silva, P. R., Oliveira, J. A. B. P., and Gomes, M. T. S. R. (2010). Study of the Influence of Polymeric Membrane Composition on the Sensitivity of Acoustic Wave Sensors for Metal Analysis. Sensors Actuators B: Chem. 150, 471–477. doi:10.1016/j.snb.2010.07.055
Viravaux, C., Oms, O., Dolbecq, A., Nassar, E., Busson, L., Mellot-Draznieks, C., et al. (2021). Temperature Sensors Based on Europium Polyoxometalate and Mesoporous Terbium Metal-Organic Framework. J. Mater. Chem. C 9, 8323–8328. doi:10.1039/d1tc01532j
Wang, B., Zheng, J.-H., Wang, X.-H., Zhao, B., Xu, L., and Liu, Z.-R. (2019). Preparation and Chemically Responsive Luminescent Switching of the Flexible Self-Supporting TbW10-Agarose Green Emission Thin Films. J. Inorg. Mater. 34, 844–850. doi:10.15541/jim20180506
Wang, C., Ying, J., Mou, H.-c., Tian, A.-x., and Wang, X.-l. (2020). Multi-functional Photoelectric Sensors Based on a Series of Isopolymolybdate-Based Compounds for Detecting Different Ions. Inorg. Chem. Front. 7, 3882–3894. doi:10.1039/d0qi00505c
Wang, C., Zhou, M., Ma, Y., Tan, H., Wang, Y., and Li, Y. (2018). Hybridized Polyoxometalate-Based Metal-Organic Framework with Ketjenblack for the Nonenzymatic Detection of H2 O2. Chem. Asian J. 13, 2054–2059. doi:10.1002/asia.201800758
Wang, D., Li, Y., Zhang, Y., Xu, X., Liu, Y., Chen, L., et al. (2020). Construction of Ln3+-Substituted Arsenotungstates Modified by 2,5-Thiophenedicarboxylic Acid and Application in Selective Fluorescence Detection of Ba2+ in Aqueous Solution. Inorg. Chem. 59, 6839–6848. doi:10.1021/acs.inorgchem.0c00223
Wang, D., Liu, L., Jiang, J., Chen, L., and Zhao, J. (2020). Polyoxometalate-based Composite Materials in Electrochemistry: State-Of-The-Art Progress and Future Outlook. Nanoscale 12, 5705–5718. doi:10.1039/c9nr10573e
Wang, J., Han, D., Wang, X., Qi, B., and Zhao, M. (2012). Biosens. Bioelectron. 36, 18–21. doi:10.1016/j.bios.2012.03.031
Wang, J., Xu, X., Zhao, L., and Yan, B. (2021). In Situ growth of Polyoxometalate in COF for Trace Monitoring of Ag+ and Hepatocellular Carcinoma Biomarker via a Dual Responsive Strategy. J. Mater. Chem. C 9, 9492–9498. doi:10.1039/d1tc01938d
Wang, L., Meng, T., Sun, J., Wu, S., Zhang, M., Wang, H., et al. (2019). Development of Pd/Polyoxometalate/nitrogen-Doping Hollow Carbon Spheres Tricomponent Nanohybrids: A Selective Electrochemical Sensor for Acetaminophen. Analytica Chim. Acta 1047, 28–35. doi:10.1016/j.aca.2018.09.042
Wang, P., Wang, X., Bi, L., and Zhu, G. (2000). Sol-gel-derived α2-K7P2W17VO62/graphite/organoceramic Composite as the Electrode Material for a Renewable Amperometric Hydrogen Peroxide Sensor. J. Electroanalytical Chem. 495, 51–56. doi:10.1016/s0022-0728(00)00371-5
Wang, T., Sun, Z., Wang, Y., Liu, R., Sun, M., and Xu, L. (2017). Enhanced Photoelectric Gas Sensing Performance of SnO2 Flower-like Nanorods Modified with Polyoxometalate for Detection of Volatile Organic Compound at Room Temperature. Sensors Actuators B: Chem. 246, 769–775. doi:10.1016/j.snb.2017.02.108
Wang, T., Zhou, Q., Ren, X., Zhou, Y., Zhang, L., Shehzad, F. K., et al. (2019). Incorporation of Keggin-type Phosphomolybdic Acid, Ionic Liquid and Carbon Nanotube Leading to Formation of Multifunctional Ternary Composite Materials: Fabrication, Characterization and Electrochemical Reduction/Detection of Iodate, Borate and Nitrite. J. Clust. Sci. 30, 973–984. doi:10.1007/s10876-019-01557-0
Wang, X., Zhao, Y., Zhang, D., Zhang, L., Bai, Z., Pang, H., et al. (2018). Int. J. Electrochem. Sci. 13, 8454–8470.
Wang, X., Zhao, Y., Zhang, D., Rong, S., and Ma, H. (2019). Dawson-type Vanadium-Substituted Tungstophosphate- Modified ITO Electrode: Preparation, Characterization and Electrochemical Determination of Dopamine. Int. J. Electrochem. Sci. 14, 3595–3609. doi:10.20964/2019.04.08
Wang, Y., Ma, Y., Zhao, Q., Hou, L., and Han, Z. (2020). Polyoxometalate-based Crystalline Catalytic Materials for Efficient Electrochemical Detection of Cr(VI). Sensors Actuators B: Chem. 305, 127469. doi:10.1016/j.snb.2019.127469
Wang, Z., Zhang, R., Ma, Y., Peng, A., Fu, H., and Yao, J. (2010). Chemically Responsive Luminescent Switching in Transparent Flexible Self-Supporting [EuW10O36]9−-Agarose Nanocomposite Thin Films. J. Mater. Chem. 20, 271–277. doi:10.1039/b917739f
Wu, H., Chen, H., Fu, M., Li, R., Ma, P., Wang, J., et al. (2019). A PHBA-Functionalized Organic-Inorganic Hybrid Polyoxometalate as a Luminescent Probe for Selectively Sensing Chromium and Calcium in Aqueous Solution. Dyes Pigm. 171, 107696. doi:10.1016/j.dyepig.2019.107696
Xin, X., Hu, N., Ma, Y., Wang, Y., Hou, L., Zhang, H., et al. (2020). Polyoxometalate-based Crystalline Materials as a Highly Sensitive Electrochemical Sensor for Detecting Trace Cr(vi). Dalton Trans. 49, 4570–4577. doi:10.1039/d0dt00446d
Xing, R., Tong, L., Zhao, X., Liu, H., Ma, P., Zhao, J., et al. (2019). Rapid and Sensitive Electrochemical Detection of Myricetin Based on polyoxometalates/SnO2/gold Nanoparticles Ternary Nanocomposite Film Electrode. Sensors Actuators B: Chem. 283, 35–41. doi:10.1016/j.snb.2018.11.129
Xu, H., Bai, Z., Wang, G., O’Halloran, K. P., Tan, L., Pang, H., et al. (2017). Voltammetric Determination of Folic Acid at Physiological pH Values by Using a Glassy Carbon Electrode Modified with a Multilayer Composite Consisting of Polyoxometalate (H8P2Mo16V2O62) and Reduced Graphene Oxide and Prepared via Layer-By-Layer Self-Assembly and In-Situ Photoreduction. Microchim. Acta 184, 4295–4303. doi:10.1007/s00604-017-2447-1
Xu, H. (2019). One-pot Preparation of Three-Dimensional Macroporous Phosphomolybdic Acid-MoS2-Reduced Graphene Oxide Hybrid for Electrochemical Detection of Nitrite. Int. J. Electrochem. Sci. 14, 7258–7269. doi:10.20964/2019.08.81
Xu, J., Xu, S., Feng, S., Hao, Y., and Wang, J. (2015). Electrochemical Sensor for Detecting Both Oxidizing and Reducing Compounds Based on Poly(ethyleneimine)/phosphotungstic Acid Multilayer Film Modified Electrode. Electrochimica Acta 174, 706–711. doi:10.1016/j.electacta.2015.06.058
Xu, M., Liu, C., Wang, Y., Wang, J., Feng, J., and Sha, J. (2021). A New Well-Dawson Polyoxometalate Based Compound Containing Helix for Electrochemical Uric Acid Biosensor. J. Clust. Sci. 32, 1543–1552. doi:10.1007/s10876-020-01845-0
Yang, M., Kim, D. S., Lee, T. J., Lee, S. J., Lee, K. G., and Choi, B. G. (2016). Polyoxometalate-grafted Graphene Nanohybrid for Electrochemical Detection of Hydrogen Peroxide and Glucose. J. Colloid Interf. Sci. 468, 51–56. doi:10.1016/j.jcis.2016.01.047
Yang, M., Kim, D. S., Yoon, J. H., Hong, S. B., Jeong, S. W., Yoo, D. E., et al. (2016). Nanopillar Films with Polyoxometalate-Doped Polyaniline for Electrochemical Detection of Hydrogen Peroxide. Analyst 141, 1319–1324. doi:10.1039/c5an02134k
Yang, M., Rong, S., Wang, X., Ma, H., Pang, H., Tan, L., et al. (2021). Preparation and Application of Keggin Polyoxometalate‐based 3D Coordination Polymer Materials as Supercapacitors and Amperometric Sensors. ChemNanoMat 7, 299–306. doi:10.1002/cnma.202000654
Ying, J., Chen, Y.-G., and Wang, X.-Y. (2019). A Series of Wells-Dawson and Keggin-Based Compounds with Uncoordinated S Donors as Fluorescence Sensors to Hg2+. J. Coord. Chem. 72, 3544–3561. doi:10.1080/00958972.2019.1699914
Ying, J., Sun, C., Jin, L., Tian, A., and Wang, X. (2021). Five Compounds Based on [TeMo6O24]6− and [β-Mo8O26]4− Anions Synthesized by Using Different Symmetrical and Asymmetric N-Donor Ligands. CrystEngComm 23, 5385–5396. doi:10.1039/d1ce00775k
Yokuş, Ö. A., Kardaş, F., Akyildirim, O., Eren, T., Atar, N., and Yola, M. L. (2016). Sensors Actuators, B Chem. 233, 47–54.
Yola, M. L., Atar, N., Eren, T., Karimi-Maleh, H., and Wang, S. (2015). Sensitive and Selective Determination of Aqueous Triclosan Based on Gold Nanoparticles on Polyoxometalate/reduced Graphene Oxide Nanohybrid. RSC Adv. 5, 65953–65962. doi:10.1039/c5ra07443f
Yola, M. L., Göde, C., and Atar, N. (2017). Molecular Imprinting Polymer with Polyoxometalate/carbon Nitride Nanotubes for Electrochemical Recognition of Bilirubin. Electrochimica Acta 246, 135–140. doi:10.1016/j.electacta.2017.06.053
Yola, M. L., Gupta, V. K., and Atar, N. (2016). New Molecular Imprinted Voltammetric Sensor for Determination of Ochratoxin A. Mater. Sci. Eng. C 61, 368–375. doi:10.1016/j.msec.2015.12.057
Yuan, J., Wang, L., Wang, Y., Dong, S., and Hao, J. (2019). Eu3+-Controlled Fluorescent Bilayer Vesicles. Langmuir 35, 4125–4132. doi:10.1021/acs.langmuir.9b00302
Zhang, B., Ying, J., Zhang, X., Wang, C., and Tian, A. (2021). Electrocatalytic, Photocatalytic, Fluorescence Sensing and CO2RR Properties of a Series of Homopolymolybdate Hybrid Coordination Polymers. New J. Chem. 45, 13340–13348. doi:10.1039/d1nj02283k
Zhang, D., Ma, H., Chen, Y., Pang, H., and Yu, Y. (2013). Amperometric Detection of Nitrite Based on Dawson-type Vanodotungstophosphate and Carbon Nanotubes. Analytica Chim. Acta 792, 35–44. doi:10.1016/j.aca.2013.07.010
Zhang, H., Lin, X., Yan, Y., and Wu, L. (2006). Luminescent Logic Function of a Surfactant-Encapsulated Polyoxometalate Complex. Chem. Commun. 2, 4575–4577. doi:10.1039/b606343h
Zhang, L., Khungwa, J., Liu, Y., Li, L., Wang, X., and Wang, S. (2019). Fabrication of Electro-Active Pt/IMo6O24/Graphene Oxide Nanohybrid Modified Electrode for the Simultaneous Determination of Ascorbic Acid, Dopamine and Uric Acid. J. Electrochem. Soc. 166, H351–H358. doi:10.1149/2.1141908jes
Zhang, L., Li, S., O’Halloran, K. P., Zhang, Z., Ma, H., Wang, X., et al. (2021). A Highly Sensitive Non-enzymatic Ascorbic Acid Electrochemical Sensor Based on polyoxometalate/Tris(2,2ˊ-Bipyridine)ruthenium (II)/chitosan-palladium Inorganic-Organic Self-Assembled Film. Colloids Surf. A: Physicochemical Eng. Aspects 614, 126184. doi:10.1016/j.colsurfa.2021.126184
Zhang, L., Wang, Q., Qi, Y., Li, L., Wang, S., and Wang, X. (2019). An Ultrasensitive Sensor Based on Polyoxometalate and Zirconium Dioxide Nanocomposites Hybrids Material for Simultaneous Detection of Toxic Clenbuterol and Ractopamine. Sensors Actuators B: Chem. 288, 347–355. doi:10.1016/j.snb.2019.03.033
Zhang, T., Liu, M., Zhang, Q., Wang, Y., Kong, X., Wang, L., et al. (2017). Sensitive Determination of Chlorogenic Acid in Pharmaceutical Products Based on the Decoration of 3D Macroporous Carbon with Au Nanoparticles via Polyoxometalates. Analyst 142, 2603–2609. doi:10.1039/c7an00493a
Zhang, T., Qin, L., Kang, S.-z., Li, G., and Li, X. (2017). Novel Reduced Graphene oxide/Ag Nanoparticle Composite Film with Sensitive Detection Activity towards Trace Formaldehyde. Sensors Actuators B: Chem. 242, 1129–1132. doi:10.1016/j.snb.2016.09.134
Zhang, W., Du, D., Gunaratne, D., Colby, R., Lin, Y., and Laskin, J. (2014). Polyoxometalate-Graphene Nanocomposite Modified Electrode for Electrocatalytic Detection of Ascorbic Acid. Electroanalysis 26, 178–183. doi:10.1002/elan.201300343
Zhang, W., Jia, G., Li, Z., Yuan, C., Bai, Y., and Fu, D. (2017). Selective Electrochemical Detection of Dopamine on Polyoxometalate-Based Metal-Organic Framework and its Composite with Reduced Graphene Oxide. Adv. Mater. Inter. 4, 1601241. doi:10.1002/admi.201601241
Zhang, X., Bao, Y., Bai, Y., Chen, Z., Li, J., and Feng, F. (2019). In Situ electrochemical Reduction Assisted Assembly of a Graphene-Gold Nanoparticles@polyoxometalate Nanocomposite Film and its High Response Current for Detection of Hydrogen Peroxide. Electrochimica Acta 300, 380–388. doi:10.1016/j.electacta.2019.01.084
Zhang, Y., Bo, X., Nsabimana, A., Munyentwali, A., Han, C., Li, M., et al. (2015). Green and Facile Synthesis of an Au Nanoparticles@polyoxometalate/ordered Mesoporous Carbon Tri-component Nanocomposite and its Electrochemical Applications. Biosens. Bioelectron. 66, 191–197. doi:10.1016/j.bios.2014.11.022
Zhang, Y., Wang, D., Zeng, B., Chen, L., Zhao, J., and Yang, G.-Y. (2020). An Unprecedented Polyhydroxycarboxylic Acid Ligand Bridged Multi-EuIII Incorporated Tellurotungstate and its Luminescence Properties. Dalton Trans. 49, 8933–8948. doi:10.1039/d0dt00729c
Zhang, Y., Zeng, B., Liu, Y., Li, P., Chen, L., and Zhao, J. (2020). A Penta‐Eu III Sandwiched Dawson Selenotungstate and its Unique Luminescence Properties. Eur. J. Inorg. Chem. 2020, 3416–3425. doi:10.1002/ejic.202000519
Zhang, Z., Li, Y., Liu, X., Zhang, Y., and Wang, D. (2018). Molecular Imprinting Electrochemical Sensor for Sensitive Creatinine Determination. Int. J. Electrochem. Sci. 13, 2986–2995. doi:10.20964/2018.03.67
Zhou, C., Li, S., Zhu, W., Pang, H., and Ma, H. (2013). A Sensor of a Polyoxometalate and Au-Pd alloy for Simultaneously Detection of Dopamine and Ascorbic Acid. Electrochimica Acta 113, 454–463. doi:10.1016/j.electacta.2013.09.109
Zhou, K., Han, H., Sha, J., Luan, S., Diao, Y., Dong, C., et al. (2021). Synthesis of POMOFs with 8-fold helix and its Composite with Carboxyl Functionalized SWCNTs for the Voltammetric Determination of Dopamine. Anal. Bioanal. Chem. 413, 5309–5320. doi:10.1007/s00216-021-03504-3
Zhou, M., Guo, L.-p., Lin, F.-y., and Liu, H.-x. (2007). Electrochemistry and Electrocatalysis of Polyoxometalate-Ordered Mesoporous Carbon Modified Electrode. Analytica Chim. Acta 587, 124–131. doi:10.1016/j.aca.2007.01.017
Zhou, S., Gu, C., Li, Z., Yang, L., He, L., Wang, M., et al. (2019). Ti3C2Tx MXene and Polyoxometalate Nanohybrid Embedded with Polypyrrole: Ultra-sensitive Platform for the Detection of Osteopontin. Appl. Surf. Sci. 498, 143889. doi:10.1016/j.apsusc.2019.143889
Zhu, D., Guo, D., Zhang, L., Tan, L., Pang, H., Ma, H., et al. (2019). Non-enzymatic Xanthine Sensor of Heteropolyacids Doped Ferrocene and Reduced Graphene Oxide via One-step Electrodeposition Combined with Layer-By-Layer Self-Assembly Technology. Sensors Actuators B: Chem. 281, 893–904. doi:10.1016/j.snb.2018.10.151
Zhu, H., Tang, W., Ma, Y., Wang, Y., Tan, H., and Li, Y. (2021). Preyssler-type Polyoxometalate-Based Crystalline Materials for the Electrochemical Detection of H2O2. CrystEngComm 23, 2071–2080. doi:10.1039/d1ce00059d
Zhu, W., Zhang, W., Li, S., Ma, H., Chen, W., and Pang, H. (2013). Fabrication and Electrochemical Sensing Performance of a Composite Film Containing a Phosphovanadomolybdate and Cobalt(II) Tetrasulfonate Phthalocyanine. Sensors Actuators B: Chem. 181, 773–781. doi:10.1016/j.snb.2013.01.050
Zou, W., González, A., Jampaiah, D., Ramanathan, R., Taha, M., Walia, S., et al. (2018). Skin Color-specific and Spectrally-Selective Naked-Eye Dosimetry of UVA, B and C Radiations. Nat. Commun. 9, 1–10. doi:10.1038/s41467-018-06273-3
Keywords: polyoxometalate (POM), POM hybrid materials, electrochemical sensors, optical sensors, piezoelectric sensors
Citation: Veríssimo MIS, Evtuguin DV and Gomes MTSR (2022) Polyoxometalate Functionalized Sensors: A Review. Front. Chem. 10:840657. doi: 10.3389/fchem.2022.840657
Received: 21 December 2021; Accepted: 20 January 2022;
Published: 08 March 2022.
Edited by:
Manuel Aureliano, University of Algarve, PortugalReviewed by:
Nadiia I. Gumerova, University of Vienna, AustriaCopyright © 2022 Veríssimo, Evtuguin and Gomes. This is an open-access article distributed under the terms of the Creative Commons Attribution License (CC BY). The use, distribution or reproduction in other forums is permitted, provided the original author(s) and the copyright owner(s) are credited and that the original publication in this journal is cited, in accordance with accepted academic practice. No use, distribution or reproduction is permitted which does not comply with these terms.
*Correspondence: Marta I. S. Veríssimo, bXZlcmlzc2ltb0B1YS5wdA==; M. Teresa S. R. Gomes, bXRnb21lc0B1YS5wdA==
Disclaimer: All claims expressed in this article are solely those of the authors and do not necessarily represent those of their affiliated organizations, or those of the publisher, the editors and the reviewers. Any product that may be evaluated in this article or claim that may be made by its manufacturer is not guaranteed or endorsed by the publisher.
Research integrity at Frontiers
Learn more about the work of our research integrity team to safeguard the quality of each article we publish.