- Key Lab of Applied Chemistry of Zhejiang Province, Department of Chemistry, College of Chemical and Biological Engineering, Zhejiang University, Hangzhou, China
Heteroatomic zeolites as an important class of zeolites, have been widely applied in industrially catalytic processes due to their unique properties. As one of the most representative heteroatomic zeolites, titanosilicate zeolites have been extensively used in the selective oxidations of organic substrates with H2O2 such as cyclohexanone ammoximation, epoxidation of alkenes, and phenol hydroxylation. In this review, recent advances in the synthesis of TS-1 zeolite are briefly summarized, including use of low-cost raw materials (organic templates, silicon, and titanium sources), development of new synthesis routes (post-treatment synthesis, dry-gel conversion synthesis, solvent-free synthesis, and microwave-assisted synthesis), and new strategy for enhanced mass transfer in TS-1 crystals (synthesis of hierarchical and nanosized TS-1 zeolite). This review might help researchers to have a deep understanding on the synthesis of TS-1 zeolite and provide a new opportunity for the design and preparation of highly efficient TS-1 catalysts in the future.
1 Introduction
Zeolites have been used in many industrial processes as the efficient catalysts due to their uniform micropores, large pore volumes, high surface areas, and excellent stabilities (Corma, 1995; Cundy and Cox, 2003; Meng and Xiao, 2014). In general, zeolite structures are always consisted of TO4 tetrahedra by sharing vertices, where the T atoms are major Si, Al, or P atoms (Arends et al., 1997; Li et al., 2014). In many cases, if T atoms become heteroatoms such as Ti, B, Ga, Fe, it is designated as heteroatomic zeolites (Dusselier and Davis, 2018; Přech, 2018).
As one of the most representative heteroatomic zeolites, TS-1 zeolite is formed by replacing silicon atoms in silicalite-1 zeolite with titanium atoms. In 1983, Taramasso et al. from Italy firstly reported the synthesis of TS-1 zeolite (Taramasso et al., 1983). Later, other titanosilicate zeolites were reported successively, mainly including Ti-Beta, Ti-ZSM-11, Ti-MOR, Ti-MWW, Ti-ITQ-7 and so on (Blasco et al., 1998; Corma et al., 2000; Díaz-Cabañas et al., 2000; Wu et al., 2001; Wu and Tatsumi, 2004; Wang et al., 2007). Among them, TS-1 zeolite has paid much attention due to its wide applications, such as cyclohexanone ammoximation, epoxidation of alkenes, phenol hydroxylation and oxidative desulfurization (Perego et al., 2001).
Due to the introduction of Ti species in the zeolite framework, TS-1 zeolite with MFI structure (Du et al., 2017) has obvious advantages such as good acid resistance, good hydrophobicity, and excellent performance for selective catalytic oxidations (Jin et al., 2007; Chen et al., 2011b). As typical examples, cyclohexanone ammoximation and epoxidation of alkenes have been performed in industrial processes, where TS-1 was employed as catalysts and hydrogen peroxide was used as a green oxidant under mild conditions (Gao et al., 2000; Perego et al., 2001; Cheng et al., 2016). In these oxidations, it is usually regarded that the tetra-coordinated Ti species in the framework and TiO6 species are the active centers (Wu et al., 2016; Xu et al., 2020).
In order to increase the catalytic performance and reduce the cost, great efforts have been paid for the synthesis of TS-1 zeolite. As a result, it is developed many new strategies and routes for synthesis of TS-1 zeolites. In this review, we briefly summarized recent advances in the synthesis of TS-1 zeolite, including use of low-cost raw materials, development of new synthesis routes, and preparation of hierarchical and nanosized zeolite crystals.
2 Use of low-cost raw materials
2.1 Organic templates
Tetrapropylammonium hydroxide (TPAOH) is the first organic template for synthesis of TS-1 zeolite. However, the TPAOH is costly. To reduce the cost, the researchers have made great efforts for use of relatively cheap organic templates to replace TPAOH. Using tetrapropylammonium bromide (TPABr) to replace TPAOH with ammonia used as the alkali source was successful to synthesize TS-1 zeolite (Müller and Steck, 1994). However, the size of the obtained product was larger than that of TS-1 zeolite synthesized with TPAOH as the organic template. To overcome this problem, organic amines as alkali sources such as hexamethylenediamine (Tuel, 1996), methylamine (Shibata et al., 1997), ethanolamine (Liu et al., 2016), and ethylamine (Zuo et al., 2011) were introduced in the synthetic systems.
In the aforementioned systems, the question is whether organic amines act as templates or only as alkali sources. Li et al. (1999) confirmed that the organic amines just acted as alkali sources rather than as organic templates when the amount of TPABr in the gel was enough.
Although the direct ability of organic amines for the synthesis of TS-1 zeolite is much weaker than that of TPA+, TS-1 zeolite could be synthesized successfully under the synergistic effect of alkali metal cations or seed crystals with some organic amines. For example, Ma et al. (1996) reported a successful synthesis of TS-1 zeolite in the presence of 1, 6-hexandiamine and n-butylamine as well as sodium hydroxide. However, the presence of sodium ions in the system partially hindered the introduction of Ti species into the zeolite framework, leading to the formation of anatase TiO2. Later, Zhang et al. (2009) synthesized TS-1 zeolite in the absence of alkali metal ions using hexamethyleneimine (HMI) with the addition of active TS-1 precursor from the conventional TPAOH system.
2.2 Silicon and titanium source
It has a great challenge for the synthesis of catalytically active TS-1 zeolite with all titanium species in the framework (Zhang et al., 2016b; Lin et al., 2021), which is strongly related to the selection of silicon and titanium sources in the synthesis. In the beginning, Taramasso et al. (1983) reported that tetraethyl orthosilicate (TEOS) and tetraethyl titanate (TEOT) as silicon and titanium sources were used for the synthesis of TS-1 zeolite. Notably, TEOT hydrolyzed rapidly, partially forming extra-framework titanium species. To solve this problem, Xing et al. (2021) reported the optimized synthesis of TS-1 zeolite from self-made polymer containing titanium and silicon prepared by TEOS and TEOT. Due to the well hydrolysis resistance of Ti-Diol-Si polymer, silicon and titanium sources have suitable matching for the hydrolysis rate in the crystallization process, which is conducive to the formation of high-quality TS-1 zeolite without extra-framework titanium species (Figure 1).
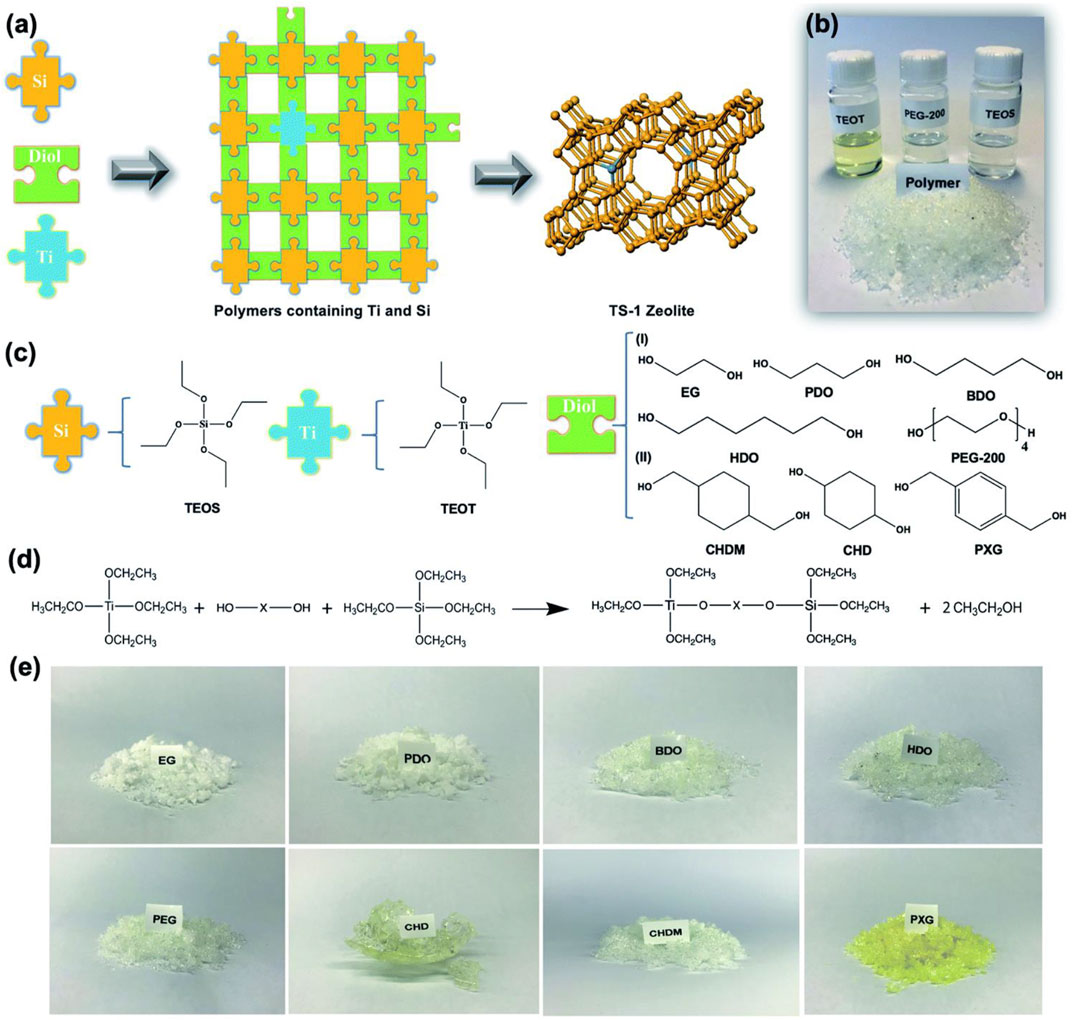
FIGURE 1. (A) Synthesis of TS-1 zeolite from Ti–diol–Si polymers. (B) Photograph of the liquid raw materials and solid polymer products. (C) Types of alkyl titanates, alkyl silicates and alkyl diols used. (D) Transesterification reaction. (E) Photograph of the Ti–diol–Si polymers. Reprinted with permission from Xing et al. (2021). Copyright 2021 Royal Society of Chemistry.
Thangaraj et al. (1992) proposed to replace traditional TEOT with tetrabutyl titanate (TBOT) due to the hydrolysis rate of TBOT matched with TEOS, thus avoiding the formation of extra-framework titanium species. In addition, they also reported the reduction of these extra-framework titanium species in the synthesis by changing the feeding sequence or adding isopropanol.
Compared with organic esters of TBOT and TEOS, inorganic silicon and titanium sources are much cheaper. Thus, the researchers have developed many inorganic titanium and silicon source as the raw materials for the synthesis of TS-1 zeolite. For the inorganic titanium sources, it has been reported titanium fluoride (TiF4, Jorda et al., 1997), TiCl3 (Gao et al., 1995), TiCl4 (Shibata et al., 1997; Zuo et al., 2011), and Ti(SO4)2 (Zhang et al., 2006). For the inorganic silicon sources, it has been reported colloidal silica (Zuo et al., 2011), fumed silica (Shibata et al., 1997; Zhang et al., 2009), solid silica gel (Zhang et al., 2006). For examples, when TiCl3 was used for titanium source (Gao et al., 1995), hydrolysis of TiCl3 is relatively slow compared with TBOT, thus avoiding the formation of the extra-framework titanium species. When the TiF4 was employed (Shibata et al., 1997; Zuo et al., 2011), it is also avoided the formation of extra-framework titanium species in the TS-1 zeolite because TiF4 is more stable than TBOT in the synthesis. Catalytic tests in cyclohexanone ammoximation and hydroxylation of phenol showed that these TS-1 zeolites from inorganic sources were comparable with the TS-1 zeolites from the organic esters. Furthermore, Zhang et al. (2006) reported that solid silica gel and titanium sulfate were used as raw materials to synthesize TS-1 zeolite with good catalytic performance. In this case, a SiO2-TiO2 precursor with Si-O-Ti bonds was prepared for the hydrothermal synthesis. Table 1 briefly summarized the synthesis of TS-1 zeolites using various raw materials. Obviously, the use of inorganic titanium and silicon as raw materials simplifies the synthetic steps, avoids the generation of anatase, and reduces the cost of TS-1 zeolite.
3 Development of new synthetic routes
3.1 Conventional hydrothermal synthesis
Hydrothermal synthesis is a conventional method for synthesis of TS-1 zeolite reported by Taramasso et al. (1983). In general, there are two steps including gelling and crystallization in the hydrothermal synthesis, where anatase is easily produced. Thus, in the process of hydrothermal synthesis, crystallization promoters, protective agents, and/or additives such as isopropyl alcohol, ammonium carbonate, and/or glycine (Thangaraj et al., 1992; Fan et al., 2008; Wang et al., 2016, 2021; Zhang et al., 2018, 2021) would be added to obtain the anatase-free TS-1 zeolite.
Fan et al. (2008) reported that the addition of (NH4)2CO3 could reduce the value of pH and then decrease rate in the crystallization of TS-1 zeolite, which was well matchable with the speed of Ti species entering into the TS-1 framework. As a result, the obtained TS-1 zeolite has high content of Ti species in the framework, giving high catalytic activities in oxidations of a variety of organic substrates. Wang et al. (2021) reported that anatase-free TS-1 zeolite could be synthesized from the help of L-lysine. The presence of L-lysine not only limited the formation of extra-framework titanium species but also ensured efficient incorporation of TiO6 (open sites) into the anatase-free TS-1 zeolite. In olefin oxidation of 1-hexene, the TS-1 zeolite from the L-lysine exhibited higher activity than the conventional TS-1, which is owing to the coexistence of TiO4 and TiO6 species in appropriate proportions in the TS-1 catalyst (Figure 2).
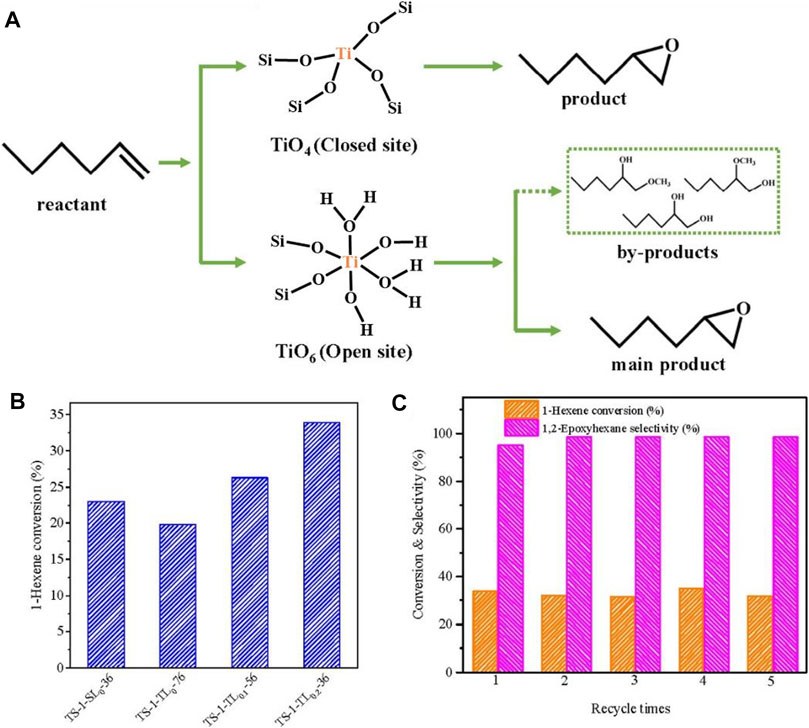
FIGURE 2. (A) Epoxidation routes directed by TiO4 (closed sites) and TiO6 (open sites) species in the TS-1 zeolites; (B) 1-Hexene conversion over the different TS-1 zeolites; (C) 1-Hexene conversion and product selectivity of the recycle test using TS-1-TL0.2-36. Reprinted with permission from Wang et al. (2021). Copyright 2021 Elsevier.
Recently, Lin et al. (2021) reported the reversed-oligomerization with UV irradiation to synthesize TS-1 zeolite by matching the hydrolysis rate of Ti and Si species. Different from the conventional route to avoid the formation of Ti oligomer by slowing down the hydrolysis of Ti precursor, this approach reverses the oligomerization of Ti monomer and accelerates the hydrolysis of Si-alkoxide simultaneously, which is matchable with the hydrolysis rate of Ti species. With the UV irradiation, the hydrolysis time of TEOS reduced from 120 to 60 min and the time of Ti oligomer formation by TBOT was less than 1 min, while the subsequent de-oligomerization of Ti oligomers to Ti monomers was successfully achieved within 60 min (Figure 3).
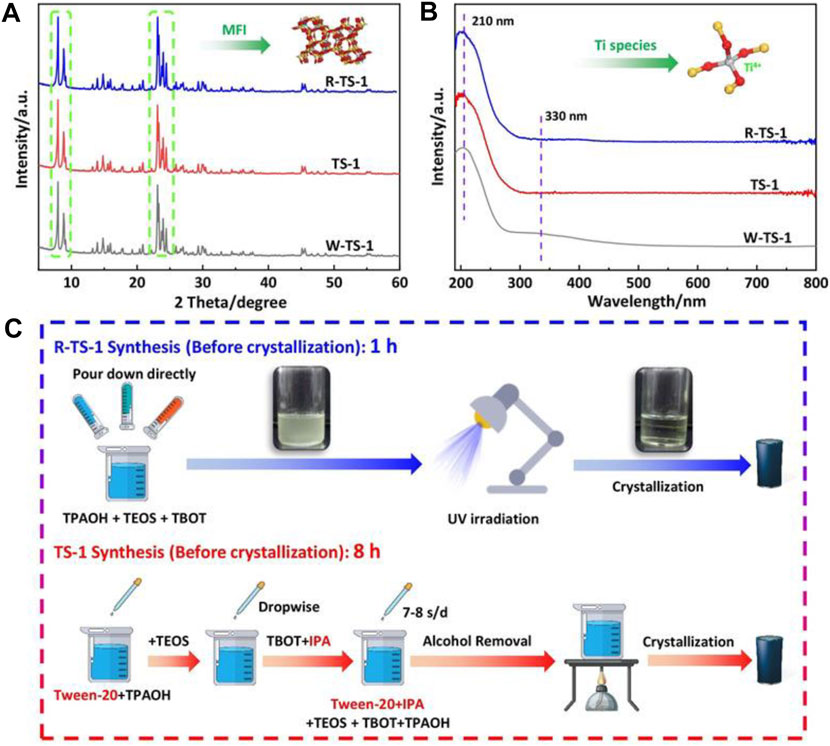
FIGURE 3. (A) XRD patterns and (B) UV spectra of R-TS-1 (with UV irradiation and without additives), TS-1 (with additives), and W-TS-1 (without UV irradiation and additives). (C) Representation of the synthetic procedures. Reprinted with permission from Lin et al. (2021). Copyright 2020 Wiley-VCH GmbH.
3.2 Post-treatment synthesis
The principle of post-treatment synthesis is to remove B or Al in the structure of ZSM-5 zeolite, generating lattice vacancies, followed by introduction of Ti species into the zeolite framework (Zhang et al., 1999). Generally, the post treatments mainly include gas-solid isomorphous substitution with TiCl4 and liquid-solid isomorphous substitution with aqueous solution of (NH4)2TiF6 (Xu et al., 1992; Liu et al., 2006).
Kraushaar and Van Hooff (1988) firstly reported the dealuminated ZSM-5 zeolite with HCl aqueous solution to form lattice vacancies and then reacted with TiCl4 to insert titanium species to obtain TS-1 zeolite. The catalytic performance in phenol oxidation for the obtained TS-1 zeolite was similar with that of TS-1 zeolite under hydrothermal conditions (Kraushaar and Van Hooff, 1989).
Yoo et al. (2000) described that the content of Ti in Ti-ZSM-5 zeolite increased with the decreasing of SiO2/Al2O3 ratio when H-ZSM-5 zeolite was a precursor. The structure and surface area of H-ZSM-5 zeolite were not affected in the process of the treatment. Compared with the direct hydrothermal synthesis of TS-1 zeolite, the obtained TS-1 zeolite by the post-treatment synthesis had relatively high conversion and selectivity in cyclohexanone ammoximation.
Liu et al. (2004) reported the effects of B-ZSM-5 zeolite precursors with different molar ratios of SiO2/B2O3 on the incorporation of titanium species into the ZSM-5 framework. With the decrease of SiO2/B2O3 ratio, the more hydroxyl nests could be obtained after HCl treatment, thus more titanium species could be incorporated into the zeolite framework, giving higher catalytic activity in propylene epoxidation.
Post-treatment synthesis of TS-1 zeolite avoids the formation of anatase in the products and the employment of organic titanium as the raw material, which could significantly reduce the cost of TS-1 zeolite. However, this repeatability is relatively poor and synthetic procedures are relatively complex, compared with conventional synthesis of TS-1 zeolite.
3.3 Dry gel conversion
In 1990, Xu et al. (1990) firstly reported a dry gel conversion (DGC) to prepare high silica and all silica zeolites. For this method, silica or aluminosilicate gels are mixed with organic templates and then crystallized in the presence of water vapor in a specific reactor. Compared with the hydrothermal synthesis, the dry gel conversion has the advantages of high yield, avoidance of separation, and reduction of wastes (You et al., 2013). Followed this idea, many researchers have devoted to synthesizing TS-1 zeolite using DGC method (Ke et al., 2007; Wang et al., 2013; Wu et al., 2014a; Yue et al., 2014; Zhang et al., 2016a; Du et al., 2019).
Zhang et al. (2016a) showed a one-step DGC method to synthesize shaped TS-1 zeolite by the employment of a small amount of TPAOH. Using this method, the TPAOH/SiO2 ratios could be reduced to 0.1, which greatly decreased the cost of TS-1 zeolite. The obtained TS-1 zeolite showed nanosized crystals (50–200 nm). Particularly, it is one step for preparation of the shaped TS-1 zeolite, which is favorable for industrial applications of TS-1 zeolite catalysts.
Du et al. (2019) synthesized hierarchical TS-1 zeolite by simply adjusting the dry gel preparation process without the addition of mesoporous organic templates using DGC method. The key to this success is to prepare the loosely porous dry gel by grinding, which was helpful for fast diffusion of steam inside the dry gel (Figure 4). Moreover, the surface Ti content of TS-1 zeolite obtained by this method was significantly higher than that of the internal section, contributing to the excellent catalytic performance in the oxidation of bulky sulfur compounds.
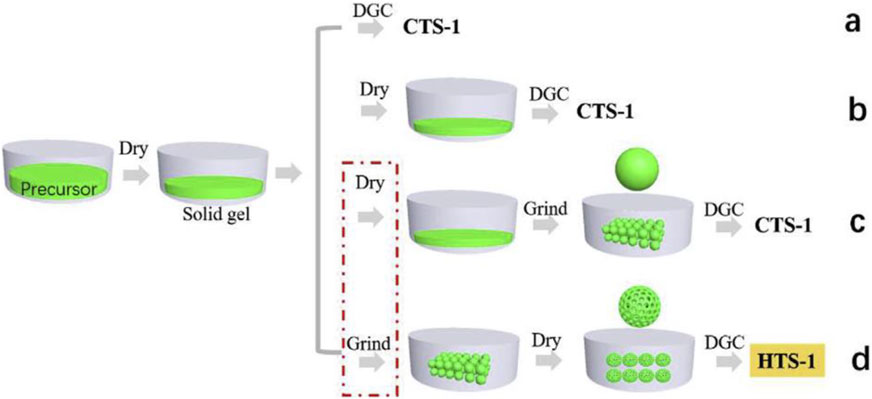
FIGURE 4. Mechanism of the formation of HTS-1 zeolite. The dry gel with compact structure was converted to conventional TS-1 (a–c) and loose compact structure was converted to hierarchical TS-1 (d). Reprinted with permission from Du et al. (2019). Copyright 2018 Elsevier.
3.4 Solvent-free synthesis
In recent years, Xiao et al. reported the solvent-free synthesis of zeolites without addition of any solvent (Ren et al., 2012). It has been successfully synthesized pure silica and aluminosilicate zeolites, aluminophosphate and silicoaluminophosphate molecular sieves (Jin et al., 2013, 2014; Wu et al., 2014b; Wu et al., 2019). Compared with conventional synthesis, solvent-free synthesis of zeolites has obvious advantages, such as high zeolite yields, high autoclave utilization, significantly reduced pollutants, reduced energy consumption, simple synthesis processes, and significantly reduced reaction pressures (Meng and Xiao, 2014). Subsequently, it was also successful to synthesize TS-1 zeolite using this method (Zhu et al., 2015; Cui et al., 2017; Fu et al., 2020; Wang et al., 2020; Liu et al., 2022).
Zhu et al. (2015) reported the synthesis of TS-1 zeolite using fumed silica, titanium sulfate, TPAOH and zeolite seeds as raw materials under solvent-free conditions. Notably, TS-1 synthesized by this route has almost the same catalytic performance with that of TS-1 zeolite synthesized by conventional hydrothermal method in the catalytic oxidation of hexane. Liu et al. (2022) reported the improved synthesis of TS-1 zeolite from solvent-free synthesis, obtaining the anatase-free nanosized TS-1 zeolite product. It just mixed the untreated seed solution prepared by TPAOH and TEOS, silicon source, and titanium source, then ground and crystallized (Figure 5). By studying the possible mechanism of TS-1 zeolite, it was found that the seed solution is the key factor to obtain nanosized TS-1 zeolite. This method has the advantages of simple operation and high yield, which might open a new opportunity to prepare nanosized TS-1 crystals for industrial applications.
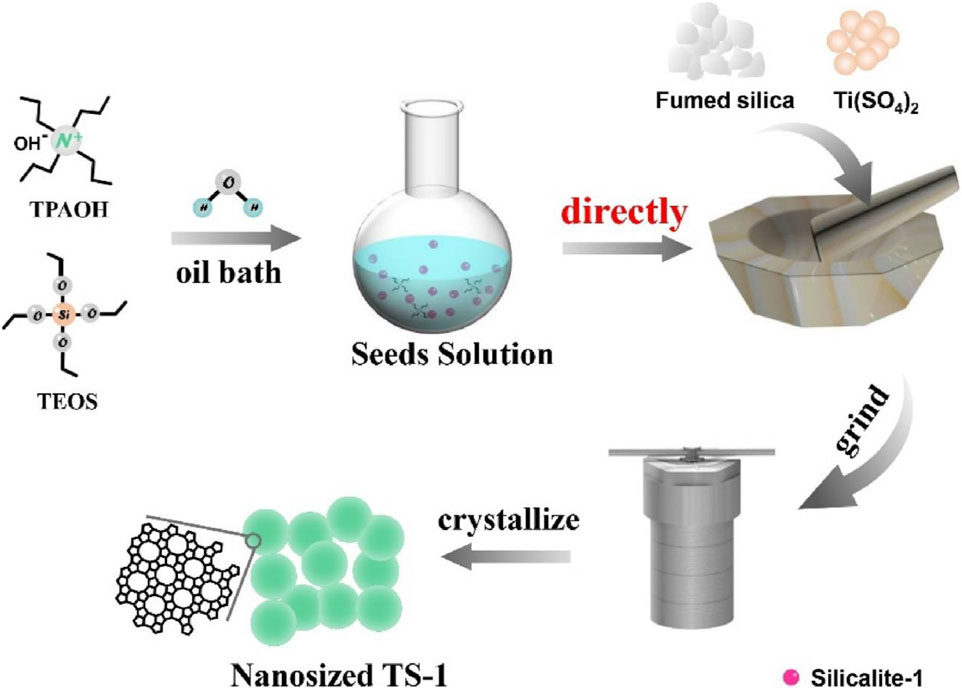
FIGURE 5. The schematic process of synthesizing anatase-free nanosized TS-1 zeolite under solvent-free conditions. Reprinted with permission from Liu et al. (2022). Copyright 2021 Elsevier.
3.5 Microwave-assisted synthesis
Microwave-assisted method as a novel route for zeolite synthesis came into the view of researchers in the 1980s and had also been used to synthesize TS-1 zeolite. Prasad et al. (2002) reported TS-1 zeolite could be obtained by microwave-assisted route and the crystallization time of TS-1 (Si/Ti = 33) was as short as 30 min. Moreover, the morphology of TS-1 zeolite could be controlled with microwave irradiation. Jin et al. (2009) showed that the use of microwave led to the formation of the small crystals adhered to each other through the b-orientation, forming a stacked TS-1 zeolite. Yang et al. (2006) proved that TS-1 zeolite prepared under microwave irradiation has a high hydrophobicity, leading to the enhanced adsorption capacity of styrene and 1-hexene, thus obtaining the improved catalytic performance of epoxidations.
With the help of microwave irradiation, the modulation of the coordination environments of Ti active sites could also be successful (Xu et al., 2020). TS-1 zeolite with octahedral coordination Ti species (mononuclear TiO6) was synthesized by adding zeolite seeds and microwave irradiation. The obtained TS-1 zeolite with mononuclear TiO6 feature had good catalytic activity and stability in the epoxidation of 1-hexene.
4 The strategies for enhanced mass transfer
Most of the catalytic active site in TS-1 zeolite are located inside of the micropores. In general, the reactants should diffuse into the micropore at first, then access to the active sites. However, the microporous size of the ten-membered ring of TS-1 zeolite is too small to diffuse the reactants and products, which strongly influences the catalytic activities (Du et al., 2016). To solve this issue, it is desirable to synthesize TS-1 zeolite with fast mass transfer (Wang et al., 2022). In the past decades, great advances have been made for synthesis of hierarchical and nanosized TS-1 crystals, effectively eliminating diffusion constraints.
4.1 Synthesis of hierarchical TS-1 zeolite
Hierarchical TS-1 zeolite usually has both microporosity and mesoporosity even macroporosity, which not only has fast mass transfer but also reduce the coke formation in the reactions. At present, there are two methods for the synthesis of hierarchical TS-1 zeolite (top-down and bottom-up routes) (Chen et al., 2016). Bottom-up route is to introduce mesopore into TS-1 zeolite by addition of mesoporous templates in the synthesis, including hard templates and soft templates. Top-down route is to introduce mesopore by the extraction of framework composition from post-treatments such as alkali (Valtchev et al., 2011). Many reviews have summarized the synthesis of hierarchical TS-1 zeolite, therefore this part is not discussed in this review (Ren et al., 2017; Yang et al., 2020a; Bai et al., 2021).
4.2 Synthesis of nanosized TS-1 crystals
In addition to the hierarchical TS-1 zeolite, nanosized TS-1 crystals are very favorable for fast mass transfer, thus improving the catalytic performances (Wang et al., 2022). At present, the synthesis of nanosized TS-1 crystals mainly includes organotemplate directing, additive assistance, and seed direction.
4.2.1 Organotemplate directing
Organic template not only plays an important role in structural directing but also controls crystalline morphology in the synthesis of zeolites. There are many literatures for synthesis of nanosized TS-1 crystals using unique organic templates (Xu et al., 2019; Shen et al., 2020; Ma et al., 2022). Na et al. (2011) firstly reported that TS-1 zeolite nanosheets with single-unit-cell thickness could be successfully synthesized from quaternary ammonium salt surfactant [C16H33–N+(CH3)2–C6H12–N+(CH3)2–C6H13] as a structure-directing agent. The obtained product has a large external specific surface area (580 m2/g) and short diffusion pathway (2 nm along the b-axis). 4-coordinated Ti species and more active sites on the external surface of TS-1 zeolite nanosheets resulted in the excellent performance in epoxidation not only for small linear alkenes but also for large alkenes. After reduction of the silanol in the external surface to increase the hydrophobicity of TS-1 zeolite by post-fluoridation, the catalytic activity and epoxidation selectivity of the cycloalkene would be significantly improved.
Li et al. (2020) used the bolaform surfactant [C6H13–N+(CH3)2–C6H12–N+(CH3)2–(CH2)12–O–(p-C6H4)2–O–(CH2)12–N+(CH3)2–C6H12–N+(CH3)2–C6H13] as a structure-directing agent to synthesize TS-1 zeolite nanosheets with superior interlayer stability and house-of-cards-like structure. Compared with the traditional TS-1 zeolite and hierarchical TS-1 zeolite synthesized by organosilane surfactant [3-(trimethoxysilyl) propyl] octadecyldimethylammonium chloride (TPOAC), the obtained TS-1 nanosheets exhibited excellent catalytic activity, recovery, and stability for the selective oxidation of bulk cyclic alkenes in liquid phase.
Although TS-1 nanosheets could be synthesized using organic templates, the high cost of organic template limits its practical applications. Further efforts should be done to develop low-cost organic templates for synthesis of TS-1 nanosheets.
4.2.2 Additive-assisted synthesis
Additives such as inorganic and organic agents, polymers, and amino acid, can influence the crystallization of TS-1 zeolite, forming nanosized crystals (Shan et al., 2010; Xu et al., 2012; Shakeri and Dehghanpour, 2019; Yang et al., 2020b; Ji et al., 2021; Li et al., 2021). Compared with designed organic templates, it is economical to use such additives to control the morphology of zeolite crystals.
Li et al. (2021) synthesized TS-1 crystals with the size less than 100 nm by a two-step method using L-lysine as an additive. The introduction of L-lysine inhibited the growth of the crystals, resulting in the formation of nanosized TS-1 crystals. In addition, L-lysine also reduced the pH value of the gel system, which is conducive to incorporate Ti to the TS-1 zeolite framework. Compared with TS-1 zeolite obtained without L-lysine, the conversion of benzene and the yield of phenol increased from 28.9% to 50.2% and 17.1%–30.8%, respectively.
Polyethylene glycol is also a good additive to synthesize nanosized TS-1 crystals with high Ti content in the framework and low content of anatase TiO2, resulting in good catalytic performance in hydroxylation of phenol, oxidation of dibenzothiophene, and deep desulfurization of fuels (Shakeri and Dehghanpour, 2019).
In addition to the organic additives, inorganic additives could be also used for synthesis of nanosized TS-1 crystals. Shan et al. (2010) prepared the TS-1 bulky particles formed by nanocrystals with the addition of H2O2 and the aggregation of TS-1 nanocrystals was promoted by the strong interaction between Ti species and H2O2 (Figure 6). Compared with organic additives, the use of inorganic additives has obvious advantage for reduction of the cost.
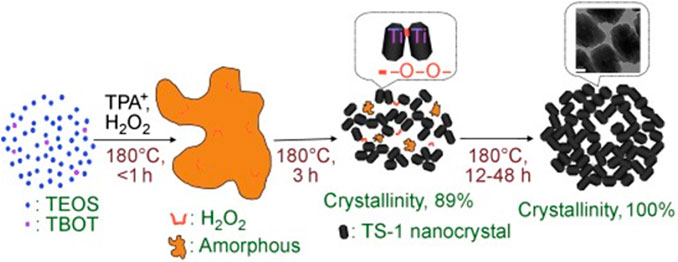
FIGURE 6. The proposed route for the formation of bulky particles formed by TS-1 zeolite nanocrystals in the presence of H2O2. Reprinted with permission from Shan et al. (2010). Copyright 2010 Wiley-VCH.
4.2.3 Seed-directed synthesis
In the process of zeolites synthesis, zeolite seeds could provide crystal nucleus to reduce the crystal size. Nanosized TS-1 crystals has also been prepared by seed-directed synthesis (Chen et al., 2011a; Zuo et al., 2012; Li et al., 2019; Liu et al., 2020).
Liu et al. (2020) reported that nanosized (50–100 nm) TS-1 zeolite could be efficiently prepared by using the recovered mother liquor within 3–8 h. The growth of TS-1 zeolite can be explained by that the reactive SiO2 (especially monosilicate species) polymerized into SimOn species to form the special secondary building units (SBUs) and then these SBUs assembled into the MFI cage, eventually forming the MFI zeolite crystal (Figure 7). The recovered mother liquor not only provides a certain amount of SBU-type SimOn species for fastening the homogenous nucleation but also enhances the supersaturation of active silica species for the formation of nanosized crystals.
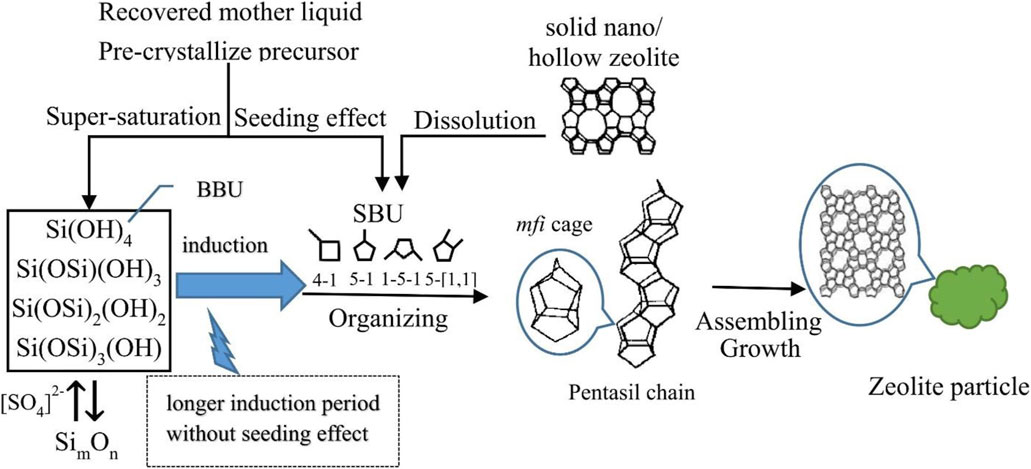
FIGURE 7. Growth model of the TS-1 zeolite (MFI-type crystal). Reprinted with permission from Liu et al. (2020). Copyright 2019 Elsevier.
Li et al. (2019) synthesized nanoscale TS-1 (360 nm × 190 nm × 640 nm) by seed-directed method in the dry gel system containing tetrapropylammonium bromide (TPABr) and n-butylamine. Chen et al. (2011a) obtained hierarchical nanocrystalline TS-1 aggregates with supermicro/mesopores using TPAOH as a single organic template with the assistance of zeolite seeds. The nanocrystalline TS-1 aggregates with the size of 300–500 nm overcame the filtration difficulties in separation, which is helpful for industrial preparation of nanosized TS-1 catalyst at a large scale.
5 Conclusion and outlooks
In summary, we simply reviewed recent advances for TS-1 zeolite synthesis. To reduce the TS-1 cost, it is discussed the use of low-cost raw materials including various organic templates, silicon and titanium sources. Furthermore, we described new routes for synthesis of TS-1 zeolite such as post-treatments, dry-gel conversion, solvent-free, and microwave-assisted approaches, which are helpful for reduction of environmentally unfriendly wastes in the synthesis. Finally, it is shown the new strategies for fast mass transfer such as introduction of hierarchical porosity into TS-1 crystals and controllable TS-1 crystals to nanosizes or nanosheets.
Although there are great progresses in the synthesis of TS-1 zeolite, there are still challenges. For examples, industrial preparation of TS-1 zeolite is generally under strong alkaline media, where a large amount of silica species are dissolved in the mother liquor. Therefore, it is strongly desirable to synthesize TS-1 zeolite under near neutral conditions; Currently, it is necessary to use organic templates for the synthesis of TS-1 zeolite, which is costly. Therefore, it is expected to develop an organotemplate-free route for the synthesis of TS-1 zeolite. In view of the wide applications of TS-1 zeolite in the industrial progresses, it should be continuously explored novel strategies for the synthesis of TS-1 zeolite with reduced cost and enhanced catalytic performance.
Author contributions
HL: Conceptualization, investigation, writing original draft; CX: Investigation; QW and F-SX: Supervision, writing—review and editing.
Funding
This work is supported by the National Natural Science Foundation of China (22172141, 21835002, and 92045303), Fundamental Research Funds for the Central Universities (2021QNA4028).
Conflict of interest
The authors declare that the research was conducted in the absence of any commercial or financial relationships that could be construed as a potential conflict of interest.
Publisher’s note
All claims expressed in this article are solely those of the authors and do not necessarily represent those of their affiliated organizations, or those of the publisher, the editors and the reviewers. Any product that may be evaluated in this article, or claim that may be made by its manufacturer, is not guaranteed or endorsed by the publisher.
References
Arends, I. W. C. E., Sheldon, R. A., Wallau, M., and Schuchardt, U. (1997). Oxidative transformations of organic compounds mediated by redox molecular sieves. Angew. Chem. Int. Ed. Engl. 36, 1144–1163. doi:10.1002/anie.199711441
Bai, R., Song, Y., Bai, R., and Yu, J. (2021). Creation of hierarchical titanosilicate TS-1 zeolites. Adv. Mat. Interfaces 8, 2001095. doi:10.1002/admi.202001095
Blasco, T., Camblor, M. A., Corma, A., Esteve, P., Guil, J. M., Martínez, A., et al. (1998). Direct synthesis and characterization of hydrophobic aluminum-free Ti−Beta zeolite. J. Phys. Chem. B 102, 75–88. doi:10.1021/jp973288w
Chen, L.-H., Li, X.-Y., Tian, G., Li, Y., Rooke, J. C., Zhu, G.-S., et al. (2011b). Highly stable and reusable multimodal zeolite TS-1 based catalysts with hierarchically interconnected three-level micro-meso-macroporous structure. Angew. Chem. Int. Ed. 50, 11156–11161. doi:10.1002/anie.201105678
Chen, L., Wang, Y. M., and He, M.-Y. (2011a). Hydrothermal synthesis of hierarchical titanium silicalite-1 using single template. Mater. Res. Bull. 46, 698–701. doi:10.1016/j.materresbull.2011.01.015
Chen, X., Vicente, A., Qin, Z., Ruaux, V., Gilson, J.-P., and Valtchev, V. (2016). The preparation of hierarchical SAPO-34 crystals via post-synthesis fluoride etching. Chem. Commun. 52, 3512–3515. doi:10.1039/C5CC09498D
Cheng, W., Jiang, Y., Xu, X., Wang, Y., Lin, K., and Pescarmona, P. P. (2016). Easily recoverable titanosilicate zeolite beads with hierarchical porosity: Preparation and application as oxidation catalysts. J. Catal. 333, 139–148. doi:10.1016/j.jcat.2015.09.017
Corma, A., Díaz-Cabañas, M. J., Domine, M. E., and Rey, F. (2000). Ultra fast and efficient synthesis of Ti-ITQ-7 and positive catalytic implications. Chem. Commun. 1725–1726. doi:10.1039/b004203j
Corma, A. (1995). Inorganic solid acids and their use in acid-catalyzed hydrocarbon reactions. Chem. Rev. 95, 559–614. doi:10.1021/cr00035a006
Cui, T.-L., Li, X.-H., and Chen, J.-S. (2017). Mesoporous TS-1 nanocrystals as low cost and high performance catalysts for epoxidation of styrene. Chin. J. Chem. 35, 577–580. doi:10.1002/cjoc.201600701
Cundy, C. S., and Cox, P. A. (2003). The hydrothermal synthesis of zeolites: History and development from the earliest days to the present time. Chem. Rev. 103, 663–702. doi:10.1021/cr020060i
Díaz-Cabañas, M.-J., Villaescusa, L. A., and Camblor, M. A. (2000). Synthesis and catalytic activity of Ti-ITQ-7: A new oxidation catalyst with a three-dimensional system of large pore channels. Chem. Commun. 761–762. doi:10.1039/b000539h
Du, Q., Guo, Y., Wu, P., Liu, H., and Chen, Y. (2019). Facile synthesis of hierarchical TS-1 zeolite without using mesopore templates and its application in deep oxidative desulfurization. Microporous Mesoporous Mater. 275, 61–68. doi:10.1016/j.micromeso.2018.08.018
Du, S., Chen, X., Sun, Q., Wang, N., Jia, M., Valtchev, V., et al. (2016). A non-chemically selective top-down approach towards the preparation of hierarchical TS-1 zeolites with improved oxidative desulfurization catalytic performance. Chem. Commun. 52, 3580–3583. doi:10.1039/C5CC10232D
Du, S., Sun, Q., Wang, N., Chen, X., Jia, M., and Yu, J. (2017). Synthesis of hierarchical TS-1 zeolites with abundant and uniform intracrystalline mesopores and their highly efficient catalytic performance for oxidation desulfurization. J. Mat. Chem. A 5, 7992–7998. doi:10.1039/C6TA10044A
Dusselier, M., and Davis, M. E. (2018). Small-pore zeolites: Synthesis and catalysis. Chem. Rev. 118, 5265–5329. doi:10.1021/acs.chemrev.7b00738
Fan, W., Duan, R.-G., Yokoi, T., Wu, P., Kubota, Y., and Tatsumi, T. (2008). Synthesis, crystallization mechanism, and catalytic properties of titanium-rich TS-1 free of extraframework titanium species. J. Am. Chem. Soc. 130, 10150–10164. doi:10.1021/ja7100399
Fu, T., Zhang, Y., Wu, D., Zhu, W., Gao, X., Han, C., et al. (2020). Solvent-free synthesis of MFI-type zeolites and their degradation properties of gas-phase styrene. J. Hazard. Mater. 397, 122630. doi:10.1016/j.jhazmat.2020.122630
Gao, H., Lu, W., and Chen, Q. (2000). Characterization of titanium silicalite-1 prepared from aqueous TiCl3. Microporous Mesoporous Mater. 34, 307–315. doi:10.1016/S1387-1811(99)00183-3
Gao, H., Suo, J., and Li, S. (1995). An easy way to prepare titanium silicalite-1 (TS-1). J. Chem. Soc. Chem. Commun. 1995, 835. doi:10.1039/c39950000835
Ji, Y., Zuo, Y., Liu, M., Wang, F., Song, C., and Guo, X. (2021). From nano aggregates to nano plates: The roles of gelatin in the crystallization of titanium silicate-1. Microporous Mesoporous Mater. 321, 111100. doi:10.1016/j.micromeso.2021.111100
Jin, C., Li, G., Wang, X., Zhao, L., Liu, L., Liu, H., et al. (2007). Synthesis, characterization and catalytic performance of Ti-containing mesoporous molecular sieves assembled from titanosilicate precursors. Chem. Mat. 19, 1664–1670. doi:10.1021/cm0625777
Jin, H., Jiang, N., Oh, S.-M., and Park, S.-E. (2009). Epoxidation of linear olefins over stacked TS-1 zeolite catalysts. Top. Catal. 52, 169–177. doi:10.1007/s11244-008-9141-9
Jin, Y., Chen, X., Sun, Q., Sheng, N., Liu, Y., Bian, C., et al. (2014). Solvent-free syntheses of hierarchically porous aluminophosphate-based zeolites with AEL and AFI structures. Chem. Eur. J. 20, 17616–17623. doi:10.1002/chem.201403890
Jin, Y., Sun, Q., Qi, G., Yang, C., Xu, J., Chen, F., et al. (2013). Solvent-free synthesis of silicoaluminophosphate zeolites. Angew. Chem. Int. Ed. 52, 9172–9175. doi:10.1002/anie.201302672
Jorda, E., Tuel, A., Teissier, R., and Kervennal, J. (1997). TiF4: An original and very interesting precursor to the synthesis of titanium containing silicalite-1. Zeolites 19, 238–245. doi:10.1016/S0144-2449(97)00067-5
Ke, X., Xu, L., Zeng, C., Zhang, L., and Xu, N. (2007). Synthesis of mesoporous TS-1 by hydrothermal and steam-assisted dry gel conversion techniques with the aid of triethanolamine. Microporous Mesoporous Mater. 106, 68–75. doi:10.1016/j.micromeso.2007.02.034
Kraushaar, B., and Van Hooff, J. H. C. (1988). A new method for the preparation of titanium-silicalite (TS-1). Catal. Lett. 1, 81–84. doi:10.1007/BF00772769
Kraushaar-Czarnetzki, B., and Van Hooff, J. H. C. (1989). A test reaction for titanium silicalite catalysts. Catal. Lett. 2, 43–47. doi:10.1007/BF00765329
Li, G., Guo, X., Wang, X., Zhao, Q., Bao, X., Han, X., et al. (1999). Synthesis of titanium silicalites in different template systems and their catalytic performance. Appl. Catal. A Gen. 185, 11–18. doi:10.1016/S0926-860X(99)00115-5
Li, K., Valla, J., and Garcia-Martinez, J. (2014). Realizing the commercial potential of hierarchical zeolites: New opportunities in catalytic cracking. ChemCatChem 6, 46–66. doi:10.1002/cctc.201300345
Li, M., Shen, X., Liu, M., and Lu, J. (2021). Synthesis TS-1 nanozelites via L-lysine assisted route for hydroxylation of benzene. Mol. Catal. 513, 111779. doi:10.1016/j.mcat.2021.111779
Li, N., Wang, M., You, Q., Bi, C., Chen, H., Liu, B., et al. (2020). Bolaform surfactant-directed synthesis of TS-1 zeolite nanosheets for catalytic epoxidation of bulky cyclic olefins. Catal. Sci. Technol. 10, 1323–1335. doi:10.1039/C9CY02282A
Li, Y., Fan, Q., Li, Y., Feng, X., Chai, Y., and Liu, C. (2019). Seed-assisted synthesis of hierarchical nanosized TS-1 in a low-cost system for propylene epoxidation with H2O2. Appl. Surf. Sci. 483, 652–660. doi:10.1016/j.apsusc.2019.03.334
Lin, D., Zhang, Q., Qin, Z., Li, Q., Feng, X., Song, Z., et al. (2021). Reversing titanium oligomer formation towards high-efficiency and green synthesis of titanium-containing molecular sieves. Angew. Chem. Int. Ed. 60, 3443–3448. doi:10.1002/anie.202011821
Liu, H., Wang, Y., Ye, T., Wang, F., Ran, S., Xie, H., et al. (2022). Fully utilizing seeds solution for solvent-free synthesized nanosized TS-1 zeolites with efficient epoxidation of chloropropene. J. Solid State Chem. 307, 122844. doi:10.1016/j.jssc.2021.122844
Liu, M., Chang, Z., Wei, H., Li, B., Wang, X., and Wen, Y. (2016). Low-cost synthesis of size-controlled TS-1 by using suspended seeds: From screening to scale-up. Appl. Catal. A General 525, 59–67. doi:10.1016/j.apcata.2016.07.006
Liu, M., Gao, J., Guo, X., Wang, X., Li, C., and Feng, Z. (2006). Characterization and catalytic properties of highly effective Ti-ZSM-5 zeolite prepared by gas−solid reaction. J. Chem. Ind. Eng. 57, 791–798. doi:10.3321/j.issn:0438-1157.2006.04.015
Liu, M., Guo, X., Wang, X., Liang, C., and Li, C. (2004). Effect of (n)SiO2/(n)B2O3 in the precursor on chemical–physics properties of Ti-ZSM-5 synthesized by gas–solid method. Catal. Today 93, 659–664. doi:10.1016/j.cattod.2004.06.026
Liu, X., Song, H., Sun, W., Wang, B., Zhang, P., Yuan, X., et al. (2020). Strong nano size effect of titanium silicalite (TS-1) zeolites for electrorheological fluid. Chem. Eng. J. 384, 123267. doi:10.1016/j.cej.2019.123267
Ma, S., Li, L., Liu, G., Lin, W., Su, K., and Li, Y. (1996). Synthesis and characterization of titanium-silicate zeolite TS-1. Chin. J. Catal. 17, 171–175.
Ma, Y., Tang, X., Hu, J., Ma, Y., Chen, W., Liu, Z., et al. (2022). Design of a small organic template for the synthesis of self-pillared pentasil zeolite nanosheets. J. Am. Chem. Soc. 144, 6270–6277. doi:10.1021/jacs.1c12338
Meng, X., and Xiao, F.-S. (2014). Green routes for synthesis of zeolites. Chem. Rev. 114, 1521–1543. doi:10.1021/cr4001513
Müller, U., and Steck, W. (1994). Ammonium-based alkaline-free synthesis of MFI-type boron and titanium zeolites. Stud. Surf. Sci. Catal. 84, 203–210.
Na, K., Jo, C., Kim, J., Ahn, W.-S., and Ryoo, R. (2011). MFI titanosilicate nanosheets with single-unit-cell thickness as an oxidation catalyst using peroxides. ACS Catal. 1, 901–907. doi:10.1021/cs2002143
Perego, C., Carati, A., Ingallina, P., Mantegazza, M. A., and Bellussi, G. (2001). Production of titanium containing molecular sieves and their application in catalysis. Appl. Catal. A General 221, 63–72. doi:10.1016/S0926-860X(01)00797-9
Prasad, M. R., Kamalakar, G., Kulkarni, S. J., Raghavan, K. V., Rao, K. N., Prasad, P. S. S., et al. (2002). An improved process for the synthesis of titanium-rich titanium silicates (TS-1) under microwave irradiation. Catal. Commun. 3, 399–404. doi:10.1016/s1566-7367(02)00144-9
Přech, J. (2018). Catalytic performance of advanced titanosilicate selective oxidation catalysts – A review. Catal. Rev. 60, 71–131. doi:10.1080/01614940.2017.1389111
Ren, L., Wu, Q., Yang, C., Zhu, L., Li, C., Zhang, P., et al. (2012). Solvent-free synthesis of zeolites from solid raw materials. J. Am. Chem. Soc. 134, 15173–15176. doi:10.1021/ja3044954
Ren, R., Song, W., Liu, G., Zang, J., and Yu, H. (2017). Recent research in preparation and application of hierarchical titanium silicalite-1 molecular sieve. J. Mol. Catal. 31, 594–604.
Shakeri, M., and Dehghanpour, S. B. (2019). Preparation of efficient TS-1 with small particle size and enhanced framework Ti. ChemistrySelect 4, 4771–4774. doi:10.1002/slct.201900457
Shan, Z., Lu, Z., Wang, L., Zhou, C., Ren, L., Zhang, L., et al. (2010). Stable bulky particles formed by TS-1 zeolite nanocrystals in the presence of H2O2. ChemCatChem 2, 407–412. doi:10.1002/cctc.200900312
Shen, Y., Li, H., Zhang, X., Wang, X., and Lv, G. (2020). The diquaternary ammonium surfactant-directed synthesis of single-unit-cell nanowires of ZSM-5 zeolite. Nanoscale 12, 5824–5828. doi:10.1039/D0NR00424C
Shibata, M., Gérard, J., and Gabelica, Z. (1997). Exploration of non conventional routes to synthesize MFI type titano- and (boro-titano)- zeolites. Stud. Surf. Sci. Catal. 105, 245–252. doi:10.1016/S0167-2991(97)80562-9
Taramasso, M., Perego, G., and Notari, B. (1983). “Preparation of porous crystalline synthetic material comprised of silicon and titanum oxdes,”. Patent no.US4410501A.
Thangaraj, A., Eapen, M. J., Sivasanker, S., and Ratnasamy, P. (1992). Studies on the synthesis of titanium silicalite, TS-1. Zeolites 12, 943–950. doi:10.1016/0144-2449(92)90159-M
Tuel, A. (1996). Crystallization of titanium silicalite-1 (TS-1) from gels containing hexanediamine and tetrapropylammonium bromide. Zeolites 16, 108–117. doi:10.1016/0144-2449(95)00109-3
Valtchev, V., Balanzat, E., Mavrodinova, V., Diaz, I., El Fallah, J., and Goupil, J.-M. (2011). High energy ion irradiation-induced ordered macropores in zeolite crystals. J. Am. Chem. Soc. 133, 18950–18956. doi:10.1021/ja208140f
Wang, J., Wang, Y., Tatsumi, T., and Zhao, Y. (2016). Anionic polymer as a quasi-neutral medium for low-cost synthesis of titanosilicate molecular sieves in the presence of high-concentration alkali metal ions. J. Catal. 338, 321–328. doi:10.1016/j.jcat.2016.03.027
Wang, L., Liu, Y., Xie, W., Zhang, H., Wu, H., Jiang, Y., et al. (2007). Highly efficient and selective production of epichlorohydrin through epoxidation of allyl chloride with hydrogen peroxide over Ti-MWW catalysts. J. Catal. 246, 205–214. doi:10.1016/j.jcat.2006.12.003
Wang, L., Xu, Y., Zhai, G., Zheng, Y., Huang, J., Sun, D., et al. (2020). Biophenol-mediated solvent-free synthesis of titanium silicalite-1 to improve the acidity character of framework Ti toward catalysis application. ACS Sustain. Chem. Eng. 8, 12177–12186. doi:10.1021/acssuschemeng.0c03685
Wang, W., Li, G., Liu, L., and Chen, Y. (2013). Titanium silicalite synthesized by dry gel conversion method and its catalytic performance. Chin. J. Catal. 33, 1236–1241. doi:10.3724/SP.J.1088.2012.20226
Wang, X., Ma, Y., Wu, Q., Wen, Y., and Xiao, F.-S. (2022). Zeolite nanosheets for catalysis. Chem. Soc. Rev. 51, 2431–2443. doi:10.1039/D1CS00651G
Wang, Y., Li, L., Bai, R., Gao, S., Feng, Z., Zhang, Q., et al. (2021). Amino acid-assisted synthesis of TS-1 zeolites containing highly catalytically active TiO6 species. Chin. J. Catal. 42, 2189–2196. doi:10.1016/S1872-2067(21)63882-2
Wu, L., Deng, X., Zhao, S., Yin, H., Zhuo, Z., Fang, X., et al. (2016). Synthesis of a highly active oxidation catalyst with improved distribution of titanium coordination states. Chem. Commun. 52, 8679–8682. doi:10.1039/C6CC03318K
Wu, M., Liu, X., Wang, Y., Guo, Y., Guo, Y., and Lu, G. (2014a). Synthesis and catalytic ammoxidation performance of hierarchical TS-1 prepared by steam-assisted dry gel conversion method: The effect of TPAOH amount. J. Mat. Sci. 49, 4341–4348. doi:10.1007/s10853-014-8130-6
Wu, P., and Tatsumi, T. (2004). A new generation of titanosilicate catalyst: Preparation and application to liquid-phase epoxidation of alkenes. Catal. Surv. Asia 8, 137–148. doi:10.1023/B:CATS.0000027015.37277.fb
Wu, P., Tatsumi, T., Komatsu, T., and Yashima, T. (2001). A novel titanosilicate with MWW structure. I. Hydrothermal synthesis, elimination of extraframework titanium, and characterizations. J. Phys. Chem. B 105, 2897–2905. doi:10.1021/jp002816s
Wu, Q., Wang, X., Qi, G., Guo, Q., Pan, S., Meng, X., et al. (2014b). Sustainable synthesis of zeolites without addition of both organotemplates and solvents. J. Am. Chem. Soc. 136, 4019–4025. doi:10.1021/ja500098j
Wu, Q., Zhu, L., Chu, Y., Liu, X., Zhang, C., Zhang, J., et al. (2019). Sustainable synthesis of pure silica zeolites from a combined strategy of zeolite seeding and alcohol filling. Angew. Chem. Int. Ed. 58, 12138–12142. doi:10.1002/anie.201906559
Xing, J., Yuan, D., Liu, H., Tong, Y., Xu, Y., and Liu, Z. (2021). Synthesis of TS-1 zeolites from a polymer containing titanium and silicon. J. Mat. Chem. A 9, 6205–6213. doi:10.1039/D0TA11876A
Xu, F., Dong, M., Gou, W., Li, J., Qin, Z., Wang, J., et al. (2012). Rapid tuning of ZSM-5 crystal size by using polyethylene glycol or colloidal silicalite-1 seed. Microporous Mesoporous Mater. 163, 192–200. doi:10.1016/j.micromeso.2012.07.030
Xu, H., Chen, W., Zhang, G., Wei, P., Wu, Q., Zhu, L., et al. (2019). Ultrathin nanosheets of aluminosilicate FER zeolites synthesized in the presence of a sole small organic ammonium. J. Mat. Chem. A 7, 16671–16676. doi:10.1039/C9TA04833B
Xu, W., Dong, J., Li, J., Li, J., and Wu, F. (1990). A novel method for the preparation of zeolite ZSM-5. J. Chem. Soc. Chem. Commun. 755. doi:10.1039/c39900000755
Xu, W., Zhang, T., Bai, R., Zhang, P., and Yu, J. (2020). A one-step rapid synthesis of TS-1 zeolites with highly catalytically active mononuclear TiO6 species. J. Mat. Chem. A 8, 9677–9683. doi:10.1039/C9TA13851J
Xu, Z., Zhang, Y., and Zheng, L. (1992). The secondary synthesis of zeolites via framework substitution for aluminum Ⅱ. Preparation and characterization of zeolite containing Ti and Fe elements. J. Mol. Catal. 6, 365–370. doi:10.16084/j.cnki.issn1001-3555.1992.05.007
Yang, G., Han, J., Liu, Y., Qiu, Z., and Chen, X. (2020a). The synthetic strategies of hierarchical TS-1 zeolites for the oxidative desulfurization reactions. Chin. J. Chem. Eng. 28, 2227–2234. doi:10.1016/j.cjche.2020.06.026
Yang, G., Han, J., Qiu, Z., Chen, X., Feng, Z., and Yu, J. (2020b). An amino acid-assisted approach to fabricate nanosized hierarchical TS-1 zeolites for efficient oxidative desulfurization. Inorg. Chem. Front. 7, 1975–1980. doi:10.1039/C9QI01543D
Yang, Y., Li, H., He, D., Wang, Y., Xu, C., Qiu, F., et al. (2006). Study on the catalytic properties of TS-1 molecular sieves synthesized under microwave irradiation. Acta Chem. Sin. 64, 1411–1415. doi:10.3321/j.issn:0567-7351.2006.14.001
Yoo, J. W., Lee, C. W., Chang, J.-S., Park, S.-E., and Ko, J. (2000). Characterization and catalytic properties of Ti-ZSM-5 prepared by chemical vapor deposition. Catal. Lett. 66, 169–173. doi:10.1023/a:1019059925685
You, Z., Liu, G., Wang, L., and Zhang, X. (2013). Binderless nano-HZSM-5 zeolite coatings prepared through combining washcoating and dry-gel conversion (DGC) methods. Microporous Mesoporous Mater. 170, 235–242. doi:10.1016/j.micromeso.2012.12.012
Yue, M. B., Sun, M. N., Xie, F., and Ren, D. D. (2014). Dry-gel synthesis of hierarchical TS-1 zeolite by using P123 and polyurethane foam as template. Microporous Mesoporous Mater. 183, 177–184. doi:10.1016/j.micromeso.2013.09.029
Zhang, F. Z., Guo, X. W., Wang, X. S., Li, G. Y., Zhao, Q., Bao, X. H., et al. (1999). Preparation and characterization of titanium-containing MFI from highly siliceous ZSM-5: Effect of precursors synthesized with different templates. Mater. Chem. Phys. 60, 215–220. doi:10.1016/S0254-0584(99)00098-X
Zhang, H., Fan, Y. F., Huan, Y. H., and Yue, M. B. (2016a). Dry-gel synthesis of shaped transition-metal-doped M-MFI (M = Ti, Fe, Cr, Ni) zeolites by using metal-occluded zeolite seed sol as a directing agent. Microporous Mesoporous Mater. 231, 178–185. doi:10.1016/j.micromeso.2016.06.001
Zhang, H., Liu, Y., He, M., and Wu, P. (2006). Synthesis of TS-1 with high catalytic performance using inorganic SiO2-TiO2 precursor. Chin. J. Catal. 27, 749–751. doi:10.3321/j.issn:0253-9837.2006.09.001
Zhang, H., Liu, Y., Jiao, Z., He, M., and Wu, P. (2009). Hydrothermal synthesis of titanium silicalite-1 structurally directed by hexamethyleneimine. Ind. Eng. Chem. Res. 48, 4334–4339. doi:10.1021/ie8016253
Zhang, J., Shi, H., Song, Y., Xu, W., Meng, X., and Li, J. (2021). High-efficiency synthesis of enhanced-titanium and anatase-free TS-1 zeolite by using a crystallization modifier. Inorg. Chem. Front. 8, 3077–3084. doi:10.1039/D1QI00311A
Zhang, T., Chen, X., Chen, G., Chen, M., Bai, R., Jia, M., et al. (2018). Synthesis of anatase-free nano-sized hierarchical TS-1 zeolites and their excellent catalytic performance in alkene epoxidation. J. Mat. Chem. A 6, 9473–9479. doi:10.1039/C8TA01439F
Zhang, T., Zuo, Y., Liu, M., Song, C., and Guo, X. (2016b). Synthesis of titanium silicalite-1 with high catalytic performance for 1-butene epoxidation by eliminating the extraframework Ti. ACS Omega 1, 1034–1040. doi:10.1021/acsomega.6b00266
Zhu, L., Zhang, J., Wang, L., Wu, Q., Bian, C., Pan, S., et al. (2015). Solvent-free synthesis of titanosilicate zeolites. J. Mat. Chem. A 3, 14093–14095. doi:10.1039/C5TA02680F
Zuo, Y., Wang, X., and Guo, X. (2011). Synthesis of titanium silicalite-1 with small crystal size by using mother liquid of titanium silicalite-1 as seed. Ind. Eng. Chem. Res. 50, 8485–8491. doi:10.1021/ie200281v
Zuo, Y., Wang, X., and Guo, X. (2012). Synthesis of titanium silicalite-1 with small crystal size by using mother liquor of titanium silicalite-1 as seeds (II): Influence of synthesis conditions on properties of titanium silicalite-1. Microporous Mesoporous Mater. 162, 105–114. doi:10.1016/j.micromeso.2012.06.016
Keywords: zeolite, TS-1, synthesis, raw materials, routes, nanoscale
Citation: Luan H, Xu C, Wu Q and Xiao F-S (2022) Recent advances in the synthesis of TS-1 zeolite. Front. Chem. 10:1080554. doi: 10.3389/fchem.2022.1080554
Received: 26 October 2022; Accepted: 09 November 2022;
Published: 22 November 2022.
Edited by:
Jingang Jiang, East China Normal University, ChinaCopyright © 2022 Luan, Xu, Wu and Xiao. This is an open-access article distributed under the terms of the Creative Commons Attribution License (CC BY). The use, distribution or reproduction in other forums is permitted, provided the original author(s) and the copyright owner(s) are credited and that the original publication in this journal is cited, in accordance with accepted academic practice. No use, distribution or reproduction is permitted which does not comply with these terms.
*Correspondence: Qinming Wu, cWlubWluZ3d1QHpqdS5lZHUuY24=; Feng-Shou Xiao, ZnN4aWFvQHpqdS5lZHUuY24=