- 1Jiangxi Key Laboratory of Organic Chemistry, Jiangxi Science and Technology Normal University, Nanchang, China
- 2College of Chemical Engineering, Shijiazhuang University, Shijiazhuang, China
- 3School of Chemistry and Chemical Engineering, Jiangxi Science and Technology Normal University, Nanchang, China
A series of carbazole-containing gold(I) complexes modified with different substituents were successfully designed and synthesized, and their molecular structures were characterized by nuclear magnetic resonance spectroscopy and mass spectrometry. The aggregation-induced behaviors of these gold(I) complexes were studied by ultraviolet/visible and photoluminescence spectroscopy. Meanwhile, their mechanical force-responsive emissive properties were also investigated via solid-state photoluminescence spectroscopy. Interestingly, all these gold(I)-based luminogenic molecules were capable of exhibiting aggregation-induced phosphorescent emission phenomena. Furthermore, their solids of three gold(I) complexes displayed contrasting mechano-responsive phosphorescence features. More specifically, trifluoromethyl or methoxyl-substituted luminophores 1 and 3 demonstrated mechanochromic behaviors involving blue-shifted phosphorescence changes, and their mechanoluminochromic phenomena were reversible. However, the solid-state phosphorescence of phenyl-substituted luminophor 2 was not sensitive to external mechanical force.
Introduction
For more than a decade, investigators in chemistry, biology, and materials science have paid more and more attention to construct photochemical/physical materials for highly efficient utilization of lights in various fields, such as solar cells, fluorescent switches, data storage, bio-applications, and photodynamic/thermal therapy (Ni et al., 2018; Ye et al., 2019; Lv et al., 2020). In particular, mechanochromic (MC) luminescence materials that display a solid-state luminous color change upon external force stimulus are a class of important functionalized smart materials, and MC metal complexes are promising candidates of this type of materials. Two key factors for developing high-efficiency MC materials are bright aggregative-state luminescence and high color contrast before and after grinding (Zhang J. et al., 2021; Yang W. et al., 2020; Dong et al., 2020; Ding et al., 2013; Wang et al., 2022). However, their light emissions of traditional luminescent dyes dramatically decrease upon aggregation due to the notorious aggregation-caused quenching (ACQ) effect, which largely limits the effective applications of emissive materials (Leung et al., 2013; Xue et al., 2016; Shi et al., 2018; Huang G. et al., 2019; Echeverri et al., 2020; Liu et al., 2021; Ge et al., 2022). Fortunately, in 2001, Tang et al. introduced an intriguing phenomenon that was known as aggregation-induced emission (AIE) (Mei et al., 2015; Chen et al., 2016; Qian and Tang, 2017; Kunitski et al., 2019; Yang et al., 2020b; Yin et al., 2021a; Sun et al., 2021). Subsequently, Park et al. reported aggregation-induced emission enhancement (AIEE) effect in 2012. Remarkably, luminogens with AIE or AIEE properties possess anti-ACQ characteristics (Dong et al., 2009; An et al., 2012; Gopikrishna et al., 2018). In the recent twenty years, AIE or AIEE luminogenic molecules have been proved to be valuable emitters for exploiting high-performance stimuli-responsive luminescent materials (Wu et al., 2021). Up till now, the majority of molecules simultaneously displaying AIE and mechanoluminochromic behaviors have been reported to be pure organic compounds (Tong et al., 2014; Li W. et al., 2017; Huang L. L. et al., 2019; Yin et al., 2021b; Zhang X. et al., 2021). In contrast, the number of metal-organic complexes possessing these noteworthy properties remains inadequate. In comparison with organic luminescent compounds, metal-containing complexes are more conducive to realizing phosphorescent emission (Zhang et al., 2014; Zhang et al., 2022). In the last 20 years, gold(I) complexes have aroused the attention of many investigators because of the presence of the fascinating intramolecular and intermolecular aurophilic interactions. Indeed, some gold(I) complexes with rich photophysical natures have been discovered. For instance, in 2008, Ito et al. reported the first example of gold(I) complex possessing reversible MC luminescence behavior (Ito et al., 2008). In 2014, Chen et al. developed the first example of gold(I) complex with aggregation-induced white light-emitting feature (Chen et al., 2014). Next, in 2015, Chen et al. also reported the first example of AIE-active gold(I) complex with crystallization-induced emission enhancement and reversible mechanochromic behaviors (Chen et al., 2015). In 2019, Wang et al. discovered the first example of an excitation wavelength-dependent nearly pure white-emissive gold(I)-containing crystal material (Wang et al., 2019). More importantly, gold(I) complexes are capable of emitting long-lived room-temperature phosphorescence, which is very significative for the exploitation of high-performance MC light-emitting materials (Chen et al., 2017). In this work, we described three novel carbazole-modified mononuclear gold(I) complexes with different substituents involving trifluoromethylphenyl, phenyl and methoxyphenyl (Figure 1), and all the three gold(I) complexes showed aggregation-induced phosphorescent emission behaviors with long lifetimes in the microsecond range in aggregated states, which were longer than the lifetimes of most pure organic AIEgens. Additionally, most luminescent mechanochromic compounds showed red-shifted emission upon application of a mechanical force, and there have only been a few reports of materials exhibiting blue-shifted emission upon mechanical stimulation. It is worth noting that the trifluoromethyl or methoxyl-substituted mononuclear gold(I) complexes 1 and 3 showed hypsochromic MC phosphorescence phenomena, while no MC phenomenon was observed for phenyl-substituted mononuclear gold(I) complex 2.
Materials and methods
Experimental
General
All reagent were carried out by using standard Schlenk techniques under an argon atmosphere, unless otherwise stated. CH2Cl2 was dried with CaH2 then distilled. THF was dried with Na and then distilled. All starting materials were obtained commercially as analytical-grade and used without further purification. Using tetramethylsilane (TMS) as an internal standard, 1H NMR (500 MHz) spectra were measured on American Varian Mercury Plus 500 spectrometer (500 MHz) in deuterated solvents and analyzed at room temperature using the Bruker NMR software package-TopSpin. 1H NMR chemical shifts were referenced to the residual solvent signal (7.26 ppm for CDCl3), and 19F NMR chemical shifts are relative to C6F6 (δ = −163.00). Mass spectra (MS) were recorded by Bruker AmaZon SL Ion Trap Mass spectrometer. Fluorescence spectra were conducted by Hitachi F-4600 fluorescence spectrophotometer and the Edinburgh FLS1000 instrument with an integrating sphere. The UV-vis absorption spectra were obtained on an Agilent 8454 UV-Vis spectrophotometer. The X-ray diffraction (XRD) patterns of complexes 1 and 3 in different solid states were recorded by Shimadzu XRD-6000 diffractometer using Ni-filtered and graphite-monochromated Cu Kα radiation (λ = 1.54 Å, 40 kV, 30 mA). Dynamic light scattering (DLS) measurements were characterized by NanoBrook 90 Plus (Brookhaven Instruments). OriginLab OriginPro 9.0 software package was used for spectral processing. All reactions using monitored by precoated TLC plates under UV light at 254 nm.
Synthesis
Synthesis of monoisonitrile ligands I-3, II-3 and III-3
Monoisonitrile ligands I-3, II-3 and III-3 were synthesized in a similar manner (Zhao et al., 2019). The synthesis of compound I-3 was taken as an example: the mixture of compound I-1 (5.46 g, 10 mmol) and formic acid (80 ml) was stirred overnight at 110°C. After completion of the reaction, formic acid was removed from the reaction system by distillation, and the residual mixture was extracted with methylene chloride (5 × 60 ml). The organic layer was washed with brine, dried over Na2SO4, and concentrated in vacuum to give the product I-2 (white solid). Next, the corresponding CH2Cl2 solution (50 ml) of I-2 and triethylamine (5 ml) was cooled to 0°C. Triphosgene (3.26 g, 11 mmol) of CH2Cl2 solution (30 ml) was added to the mixture drop by drop and then refluxed for 3 h. After the reaction was completed, the mixture was cooled to room temperature. 10% Na2CO3 solution (80 ml) was dropped to the system for neutralization. The mixture was extracted with trichloromethane (3 × 50 ml), the organic layer was washed with brine, dried over Na2SO4, and concentrated in vacuum. The residue was purified by column chromatography and the expected white isonitrile ligand I-3 was obtained in 70% yield.
Synthesis of gold(I) chloride complexes I-4, II-4 and III-4
The synthesis of gold(I) chloride complexes I-4, II-4 and III-4 were prepared according to the procedure described in reference (Seki et al., 2016).
Synthesis of complexes 1-3
As displayed in Scheme 1, the synthesis of complex 1 was taken as an example: gold(I) chloride complex I-4 (753.1 mg, 1 mmol) was added to a dried reaction flask and dried with three cycles of vacuum/argon. Then, organic zinc reagent (554.7 mg, 1.5 mmol) was dissolved in anhydrous THF (50 ml) at 0°C and stirred for 12 h. At the end of the reaction, the mixture was quenched with phosphate buffer solution (PBS) and extracted three times with CH2CH2. The organic phase was dried with sodium sulfate, and the solvent was removed in vacuo. Finally, the expected white solid products 1 was obtained by flash column chromatography with yield of 65%. 1: 1H NMR (500 MHz, CDCl3) δ 8.43 (s, 2H), 8.14 (d, J = 10.0 Hz, 1H), 7.93 (d, J = 5.0 Hz, 2H), 7.82 (s, 6H), 7.76 (m, J = 5.0, 6H), 7.58–7.54 (m, 4H), 3.95 (s, 1H), 3.88 (s, 3H). ESI-MS (m/z): ESI-MS (m/z): [M + H]+ of C41H25AuF6N2O2: 889.1564 (calcd), 889.1570 (found). 2: Yield: 71%. 1H NMR (500 MHz, CDCl3) δ 8.40 (s, 2H), 7.93 (d, J = 5.0 Hz, 2H), 7.83 (m, 4H), 7.72 (t, J = 7.5 Hz, 6H), 7.59 (d, J = 10.0 Hz, 2H), 7.53–7.49 (m, 6H), 7.38 (t, J = 7.5 Hz, 2H), 3.88 (s, 3H). ESI-MS (m/z): [M + H]+ of C39H27AuN2O2: 753.1816 (calcd), 758.1814 (found). 3: Yield: 71%. 1H NMR (500 MHz, CDCl3) δ 8.33 (s, 2H), 7.93 (d, J = 5.0 Hz, 2H), 7.81 (s, 3H), 7.66 (d, J = 5.0 Hz, 7H), 7.58 (d, J = 10.0 sHz, 2H), 7.51 (d, J = 10.0 Hz, 2H), 7.05 (d, J = 10.0 Hz, 4H), 3.89 (s, 9H). ESI-MS (m/z): ESI-MS (m/z): [M + H]+ of C41H31AuN2O4: 813.2028 (calcd), 813.2027 (found).
Results and discussion
Synthesis
The monoisonitrile ligands I-3, II-3 and III-3 were prepared according to the procedure described in reference (Seki et al., 2016). The complexes 1-3 were obtained in high yields and the strategies were presented in Scheme 1.
Aggregation-induced phosphorescent emission properties of complexes 1-3
In order to survey the aggregation-induced emission properties of complexes 1-3, the UV-vis absorption spectra with different water fractions (fW) were investigated in N, N-dimethylformamide (DMF)-H2O mixtures (Osawa et al., 2010; Li Y. et al., 2017). The results showed that the level-off tails appeared in the visible region with increasing fW, which could be attributed to the well-known Mie scattering effect and indicated the formation of nano-aggregates at the same time (Supplementary Figure S1).
Subsequently, the photoluminescence spectra of complexes 1-3 in DMF-H2O mixtures with varying water fractions were investigated. The solution of these complexes in pure DMF showed broad and Gaussian shaped emission peaks at around 420 nm, which might be originated from charge transfer (CT) states, rather than ligand-localized transitions. As shown in Figure 2, complex 1 emitted very weak blue emission in pure DMF under UV light excited at 365 nm (Hunks et al., 2000; Ishi-I et al., 2019). However, when the fW reached 40%, a new broad emission peak was detected at around 600 nm and the mixture showed obvious yellow emission. As the water fractions continuously increased to 70%, the emission intensity of complex 1 was also enhanced significantly. Clearly, the bright yellow emission of complex 1 could be attributed to the formation of nano-aggregates, which was further confirmed by dynamic light scattering (DLS) measurements in DMF-water mixtures with 90% volume fraction of water (Figure 3). Besides, the formation of aurophilic interactions after aggregating was also responsible for the yellow emission of complex 1. Decay curve gave its phosphorescence lifetime values as 0.97 μs in aggregated state (Supplementary Figure S2). These results indicated the aggregation-induced phosphorescent emission (AIPE) characteristic of complex 1. Similarly, bright yellow emissions could also be found until the water fractions increased to 50% for complexes 2 and 3. The microsecond lifetimes (1.03 μs for 2; 0.91 μs for 3) in aggregation proved their excellent AIPE properties. In addition, time resolved fluorescence data of 1-3 have been provided and discussed in this manuscript. Taking compound 1 as a representative example, the decay curve gave its phosphorescence lifetime values as 0.72 μs in aggregated state pure DMF at room temperature (quantum yield of 1%). The phosphorescence lifetime values of compound 2 was 0.47 μs (quantum yield of 0.2%). The phosphorescence lifetime values of compound 3 was 0.35 μs (quantum yield of 0.6%) (Supplementary Figure S3).
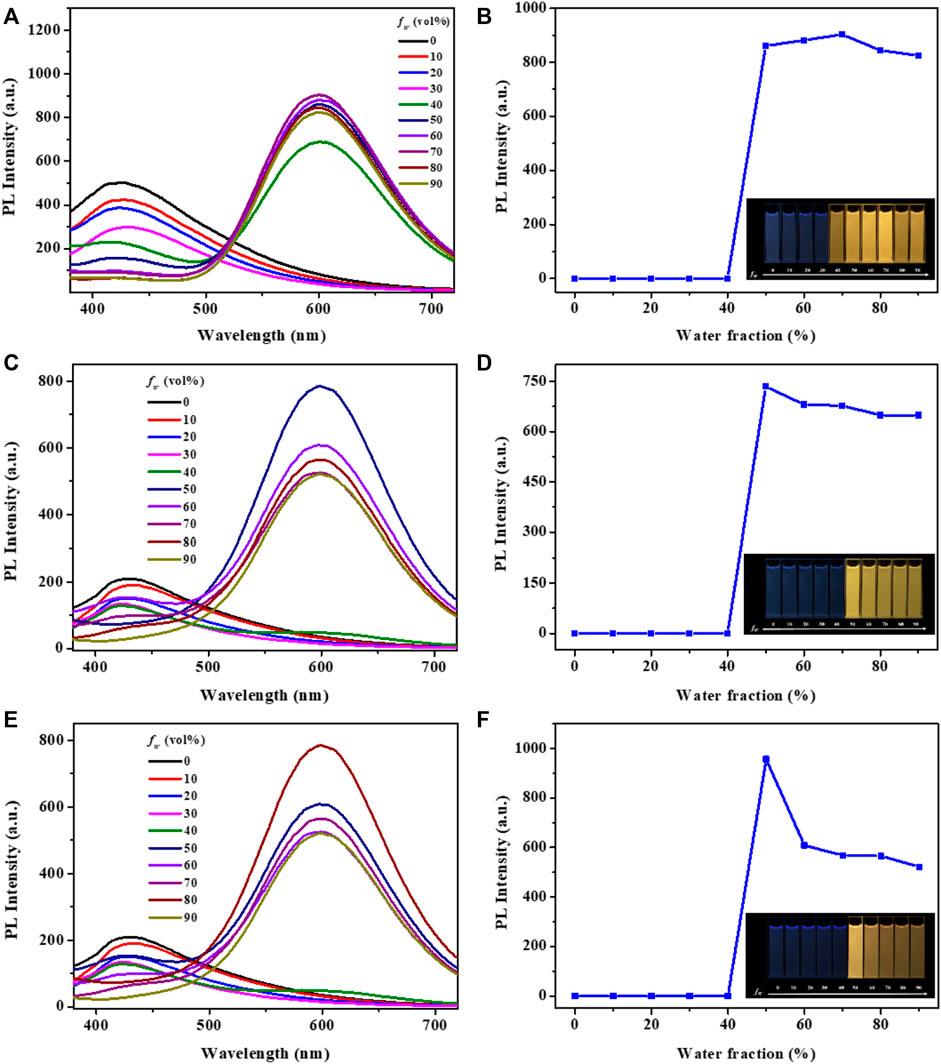
FIGURE 2. Fluorescence spectra of the complexes 1 (A), 2 (C), 3 (E) in DMF-H2O mixtures with various water fractions. Changes in emission intensity of complexes 1 (B), 2 (D), 3 (F) in DMF-H2O mixtures with various water fractions. Inset: Fluorescence images of complexes 1 (B), 2 (D), 3 (F) in different DMF-H2O mixtures under 365 nm UV lamp (c: 2.0 × 10–5 mol L−1).
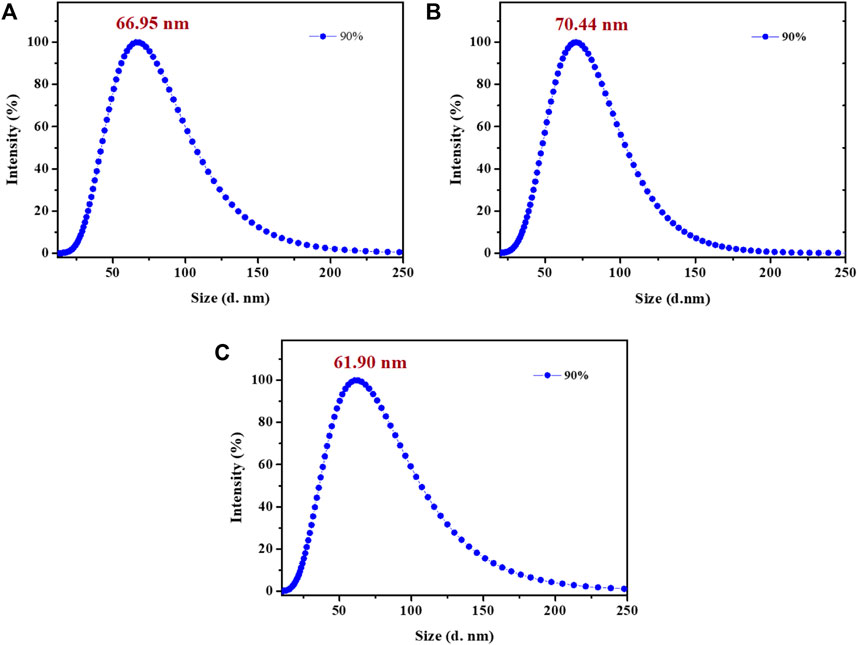
FIGURE 3. Size distribution curves of 1 (A), 2 (B) and 3 (C) in DMF-water mixtures with 90% volume fraction of water (Ex: 365 nm; c: 2.0 × 10–5 mol L−1).
Reversible phosphorescent mechanochromisms of complexes 1 and 3
Due to the AIPE characteristics of complexes 1-3, their solids also emitted orange or yellow photoluminescence with the emission peaks at 595 nm, 593 nm and 612 nm, respectively (Figure 4). Their microsecond lifetimes (1.17 μs for 1; 0.96 μs for 2; 0.43 μs for 3) indicated that the emissions of complexes 1-3 were originated from phosphorescences (Figure 5). Interestingly, these complexes exhibited various mechano-responsive behaviors (Chi et al., 2012). As displayed in Figure 4, the solid of complex 1 emitted bright orange phosphorescence with the quantum yield of 8%. After grinding, a blue-shifted emission band was observed at 582 nm and the orange color emission was converted to yellow emission. Treating the ground powder with CH2Cl2 for 30 s, the yellow emission solid returned to its original orange color emission. Furthermore, such mechanical stimulation induced luminescence conversion between orange and yellow could be repeated several times. These experiments indicated that complex 1 exhibited reversible hypsochromic phosphorescence mechanochromism. A similar stimuli-responsive phenomenon occurred in complex 3. Reversible conversion between orange-red phosphorescence and yellow phosphorescence could be realized by grinding and fuming. Nevertheless, the emission of complex 2 did not change under the mechanical stimulation. This might be due to the unchanged molecular arrangement of the solid 2 after grinding (Yang et al., 2020c; Zhao et al., 2012). What’s more, the absorption spectra of solids 1-3 in various states have been provided (Supplementary Figure S4).
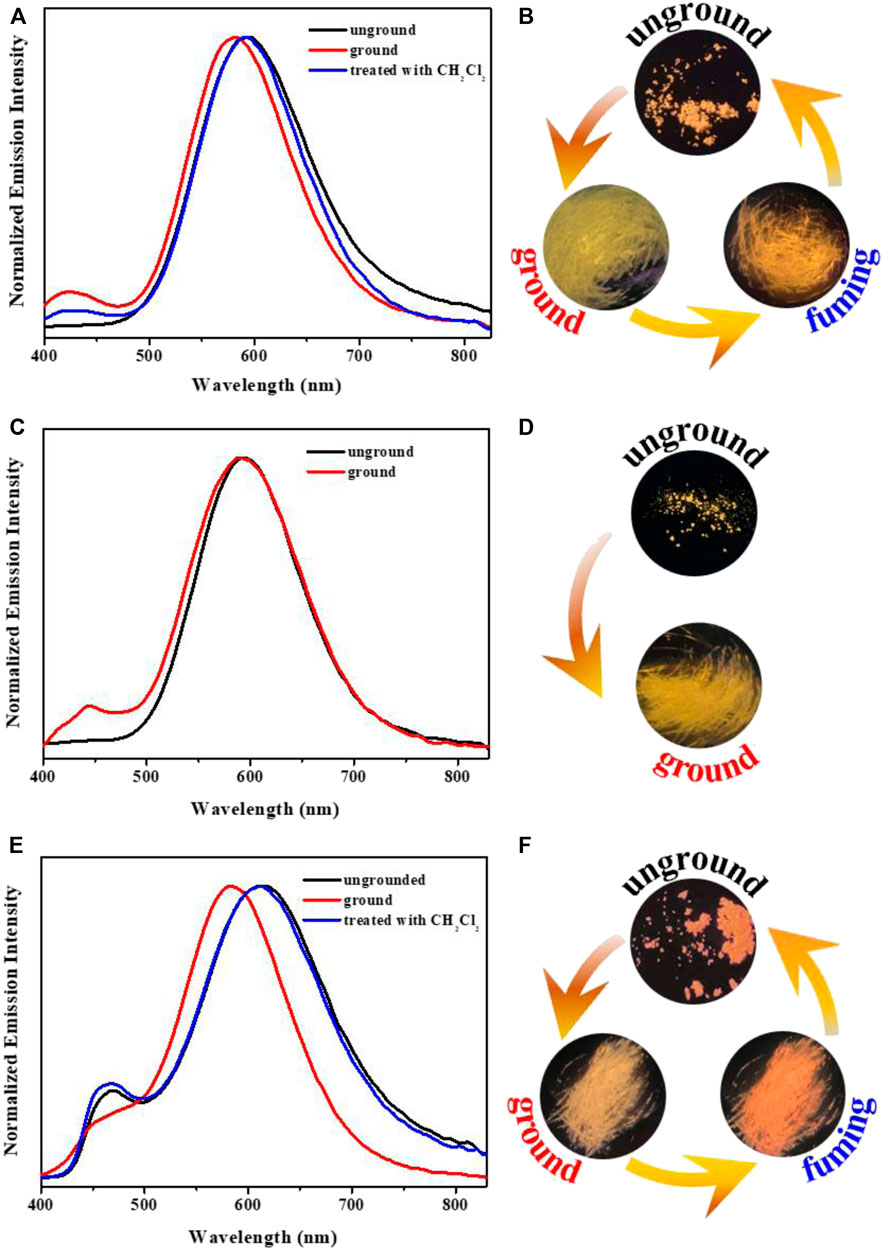
FIGURE 4. Solid-state PL spectra of complexes 1 (A), 2 (C), 3 (E) in various solid states; Fluorescence images of 1 (B), 2 (D), 3 (F) in different solid states.
In order to further explore the phosphorescence mechanochromisms of these complexes, the powder X-ray diffraction (PXRD) spectra were carried out. As displayed in Figure 6, the original solid samples of the complex 1 and 3 showed sharp and strong diffraction peaks, indicating the orderly crystalline states. On the contrary, the diffraction peaks decreased and even disappeared after grinding, which mean that the ground states were in amorphous states. As a matter of fact, the original strong diffraction peaks occurred again after fuming the ground solid with CH2Cl2. Therefore, the PXRD results revealed that the reversible crystalline-amorphous phase transformation was one of the main causes of the reversible hypsochromic phosphorescence mechanochromisms for complexes 1 and 3.
Conclusion
In summary, a series of carbazole-containing gold(I) complexes modified with different substituents were successfully designed and synthesized. These novel complexes displayed AIPE behaviors in DMF-water mixtures. We suspected that bright yellow emissions were due to the formation of aurophilic interactions in aggregated states. In solid states, the emission colors of these complexes could be regulated by different substituents. In addition, complexes 1 and 3 exhibited reversible blue-shifted phosphorescence mechanochromisms. The PXRD results revealed that the stimuli-response behaviors were resulted from the crystalline-amorphous phase transformation. This work would be beneficial to design novel AIPE-active metal-organic compounds with remarkable phosphorescent mechanochromisms.
Data availability statement
The original contributions presented in the study are included in the article/Supplementary Material, further inquiries can be directed to the corresponding authors.
Author contributions
ZC designed the project. ZC, X-WD, and X-YW wrote the manuscript. ZC, X-WD, X-YW, A-QW, and W-TL performed the syntheses of gold(I) complexes 1-3. ZC, X-WD, and X-YW conducted the characterization of gold(I) complexes 1-3. ZC and X-YW provided critical advice during the manuscript writing. All authors have given approval to the final version of the manuscript.
Funding
This study was financially supported by the National Natural Science Foundation of China (no. 22061018), the Natural Science Foundation for Distinguished Young Scholars of Jiangxi Province (20212ACB213003), the Academic and Technical Leader Plan of Jiangxi Provincial Main Disciplines (20212BCJ23004) and Shijiazhuang University Doctoral Research Startup Fund Project (22BS005).
Conflict of interest
The authors declare that the research was conducted in the absence of any commercial or financial relationships that could be construed as a potential conflict of interest.
Publisher’s note
All claims expressed in this article are solely those of the authors and do not necessarily represent those of their affiliated organizations, or those of the publisher, the editors and the reviewers. Any product that may be evaluated in this article, or claim that may be made by its manufacturer, is not guaranteed or endorsed by the publisher.
Supplementary material
The Supplementary Material for this article can be found online at: https://www.frontiersin.org/articles/10.3389/fchem.2022.1083757/full#supplementary-material
References
An, B. K., Gierschner, J., and Park, S. Y. (2012). π-Conjugated cyanostilbene derivatives: A unique self-assembly motif for molecular nanostructures with enhanced emission and transport. Acc. Chem. Res. 45, 544–554. doi:10.1021/ar2001952
Chen, Z., Wu, D., Han, X., Liang, J. H., Yin, J., Yu, G. Ao., et al. (2014). A novel fluorene-based gold(i) complex with aggregate fluorescence change: A single-component white light-emitting luminophor. Chem. Commun. 50, 11033. doi:10.1039/c4cc04469j
Chen, Z., Zhang, J., Song, M., Yin, J., Yu, G. A., and Liu, S. H. (2015). A novel fluorene-based aggregation-induced emission (AIE)-active gold(I) complex with crystallization-induced emission enhancement (CIEE) and reversible mechanochromism characteristics. Chem. Commun. 51, 326–329. doi:10.1039/c4cc08087d
Chen, S. J., Wang, H., Hong, Y., and Tang, B. Z. (2016). Fabrication of fluorescent nanoparticles based on AIE luminogens (AIE dots) and their applications in bioimaging. Mat. Horiz. 3, 283–293. doi:10.1039/c6mh00060f
Chen, Z., Liu, G., Pu, S., and Liu, S. H. (2017). Carbazole-based aggregation-induced emission (AIE)-Active gold(I) complex: Persistent room-temperature phosphorescence, reversible mechanochromism and vapochromism characteristics. Dyes Pigm. 143, 409–415. doi:10.1016/j.dyepig.2017.05.003
Chi, Z., Zhang, X., Xu, B., Zhou, X., Ma, C., Zhang, Y., et al. (2012). Recent advances in organic mechanofluorochromic materials. Chem. Soc. Rev. 41, 3878–3896. doi:10.1039/C2CS35016E
Ding, D., Li, K., Liu, B., and Tang, B. Z. (2013). Bioprobes based on AIE fluorogens. Acc. Chem. Res. 46, 2441–2453. doi:10.1021/ar3003464
Dong, S. C., Li, Z., and Qin, J. G. (2009). New carbazole-based fluorophores: Synthesis, characterization, and aggregation-induced emission enhancement. J. Phys. Chem. B 113, 434–441. doi:10.1021/jp807510a
Dong, Y., Zhang, J., Li, A., Gong, J., He, B., Xu, S., et al. (2020). Structure-tuned and thermodynamically controlled mechanochromic self-recovery of AIE-active Au(i) complexes. J. Mat. Chem. C Mat. 8, 894–899. doi:10.1039/C9TC06297A
Echeverri, M., Ruiz, C., Gámez-Valenzuela, S., Martín, I., Delgado, M., Gutierrez-Puebla, E., et al. (2020). Untangling the mechanochromic properties of benzothiadiazole-based luminescent polymorphs through supramolecular organic framework topology. J. Am. Chem. Soc. 142, 17147–17155. doi:10.1021/jacs.0c08059
Ge, W., Zhang, F., Wang, D., Wei, Q., Li, Q., Feng, Z., et al. (2022). Highly tough, stretchable, and solvent-resistant cellulose nanocrystal photonic films for mechanochromism and actuator properties. Small 18, 2107105. doi:10.1002/smll.202107105
Gopikrishna, P., Meher, N., and Iyer, P. K. (2018). Functional 1, 8-naphthalimide AIE/AIEEgens: Recent advances and prospects. ACS Appl. Mat. Interfaces 10, 12081–12111. doi:10.1021/acsami.7b14473
Huang, G., Xia, Q., Huang, W., Tian, J., He, Z., Li, B., et al. (2019a). Multiple anti-counterfeiting guarantees from a simple tetraphenylethylene derivative-high-contrasted and multi-state mechanochromism and photochromism. Angew. Chem. Int. Ed. 58, 17814–17819. doi:10.1002/anie.201910530
Huang, L. L., Liu, L., Li, X. J., Hu, H., Chen, M. X., Yang, Q. Y., et al. (2019b). Crystal-state photochromism and dual-mode mechanochromism of an organic molecule with fluorescence, room-temperature phosphorescence, and delayed fluorescence. Angew. Chem. Int. Ed. 58, 16445–16450. doi:10.1002/anie.201908567
Hunks, W., Jennings, M., and Puddephatt, R. J. (2000). Self-association in gold chemistry: A tetragold(I) complex linked by both aurophilic and hydrogen bonding. Inorg. Chem. 39, 2699–2702. doi:10.1021/ic991497k
Ishi-I, T., Tanaka, H., Youfu, R., Aizawa, N., Yasuda, T., Kato, Si., et al. (2019). Mechanochromic fluorescence based on a combination of acceptor and bulky donor moieties: Tuning emission color and regulating emission change direction. New J. Chem. 43, 4998–5010. doi:10.1039/C8NJ06050A
Ito, H., Saito, T., Oshima, N., Kitamura, N., Ishizaka, S., Hinatsu, Yukio., et al. (2008). Reversible mechanochromic luminescence of [(C6F5Au)2(µ-1, 4-diisocyanobenzene)]. J. Am. Chem. Soc. 130, 10044–10045. doi:10.1021/ja8019356
Kunitski, M., Eicke, N., Huber, Pia., Köhler, J., Zeller, S., Voigtsberger, J., et al. (2019). Double-slit photoelectron interference in strong-field ionization of the neon dimer. Nat. Commun. 10 (1), 5161. doi:10.1038/s41467-018-07882-8
Leung, C., Hong, Y., Chen, S., Zhao, E., Lam, J., and Tang, B. Z. (2013). A photostable AIE luminogen for specific mitochondrial imaging and tracking. J. Am. Chem. Soc. 135, 62–65. doi:10.1021/ja310324q
Li, W., Luo, W., Li, K., Yuan, W., and Zhang, Y. (2017a). Aggregation-induced phosphorescence and mechanochromic luminescence of a tetraphenylethene-based gold(I) isocyanide complex. Chin. Chem. Lett. 28, 1300–1305. doi:10.1016/j.cclet.2017.04.008
Li, Y., Ma, Z. Y., Li, A., Xu, W. Q., Wang, Y. C., Jiang, H., et al. (2017b). A single crystal with multiple functions of optical waveguide, aggregation-induced emission, and mechanochromism. ACS Appl. Mat. Interfaces 9, 8910–8918. doi:10.1021/acsami.7b00195
Liu, C., Bai, H., He, B., He, X., Zhang, J., Chen, C., et al. (2021). Functionalization of silk by AIEgens through facile bioconjugation: Full-color fluorescence and long-term bioimaging. Angew. Chem. Int. Ed. 60, 12424–12430. doi:10.1002/anie.202015592
Lv, C., Liu, W., Luo, Q., Yi, H., Yu, H., Yang, Z., et al. (2020). A highly emissive AIE-active luminophore exhibiting deep-red to near-infrared piezochromism and high-quality lasing. Chem. Sci. 11, 4007–4015. doi:10.1039/D0SC01095B
Mei, J., Leung, N. L. C., Kwok, R. T. K., Lam, J. W. Y., and Tang, B. Z. (2015). Aggregation-induced emission: Together we shine, united we soar. Chem. Rev. 115, 11718–11940. doi:10.1021/acs.chemrev.5b00263
Ni, J., Zhang, P., Jiang, T., Chen, Y., Su, H., Wang, D., et al. (2018). Red/NIR-emissive benzo[d]imidazole-cored AIEgens: Facile molecular design for wavelength extending and in vivo tumor metabolic imaging. Adv. Mat. 30, 1805220. doi:10.1002/adma.201805220
Osawa, M., Kawata, I., Igawa, S., Hoshino, M., Fukunaga, T., and Hashizume, D. (2010). Vapochromic and mechanochromic tetrahedral gold(I) complexes based on the 1, 2-bis (diphenylphosphino)benzene ligand. Chem. Eur. J. 16, 12114–12126. doi:10.1002/chem.201001908
Qian, J., and Tang, B. Z. (2017). AIE luminogens for bioimaging and theranostics: From organelles to animals. Chem 3, 56–91. doi:10.1016/j.chempr.2017.05.010
Seki, T., Takamatsu, Y., and Ito, H. (2016). A screening approach for the discovery of mechanochromic gold(I) isocyanide complexes with crystal-to-crystal phase transitions. J. Am. Chem. Soc. 138, 6252–6260. doi:10.1021/jacs.6b02409
Shi, P., Duan, Y., Wei, W., Xu, Z., Li, Z., and Han, T. (2018). A turn-on type mechanochromic fluorescent material based on defect-induced emission: Implication for pressure sensing and mechanical printing. J. Mat. Chem. C Mat. 6, 2476–2482. doi:10.1039/C7TC05683D
Sun, J. M., Li, H., Gu, X. G., and Tang, B. Z. (2021). Photoactivatable biomedical materials based on luminogens with aggregation-induced emission (AIE) characteristics. Adv. Healthc. Mat. 10, 2101177. doi:10.1002/adhm.202101177
Tong, J., Wang, Y., Mei, J., Wang, J., Qin, A., Sun, J. Z., et al. (2014). A 1, 3-indandione-functionalized tetraphenylethene: Aggregation induced emission, solvatochromism, mechanochromism, and potential application as a multiresponsive fluorescent probe. Chem. Eur. J. 20, 4661–4670. doi:10.1002/chem.201304174
Wang, X. Y., Hu, Y. X., Yang, X. F., Yin, J., Chen, Z., and Liu, S. H. (2019). Excitation wavelength-dependent nearly pure white light-emitting crystals from a single gold(I)-Containing complex. Org. Lett. 21, 9945–9949. doi:10.1021/acs.orglett.9b03875
Wang, J., Yue, B., Jia, X., Cao, R., Niu, X., Zhao, H., et al. (2022). Mechanical stimuli-induced multiple photophysical responsive AIEgens with high contrast properties. Chem. Commun. 58, 3517–3520. doi:10.1039/D1CC06931D
Wu, Z. N., Yao, Q. F., Zang, S.-Q., and Xie, J. P. (2021). Aggregation-induced emission in luminescent metal nanoclusters. Natl. Sci. Rev. 8, nwaa208. doi:10.1093/nsr/nwaa208
Xue, P., Ding, J., Wang, P., and Lu, R. (2016). Recent progress in the mechanochromism of phosphorescent organic molecules and metal complexes. J. Mat. Chem. C Mat. 4, 6688–6706. doi:10.1039/C6TC01503D
Yang, W., Yang, Y., Qiu, Y., Cao, X., Huang, Z., Gong, S., et al. (2020a). AIE-active multicolor tunable luminogens: Simultaneous mechanochromism and acidochromism with high contrast beyond 100 nm. Mat. Chem. Front. 4, 2047–2053. doi:10.1039/D0QM00247J
Yang, J., Fang, M., and Li, Z. (2020b). Organic luminescent materials: The concentration on aggregates from aggregation-induced emission. Aggregate 1, 6–18. doi:10.1002/agt2.2
Yang, J., Li, K., Wang, J., Sun, S., Chi, W., Wang, C., et al. (2020c). Controlling metallophilic interactions in chiral gold(I) double salts towards excitation wavelength-tunable circularly polarized luminescence. Angew. Chem. Int. Ed. 59, 6915–6922. doi:10.1002/anie.202000792
Ye, F., Liu, Y., Chen, J., Liu, S., Zhao, W., and Yin, J. (2019). Tetraphenylene-coated near-infrared benzoselenodiazole dye: AIE behavior, mechanochromism, and bioimaging. Org. Lett. 21, 7213–7217. doi:10.1021/acs.orglett.9b02292
Yin, Y., Chen, Z., Li, R., Yuan, C., Shao, T., Wang, K., et al. (2021a). Ligand-triggered platinum(II) metallacycle with mechanochromic and vapochromic responses. Inorg. Chem. 60, 9387–9393. doi:10.1021/acs.inorgchem.1c00233
Yin, Y., Hu, H., Chen, Z., Liu, H., Fan, C., and Pu, S. (2021b). Tetraphenylethene or triphenylethylene-based luminophors: Tunable aggregation-induced emission (AIE), solid-state fluorescence and mechanofluorochromic characteristics. Dyes Pigm. 184, 108828. doi:10.1016/j.dyepig.2020.108828
Zhang, X. P., Xie, T. Q., Cui, M. X., Yang, L., Sun, X. X., Jiang, J., et al. (2014). General design strategy for aromatic ketone-based single-component dual-emissive materials. ACS Appl. Mat. Interfaces 6 (4), 2279–2284. doi:10.1021/am405209w
Zhang, J., He, B., Hu, Y., Alam, P., Zhang, H., Lam, J., et al. (2021a). Stimuli-responsive AIEgens. Adv. Mat. 33, 2008071. doi:10.1002/adma.202008071
Zhang, X., Ma, Z. M., Li, X. J., Qian, C., Liu, Y., Wang, S. T., et al. (2021b). Multiresponsive tetra-arylethene-based fluorescent switch with multicolored changes: Single-crystal photochromism, mechanochromism, and acidichromism. ACS Appl. Mat. Interfaces 13, 40986–40994. doi:10.1021/acsami.1c12187
Zhang, X. P., Liu, J. K., Chen, B., He, X. W., Li, X. Y., Wei, P., et al. (2022). Highly efficient and persistent room temperature phosphorescence from cluster exciton enables ultrasensitive off-on VOC sensing. Matter 5 (10), 3499–3512. doi:10.1016/j.matt.2022.07.010
Zhao, N., Yang, Z., Lam, J. W. Y., Sung, H. H. Y., Xie, N., Chen, S., et al. (2012). Benzothiazolium-functionalized tetraphenylethene: An AIE luminogen with tunable solid-state emission. Chem. Commun. 48, 8637–8639. doi:10.1039/c2cc33780k
Keywords: carbazole, Gold(I) complexes, different substituents, aggregation-induced phosphorescent emission, mechano-responsive phosphorescence
Citation: Chen Z, Deng X-W, Wang X-Y, Wang A-Q and Luo W-T (2022) Carbazole-based aggregation-induced phosphorescent emission-active gold(I) complexes with various phosphorescent mechanochromisms. Front. Chem. 10:1083757. doi: 10.3389/fchem.2022.1083757
Received: 29 October 2022; Accepted: 21 November 2022;
Published: 01 December 2022.
Edited by:
Benzhao He, Beijing Normal University, ChinaReviewed by:
Yuncong Chen, Nanjing University, ChinaXuepeng Zhang, University of Science and Technology of China, China
Copyright © 2022 Chen, Deng, Wang, Wang and Luo. This is an open-access article distributed under the terms of the Creative Commons Attribution License (CC BY). The use, distribution or reproduction in other forums is permitted, provided the original author(s) and the copyright owner(s) are credited and that the original publication in this journal is cited, in accordance with accepted academic practice. No use, distribution or reproduction is permitted which does not comply with these terms.
*Correspondence: Zhao Chen, chenzhao666@126.com; Xiao-Yan Wang, wangxiaoyan629@163.com
†These authors have contributed equally to this work