- College of Chemistry and Chemical Engineering, Henan Engineering Laboratory of Green Synthesis for Pharmaceuticals, and Henan Key Laboratory of Biomolecular Recognition and Sensing, Shangqiu Normal University, Shangqiu, China
A novel valine-based isocyanonaphthalene (NpI) was designed and synthesized by using an easy method and enabled the selective fluorescence detection of Hg2+. The chemodosimeter can display an immediate turn-on fluorescence response (500-fold) towards target metal ions upon the Hg2+-mediated conversion of isocyano to amino within NpI. Based on this specific reaction, the fluorescence-enhancement probe revealed a high sensitivity toward Hg2+ over other common metal ions and exhibited excellent aqueous solubility, good antijamming capability, high sensitivity (detection limit: 14.2 nM), and real-time detection. The response mechanism of NpI was supported by NMR spectroscopy, MS analysis and DFT theoretical calculation using various techniques. Moreover, a dipeptidomimetic NpI probe was successfully applied to visualize intracellular Hg2+ in living cells and monitor Hg2+ in real water samples with good recoveries and small relative standard deviations.
Introduction
Mercury in the environment is one of the most hazardous heavy metals because of its known toxicity and accumulated features towards some aquatic life and humans. Various processes can lead to the release and accumulation of mercury from human activities, such as the burning of coal and gasoline, solid waste incineration, the daily diet of fish and sea mammals, and the instrument industry. Studies revealed that exposure of the body to inorganic mercury in health risks (mostly in the form of Hg2+), will result in substantial damage to the nervous system and endocrine system. Besides, these mercuric salts have a high affinity for thiol groups containing proteins and enzymes, which are mainly damaged kidneys and then widely distributed throughout the body. The quick and effective detection of Hg2+ analytics from possible natural and tap water pollution is of great importance (Celo, et al., 2006; Driscoll, et al., 2013). Therefore, developing novel fluorescent sensors for Hg2+ with good selectivity, high sensitivity. and more importantly applicable to complex environmental and biological systems, has received considerable interest in the areas (Carter, et al., 2014; Wu, et al., 2017).
Over the past few years, researchers have tried to develop efficient Hg2+ probes based on small organic molecules, polymers, and metal-organic complexes (Chen, et al., 2019; Lu, et al., 2016; Oing, et al., 2021; Ru, et al., 2014; Singha, et al., 2019; Thomas, et al., 2007). Fluorescent sensors based on small molecules, in particular, have significant advantages such as their exquisite and noninvasive size, high sensitivity, selectivity, and fast response time. To date, numerous fluorescent Hg2+ (Chen, et al., 2015; Kim, et al., 2012) probes have been reported based on varieties of fluorophore like Rhodamine (Bera, et al., 2014; Chang, et al., 2018; Su, et al., 2017; Tang, et al., 2017; Zhao, et al., 2010), BODIPY (Hatai, et al., 2012; Lu, et al., 2009; Yoon, et al., 2005), Coumarin (Sie, et al., 2017; Puthiyedath and Bahulayan, 2018), Schiff base (Chen, et al., 2018; Duan, et al., 2019; Sarkar, et al., 2016; Wang, et al., 2018b; Wang, et al., 2018d; Zhang, et al., 2017), AIE luminophore (Chen, et al., 2017; Selvaraj, et al., 2021; Wang, et al., 2018a), Peptide-based benzoxazole unit (Oliveira, et al., 2011; Oliveira, et al., 2013), etc. These probes were usually prepared either through the complexation with the multiple binding sites or through a chemical reaction with the proper functional group onto fluorescent molecules. However, some of the sensors often show some limitations, such as laborious and expensive synthesis, fluorescence quenching measurement, poor aqueous solubility or slow dissolution, and limited selectivity as a result of interference from competing metal ions. Therefore, the development and improvement of specific responses towards Hg2+ based on fluorescence-enhancement, which leads to more sensitivity and robustness than fluorescence-quenching, is still a highly active field in environmental sensor and sensing.
Currently, fluorescent probes based on naphthalene (Np) skeleton have received much attention over the last several years (Banerjee, et al., 2012; Roek, et al., 2000). Among many classes of fluorophores, Np provides an ideal platform that can be derivatized with ease for design and development of fluorescent chemosensors such as, fluorescent sensors derived using naphthalene moiety and suitable amine derivative which often provide an excellent functionalized backbone for a variety of analytes (Das and Goswami, 2017), including the hydrazide derivative containing naphthyl backbone (Pannipara, et al., 2018), various naphthyl Schiff base derivatives (Das, et al., 2013; Zhang, et al., 2013), naphthalimides (Shu, et al., 2015) and naphthyl polymer materials (DeRosa, et al., 2014).
In addition, the isocyano group of isocyanides is an extremely versatile and functional building block with excellent reactivity including the ability to react with both nucleophiles, electrophiles, and radicals, simultaneously (Songab and Xu, 2017; Zhang and Studer, 2015; Zhang, et al., 2020). They are used as privileged synthons for multicomponent reactions (MCRs), heterocyclic ring formation, and other insertion reactions in organic and drug synthesis (Chakrabarty, et al., 2014; Sadjadi, et al., 2016; Vavsari, et al., 2020). Isocyanides have also attracted the attention of coordination chemists due to their strong σ-donor and weak π-acceptor properties (Boyarskiy, et al., 2015; Yamamoto, 1980), and for advances in the synthesis and application of helical polymers, and the screw-sense polyisocyanides have been extensively used to the living polymerization of the corresponding isocyanide monomers (Wang, et al., 2018c; Zhou, et al., 2021). Notably, the chemical reactions based on the isonitrile group have recently been exploited for the design of fluorescent Hg2+ probes (Adamoczky, et al., 2020; Nagy, et al., 2019; Zhang, et al., 2018), which involves the Hg2+-mediated conversion of isonitrile to the amino group. Only two isocyano-functionalized fluorescent probes thay display distinct optical response have been explored. Xie group synthesized a isocyano-functionalized 1,8-naphthalimide derivative for ratiometric fluorescent sensing of Hg2+. Subsequently, the Kéki group designed a series of isocyanonaphthalene (NpI) derivatives as fluorescence probes for Hg2+. However, some problems still exist such as the use of substantial amounts of organic solvent, the relatively slow response time, and poor anti-interference ability.
Recently, we developed a series of new polyisocyanopeptides bearing amino-acid ester side chains as a novel weakly orienting medium for RDC-based configurational analysis of small organic molecules, and the related dipeptidomimetic isonitrile monomers of polyisocyanides are much synthesized and studied (Li, et al., 2017; Li, et al., 2018). To our best knowledge, compared with the well-established study of isocyanides in polymerization and organometallic domain, and traditional organic synthesis, the construction of dipeptidomimetic isocyanide-based chemosensors was rarely focused on, which may be due to its high reactivity and the lack of excellent synthesis methods. Furthermore, the introduction of amino-based dipeptidomimetic pendant to chromophores can efficiently increase its biocompatibility for fluorescent bioimaging in living systems. Herein, we disclose a novel valine-based naphthyl isocyanide (named NpI, Scheme 1) for trace level detection of mercury ions and visualize its bio-imaging in live cells. The enhanced fluorescent probe exhibited distinctive selectivity over other metal ions, fast response, and excellent sensitivity toward Hg2+. In this work, we present its synthesis depending on our mature method, characterization, sensing behavior in detail, and the related mechanism which was also described by the reduction of the isonitrile group to the resulting primary amine as a chemical reaction process. Furthermore, valine-based dipeptidomimetic isocyanonaphthalene chemosensor NpI can be successfully applied to the imaging of intracellular Hg2+ ions due to its good biocompatibility.
Materials and Methods
Materials
Dimethyl sulfoxide (DMSO), analytical HgCl2, all synthetic starting materials, and solvents were used as received without further purification. Dry dichloromethane (DCM) was freshly distilled over calcium hydride (CaH2) under argon atmosphere. The chlorate salts used in the form of aqueous stock solutions were Al3+, Co2+, Cd2+, Cr3+, Fe3+, Ca2+, K+, Mg2+, Li+ and Na+, and other Cu2+, Mn2+, Ni2+, Pb2+, Sr2+ and Zn2+ were prepared from its sulfate salts. These stock solutions were provided by the Key Laboratory of Biomolecular Recognition and Sensing of Shangqiu Normal University. All fluorescence measurements were performed at room temperature on Agilent technologies carrying an eclipse fluorescence spectrophotometer. UV-visible spectra were recorded on a Perkin-Elmer (Lambda 850) spectrometer. The pH measurements were made with a Model PHS-3C. The measurement procedure including the stock solution of NpI, the stock solution of analytes, etc., are presented in detail in the Supplementary Material.
Synthesis and Characterization of Mercury Sensor NpI
The synthesis of intermediates 1-2 was described in detail in the Supplementary Material. Under the argon atmosphere, N-formyl-dipeptidyl amide 2 (0.85 g, 2.1 mmol) was dissolved in 25 ml dry CH2Cl2, triethylamine (Et3N, 0.75 ml, 5.5 mmol, 2.0 equiv) was added, and the reaction mixture was cooled to 0°C. Over a period of 1 h, a solution of triphosgene (BTC, 443 mg, 1.5 mmol) in 10 ml dry CH2Cl2 was added dropwise into the mixture via additional vessel, the temperature of reaction system was maintained at 0°C. The resulting reaction mixture was stirred for an additional 3 h at room temperature. An ice-cold saturated NaHCO3 aqueous solution (10 ml) was added and the mixture was stirred vigorously for 5 min. The organic part was separated and next extracted with CH2Cl2, washing once with brine and dried over anhydrous Na2SO4, filtered, and concentrated. Purification on silica column chromatography (Petroleum ether: EtOAc = 3:1) afforded desired probe NpI (0.61 g, yield: 81%). M. p. = 128.1–128.5°C. [α] 20D = +47.48 (c 0.20, in CHCl3). 1H NMR (400 MHz, CDCl3) δ (ppm) 8.30 (d, J = 1.7 Hz, 1H), 7.94–7.86 (m, 4H), 7.47 (d, J = 8.7 Hz, 1H), 7.43–7.30 (m, 5H), 6.87 (d, J = 8.6 Hz, 1H), 5.27 (d, J = 12.2 Hz, 1H), 5.20 (d, J = 12.2 Hz, 1H), 4.89 (dd, J = 8.6, 4.8 Hz, 1H), 2.37–2.32 (m, 1H), 1.02 (d, J = 6.9 Hz, 3H), 0.98 (d, J = 6.8 Hz, 3H). 13C NMR (100 MHz, CDCl3) δ (ppm) 172.1, 166.7, 165.5, 135.2, 134.2, 133.2, 132.1, 130.8, 128.8, 128.7, 128.6, 128.5, 128.3, 127.5, 125.6, 125.4, 124.4, 67.4, 57.6, 31.8, 19.1, and 17.9. FT-IR (cm−1): 3265, 2950, 2125, 1730, 1635, 1535, 1200, 700. HRMS (ESI): m/z: anal. calcd for C24H22N2O3 [M + Na]+409.1630, found 409.1649.
Results and Discussion
Design and Synthesis of Probe NpI
The NpI is composed of a naphthalene fluorophore bearing a valine benzyl ester as the pendant and a -N≡C moiety as a specific recognition site for Hg2+. The practical synthetic route of the new dipeptidomimetic NpI is shown in Scheme 1, and the target compound and related precursors were fully characterized via standard NMR spectroscopy and high-resolution mass spectrometry (HRMS).
A concise and highly efficient synthetic route to dipeptidomimetic NpI is presented here. The traditional method of preparing the intermediate 1 analogue is cumbersome and not environmentally friendly. Such as, intermediate 1 has been prepared mainly by condensation reaction with para-nitro aromatic acid, and then a need for reducing the nitro group of the aromatic moiety under Pd/C catalyzed hydrogenation condition, and the synthesis of this compound usually consists of two steps (Kajitani, et al., 2006; Reller, et al., 2017). Herein, a novel method is proposed to generate dipeptidyl amide 1 from the corresponding para-amino aromatic acid instead of para-nitro aromatic acid. Thus, the synthesis of sensor NpI is very straightforward through a three-step synthetic route (Scheme 1). First, the direct coupling reaction of 6-amino-2-naphthoic acid and valine benzyl ester affords dipeptidyl amide 1 in 84% yield. N-Formylation of intermediate 1 with formic acid proceeds smoothly to afford the desired intermediate 2 in 72% yield. Finally, base-promoted conversion of triphosgene with 2 via a dehydration reaction furnishes NpI in 81% yield (the general synthesis procedure is illustrated in Supplementary Scheme S1).
As referred to previously, the synthesis of NpI bearing valine benzyl ester is crucial in fluorescent sensor applications. Therefore, the chemical structure of the novel dipeptidomimetic NpI has been elucidated by means of spectroscopic methods. In FT-IR spectrum of NpI, the characteristic vibration for the ester carbonyl C=O bond and amide carbonyl C=O bond was located at 1730 and 1635 cm−1 respectively, and the stretching vibration absorption of isocyanic N≡C bond were clearly observed at 2125 cm−1. 1H NMR spectrum of NpI showed to two doublet signals at δ ∼1.0 and multiple signals at ∼2.35 ppm, which corresponds to the classical isopropyl group of valine residue (Supplementary Figure S5), suggesting that the valine-based dipeptidomimetic has been completely condensed onto naphthalene backbone. The successful synthesis of sensor NpI can also be supported by 13C NMR structural characterization (Supplementary Figure S6). The carbon signal of NpI exhibits ester- and amide-carbonyl carbon signals at δ ∼172 and ∼166 ppm, significantly, and a quaternary carbon of isocyano group showed to the lowest signal at δ ∼165 ppm. According to the above-mentioned detailed characterization analyses, we can conclude that the novel dipeptidomimetic NpI sensor has been successfully synthesized in three steps using a simple methodology.
Absorption Spectroscopy of Dipeptidomimetic NpI to Hg2+
With probe NpI on hand, the spectral properties of the sensor in the presence or absence of Hg2+ ions were determined. We first checked the UV-vis spectrum of the probe, as shown in Figure 1, and the absorption spectrum of dipeptidomimetic NpI has exhibited an obvious maximum absorption peak around 320 nm in the PBS buffer solutions at room temperature (containing 0.5% DMSO), which can be ascribed to the naphthyl ring π-scaffold transition band. Whereas, a new broad absorption band centered at around 350 nm was monitored on incremental addition of Hg2+, which is slightly red-shifted from the absorption maximum in solution. Consequently, fluorescence spectra were recorded under UV excitation, the free NpI has almost non-fluoresce emissive. However, the fluorescent intensity of NpI increased rapidly upon the addition of Hg2+, and 1.0 equitv of Hg2+ ions triggered a 500-fold emission enhancement and the fluorescence behavior was similar to that of naphthaline fluorophore (Zhang, et al., 2018). Then, the influence of the buffer system on the fluorescence property and response of probe NpI to Hg2+ was investigated. The screening of solutions indicates that the PBS buffer solutions containing 0.5% DMSO were optimal and suitable for further sensing application (Supplementary Figure S10).
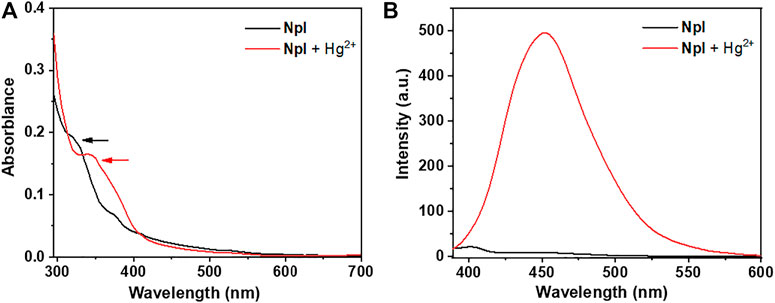
FIGURE 1. (A) Absorption spectra of NpI (black line) and NpI + 1.0 equiv HgCl2 (red line) in PBS buffer solutions. (B) Emission spectra of NpI (black line) and in the presence of 1.0 equiv HgCl2 (red line) in PBS buffer solutions.
Selectivity Investigation and Fluorescence Titration Investigation
To obtain an insight into the probing property and demonstrate the high selectivity of probe NpI in practice, different metal cations were introduced into the solution of NpI, and we investigated the effects of 17 different metal ions on the fluorescence of NpI under the same conditions. An enhancing effect was observed while interacting with Hg2+, while the individual addition of other ions like Al3+, Ca2+, Cd2+, Ni2+, Co2+, Cr3+, Cu2+, Fe3+, K+, Li+, Na+, Mg2+, Mn2+, Pb2+, Sr2+and Zn2+ exerted no effect on any apparent emission enhancement. Figure 2 depicted the fluorescence responses of NpI to various environmentally relevant metal ions. These observations indicate that probe NpI only showed sensitively fluorescent turn-on response to Hg2+ ions. Interestingly, from the photos of probe NpI before and after treatment of Hg2+, we can clearly find that with the addition of Hg2+, the UV light probing platform displayed weak alteration (under portable 365 nm UV lamp), it emitted visible pale-blue light, and such alteration can be easily observed by the naked eyes. The finding results suggest that NpI has the immense potential to act as a selective Hg2+-detection probe. Moreover, to further confirm the selectivity of the tested metal sensing response of NpI, the competitive experiments were subsequently carried out. The experimental results were presented in Figure 2, as observed, the fluorescence emission intensity monitored in the presence of 1.0 equiv of Hg2+ ion mixed with 1.0 equiv of various cations, respectively, red columns represented the fluorescence intensity of probe NpI in the presence of Hg2+ and other competing metal ions. These aforementioned results indicated that NpI was not interfered with by other metal cations and a Hg2+ ion-specific fluorescent recognition sensor. Such an outstanding specificity would effectively avoid false positives.
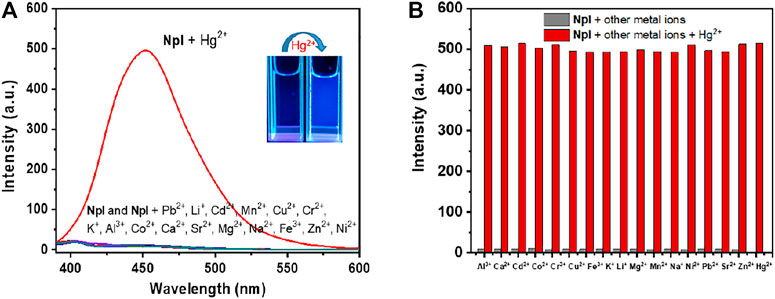
FIGURE 2. (A) Fluorescence enhancement of NpI (1 μM, pH = 7.4, 10 mM PBS) in the presence of other competing metal ions (1 μM, λex = 350 nm). Inset: fluorescence changes of NpI under the portable UV lamp at 365 nm by the naked eyes. (B) Column diagram for fluorescence intensity changes of probe NpI (1 μM) to various 1 μM competing transition-metal ions. The lower one (gray bar) is in the presence of only competing metal ions with NpI; the higher one (red bar) is in the presence of a mixture of the analyte and Hg2+ with NpI.
Under the optimized condition, the sensitivity of probe NpI was then carried out by fluorescence emission response toward different concentrations of Hg2+ ion, as shown in Figure 3. As expected, the fluorescence intensity increased upon incremental addition concentrations of Hg2+. While the fluorescence intensity enhanced nearly 500-fold when 1.0 equiv Hg2+ ion was added to the same solution. The plot of fluorescence intensity of NpI at 450 nm linearly increased up to gradual addition of Hg2+ concentration from 0.1 to 2.0 μM. There is a good linear relationship between the fluorescence response and the added Hg2+ concentration (R2 = 0.991). The detection limit of NpI for the concentration-dependent response manner of Hg2+ was measured to be 14.2 nM (2.8 ppb) based on 3σ/k.
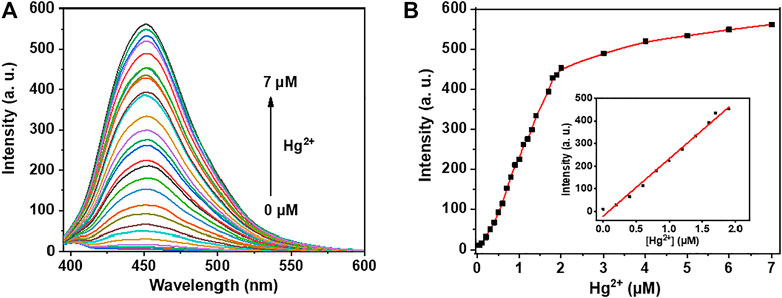
FIGURE 3. (A) Fluorescence titration of NpI (1 μM) with Hg2+ ion (0–7.0 μM) in PBS aqueous buffer (10 mM, pH = 7.4). (B) Linear relationship between fluorescence emission intensity and added Hg2+ concentrations. Inset: the calibration curve, the linear relationship is expressed as y = 253x−19.9 (R2 = 0.991).
The Effect of pH and Responding Speed Investigation on the Detection of Hg2+
The detection of heavy and transitional metal ions in acidic conditions is indispensable for practical applications because the low pH facilitates metal solubility in water, and the contamination of heavy ions to the environment system, will be more serious in acidic conditions, and it would be more useful in identifying the total soluble heavy metal ions in usual water. Therefore, studies on the pH effect (in the range of pH 3.0–11.0 of sensing PBS buffer) on the fluorescence response of NpI were observed in the presence of Hg2+ ion. As recorded by the results in Figure 4, the probe NpI was stable and provided a good spectral response to HgCl2 heavy metal ion over a wide pH range from 5.0 to 9.0, indicating that the probe can endure the acidity of the river and lake water and has a strong spectral response to the analyte (Supplementary Figure S11). As a chemosensor for practical applications, short responding time or high response speed is advantageous for sensors to raise the detection efficiency in a certain range, that is, realize the real-time analysis. Therefore, the time-dependent fluorescent changes of the NpI (1 μM) in the presence of 1.0 equiv of Hg2+ ion was measured under the same PBS buffer solution. As can be seen from Figure 4, the recognition interaction was almost completed within 1 min, and it showed an almost undetectable time-delay when the addition of Hg2+ ion. The result showed that sensor NpI was a sensitive sensor and could be applied in real-time monitoring of target metals in some environmental analysis.
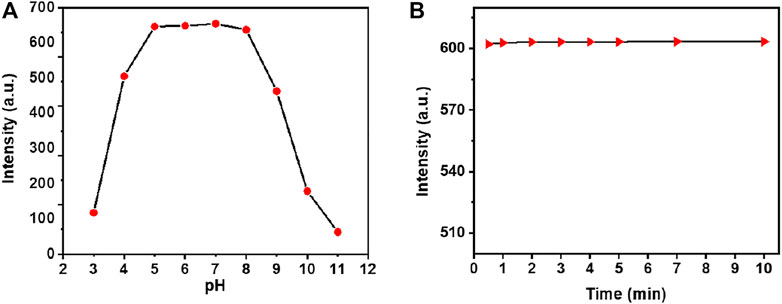
FIGURE 4. (A) The pH titration plots for probe NpI at different pH PBS buffer solutions (containing 0.5% DMSO as a cosolvent). (B) Time evolution of NpI (1 μM) in PBS aqueous (pH = 7.4) in the presence of 1.0 equiv Hg2+ ion.
Plausible Detection Mechanism and Theoretical Computations
According to similar mechanism studies (Adamoczky, et al., 2020; Nagy, et al., 2019; Zhang, et al., 2018) which involve aromatic isocyanides as high effective sensors for the quantification of target metal Hg2+, the sensing mechanism can be also speculated to the Hg2+-induced hydration of isocyano group to amino group in water. To verify this conversion process, the fluorescence response of precursor compound, i.e., intermediate 1, was firstly investigated toward Hg2+ under the identical measurement experimental condition of dipeptidomimetic NpI sensor. One can find that the precursor intermediate 1 was an almost indiscernible response to Hg2+ (Supplementary Figure S12). Meanwhile, the fluorescence spectra of NpI + Hg2+ and intermediate 1 were almost the same to each other, and this phenomenon has been brought to our attention. To get circumstantial evidence regarding the probing detection mechanism, NMR experiments have been performed by the concomitant addition of NpI into HgCl2 in deuterated reagents. Figure 5 shows the 13C NMR spectrum of free NpI in CDCl3, the ester-, amide-carbonyl, and isocyanic carbon signals were clearly discernible at δ 172, 166, and 165 ppm, respectively. However, upon the addition of a certain amount of HgCl2, the signal intensity of the isocyano group gradually disappeared at δ 165 ppm. The above results indicated the conversion of the isocyano moiety to amino group in water by Hg2+-assisted hydration reaction. Subsequently, we carried out experimental verification by quickly mixing NpI/HgCl2 in chloroform system, the reaction solution immediately turned yellow-ish green, the mixture was washed with H2O and purified on TLC, and the NpI/Hg2+-induced trace product was analyzed by 1H NMR. Interestingly, the spectra of NpI/Hg2+and intermediate 1 were exactly the same (Supplementary Figure S13), suggesting that the NpI-based probing system could be, indeed, assigned to reaction-type probing mechanism instead of complexation mechanism.
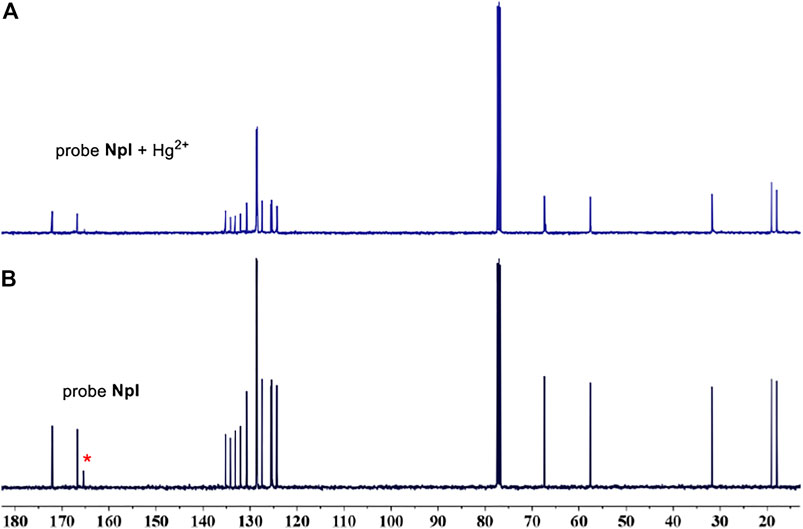
FIGURE 5. (A) The 13C NMR (CDCl3, 400 MHz, 298 K) spectra of NpI. (B) Evolution of the 13C NMR spectra of NpI with the addition of HgCl2.
To further get direct evidence for reaction-type products formed in the PBS aqueous solution/DMSO mixture, ESI-MS analysis was performed from aqueous medium. The 1:1 reaction mixtures were immediately analyzed similarly to those for the fluorescence experiments by HRMS (Supplementary Figure S14). The determination of mass spectrum from NpI/Hg2+-induced trace product displayed at m/z of 377.1962, which was consistent with the intermediate 1 (m/z calc = 376.18). Based on above analyses, the plausible probe mechanism was described by the reduction of the isonitrile moiety to primary amine as a chemical reaction-type process.
DFT theoretical calculations were also performed to further elucidate the above experimental findings, the optimized electronic structural models of precursor 1, and NpI calculated at B3LYP/6-31G (d,p) level of theory using Gaussian16. Calculated electron density surfaces of HOMO/LUMO of intermediate 1 and NpI are displayed in Figure 6. As revealed by relative energies and electron density, HOMO electron of precursor 1 focused on the amino and naphthalene core, and the marked conjugation bridge was established between two phenyl groups of naphthalene units, LUMO electron of precursor 1 distributed mainly in the scaffold of naphthalene, which can lead to a smaller energy gap between HOMO and LUMO (4.28 eV). This theoretically supports that the intramolecular charge transfer (ICT) process had tremendous importance due to the electron-donating amino group. However, as for NpI, the ICT effect seems much weaker and the electron bridge was not observed in its HOMO. Our DFT calculations reveal that the larger HOMO-LUMO energy gap was formed as the result of poor electronic distribution due to the electron-withdrawing isocyano group.
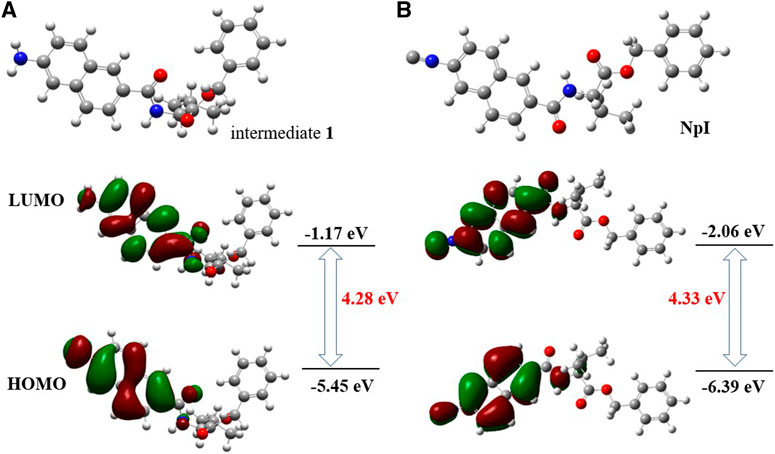
FIGURE 6. Calculated DFT energy and frontier molecular orbital diagrams of (A) precursor 1 and (B) NpI.
Applications in Living Cells and Real Water Samples
The good fluorescence property of NpI prompted us to check the potential application in a living system, in addition, NpI-containing valine residue as chemosensor may be applied to the imaging of intracellular Hg2+ due to its known biocompatibility. As shown in Figure 7, the bioimaging application of NpI to detect intracellular Hg2+ ion was observed by fluorescence confocal images of MCF-7 cells (human breast adenocarcinoma cell line). The MCF-7 cells were incubated with free probe NpI (10 µM) for 1 h and showed no detectable fluorescence. After further incubating NpI-stained MCF-7 cells with Hg2+ ion (50 µM) for another 1 h, a weak blue emission was initially noticed. These fluorescent imaging experimental results demonstrate that dipeptidomimetic NpI has the capability to visualize intracellular Hg2+ ion for biological samples.
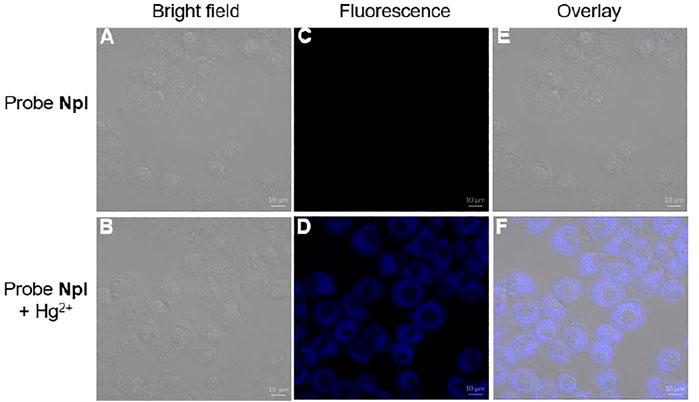
FIGURE 7. Fluorescent confocal bioimaging of MCF-7 cells: (A, C, E) cells incubated with free probe NpI, (B, D, F) images were captured after 30 min of treatment with probe NpI and Hg2+, respectively. (A, B) bright-field images, (C, D) fluorescence images, (E, F) merged images.
The potential of probe NpI to detection of Hg2+ in real water samples were demonstrated, including tap water, river water (Shangqiu Yellow River Old Riverway) and lake water (Shangqiu South Lake). The collected water samples were filtered (0.22 mm filter) and the pH were adjusted to 7.4 using a phosphate buffer. Each pretreated sample was mixed with an equal volume of DMSO and then spiked with different concentrations of Hg2+. Then 10 μM of probe was added into each sample and the fluorescence spectrum was measured. As shown in Table 1, the calculated recoveries ranging from 93.9% to 105.8%, the relative standard deviations (RSDs) are less than 6.4%, and the relative error is less than 6.5%. The performance of our synthesized probe NpI identified for selective Hg2+ detection was also compared with some preciously reported fluorescent Hg2+ chemosensors (Supplementary Table S1). It could be seen that the proposed probe exhibits comparable or superior analytical performances in water as compared to some of earlier reported probes. These observations indicated that probe NpI can be potentially utilized in environmental samples.
Conclusion
In conclusion, we have developed a new NpI fluorescent chemodosimeter for the rapid detection of the Hg2+ ion. Dipeptidomimetic NpI displayed distinct turn-on fluorescence alteration toward Hg2+ in aqueous solution and can act as a highly sensitive sensor for the real-time analysis. Besides that, NpI exhibited fast fluorescence response and low detection limit of 14.2 nM. Furthermore, the probing mechanism of NpI was proposed as Hg2+-assisted hydration conversion of isocyano group to amino groups and was verified by NMR spectroscopy, MS analysis, and DFT calculation. It is worth mentioning that dipeptidomimetic NpI successfully penetrated the cell membrane and was efficiently used for fluorescence confocal bioimaging of Hg2+ ion in MCF-7 cells. Real water sample measurements further demonstrated the practical utility of this probe for environmental applications.
Data Availability Statement
The original contributions presented in the study are included in the article/Supplementary Material, further inquiries can be directed to the corresponding authors.
Author Contributions
G-WL conceived the project and supervised the study. X-JW constructed the workflow and completed the paper. Y-PC and Q-LS conducted the experiments and characterized the samples. All authors listed have made a substantial, direct and intellectual contribution to the work, and approved it for publication.
Funding
The project was financially co-supported by the National Natural Science Foundation of China (21804086, U1504207, 21804085). We are also grateful for the Young Core Instructor Project from the Higher Education Institutions of Henan Province (2020GGJS203).
Conflict of Interest
The authors declare that the research was conducted in the absence of any commercial or financial relationships that could be construed as a potential conflict of interest.
Publisher’s Note
All claims expressed in this article are solely those of the authors and do not necessarily represent those of their affiliated organizations, or those of the publisher, the editors and the reviewers. Any product that may be evaluated in this article, or claim that may be made by its manufacturer, is not guaranteed or endorsed by the publisher.
Supplementary Material
The Supplementary Material for this article can be found online at: https://www.frontiersin.org/articles/10.3389/fchem.2022.813108/full#supplementary-material
References
Adamoczky, A., Nagy, L., Nagy, M., Zsuga, M., and Kéki, S. (2020). Conversion of Isocyanide to Amine in the Presence of Water and Hg(II) Ions: Kinetics and Mechanism as Detected by Fluorescence Spectroscopy and Mass Spectrometry. Ijms 21, 5588. doi:10.3390/ijms21155588
Banerjee, A., Sahana, A., Das, S., Lohar, S., Guha, S., Sarkar, B., et al. (2012). A Naphthalene Exciplex Based Al3+ Selective On-type Fluorescent Probe for Living Cells at the Physiological pH Range: Experimental and Computational Studies. Analyst 137, 2166–2175. doi:10.1039/c2an16233d
Bera, K., Das, A. K., Nag, M., and Basak, S. (2014). Development of a Rhodamine-Rhodanine-Based Fluorescent Mercury Sensor and its Use to Monitor Real-Time Uptake and Distribution of Inorganic Mercury in Live Zebrafish Larvae. Anal. Chem. 86, 2740–2746. doi:10.1021/ac404160v
Boyarskiy, V. P., Bokach, N. A., Luzyanin, K. V., and Kukushkin, V. Y. (2015). Metal-Mediated and Metal-Catalyzed Reactions of Isocyanides. Chem. Rev. 115, 2698–2779. doi:10.1021/cr500380d
Carter, K. P., Young, A. M., and Palmer, A. E. (2014). Fluorescent Sensors for Measuring Metal Ions in Living Systems. Chem. Rev. 114, 4564–4601. doi:10.1021/cr400546e
Celo, V., Lean, D. R. S., and Scott, S. L. (2006). Abiotic Methylation of Mercury in the Aquatic Environment. Sci. Total Environ. 368, 126–137. doi:10.1016/j.scitotenv.2005.09.043
Chakrabarty, S., Choudhary, S., Doshi, A., Liu, F.-Q., Mohan, R., Ravindra, M. P., et al. (2014). Catalytic Isonitrile Insertions and Condensations Initiated by RNC-X Complexation. Adv. Synth. Catal. 356, 2135–2196. doi:10.1002/adsc.201400017
Chang, L. L., Gao, Q., Liu, S., Hu, C. C., Zhou, W. J., and Zheng, M. M. (2018). Selective and Differential Detection of Hg2+ and Cu2+ with Use of a Single Rhodamine Hydrazone-type Probe in the Absence and Presence of UV Irradiation. Dyes Pigm. 153, 117–124. doi:10.1016/j.dyepig.2018.02.013
Chen, G., Guo, Z., Zeng, G., and Tang, L. (2015). Fluorescent and Colorimetric Sensors for Environmental Mercury Detection. Analyst 140, 5400–5443. doi:10.1039/c5an00389j
Chen, S., Pang, C., Chen, X., Yan, Z., Huang, S., Li, X., et al. (2019). Research Progress in Design, Synthesis and Application of Multifunctional Fluorescent Probes. Chin. J. Org. Chem. 39, 1846–1857. doi:10.6023/cjoc201901033
Chen, S., Wang, W., Yan, M., Tu, Q., Chen, S.-W., Li, T., et al. (2018). 2-Hydroxy Benzothiazole Modified Rhodol: Aggregation-Induced Emission and Dual-Channel Fluorescence Sensing of Hg2+ and Ag+ Ions. Sensors Actuators B: Chem. 255, 2086–2094. doi:10.1016/j.snb.2017.09.008
Chen, Y., Zhang, W., Cai, Y., Kwok, R. T. K., Hu, Y., Lam, J. W. Y., et al. (2017). AIEgens for Dark Through-Bond Energy Transfer: Design, Synthesis, Theoretical Study and Application in Ratiometric Hg2+ Sensing. Chem. Sci. 8, 2047–2055. doi:10.1039/c6sc04206f
Das, A. K., and Goswami, S. (2017). 2-Hydroxy-1-naphthaldehyde: A Versatile Building Block for the Development of Sensors in Supramolecular Chemistry and Molecular Recognition. Sensors Actuators B: Chem. 245, 1062–1125. doi:10.1016/j.snb.2017.01.068
Das, S., Dutta, M., and Das, D. (2013). Fluorescent Probes for Selective Determination of Trace Level Al3+: Recent Developments and Future Prospects. Anal. Methods 5, 6262–6285. doi:10.1039/c3ay40982a
Driscoll, C. T., Mason, R. P., Chan, H. M., Jacob, D. J., and Pirrone, N. (2013). Mercury as a Global Pollutant: Sources, Pathways, and Effects. Environ. Sci. Technol. 47, 4967–4983. doi:10.1021/es305071v
Duan, Q., Lv, X., Liu, C., Geng, Z., Zhang, F., Sheng, W., et al. (2019). Dichlororesorufin-Based Colorimetric and Fluorescent Probe for Ultrasensitive Detection of Mercury Ions in Living Cells and Zebrafish. Ind. Eng. Chem. Res. 58, 11–17. doi:10.1021/acs.iecr.8b04386
Hatai, J., Pal, S., Jose, G. P., and Bandyopadhyay, S. (2012). Histidine Based Fluorescence Sensor Detects Hg2+ in Solution, Paper Strips, and in Cells. Inorg. Chem. 51, 10129–10135. doi:10.1021/ic300530f
Kajitani, T., Okoshi, K., Sakurai, S.-i., Kumaki, J., and Yashima, E. (2006). Helix-sense Controlled Polymerization of a Single Phenyl Isocyanide Enantiomer Leading to Diastereomeric Helical Polyisocyanides with Opposite helix-sense and Cholesteric Liquid Crystals with Opposite Twist-Sense. J. Am. Chem. Soc. 128, 708–709. doi:10.1021/ja0576536
Kim, H. N., Ren, W. X., Kim, J. S., and Yoon, J. (2012). Fluorescent and Colorimetric Sensors for Detection of lead, Cadmium, and Mercury Ions. Chem. Soc. Rev. 41, 3210–3244. doi:10.1039/c1cs15245a
Li, G.-W., Cao, J.-M., Zong, W., Hu, L., Hu, M.-L., Lei, X., et al. (2017). Helical Polyisocyanopeptides as Lyotropic Liquid Crystals for Measuring Residual Dipolar Couplings. Chem. Eur. J. 23, 7653–7656. doi:10.1002/chem.201700539
Li, G.-W., Liu, H., Qiu, F., Wang, X.-J., and Lei, X.-X. (2018). Residual Dipolar Couplings in Structure Determination of Natural Products. Nat. Prod. Bioprospect. 8, 279–295. doi:10.1007/s13659-018-0174-x
Lu, H., Xiong, L., Liu, H., Yu, M., Shen, Z., Li, F., et al. (2009). A Highly Selective and Sensitive Fluorescent Turn-On Sensor for Hg2+ and its Application in Live Cell Imaging. Org. Biomol. Chem. 7, 2554–2558. doi:10.1039/b902912e
Lü, Y., Sun, Q., Hu, B., Chen, X., Miao, R., and Fang, Y. (2016). Synthesis and Sensing Applications of a New Fluorescent Derivative of Cholesterol. New J. Chem. 40, 1817–1824. doi:10.1039/c5nj02601f
Nagy, M., Kovács, S. L., Nagy, T., Rácz, D., Zsuga, M., and Kéki, S. (2019). Isocyanonaphthalenes as Extremely Low Molecular Weight, Selective, Ratiometric Fluorescent Probes for Mercury(II). Talanta 201, 165–173. doi:10.1016/j.talanta.2019.04.007
Oliveira, E., Genovese, D., Juris, R., Zaccheroni, N., Capelo, J. L., Raposo, M. M. M., et al. (2011). Bioinspired Systems for Metal-Ion Sensing: New Emissive Peptide Probes Based on Benzo[d]oxazole Derivatives and Their Gold and Silica Nanoparticles. Inorg. Chem. 50, 8834–8849. doi:10.1021/ic200792t
Oliveira, E., Lorenzo, J., Cid, A., Capelo, J. L., and Lodeiro, C. (2013). Non-toxic Fluorescent Alanine-Fluorescein Probe with green Emission for Dual Colorimetric/fluorimetric Sensing. J. Photochem. Photobiol. A: Chem. 269, 17–26. doi:10.1016/j.jphotochem.2013.06.015
Ong, S. Y., Zhang, C., Dong, X., and Yao, S. Q. (2021). Recent Advances in Polymeric Nanoparticles for Enhanced Fluorescence and Photoacoustic Imaging. Angew. Chem. Int. Ed. 60, 17797–17809. doi:10.1002/anie.202101964
Pannipara, M., Al-Sehemi, A. G., Irfan, A., Assiri, M., Kalam, A., and Al-Ammari, Y. S. (2018). AIE Active Multianalyte Fluorescent Probe for the Detection of Cu2+, Ni2+ and Hg2+ Ions. Spectrochimica Acta A: Mol. Biomol. Spectrosc. 201, 54–60. doi:10.1016/j.saa.2018.04.052
Puthiyedath, T., and Bahulayan, D. (2018). A Click Derived Triazole-Coumarin Derivative as Fluorescence On-Off PET Based Sensor for Ca2+and Fe3+ Ions. Sensors Actuators B: Chem. 272, 110–117. doi:10.1016/j.snb.2018.05.126
Reller, M., Wesp, S., Koos, M. R. M., Reggelin, M., and Luy, B. (2017). Biphasic Liquid crystal and the Simultaneous Measurement of Isotropic and Anisotropic Parameters by Spatially Resolved NMR Spectroscopy. Chem. Eur. J. 23, 13351–13359. doi:10.1002/chem.201702126
Roek, D. P., Chateauneuf, J. E., and Brennecke, J. F. (2000). A Fluorescence Lifetime and Integral Equation Study of the Quenching of Naphthalene Fluorescence by Bromoethane in Super- and Subcritical Ethane. Ind. Eng. Chem. Res. 39, 3090–3096. doi:10.1021/ie000057u
Ru, J.-X., Guan, L.-P., Tang, X.-L., Dou, W., Yao, X., Chen, W.-M., et al. (2014). Turn-on Phosphorescent Chemodosimeter for Hg2+ Based on a Cyclometalated Ir(III) Complex and its Application in Time-Resolved Luminescence Assays and Live Cell Imaging. Inorg. Chem. 53, 11498–11506. doi:10.1021/ic501417s
Sadjadi, S., Heravi, M. M., and Nazari, N. (2016). Isocyanide-based Multicomponent Reactions in the Synthesis of Heterocycles. RSC Adv. 6, 53203–53272. doi:10.1039/c6ra02143c
Samonina-Kosicka, J., DeRosa, C. A., Morris, W. A., Fan, Z., and Fraser, C. L. (2014). Dual-Emissive Difluoroboron Naphthyl-Phenyl β-Diketonate Polylactide Materials: Effects of Heavy Atom Placement and Polymer Molecular Weight. Macromolecules 47, 3736–3746. doi:10.1021/ma5006606
Sarkar, P. K., Polley, N., Chakrabarti, S., Lemmens, P., and Pal, S. K. (2016). Nanosurface Energy Transfer Based Highly Selective and Ultrasensitive “Turn on” Fluorescence Mercury Sensor. ACS Sens. 1, 789–797. doi:10.1021/acssensors.6b00153
Selvaraj, M., Rajalakshmi, K., Ahn, D.-H., Yoon, S.-J., Nam, Y.-S., Lee, Y., et al. (2021). Tetraphenylethene-based Fluorescent Probe with Aggregation-Induced Emission Behavior for Hg2+ Detection and its Application. Analytica Chim. Acta 1148, 238178. doi:10.1016/j.aca.2020.12.053
Shu, W., Yan, L., Liu, J., Wang, Z., Zhang, S., Tang, C., et al. (2015). Highly Selective Fluorescent Probe for the Sensitive Detection of Inorganic and Organic Mercury Species Assisted by H2O2. Ind. Eng. Chem. Res. 54, 8056–8062. doi:10.1021/acs.iecr.5b02153
Sie, Y.-W., Wan, C.-F., and Wu, A.-T. (2017). A Multifunctional Schiff Base Fluorescence Sensor for Hg2+, Cu2+ and Co2+ Ions. RSC Adv. 7, 2460–2465. doi:10.1039/c6ra24704k
Singha, S., Jun, Y. W., Sarkar, S., and Ahn, K. H. (2019). An Endeavor in the Reaction-Based Approach to Fluorescent Probes for Biorelevant Analytes: Challenges and Achievements. Acc. Chem. Res. 52, 2571–2581. doi:10.1021/acs.accounts.9b00314
Song, B., and Xu, B. (2017). Metal-catalyzed C-H Functionalization Involving Isocyanides. Chem. Soc. Rev. 46, 1103–1123. doi:10.1039/c6cs00384b
Su, W., Yuan, S., and Wang, E. (2017). A Rhodamine-Based Fluorescent Chemosensor for the Detection of Pb2+, Hg2+ and Cd2+. J. Fluoresc. 27, 1871–1875. doi:10.1007/s10895-017-2124-0
Tang, X., Han, J., Wang, Y., Ni, L., Bao, X., Wang, L., et al. (2017). A Multifunctional Schiff Base as a Fluorescence Sensor for Fe3+ and Zn2+ Ions, and a Colorimetric Sensor for Cu2+ and Applications. Spectrochimica Acta Part A: Mol. Biomol. Spectrosc. 173, 721–726. doi:10.1016/j.saa.2016.10.028
Thomas, S. W., Joly, G. D., and Swager, T. M. (2007). Chemical Sensors Based on Amplifying Fluorescent Conjugated Polymers. Chem. Rev. 107, 1339–1386. doi:10.1021/cr0501339
Vavsari, V. F., Shakeri, P., and Balalaie, S. (2020). Application of Chiral Isocyanides in Multicomponent Reactions. Coc 24, 162–183. doi:10.2174/1385272824666200110095120
Wang, K., Li, J., Ji, S., Li, L., Qiu, Z., Pan, C., et al. (2018a). Fluorescence Probes Based on AIE Luminogen: Application for Sensing Hg2+ in Aqueous media and Cellular Imaging. New J. Chem. 42, 13836–13846. doi:10.1039/c8nj02245c
Wang, L., Qu, Y., Yang, Y., Cao, J., and Wang, L. (2018b). A Tetrasulphite-Containing Fluorescent Chemodosimeter for Hg2+ with Optimized Selectivity towards Ag+. Sensors Actuators B: Chem. 269, 70–78. doi:10.1016/j.snb.2018.04.099
Wang, Q., Chu, B.-F., Chu, J.-H., Liu, N., and Wu, Z.-Q. (2018c). Facile Synthesis of Optically Active and Thermoresponsive star Block Copolymers Carrying Helical Polyisocyanide Arms and Their Thermo-Triggered Chiral Resolution Ability. ACS Macro Lett. 7, 127–131. doi:10.1021/acsmacrolett.7b00875
Wang, S., Lin, B., Chen, L., Li, N., Xu, J., Wang, J., et al. (2018d). Branch-migration Based Fluorescent Probe for Highly Sensitive Detection of Mercury. Anal. Chem. 90, 11764–11769. doi:10.1021/acs.analchem.8b03547
Wu, D., Sedgwick, A. C., Gunnlaugsson, T., Akkaya, E. U., Yoon, J., and James, T. D. (2017). Fluorescent Chemosensors: the Past, Present and Future. Chem. Soc. Rev. 46, 7105–7123. doi:10.1039/c7cs00240h
Yamamoto, Y. (1980). Zerovalent Transition Metal Complexes of Organic Isocyanides. Coord. Chem. Rev. 32, 193–233. doi:10.1016/s0010-8545(00)80375-6
Yoon, S., Albers, A. E., Wong, A. P., and Chang, C. J. (2005). Screening Mercury Levels in Fish with a Selective Fluorescent Chemosensor. J. Am. Chem. Soc. 127, 16030–16031. doi:10.1021/ja0557987
Zhang, B., and Studer, A. (2015). Recent Advances in the Synthesis of Nitrogen Heterocycles via Radical cascade Reactions Using Isonitriles as Radical Acceptors. Chem. Soc. Rev. 44, 3505–3521. doi:10.1039/c5cs00083a
Zhang, S., Wu, X., Niu, Q., Guo, Z., Li, T., and Liu, H. (2017). Highly Selective and Sensitive Colorimetric and Fluorescent Chemosensor for Rapid Detection of Ag+, Cu2+ and Hg2+ Based on a Simple Schiff Base. J. Fluoresc. 27, 729–737. doi:10.1007/s10895-016-2005-y
Zhang, X., Evanno, L., and Poupon, E. (2020). Biosynthetic Routes to Natural Isocyanides. Eur. J. Org. Chem. 2020, 1919–1929. doi:10.1002/ejoc.201901694
Zhang, X., Shi, W., Chen, X., and Xie, Z. (2018). Isocyano-functionalized, 1,8-Naphthalimide-Based Chromophore as Efficient Ratiometric Fluorescence Probe for Hg2+ in Aqueous Medium. Sensors Actuators B: Chem. 255, 3074–3084. doi:10.1016/j.snb.2017.09.132
Zhang, Y., Shi, B., Zhang, P., Huo, J., Chen, P., Lin, Q., et al. (2013). A Highly Selective Dual-Channel Hg2+ Chemosensor Based on an Easy to Prepare Double Naphthalene Schiff Base. Sci. China Chem. 56, 612–618. doi:10.1007/s11426-012-4798-0
Zhao, Y., Sun, Y., Lv, X., Liu, Y., Chen, M., and Guo, W. (2010). Rhodamine-based Chemosensor for Hg2+ in Aqueous Solution with a Broad pH Range and its Application in Live Cell Imaging. Org. Biomol. Chem. 8, 4143–4147. doi:10.1039/c0ob00013b
Keywords: dipeptidomimetic, isocyanonaphthalene, mercury ion, fluorescent probe, cell imaging
Citation: Wang X-J, Li G-W, Cheng Y-P, Sun Q-L, Hao Y-Q, Wang C-H and Liu L-T (2022) Design and Synthesis of Dipeptidomimetic Isocyanonaphthalene as Enhanced-Fluorescent Chemodosimeter for Sensing Mercury Ion and Living Cells. Front. Chem. 10:813108. doi: 10.3389/fchem.2022.813108
Received: 12 November 2021; Accepted: 24 January 2022;
Published: 04 March 2022.
Edited by:
Yao Sun, Central China Normal University, ChinaCopyright © 2022 Wang, Li, Cheng, Sun, Hao, Wang and Liu. This is an open-access article distributed under the terms of the Creative Commons Attribution License (CC BY). The use, distribution or reproduction in other forums is permitted, provided the original author(s) and the copyright owner(s) are credited and that the original publication in this journal is cited, in accordance with accepted academic practice. No use, distribution or reproduction is permitted which does not comply with these terms.
*Correspondence: Gao-Wei Li, ligaowei@sqnu.edu.cn; Yuan-Qiang Hao, hao0736@163.com