- 1School of Chinese Materia Medica, Tianjin University of Traditional Chinese Medicine, Tianjin, China
- 2State Key Laboratory of Microbial Technology, Institute of Microbial Technology, Shandong University, Qingdao, China
Lichens are important sources of versatile bioactive compounds. Two new dibenzofurans (1–2), a multi-substituted single benzene ring (3), and two organic acid compounds (4–5) along with 25 known compounds (6–30) were isolated from the lichen Usnea diffracta Vain. Their structures were identified by physicochemical properties and spectral analyses. Compounds 1–30 were tested for inhibitory activities against Staphylococcus aureus, Escherichia coli, and Candida albicans by the disk diffusion method and microdilution assay respectively. Compound 3 showed moderate inhibitory activities against S. aureus and E. coli with the inhibition zone (IZ) of 6.2 mm and 6.3 mm, respectively. Depside 10 exhibited good activity against S.aureus and C. albicans with 6.6 mm and 32 μg/ml, respectively. The acetylcholinesterase inhibitory activities of compounds 1, 2, and 6–8 with the characteristic dibenzofuran scaffold were evaluated var anti-AChE assay and a molecular docking study. Compound 2 could better inhibit AChE at the concentration of 0.3 μmol/ml with a value of 61.07 ± 0.85%. The molecular docking study also demonstrated that compound 2 had the strongest binding affinity among the five dibenzofurans, and the “-CDOCKER Energy” value was 14.4513 kcal/mol.
1 Introduction
Lichens are autotrophic symbionts which are composed of mycobiontic fungi (mainly Ascomycetes) and one or more photosynthetic organisms (algae or cyanobacteria). Lichens have a remarkable ability to survive in extreme environmental conditions ranging from deserts to polar areas. Their specific secondary metabolites can protect them from physical and biological attacks (Reddy et al., 2019; Popovici et al., 2022). Lichens are not only ecologically important but also significant medicinal natural product resources, especially phenolic secondary metabolites, including phenols (orinol), dibenzofurans (usnic acid and its derivatives), depsidones (norstictic acid), depsides (diffractaic acid), depsones (picrolichenic acid), and pulvinic acid derivatives (vulpinic acid). There are about 26,000 species of lichens belonging to 500 genera in the world, among which more than 360 Usnea species are found (Salgado et al., 2018).
Usnea diffracta Vain, belonging to the genus Usnea, is widely used as a folk medicine for the treatment of diarrhea, stomachache, ulcer, tuberculosis, pneumonia, malaria, wounds, and parasitic diseases (Singh B. et al., 2016; Zhao et al., 2019). Depsidones, depsides, depsones, quinones, polyphenolics, polysac-charides, and dibenzofurans are the main compounds of Usnea (Salgado et al., 2018). Previous studies have showed numerous good pharmacological activities of U. diffracta, such as antimicrobial, antioxidant, antitumor, antiviral, anti-inflammatory, cardiovascular protective, anti-genotoxic, and antiproliferative effects (Behera et al., 2012; Lai et al., 2013; Ceker et al., 2015; Koparal, 2015; Nishanth et al., 2015; Singh S. et al., 2016; Vanga et al., 2017; Yang et al., 2017). It is noteworthy that most of these pharmacological activities are attributed to the availability of bioactive depsides and dibenzofuran derivatives (especially usnic acid) (Sun et al., 2016). Usnea diffracta and its major constituent usnic acid are potent antimicrobial agents to inhibit growth of different planktonic bacteria and fungi strains (Helena et al., 2012).
Alzheimer’s disease (AD) is a serious degenerative disorder of the central nervous system, characterized by a variety of symptoms, including memory loss, emotional behavior, and cognitive impairment (Singh et al., 2013). One of its pathogeneses mainly includes cholinergic system damage. The cholinergic system of AD patients involves two main cholinesterases (ChE), acetylcholin-esterase (AChE) and butyrylcholinesterase (BChE) (Colovic Mirjana et al., 2013). AChE inhibitors mainly act on the catalytic active site (CAS) at the bottom of AChE, which can relieve the symptoms of mild-to-moderate AD patients (Zhu et al., 2019). At present, cholinesterase inhibitors are mainly selective acetycholinesterase inhibitors (AChEIs) such as tacrine, donepezil, and galantamine (Greig et al., 2005; Furukawa-Hibi et al., 2011; Wu et al., 2016). These drugs have greater side effects or lower response rates in the long-term treatment of patients with mild-to-moderate AD. In order to find natural lead compounds for the treatment of Alzheimer’s disease, benzofurans, as an important pharmacophore, have received extensive attention in the past few years because of their attractive cholinesterase inhibitory activity (Rizzo et al., 2012; Khanam and Shamsuzzaman, 2015; Delogu et al., 2016; Wang et al., 2017). The bibenzofuran structures of usnic acid derivatives are similar to those of galantamine, an anticholinesterase drug for AD. Usnic acid enantiomers show antioxidant and anti-inflammatory activities, which are involved in AD pathogenesis (Araújo et al., 2015; Cazarin et al., 2020).
Hence, in the current research, 30 compounds including five new compounds were isolated and identified from U. diffracta. The complete isolation and structural elucidation of all compounds and their antimicrobial activities against two pathogenic bacteria including one Gram-positive strain (Staphylococcus aureus) and one Gram-negative bacterial strain (Escherichia coli) and fungus strain (Candida albicans) were described. Among them, the inhibitory activities on AChE of usnic acid derivatives 1, 2, and 6–8 were reported. The interaction between these compounds and the active centers of AChE were investigated by molecular docking.
2 Results
2.1 Isolated compounds from Usnea diffracta Vain
Thirty compounds (Figure 1) were separated and identified from the ethanol extract of the dry lichen body of U. diffracta Vain, including five dibenzofuran compounds (1, 2, and 6–8), eleven multi-substituted single benzene ring compounds (3 and 13–22) and four depsides compounds (9–12), four organic acid compounds (4, 5, 24, and 25), one organic acid ester compound (23), two fatty acids containing furan ring (26 and 27), one furfural compound (28), one amino acid compound (29), and one nucleoside compound (30). After searching the SciFinder database, compounds 1–5 were identified as new compounds and their structural analysis as follows.
2.1.1 Usenamine G (1)
Pale yellow oil (CH3OH). [α]25D -132.0° (c 0.05, CH3OH); CD (0.59 mg/ml, CH3OH) λmax (Δε) 304 (−2.19) nm, 278 (+1.74) nm; UV (CH3OH) λmax (log ε): 223 (3.92) nm, 296 (4.16) nm; IR (KBr): νmax 3426, 1639, 1541, 1420, and 1015 cm−1; 1H NMR (500 MHz, CD3OD) and 13C NMR (125 MHz, CD3OD) data; HR-ESI-MS m/z 476.1931 [M + H]+ (Calcd. For C24H29NO9, 476.1921) (Table 1; Supplementary Figures S1–S11).
The 1H NMR data of 1 in CD3OD showed signals for five methyl groups: δ 1.23 (3H, t), 1.55 (3H, s), 1.96 (3H, s), 2.52 (3H, s), and 2.55 (3H, s); four methylene groups: δ 2.43 (2H, t), 1.94–2.01 (2H, m), 3.59 (2H, t), 3.88 (1H, q) and 3.87 (1H, q); two single hydrogen signals: δ 3.10 (1H, d) and 3.15 (1H, d). The coupling constants at δ 3.10 and 3.15 are both 15.4 Hz, which is speculated to be a carbon coupling hydrogen signal. The 13C NMR spectrum showed 24 carbon resonances that consisted of three carbonyl signals (δ 202.8, 200.0, and 194.1), aromatic carbon signals and alkene carbon signals (δ 161.2, 163.8, 158.5, 107.6, 106.8, 106.5, and 102.3), the chemical shifts 176.2 and 176.1 may contain carboxyl signals, as well as oxycarbon and aliphatic carbon signals. According to the DEPT 135 spectra (Supplementary Figure S11), five methylenes (δ 60.7, 44.2, 43.7, 31.7, and 25.4) were existed in compound 1. The HSQCs (Supplementary Figure S8) indicated directly linked hydrocarbon correlations. Combined with 1H-1H COSY, the correlations (Figure 2; Supplementary Figure S7) from δH 3.10 to δH 3.15, δH 1.23 to δH 3.88/3.87, and δH 1.97 to δH 2.43/3.59 further verified the existence of -CH2-, -OCH2CH3, and -CH2-CH2-CH2-. The HMBCs (Figure 2; Supplementary Figure S9) from δH 1.55 to δC 200.0/111.6/107.6/61.2 indicated that the angular methyl group was located at C-9b, while those from δH 2.55 to δC 102.3/202.8 suggested that C-14 was connected to C-6. The correlations from δH 2.52 to δC 106.8/176.1 established the connection of C-11 with C-2. The HMBCs from δH 3.88/3.87 to δC 15.7/111.6 suggested the connection of an ethoxy group at C-4a and from δH 2.43/1.94–2.01 to δC 176.2 and δH 3.59 to δC 176.1 revealed the presence of a C-2 enamine moiety in compound 1. The configuration of the double bond was determined by the NOESY data (Figure 2; Supplementary Figure S10) δH (2.52) - δH (3.59) as E, analysis of the correlation between δH (1.55/2.55) and δH (3.88/3.87) dedicated that 9b-CH3 and 4a-OCH2CH3 were on the same side. In addition, the CD spectrum (Supplementary Figure S2) of compound 1 was compared with the related literature previously published (Yu X. et al., 2016). Compound 1 presented one negative maximum at 298 nm and positive at 278 nm, which was consistent with the CD spectrum of Usneamine B, indicating that the absolute configuration of compound 1 was 4aR/9bR, as represented in Figure 2. Overall, compound 1 was identified as a new compound by SciFinder inquiry, named Usenamine G.
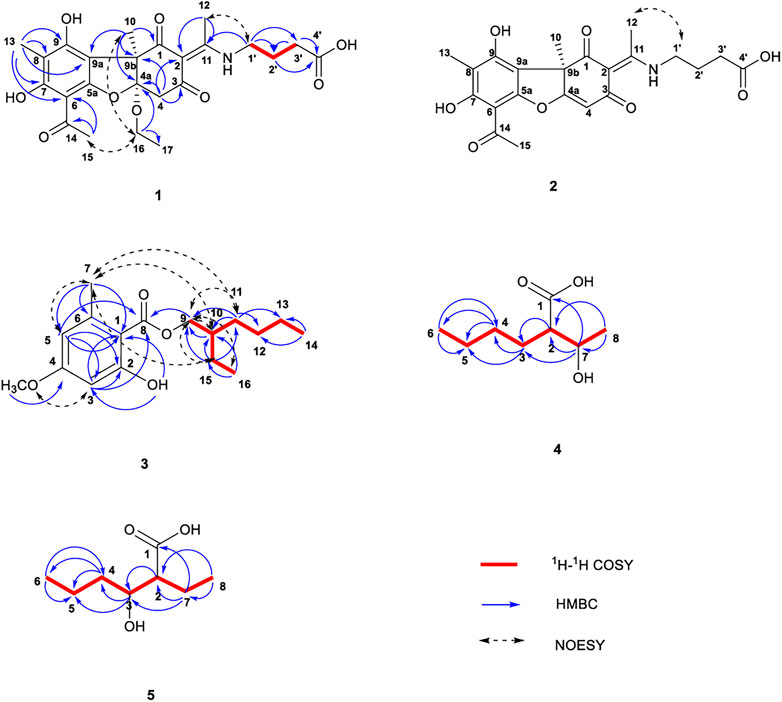
FIGURE 2. Chemical structures of compounds 1–5 along with the key 1H-1H COSY, HMBC, and NOESY interactions.
2.1.2 Usenamine H (2)
Pale yellow oil (CH3OH). [α]25D +104.0° (c 0.05, CH3OH); CD (0.59 mg/mL, CH3OH) λmax (Δε) 332 (+0.59) nm; 1H NMR (600 MHz, DMSO-d6) and 13C NMR (150 MHz, DMSO-d6) data; HR-ESI-MS m/z 430.1518 [M + H]+ (Calcd. For C22H23NO8, 430.1502) (Table 1; Supplementary Figures S12–S16). A comparison of the 1H and 13C NMR data of compound 2 with the 1H (500 MHz, CD3OD) and 13C NMR (125 MHz, CD3OD) data of 1 revealed their structural similarities, except for the presence of alkene proton signal at δH 5.87 (1H, s)/δC 101.4 (C-4), and the absence of -OCH2 - (C-16) and -CH3 segments (C-17). The NOESY correlation (Supplementary Figure S16) between H-12 (δ 2.58) and H-1′ (δ 3.54–3.59) suggested an E-configuration for the double bond and it was the same as compound 1 (Figure 2). The similar sign of the specific rotation [α]25D +104.0° (c .05, CH3OH) and positive at 333 nm, which was consistent with the CD spectrum of Usneamine A (Yu X. et al., 2016), indicating that the absolute configuration was 9bR, as represented in Figure 2. The spectroscopic data were in accord with the literature (Bruno et al., 2013), compound 2 was identified as a new compound by SciFinder inquiry, named Usneamine H.
2.1.3 2-Ethylhexyl-4-methoxy orsellinate (3)
White oil (CH3OH). [α]25D -12.0° (c 0.05, CH3OH); CD (0.09 mg/ml, CH3OH) λmax (Δε) 227 (+0.28) nm, 253 (+0.08) nm, 266 (-0.06) nm, 227 (+0.03) nm; UV (CH3OH) λmax (log ε): 215 (4.27) nm, 263 (4.03) nm, 301 (3.61) nm; IR (KBr): νmax 3462, 2960, 2931, 1653, 1617, 1576, 1456, 1257, 1159 cm−1; 1H NMR (500 MHz, CDCl3) and 13C NMR (125 MHz, CDCl3) data; HR-ESI-MS m/z 295.1897 [M + H]+ (Calcd. For C17H26O4, 295.1909) (Table 1; Supplementary Figures S17–S27).
The 1H NMR data of 3 in CDCl3 showed signals for a hydroxyl: δ 11.95 (1H, s); two m-position hydrogen of benzene ring: δ 6.33 (1H, d, J = 2.4 Hz) and 6.28 (1H, d, J = 2.4 Hz); a -O-CH2- fragment: δ 4.27 (2H, m); a methoxy group: δ 3.80 (3H, s); a methyl attached to the benzene ring: δ 2.52 (3H, s); an aliphatic chain: δ 1.71 (1H, m), 1.45 (2H, m), 1.39 (2H, m), and 1.32 (4H, m); and two terminal methyl of aliphatic chain: δ 0.94 (3H, t, J = 7.5 Hz) and 0.90 (3H, t, J = 7.0 Hz). The 13C NMR spectrum revealed 17 carbon resonances that consisted of a -COO- fragment signal (δ 172.2), a -O-CH2- fragment signal (δ 67.6), a -O-CH3 fragment signal (δ 55.3), six aromatic carbon signals (δ 105.5, 165.7, 98.8, 163.8, 111.1, and 143.0) and eight aliphatic carbon signals (δ 38.8, 30.6, 29.0, 24.6, 24.0, 23.0, 14.0, and 11.1). The DEPT spectra (Supplementary Figure S27) demonstrated five methylene signals: δ 67.6, 30.6, 29.0, 24.0, and 23.0. The HSQCs (Supplementary Figure S24) indicated directly linked hydrocarbon correlations. According to the HMBCs (Figure 2; Supplementary Figure S25), correlations from δH (0.94) to δC (24.0/38.8), δH (0.90) to δC (23.0/29.0), δH (1.32) to δC (14.0/23.0/29.0/30.6), and δH (1.39) to δC (23.0/29.0) showed the presence of -CH2-CH2-CH2-CH3 fragment in the aliphatic chain, and δH (0.94) to δH (1.45) showed -CH2-CH3. The correlations from δH (1.38) to δC (38.0/67.6), δH (1.45) to δC (67.6/38.8/11.1/30.6), δH (1.71) to δC (67.6/24.0/11.1/30.6), and δH (4.27) to δC (23.0/30.6/38.8/172.7) together indicated the fragment of CH3-CH2-CH-CH2-OOC-, in which -CH- was linked to the butyl group. The δH (2.51)–δC (143.0/111.1/105.5) and δH (3.80)–δC (163.8) revealed that the methyl and oxymethyl existed on the benzene ring. At the same time combined with COSY correlations (Supplementary Figure S23), the planar structure of compound 3 was shown in Figure 2. The main hydrogen-related signals shown by the NOESY spectra (Figure 2; Supplementary Figure S26) were δH (4.27)–δH (0.94/1.32/1.39/1.45/1.71), δH (2.51)–δH (1.32/1.39/1.45/1.71), δH (6.33)–δH (3.80), and δH (6.28)–δH (2.52). In addition, the CD spectrum (Supplementary Figure S18) of compound 3 was compared with the related literature previously published (Nahrstedt et al., 1990). Compound 3 presented one negative which was consistent with (S)-2-Methylbutan-1-yl-β-D-glucopyranoside, indicating that the absolute configuration of C-10 was S. Through SciFinder database query, it was confirmed that compound 3 was a new compound and shared the similar structure with 2-ethylhexyl orsellinate (Bui et al., 2020), named 2-ethylhexyl-4-methoxy orsellinate.
2.1.4 2-(1-Hydroethyl)-hexanoic acid (4)
White oil (CH3OH). [α]25D -8.0° (c 0.07, CH3OH); CD (0.56 mg/mLl CH3OH) λmax (Δε) 199 (-0.22) nm; UV (CH3OH) λmax (log ε): 202 (2.68) nm, 222 (2.35) nm, 274 (1.70) nm; IR (KBr): νmax 3416, 2958, 2932, 1694, 1403, 1204, and 1119 cm−1; 1H NMR (600 MHz, CD3OD) and 13C NMR (150 MHz, CD3OD) data; HR-ESI-MS m/z 159.1038 [M–H]- (Calcd. For C8H16O3, 159.1021) (Table 1; Supplementary Figures S28–S38).
The 1H NMR data of compound 4 in CD3OD showed signals for 14 hydrogen atoms. According to the chemical shift, 1.19 (3H, d, J = 6.3 Hz), it is inferred that there is a -CH-CH3 fragment in the structure, and the shift 0.91 (3H, t, J = 6.9 Hz) showed the existence of -CH2-CH3. The 13C NMR spectrum showed eight carbon resonances that consisted of one carbonyl signals (δ 179.6), one -O-C- fragment signal (δ 69.7), one -O-CH3 fragment signal (δ 55.4), and five aliphatic carbon signals (δ 31.0, 29.6, 23.8, 21.3, and 14.3). According to the DEPT 135 spectra (Supplementary Figure S38), three methylenes (δ 31.0, 29.6, and 23.8) were existed in compound 4. The HSQC spectrum (Supplementary Figure S35) revealed directly linked hydrocarbon correlations. Correlations presented in 1H-1H COSY (Figure 2; Supplementary Figure S34) from δH (1.19) to δH (3.86), δH (3.86) to δH (2.28), δH (1.51/1.58) to δH (1.34/2.28), and δH (1.34) to δH (.91) further verified the existence of -CH-CH3 and -CH2-CH2-CH3. The HMBCs (Figure 2; Supplementary Figure S36) from δH (.91/1.51/1.58) to δC (23.8/31.0) and δH (1.34) to δC (14.3/23.8/31.0) indicated the presence of CH3-CH2-CH2-CH2-. The correlations from δH (2.28) to δC (21.3/29.6/69.7) and δH (3.86) to δC (29.6/55.4/179.6) showed the fragment of COOH-CH-CH-OH. The signal of δH (1.19) - δC (55.4/69.7) confirmed that the terminal methyl (C-8) was connected to C-7. The main hydrogen-related signals shown by the NOESY spectra (Supplementary Figure S37) were δH (2.28) - δH (3.86/1.51/1.58/1.34/1.21) and δH (3.86) - δH (1.51/1.58/1.21). The structure and spectroscopic data were shown in Figure 2 and Table 1. Overall, compound 4 was identified as a new compound by SciFinder inquiry, named 2-(1-hydroethyl)-hexanoic acid. Its configuration needs to be further determined.
2.1.5 2-Ethyl-3-hydroxyhexanoic acid (5)
White oil (CH3OH). [α]25D -8.0° (c 0.05, CH3OH); 1H NMR (600 MHz, CD3OD) and 13C NMR (150 MHz, CD3OD) data; HR-ESI-MS m/z 159.0546 [M–H]- (Calcd. For C8H16O3, 159.1021) (Table 1; Supplementary Figures S39–S46).
A comparison of the 1H and 13C NMR data of compound 5 and compound 4 revealed their structural similarities, except for the presence of two proton signals at δH 1.60 (2H, m)/δC 23.1 (C-7) and one proton signal at δH 3.67 (1H, m)/δC 73.0 (C-3). According to the chemical shift, it is inferred that the hydroxyl group (C-7) of compound 4 was connected to the C-3 in compound 5. Also, the 1H NMR data of compound 5 showed signals for 14 hydrogen and the 13C NMR spectrum showed eight carbon resonances. According to the DEPT 135 spectra (Supplementary Figure S46), three methylenes were existed in compound 5. The HSQC spectrum (Supplementary Figure S43) revealed directly linked hydrocarbon correlations. Correlations presented in 1H-1H COSY (Supplementary Figure S42) from δH (1.50) to δH (3.67) and δH (1.60) to δH (0.94) further verified the existence of -CH2-CH2-CH-OH and -CH2-CH3. The relations of δH (0.94)–(1.60/3.67) showed that the -CH-COOH fragment was linked to -CH-OH and -CH2-CH3 fragment and δH (0.94)–(1.38) indicated that the terminal methyl was connected to the -CH2-CH2-. The correlations of H-2/C-1, 3, 4, 7, 8; H-3/C-1, 2, 4, 5; H-4/C-3, 5, 6; H-5/C-3, 4, 6; H-6/C-4, 5; H-7/C-1, 2, 3, 4, 8; and H-8/C-2, 7 were observed in HMBC (Supplementary Figure S44) and H-2/H-4, 7, 3 and H-6/H-4, 7, 5 in NOESY (Supplementary Figure S45). The structure and spectroscopic data were shown in Figure 2; Table 1. The configuration of the two chiral carbons could not be determined. Compound 5 was identified as a new compound by SciFinder inquiry, named 2-ethyl-3-hydroxyhexanoic acid (Wahl et al., 2001).
The known compounds isolated and identified by spectroscopic techniques were Usneamine F (6) (Yu X. et al., 2016), Usneamine E (7) (Yu X. et al., 2016), (+)-usnic acid (8) (Laxi et al., 2013), 2′-O-methylevernol (9) (Huneck et al., 1989), 3-hydroxy-5-methylphenyl-2-hydroxy-4-methoxy-6-methylbenzoate (10) (Yu X.L. et al., 2016), evernic acid (11) (Tang et al., 2015), lecanorin (12) (Bui et al., 2020), ethyl everninate (13) (Yu X.L. et al., 2016), evernesic acid (14) (Nishitoba et al., 1987), methyl-2,4-dihydroxy-3,6-dimethylbenzoate (15) (Feng et al., 2009), bis-(2S-ethylhexyl)benzene-1,2-dicarboxylate (16) (Su et al., 2009), dibutyl phthalate (17) (Li et al., 2009), orsellinaldehyde (18) (Zheng et al., 2012), ethyl orsellinate (19) (Tuan et al., 2020), n-butyl orsellinate (20) (Li et al., 2006), orcinol (21) (Eliwa et al., 2019), orsellinic acid (22) (Wang et al., 2009), α-linolenic acid methyl ester (23) (Zou et al., 2021), α-linolenic acid (24) (Qin et al., 2010), linoleic acid (25) (Zhao et al., 2020), constipatic acid (26) (Chester and Elix, 1979), 18R-hydroxy-dihydroalloprotolichesterinic acid (27) (Rezanka and Guschina, 2001), 5-hydromethyl furaldehyde (28) (Feng et al., 2011), 4-(acylamino)butyramides (29) (Graham et al., 1987), and uridine (30) (Gu et al., 2017); (Figure 1).
2.2 Antimicrobial activity
In this study, the antimicrobial activities of the isolated compounds were studied in vitro. The inhibition zone of the compounds against S. aureus and E. coli were determined by the disk diffusion method and the values of minimal inhibitory concentration (MIC) against C. albicans by microdilution assay. The results (Table 2) revealed that when the amount of compound was 3.2 μg/disk, the inhibition zone (IZ) of compounds 3, 9, 10, and 18 were 6.2, 6.4, 6.6, and 6.6 mm, respectively, and others had no effect in the preliminary screening experiment of anti-S. aureus. Among them, 10 and 18 exhibited better antibacterial activities against S. aureus than other compounds, but lower than the positive control gentamicin sulfate. In addition, compounds 3 and 30 can better inhibit E. coli with IZ of 6.3 mm than other compounds at 3.2 μg/disk. The assay of anti-C. albicans showed that the MIC value of tested compound 10 was 32 μg/ml, which exhibited the strongest inhibitory activity among all compounds. In conclusion, the results of the preliminary screening demonstrated that compound 3 had certain inhibitory activities against S. aureus and E. coli but no anti-C. albicans activity at 64 μg/ml, compound 9 had certain inhibitory effect on S. aureus and C. albicans with MIC of 64 μg/ml. Furthermore, in this preliminary screening, compound 10 demonstrated the strongest inhibition against S. aureus and C. albicans among all compounds.
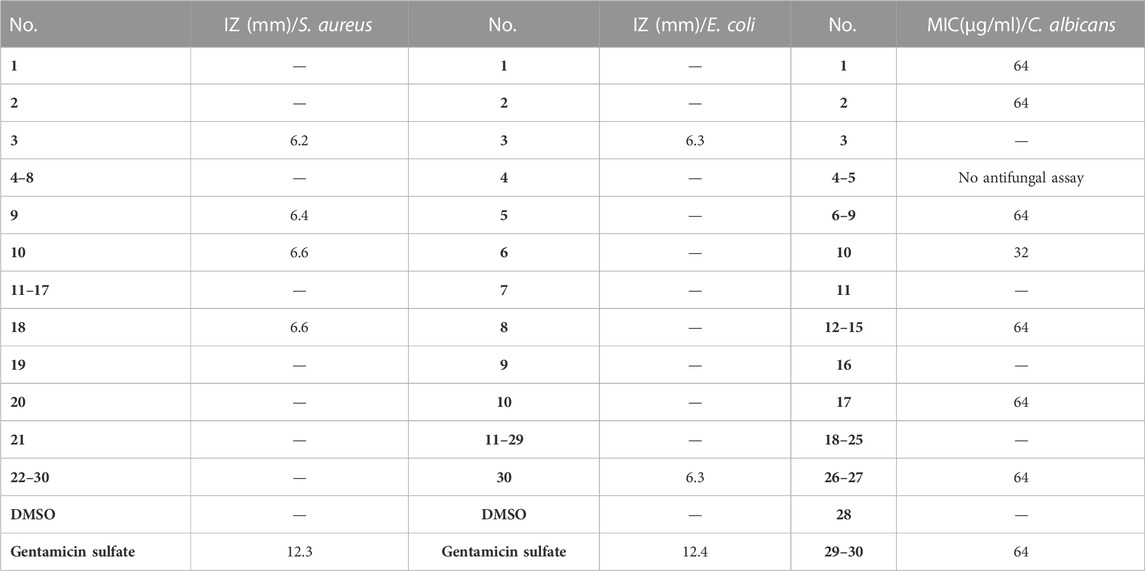
TABLE 2. Antimicrobial activities of compounds from U. diffracta Vain. (The IZ value indicated the diameter of the inhibition zone at 3.2 μg/disk. The MIC value indicated the minimal inhibitory concentration. “—” indicated no inhibitory activity in 3.2 μg/disk or 64 μg/ml.)
2.3 Acetylcholinesterase inhibitory activity
The activities of five dibenzofurans [Usneamine G (1), Usenamine H (2), Usneamine F (6), Usneamine E (7), and (+)-usnic acid (8)] against AChE were evaluated in the current work. Compound 2 (at the concentration of 0.3 μmol/ml) exhibited the strongest AChE inhibitory activity among the five compounds, with the inhibition value of 67.56 ± 0.49% (as shown in Table 3). Others also exhibited moderate AChE inhibition at the concentration of 0.3 μmol/ml with values of 61.18 ± 1.16%, 61.07 ± 0.85%, 57.61 ± 0.23%, and 58.06 ± 0.73%, respectively.
2.4 Molecular docking study
In order to study the binding affinity related to the inhibition of acetylcholinesterase, five compounds of dibenzofurans: Usneamine G (1), Usenamine H (2), Usneamine F (6), Usneamine E (7), and (+)-usnic acid (8) were simulated. The results of molecular docking revealed that the five compounds had good binding affinities with the active pockets of AChE, and the ‘-CDOCKER Energy’ values were 1.01935 kcal/mol, 14.4513 kcal/mol, -1.95894 kcal/mol, 12.8461 kcal/mol, and -3.65539 kcal/mol, respectively, higher than that of the original ligand galantamine (-9.06147 kcal/mol). Among them, compound 2 exhibited the highest AChE inhibition, which was consistent with the previous work. The interactions data for compounds 1, 2, and 6–8 docked into the active site gorge of AChE is shown in Table 4.
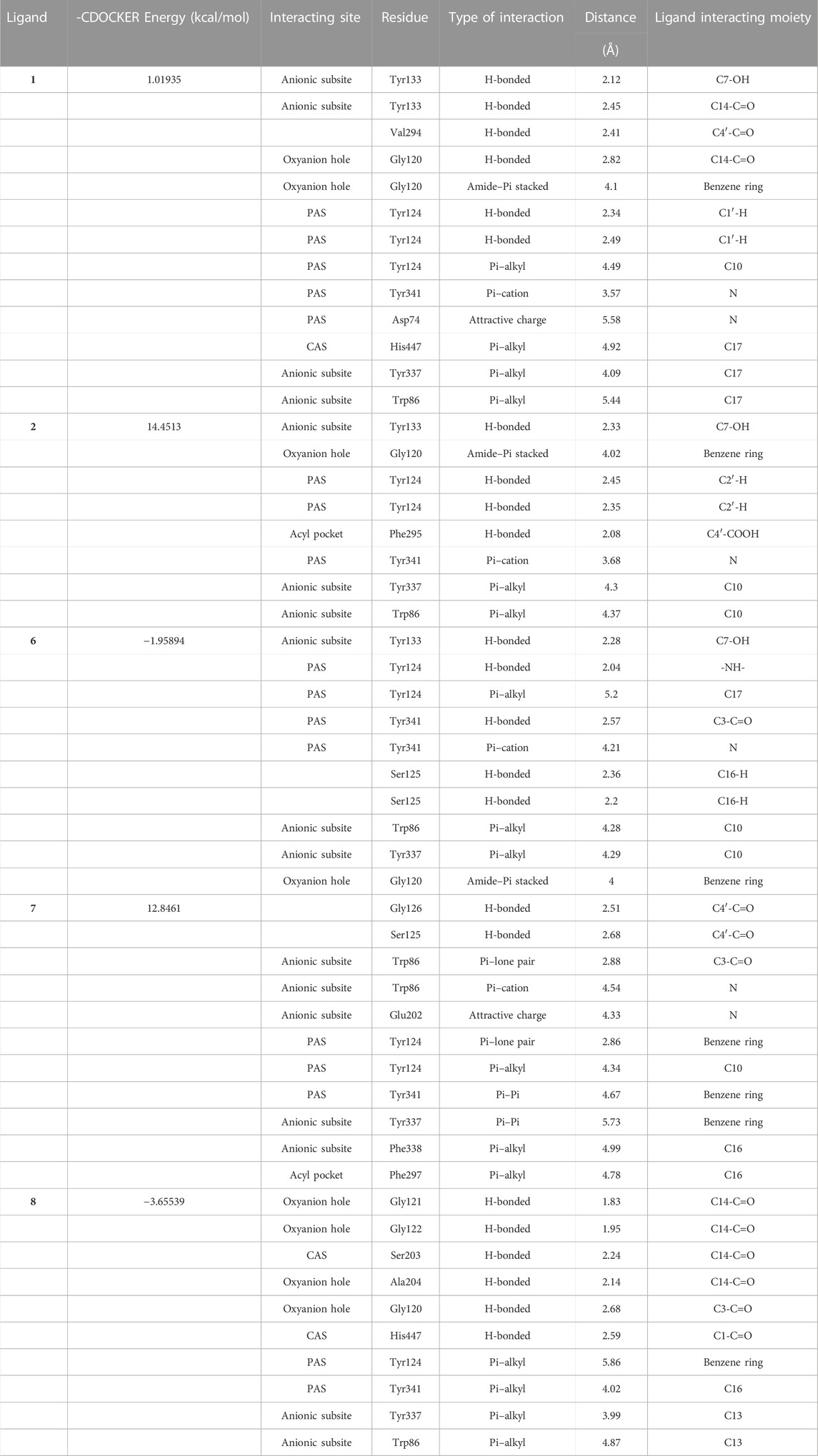
TABLE 4. Binding interaction data of compounds 1, 2, and 6–8 docked into the active site gorge of AChE.
Regarding AChE, the higher of ‘-CDOCKER Energy’ meant interactions between the five compounds and the 4EY6 were stronger than galantamine. Hydrogen bonding, attractive charge, amide–Pi stacked, Pi–Pi, Pi–alkyl, Pi–cation, Pi–anion, Pi–lone pair interactions were found to be involved between the compounds and the active site residues Tyr133, Tyr337, Trp86, Glu202, and Phe338 in the anionic subsite; Tyr124, Tyr341, and Asp74 in the peripheral active site (PAS); His447 and Ser203 in the catalytic active site (CAS); Gly120, Gly121, Gly122, and Ala204 in the oxyanion hole; and Phe297 and Phe295 in the acyl pocket (Namdaung et al., 2018; Saenkham et al., 2020). Compound 2 was found with the highest AChE (PDB: 4EY6) inhibition, which showed eight tight bindings to key residues (Table 4). The 3D and 2D molecular interaction of compound 2 in the binding pocket of AChE are clearly explained in Figure 3 and Figure 4. The results suggested that compound 2 formed four hydrogen bondings to key residues, namely, two hydrogen atoms of C2’ to Tyr124 (2.45 Å and 2.35 Å) at PAS, C4′-COOH to Phe295 (2.08 Å) at acyl pocket, C7-OH to Tyr133 (2.33 Å) at anionic subsite. Secondly, the benzene ring interacted with the Gly120 (4.02 Å) of the oxyanion hole to form amide–Pi stacked, and C10 interacted with Tyr337 (4.30 Å) and Tyr86 (4.37 Å) at anionic subsite to form Pi–alkyl interaction. In addition, the Pi–catinon interaction between the N atom and the Tyr341 (3.68 Å) located in PAS can also be observed. These interactions played a vital role for anti-AChE activity and may be useful to further explain the inhibition exhibited by compound 2.
3 Discussion
Usnea is a kind of lichen traditional Chinese medicine with high-medicinal value, distributed in polar and tropical regions, and widely distributed in Sichuan, Inner Mongolia, Yunnan, Heilongjiang, and other places in China. There are more than 10 common species, such as U. diffracta Vain, U. cavernosa Tuck, and U. longissima Ach, among which U. longissima Ach is a large species studied widely (Salgado et al., 2018; Ullah et al., 2019). The phytochemistry of the Usnea genus show that it contains more than 60 compounds, which mainly belong to depsides, depsidones, depsones, lactones, quinones, phenolics, polysaccharides, fatty acids, and dibenzofurans (Salgado et al., 2018). Among them, depsides, depsidones, depsones, and dibenzofurans are the unique phenolic components of lichens. These representative aromatic products are compounds derived from the acetyl-polymalonyl pathway, the most characteristic being formed by the bonding of two or three orcinol or 3-orcinol-type phenolic units through ester, ether, and carbon–carbon linkages (Shukla et al., 2010).
The purpose of this study was to determine the chemical constituents from the dry lichen body of Usnea diffracta Vain. Following the isolation procedure in this article, we isolated 30 compounds including 16 phenols from U. diffracta Vain, which specifically belonged to dibenzofurans, multi-substituted single benzene ring, depsides, organic acid, organic acid ester, fatty acids containing furan ring, furfural, amino acid, and nucleoside compound. After searching the SciFinder database, compounds 1–5 were identified as new compounds. Most of the compounds were multi-substituted single benzene ring, dibenzofurans, and depsides, all of which were recognized as the main secondary metabolites of Usnea.
Usnea lichen has a long history of antimicrobial use as a folk medicine. Modern scientific research reveals that its crude extracts and monomer compounds have good progress in antimicrobial activities. Derivatives of depsides and dibenzofurans are the main resources of its antimicrobial activities, especially the usnic acid is the most prominent which has a relatively wide antimicrobial spectrum. Several studies have confirmed that usnic acid can inhibit growth of different planctonic bacteria and fungi strains, such as Klebsiella pneumoniae, Bacillus subtilis, Bacillus cereus, Bacillus mycoides, Staphylococcus aureus, and Escherichia coli (Sultana and Afolayan, 2011; Helena et al., 2012; Rankovic et al., 2012). The antimicrobial activity of Usnea diffracta Vain ethyl acetate extracts against Actinomyces viscosus and Streptococcus mutans assays showed that ethyl acetate extracts were resistant to these strains (Qi et al., 2009). In this study, the disk diffusion method and the microdilution method were designed to screen the activities of the isolated compounds against Staphylococcus aureus, Escherichia coli, and Candida albicans. An anti-C. albicans assay was not performed due to the insufficient amount of compounds 4–5. The results exhibited that compound 3 had certain inhibitory activities against S. aureus and E. coli but no anti-C. albicans activity at 64 μg/ml, compound 9 had certain an inhibitory effect on S. aureus and C. albicans with the MIC of 64 μg/ml, and compound 10 demonstrated the strongest inhibition against S. aureus and C. albicans among all compounds. Among them, compounds 9 and 10 belong to depsides. Although five dibenzofurans (1, 2, and 6–8) have no antibacterial effect, they can inhibit the growth of C. albicans with the MIC of 64 μg/ml. This further confirmed that derivatives of depsides and dibenzofurans were the main resources of its antimicrobial activities. The result that (+)-usnic acid (8) studied in this study showed no activity against S. aureus and E. coli does not mean that it is inconsistent with previous studies, but can only demonstrate that there is no bacteriostatic effect at this concentration. As this antimicrobial activity assay is only conducted in a certain concentration range for primary activity screening, and cannot be concluded that other compounds have no effect, the follow-up needs to be more detailed and in-depth study of the antimicrobial activities of these compounds.
Since benzofuran has been reported to be a pharmacophore with cholinesterase inhibitory activity, and dibenzofurans, such as (+)-usnic acid, are similar to galantamine in structure, an anticholinesterase drug for AD. We designed experiments to observe the cholinesterase inhibitory activity of the isolated dibenzofurans 1, 2, and 6–8 and to study whether they can be potential cholinesterase inhibitors or drugs for the treatment of AD. The assay of AChE inhibitory activity was determined by the improved spectrophotometric Ellman’s method (Zhu et al., 2019; Saenkham et al., 2020). Results demonstrated that the five compounds also exhibited AChE inhibition at the concentration of 0.3 μmol/ml with values of 1.01935 kcal/mol, 14.4513 kcal/mol, −1.95894 kcal/mol, 12.8461 kcal/mol, and -3.65539 kcal/mol, respectively. To follow up our results and study the binding affinity related to the inhibition of acetylcholinesterase, molecular docking simulations were performed. The 3D crystal structure of recombinant human AChE complexed with galantamine (code ID: 4EY6) was obtained from the protein data bank (PDB) with a resolution of 2.4 Å and docked with the 3D structure of five compounds (Cheung et al., 2012). Docking analysis exhibited that the investigated compounds had a high affinity for the acetylcholinesterase’s active sites and higher ‘–CDOCKER Energy’ than the original ligand molecule ranged from -3.65539 kcal/mol ((+)-usnic acid) to 14.4513 kcal/mol (Usneamine H) for the target enzyme. Usneamine H exhibited the highest AChE inhibition, which was consistent with the assay of AChE inhibitory activity. It formed four hydrogen bonding, amide–Pi stacked, Pi–catinon interaction, two Pi–alkyl interactions to key residues at PAS, acyl pocket, anionic subsite, and oxyanion hole of AChE. These previous interactions assisted in the understanding of anti-AChE activity. However, considering the simplicity of our anti-acetylcholinesterase activity experiments, whether the isolated compounds can become potential cholinesterase inhibitors still needs further research, such as cell assays and in vivo assays.
4 Materials and methods
4.1 Plant collection
The dry lichens of Usnea diffracta Vain were collected from the Anguo traditional Chinese medicine market in Baoding (Hebei, China) in September 2018, and were identified by Professor Lijuan Zhang of Tianjin University of Traditional Chinese Medicine. The voucher specimen (UD-2018-C412-1) has been deposited at the College of Traditional Chinese Medicine, Tianjin University of Traditional Chinese Medicine.
4.2 Chemicals and reagents
Chromatographic grade methanol and acetonitrile were obtained from (Concord Technology (Tianjin) Co., Ltd.). Analytical grade petroleum ether, dichloromethane, ethyl acetate, methanol, trifluoroacetic acid, formic acid, and dimethyl sulfoxide were obtained from (Concord Technology (Tianjin) Co., Ltd.). 5,5-dithiobis [2-nitrobenzoic acid] (DTNB, CAS: 69-78-3), acetylthiocholine iodide (ATCI, CAS: 2260-50-6), and acetylcholinesterase (CAS: 9000-81-1) from Electrophorus electricus (Sigma Aldrich). Brain–Heart Infusion Agar and Broth were obtained from Qingdao Hope Bio-Technology Co., Ltd. Gentamicin sulfate (CAS: 1405-41-0) was obtained from Shanghai Aladdin Biochemical Technology Co., Ltd.
4.3 General
The CD spectrum was recorded by a J-1500 circular dichroic spectrometer (JASCO Co., Japan). NMR spectroscopy was performed on a Bruker AVANCE Ⅲ NMR spectrometer operating at 600 MHz for (1H), 150 MHz (13C) or Bruker AVANCE Ⅲ 500 NMR spectrometer (Bruker Co., Switzerland). The deuterated solvents used were DMSO-d6 and CD3OD and the chemical shifts were recorded in ppm. UV spectra were taken on a UV-6150S dual-beam UV-vis spectrophotometer (Shanghai MADAPA Instruments Co., Ltd.). The IR spectrum was detected using a Bruker ALPHA IR spectrophotometer (Bruker Co., Switzerland.). Specific optical rotations were measured using an AUTOPOLV polarimeter (Rudolph Co., United States). The relative molecular mass was measured on a Waters Xevo G2-SUPLC-Q/TOF spectrometer (Waters Co., United States). The samples were prepared by a LC-20AR system, LC-20AP system (Shimadzu, Kyoto, Japan), and Waters 2535 system (Waters Co., United States). Column chromatography included silica gel (200–300 and 300–400 mesh, Qingdao Marine Chemical Co., Qingdao, China), ODS (5–50 μm, YMC Co., Japan), YMC-pack-A (5 μm, 10 × 250 mm, YMC Co., Japan), Shim Pack PRC (ODS 15 μm, 20 × 250 mm, Japan), Sephadex LH-20 (Pharmacia, Sweden), and Macroreticular absorbing resin AB-8 (Tianjin Saizhiwei Technology Co., Tianjin, China). TLC was performed on TLC plates pre-coated with silica gel GF-254 (Qingdao Marine Chemical Co., Qingdao, China). The antimicrobial activities were measured by an HJH-C1112B clean bench (Shanghai Zhicheng Analytical Instrument Manufacturing Co., Shanghai, China), an HNYC-2102C incubation oscillator (Tianjin Honour Instrument Co., Tianjin, China), a YXQ-LS-75S11 vertical pressure steam sterilization pot (Shanghai Boxun Industry & commerce Co., Shanghai, China), a GEN10SUV-Vis ultraviolet spectrophotometer (Thermo Fisher Scientific Co., United States).
4.4 Extraction and isolation
The dry lichens of Usnea diffracta Vain (4.0 Kg; sampling: September 2018) were extracted with 95% v/v EtOH/H2O three times for 3 h each time and then 70% v/v EtOH/H2O three times for 3 h each time. The extracts were concentrated to afford the crude extract (1.7 kg). The crude extract was dissolved in 5 L pure water to make suspension, and then successively extracted with petroleum ether, dichloromethane, and ethyl acetate. The filtered combined solution of each solvent extraction was evaporated to yield the petroleum ether (101.2 g), CH2Cl2 (672.5 g), EtOAC (218.1 g), and post-extraction (650.3 g) extracts. The post-extraction extract (650.3 g) was dissolved in pure water and passed through AB-8 macroporous resin (3.5 kg) column, which was divided into insoluble precipitation layer (250.4 g), water layer (149.6 g), 30% ethanol layer (90.3 g), 60% ethanol layer (41.4 g), and 100% ethanol layer (73.5 g).
The petroleum ether extract (2.0 g) was fractionated by column chromatography (CC) using silica gel (300–400 mesh) as the adsorbent. Eluting with a gradient system of petroleum ether–ethyl acetate (100:1–14:1–7:1–3:1) to afford 12 main fractions (Fr1-12) based on TLC investigations. Fraction Fr2 (100:1) was precipitated and crystallized, washed with petroleum ether, and filtered to obtain compound 13 (100.0 mg) as a white acicular crystal. Fraction Fr10 (14:1) was precipitated and crystallized, washed with petroleum ether and dichloromethane, and filtered to obtain compound 14 (40.0 mg) as a white acicular crystal. Fraction Fr7 (25.5 mg, 14:1) was further purified on a Sephadex LH-20 column with dichloromethane-methanol (1:1) to obtain Fr (7-1)–(7-8). Fr7-4 precipitated and crystallized, washed with dichloromethane, and filtered to obtain compound 8 (9.8 mg) as a yellow acicular crystal. Fr7-7 was further purified on a Sephadex LH-20 column with dichloromethane-methanol (1:1) and filtered crystallization to obtain compound 15 (4.7 mg) as a white acicular crystal.
The CH2Cl2 extract (50.0 g) was fractionated by CC (200–300 mesh) eluting with a gradient system of petroleum ether–ethyl acetate (300:1-1:1) to afford 13 main fractions (Fr1–13), CH2Cl2-CH3OH (10:1-1:1) to afford Fr14. Fraction Fr13 (2.3 g, 1:1) was further purified on a Sephadex LH-20 column with dichloromethane-methanol (1:2) to obtain Fr (13-1)–(13-5). Fr13-4 (0.68 g) was subjected to the 5–50 μm ODS column chromatography and eluted with a gradient of methanol/water (5:95-100:0, v/v) to afford eight fractions Fr (13-4-1)–(13-4-8). The fraction Fr13-4-4 (213.0 mg) was precipitated and crystallized. The crystals (6.0 mg) were filtered and prepared by Waters C18 preparation column (10 mm × 150 mm) in Waters 2535 preparative high performance liquid chromatography (210 nm, 280 nm). Compound 27 (3.2 mg, tR 10 min) was obtained with acetonitrile/water (65:35, v/v, 2.0 mL/min) as eluent. The remaining fraction was also prepared by the same condition to afford compound 6 (48.2 mg, tR 77 min), compound 26 (10.3 mg, tR 71 min), and compound 7 (4.8 mg, tR 86 min) were obtained with methanol/water (68:32, v/v, 2.0 mL/min) as eluent. The remaining Fr13 were combined into Fr14 and Fr13 + 14 (10.8 g). This fraction was subjected to 5–50 μm ODS column chromatography (11.5 × 3 cm, 38 g) and eluted with a gradient of methanol/water (5:95-100:0, v/v) to afford nine fractions Fr ((13 + 14)-1)-Fr ((13 + 14)-9). Fraction Fr ((13 + 14)-4 (1.2 g) was prepared by Shim Pack PRC (201 nm, 283 nm) with a gradient system of methanol/water (70:30, v/v, 6.0 mL/min) as eluent to afford 12 fractions Fr ((13 + 14)-4)-1-Fr ((13 + 14)-4–12). Fr (13 + 14)-4-11 (tR 90–95 min, 50.7 mg) was further prepared by Waters C18 preparation column (10 mm × 150 mm) (201 nm, 283 nm) with a gradient system of acetonitrile/water (50:50, v/v, 2.0 mL/min) as eluent to yield compound 2 (tR 20 min, 25.3 mg) and compound 1 (tR 26 min, 10.0 mg).
The EtOAC extract (100.0 g) was fractionated by CC (200–300 mesh) eluting with a gradient system of petroleum ether–ethyl acetate (150:1-1:1) to afford 10 main fractions (Fr1-10). Fr2 (110:1, 470.2 mg) was subjected to the CC eluted with petroleum ether to afford Fr (2-1)-(2-4). Fr (2-3) (300.1 mg) was prepared by a YMC column (201 nm, 254 nm) with a gradient system of methanol/water (70%–100% 25 min, 100% 15 min, 100%–70% 10 min, 1.5 mL/min) as eluent to yield Fr (2-3-1)–(2-3–7). Compounds 16 (6.1 mg) and 23 (2.2 mg) were prepared by scraping thin layer silica gel with petroleum ether–ethyl acetate (25:1) from Fr2-3-7 (17.0 mg, tR 42 min). Fr3 (40:1, 45.0 mg) was successively purified on a CC eluting with a gradient system of petroleum ether–ethyl acetate (120:1) and a YMC column (201 nm, 254 nm) with methanol/water (70%–95% 25 min, 95%–100% 5 min, 100%–70% 10 min, 1.5 mL/min) as eluent to afford compound 9 (3.0 mg, tR 35 min). Fr4 (25:1) was successively purified on a CC eluting with a gradient system of petroleum ether–ethyl acetate (120:1-30:1) and a YMC column (201 nm, 254 nm) with methanol/water (70%–100% 25 min, 100% 15 min, 100%–70% 10 min, 1.5 mL/min) as eluent to afford compound 17 (8.0 mg, tR 30 min). Fr4-4 (70:1, 90.0 mg) was prepared by a YMC column under the same condition, then scraper was prepared by thin layer silica gel, and compounds 24 (3.5 mg) and 25 (4.8 mg) were obtained by the expansion of petroleum ether–ethyl acetate 8-1 with 0.01% formic acid. Fr6 (5:1, 430.0 mg) was successively purified on a CC eluting with a gradient system of petroleum ether–ethyl acetate (30:1, 0.01% formic acid) and a YMC column (201 nm, 254 nm) under the same condition to afford compounds 18 (2.8 mg, tR 13.5 min) and 10 (70.1 mg, tR 25.5 min). Fr8 (5:1, 7.0 g) was successively purified on a CC, ODS column, and a YMC column (201 nm, 254 nm) with methanol/water (30%–70% 30 min, 70% 15 min, 70%–90% 15 min, 90%–100% 10 min, 100% 10 min, 100%–30% 10 min, 1.5 mL/min) as eluent to afford Fr (8-1)-(8-8). Compound 22 (12.8 mg) was obtained from Fr8-3 (tR 29–39 min) by a YMC column with 40% elution of methanol/water. Fr8-6 (tR 51–52 min, 20.0 mg) was further prepared by a YMC column with a gradient system of acetonitrile/water (60:40, v/v) as eluent to yield compound 12 (16.8 mg).
The 60% ethanol layer (2.7 g) was fractionated by 5–50 μm ODS column chromatography and eluted with a gradient of methanol/water (5:95-100:0, v/v) to afford 14 fractions (Fr1-14). Fraction Fr4 (33.1 mg, 30:70) was further purified on a Sephadex LH-20 column with methanol to obtain Fr (4-1)-(4-4). Fr (4-2) was prepared by a YMC column (210 nm, 278 nm) with a gradient system of methanol/water (50:50, v/v, 1.5 mL/min) as eluent to yield four fractions Fr (4-2-1)-(4-2-4). Fr4-2-4 (tR 17-24 min, 10.9 mg) was further prepared by a YMC semi-preparative column (210 nm, 278 nm) with a gradient system of methanol/0.05% trifluoroacetic acid water (50:50, v/v, 1.5 mL/min) as eluent to yield Fr (4-2-4-1)-(4-2-4-4). Fr4-2-4-3 (tR 16–21 min, 7.5 mg) was further prepared by a YMC column (210 nm, 278 nm) with a gradient system of acetonitrile/0.07% trifluoroacetic acid water (25:75, v/v, 1.5 mL/min) as eluent to yield compound 5 (tR 17 min, 3.0 mg) and compound 4 (tR 18.5 min, 1.5 mg). Fr7 (49.1 mg, 50:50) was subjected to the Sephadex LH-20 column with methanol and CC (200–300 mesh) eluting with a gradient system of petroleum ether–ethyl acetate (15:1) to afford compound 19 (3.6 mg). Fr10 (162.1 mg, 70:30) was purified on a Sephadex LH-20 column with methanol to obtain Fr (10-1)-(10-7). Fr10-5 (13.8 mg) was fractionated by CC (200–300 mesh) eluting with a gradient system of petroleum ether–ethyl acetate (1:1) to afford compound 11 (6.7 mg). Fr10-4 (6.1 mg) was fractionated by CC (200–300 mesh) with petroleum ether–ethyl acetate (50:1) to yield compound 20 (1.7 mg). Fraction Fr14 was successively purified on a Sephadex LH-20 column with methanol and CC (200–300 mesh) eluting with a gradient system of petroleum ether–ethyl acetate (150:1) to afford compound 3 (14.5 mg).
The 30% ethanol layer (75.5 g) was fractionated by CC (200–300 mesh) eluting with a gradient system of dichloromethane-methanol (10:1-1:1) ∼ methanol ∼ methanol-water (1:1) to afford Fr1-6. Fr1 (10:1, 7.5 g) was further purified by CC with dichloromethane-methanol (80:1-8:1) to obtain Fr (1-1)-(1–5). Fr1-2 (80:1, 650.1 mg) was successively purified on a ODS column eluting with methanol/water (5:95-100:0), CC (petroleum ether-ethyl acetate (5:1-1:1) ∼ dichloromethane-methanol (10:1-1:1)) and a YMC column (201 nm, 281 nm) with methanol/water (40:60, 1.5 mL/min) as eluent to afford compound 21 (6.7 mg, tR 21 min). Fr1-3 (20:1) was subjected to a ODS column eluting with methanol/water (5:95-100:0) to obtain Fr (1-3-1)-(1-3-10). Fr1-3-1 (5:95, 1.58 g) was successively prepared by CC (petroleum ether–ethyl acetate (5:2-3:2) ∼ dichloromethane-methanol (1:1)) and a YMC column with methanol/water (53:47) as eluent to afford compound 28 (5.8 mg, tR 10 min). The remaining Fr1-3-1 were combined into Fr1-3-2 and Fr1-3-3 (1.4 g). This fraction was successively prepared by Shim Pack PRC (201 nm, 281 nm) with methanol/water (10:90, v/v, 7.0 mL/min) and a YMC column (201 nm, 281 nm) with methanol/water (5:95, 1.5 mL/min) as eluent to afford compounds 29 (3.0 mg, tR 20 min) and 30 (2.0 mg, tR 26 min).
The compounds were characterized by CD, UV, IR, HR-ESI-MS, 1H NMR, 13C NMR data, and 2D NMR.
4.5 In vitro antimicrobial activity
Referring to the disk diffusion method (Noumi et al., 2018), the inhibition zone of compounds against Staphylococcus aureus ATCC6358 and Escherichia coli BL21 were determined in order to preliminarily screen their antibacterial activities. RPMI1640 medium microdilution assay (M27-Ed4) (Clinical Laboratory Standard Institute, 2017) issued by the CLSI was performed to identify the minimal inhibitory concentration of compounds against Candida albicans ATCC90028. The antimicrobial effect was tested against two pathogenic bacteria including one Gram-positive strain (S. aureus) and one Gram-negative bacterial strain (E. coli) and fungus strain (C. albicans). The detailed protocol can be found in Supplemental Materials.
4.6 Anti-AChE assay
The assay of AChE inhibitory activity was determined by the improved spectrophotometric Ellman’s method (Zhu et al., 2019; Saenkham et al., 2020). In brief, 140 μL of 100 mM sodium phosphate buffer (pH 8.0), 20 μL of a solution of AChE (0.05 unit/mL), and 20 μL of test compound were mixed in 96-well plates, and then incubated at 30 °C for 15 min. The reaction was initiated by adding 20 μL of mixture solution of 10 μL 5,5′-dithio-bis-nitrobenzoic acid (DTNB) (10.0 mM) and 10 μL ATCl (7.5 mM), then catalyzed by enzymes at a wavelength of 412 nm and the absorbance was measured after 30 min of incubation at 37 °C. We replaced the test compound with 20 μL of phosphate buffer salt (100 mM) as a blank control. All reactions were performed in 96-well microplates in triplicate. Percentage of inhibition was calculated by the following equation:
Data were expressed as means ± SD and determined with SPSS 21.0 software.
4.7 Molecular docking
Molecular docking studies were generated by using the Discovery Studio 2019 Client program. The 3D crystal structure of recombinant human AChE complexed with galantamine (code ID: 4EY6) was obtained from the protein data bank (PDB) with a resolution of 2.4 Å (Cheung et al., 2012). The 4EY6 protein was optimized through the Prepare Protein function of DS software, and the active pocked was defined by the original ligand molecule. Before molecular docking, the original ligand galantamine was redocked to the AChE active pocket. Default settings were kept for parameters. The structural differences before and after molecular docking were compared. The results showed that the RMSD value was 0.5002 Å, indicating the rationality of the selected docking parameters and scoring function. Therefore, this parameter can be used for subsequent molecular docking research. The small molecules were introduced into DS software, and optimized by Prepare Ligands and Minimization. The CDOCKER module was used to simulate the molecular docking between small molecules and AChE. The docking results were analyzed using Discovery Studio Visualizer. Evaluation of the molecular docking was performed according to ‘–CDOCKEREnergy’ value (Yu et al., 2018).
5 Conclusion
In this study, we exhibited how to isolate secondary metabolites from the dry lichen body of Usnea diffracta Vain and confirmed their structures by physicochemical properties and various spectral methods. We isolated 30 compounds which belonged to dibenzofurans, multi-substituted benzenes, depsides, organic acids, fatty acids, amino acid, furfural, and nucleoside. It contains five new compounds (1–5). The results of preliminary screening experiments on antibacterial and antifungal activities revealed that the new compound 3 had certain inhibitory activities against S. aureus and E. coli with IZ of 6.2 mm and 6.3 mm, depsides 9 and 10 had certain inhibitory effect on S. aureus and C. albicans. Compound 10 demonstrated the strongest inhibition against S. aureus and C. albicans with 6.6 mm and 32 μg/ml, respectively. Five isolated dibenzofurans (1, 2, and 6–8) were evaluated for their biological properties toward specific target human AChE involved in AD. This study clearly demonstrated that they could effectively inhibit AChE at 0.3 μmol/ml. The most anti-AChE activity was demonstrated by the lichen secondary metabolite Usneamine H (2), which was identified as a new compound. Furthermore, the result of molecular docking was consistent with activity experimental data. This compound exhibited the strongest binding affinity and formed several tight bindings to key residues located in the peripheral active site, acyl pocket, anionic subsite, and the oxyanion hole of AChE. In addition, whether these compounds can be potential antimicrobial agents or drug candidate for the treatment of AD needs further study.
Data availability statement
The original contributions presented in the study are included in the article/Supplemental Material; further inquiries can be directed to the corresponding author.
Author contributions
Conceptualization: L-NW; methodology: L-NW, Y-MH, B-QL, and C-SW; validation: L-NW, Y-MH, B-QL, and C-SW; data curation: Y-MH and B-QL; writing—original draft preparation: Y-MH; writing—review and editing: L-NW, Y-MH, Y-CY, and QZ; supervision: L-NW; project administration: L-NW; and funding acquisition: L-NW. All authors read and agreed to the published version of the manuscript.
Funding
This research was funded by the Tianjin Research Program of Application Foundation and Advanced Technology, China (No.18JCYBJ28900), and National Key R&D Program of China (2019YFC1711000).
Conflict of interest
The authors declare that the research was conducted in the absence of any commercial or financial relationships that could be construed as a potential conflict of interest.
The reviewer JZ declared a shared affiliation with the author C-SW to the handling editor at the time of review.
Publisher’s note
All claims expressed in this article are solely those of the authors and do not necessarily represent those of their affiliated organizations, or those of the publisher, the editors, and the reviewers. Any product that may be evaluated in this article, or claim that may be made by its manufacturer, is not guaranteed or endorsed by the publisher.
Supplementary material
The Supplementary Material for this article can be found online at: https://www.frontiersin.org/articles/10.3389/fchem.2022.1063645/full#supplementary-material
References
Araújo, A. A., Melo, M. G., Rabelo, T. K., Nunes, P. S., Santos, S. L., Serafini, M. R., et al. (2015). Review of the biological properties and toxicity of usnic acid. Nat. Prod. Res. 29 (23), 2167–2180. doi:10.1080/14786419.2015.1007455
Behera, B. C., Mahadik, N., and Morey, M. (2012). Antioxidative and cardiovascular protective activities of metabolite usnic acid and psoromic acid produced by lichen species Usnea complanata under submerged fermentation. Pharm. Biol. 50, 968–979. doi:10.3109/13880209.2012.654396
Bruno, M., Trucchi, B., Burlando, B., Ranzato, E., Martinotti, S., Akkol, E. R., et al. (2013). (+)-Usnic acid enamines with remarkable cicatrizing properties. Bioorgan Med. Chem. 21 (7), 1834–1843. doi:10.1016/j.bmc.2013.01.045
Bui, V. M., Duong, T. H., Nguyen, T. A. M., Nguyen, T. N. V., Nguyen, N. H., Nguyen, H. H., et al. (2020). Two new phenolic compounds from the Vietnamese lichen Parmotrema tinctorum. Nat. Prod. Res. 36 (13), 3429–3434. doi:10.1080/14786419.2020.1864367
Cazarin, C. A., Dalmagro, A. P., Gonçalves, A. E., Boeing, T., Silva, L. M., Corrêa, R., et al. (2020). Usnic acid enantiomers restore cognitive deficits and neurochemical alterations induced by Aβ 1–42 in mice. Behav. Brain Res. 397, 112945. doi:10.1016/j.bbr.2020.112945
Ceker, S., Orhan, F., Kizil, H. E., Alpsoy, L., Gulluce, M., Aslan, A., et al. (2015). Genotoxic and antigenotoxic potentials of two Usnea species. Toxicol. Ind. Health. 31, 990–999. doi:10.1177/0748233713485889
Chester, D. O., and Elix, J. A. (1979). Three new aliphatic acids from lichens of genus parmelia (subgenus xanthoparmelia). Aust. J. Chem. 32 (11), 2565–2569. doi:10.1071/ch9792565
Cheung, J., Rudolph, M. J., Burshteyn, F., Cassidy, M. S., Cary, E. N., Love, J., et al. (2012). Structures of human acetylcholinesterase in complex with pharmacologically important ligands. J. Med. Chem. 55, 10282–10286. doi:10.1021/jm300871x
Clinical Laboratory Standard Institute (2017). Reference method for broth dilution antifungal susceptibility testing of yeasts: Aproved standard. 4th ed. Wayne, PA: American Clinical Laboratory Standard Institute (CLSI). M27-E4.
Colovic Mirjana, B., Krstic Danijela, Z., Lazarević-Pašti Tamara, D., Bondžić Aleksandra, M., and Vasić Vesna, M. (2013). Acetylcholinesterase inhibitors: Pharmacology and toxicology. Curr. Neuropharmacol. 11 (3), 315–335. doi:10.2174/1570159x11311030006
Delogu, G. L., Matos, M. J., Fanti, M., Era, B., Medda, R., Pieroni, E., et al. (2016). 2-Phenylbenzofuran derivatives as butyrylcholinesterase inhibitors: synthesis, biological activity and molecular modeling. Bioorg. Med. Chem. Lett. 26, 2308–2313. doi:10.1016/j.bmcl.2016.03.039
Eliwa, E., Abdel-Razek, A., Frese, M., Halawa, A., El-Agrody, A., Bedair, A., et al. (2019). New naturally occurring phenolic derivatives from marine Nocardiopsis sp. AS23C: Structural elucidation and in silico computational studies. Vietnam J. Chem. 57, 164–174. doi:10.1002/vjch.201900010
Feng, B.-M., Liu, J.-Y., Wang, H.-G., Shi, L.-Y., Tang, L., and Wang, Y.-Q. (2011). Isolation and identification of chemical constituents of root of Girardinia suborbiculata. C.J.Chen subsp.triloba. J. Shenyang Pharm. Univ. 28 (05), 364–367. doi:10.14066/j.cnki.cn21-1349/r.2011.05.004
Feng, J., Yang, X.-W., Su, S.-D., and He, C. (2009). Chemical constituents from Usnea longgisima. China J. Chin. Mater. Med. 34 (06), 708–711.
Furukawa-Hibi, Y., Alkam, T., Nitta, A., Matsuyamaet, A., Mizoguchi, H., Suzuki, K., et al. (2011). Butyrylcholinesterase inhibitors ameliorate cognitive dysfunction induced by amyloid-β peptide in mice. Behav. Brain Res. 225, 222–229. doi:10.1016/j.bbr.2011.07.035
Graham, D. W., Ashton, W. T., Barash, L., Brown, J. E., Brown, R. D., Canning, L. F., et al. (1987). Inhibition of the mammalian β-lactamase renal dipeptidase (dehydropeptidase-I) by Z-2-(acylamino)-3-substituted-propenoic acids. J. Med. Chem. 30 (6), 1074–1090. doi:10.1021/jm00389a018
Greig, N. H., Utsuki, T., Ingram, D. K., Wang, Y., Pepeu, G., Scali, C., et al. (2005). Selective butyrylcholinesterase inhibition elevates brain acetylcholine, augments learning and lowers Alzheimer β-amyloid peptide in rodent. Proc. Natl. Acad. Sci. U. S. A. 102 (47), 17213–17218. doi:10.1073/pnas.0508575102
Gu, X.-L., Jin, Y., Zhang, L.-Y., Fan, J.-T., and Du, S.-H. (2017). Study on the chemical constituents from the n-Butanol extract of Typhonii Rhizoma. Res. Pract. Chin. Med. 31 (04), 30–32. doi:10.13728/j.1673-6427.2017.04.009
Helena, P. R., Rodrigo, L., and Soares, M.-G. M. J. (2012). Effect of usnic acid on Candida orthopsilosis and C. parapsilosis. Antimicrob. agents Chemother. 56 (1), 595–597. doi:10.1128/AAC.05348-11
Huneck, S., Schmidt, J., and Tabacchi, R. (1989). Thermal decomposition of lichen depsides. Z. Naturforsch B 44, 1283–1289. doi:10.1515/znb-1989-1023
Khanam, H., and Shamsuzzaman, (2015). Bioactive benzofuran derivatives: a review. Eur. J. Med. Chem. 97, 483–504. doi:10.1016/j.ejmech.2014.11.039
Koparal, A. T. (2015). Anti-angiogenic and antiproliferative properties of the lichen substances (-) usnic acid and vulpinic acid. Z. Naturforsch. C 70, 159–164. doi:10.1515/znc-2014-4178
Lai, D., Odimegwu, D. C., Esimone, C., Thomas, G., and Peter, P. (2013). Phenolic compounds with in vitro activity against respiratory syncytial virus from the Nigerian lichen Ramalina farinacea. Planta Med. 79, 1440–1446. doi:10.1055/s-0033-1350711
Laxi, N., Tang, Y.-X., Bao, H.-Y., and Tuli, G. (2013). Chemical constituents from Usnea longgisima, a traditional Mongolian medicine. China J. Chin. Mater. Med. 38 (13), 2125–2128.
Li, G.-H., Li, L., Duan, M., and Zhang, K.-Q. (2006). The chemical constituents of the fungus stereum sp. Chem. Biodivers. 3 (2), 210–216. doi:10.1002/cbdv.200690024
Li, J., Yin, B., Liu, Y., Wang, L.-Q., and Chen, Y.-G. (2009). Mono-aromatic constituents of Dendrobium longicornu. Chem. Nat. Compd. 45 (2), 234–236. doi:10.1007/s10600-009-9293-2
Nahrstedt, A., Economou, D., and Wray, V. (1990). 2-Methylbutan-l-yl-β/-D-glucoside, a hemiterpene glucoside from bystropogon plumosus. J. Nat. Prod. 53 (5), 1387–1389. doi:10.1021/np50071a045
Namdaung, U., Athipornchai, A., Khammee, T., Kuno, M., and Suksamrarn, S. (2018). 2-Arylbenzofurans from Artocarpus lakoocha and methyl ether analogs with potent cholinesterase inhibitory activity. Eur. J. Med. Chem. 143, 1301–1311. doi:10.1016/j.ejmech.2017.10.019
Nishanth, K. S., Sreerag, R. S., Deepa, I., Mohandas, C., and Nambisan, B. (2015). Protocetraric acid: an excellent broad spectrum compound from the lichen Usnea albopunctata against medically important microbes. Nat. Prod. Res. 29, 574–577. doi:10.1080/14786419.2014.953500
Nishitoba, Y., Nishimura, I., Nishiyama, T., and Mizutani, J. (1987). Lichen acids, plant growth inhibitors from Usnea longissima. Phytochemistry 6 (12), 3181–3185. doi:10.1016/S0031-9422(00)82466-8
Noumi, E., Merghni, A., Alreshidi, M. M., Haddad, O., Akmadar, G., Martino, M. D., et al. (2018). Chromobacterium violaceum and Pseudomonas aeruginosa PAO1: Models for evaluating anti-quorum sensing activity of Melaleuca alternifolia essential oil and its main component terpinen-4-ol. Molecules 23, 2672. doi:10.3390/molecules23102672
Popovici, V., Bucur, L., Gîrd, C. E., Rambu, D., Calcan, S. I., Cucolea, E. I., et al. (2022). Antioxidant, cytotoxic, and rheological properties of canola oil extract of Usnea barbata (L.) weber ex F.H. Wigg from călimani mountains, Romania. Plants 11, 854. doi:10.3390/PLANTS11070854
Qi, H. Y., Jin, Y. P., and Shi, Y. P. (2009). ChemInform abstract: A new depsidone from usnea diffracta. Chin. Chem. Lett. 20 (2), 187–189. doi:10.1002/chin.200924204
Qin, C.-P., Wang, X.-R., Tian, Y.-Q., and Wang, L. (2010). Study on the secondary metabolites from Mariannaea campospora SIIA-F12361. Chin. J. Antibiot. 35 (11), 877–880. doi:10.13461/j.cnki.cja.004692
Rankovic, B., Kosanic, M., Stanojkovic, T., Vasiljević, P., and Manojlović, N. (2012). Biological activities of Toninia candida and Usnea barbata together with their norstictic acid and usnic acid constituents. J. Mol. Sci. 13 (2), 14707–14722. doi:10.3390/ijms131114707
Reddy, S. D., Siva, B., Kumar, K., Babu, V. S. P., Sravanthi, V., Boustie, J., et al. (2019). Comprehensive analysis of secondary metabolites in Usnea longissima (lichenized Ascomycetes, parmeliaceae) using UPLC-ESI-QTOF-MS/MS and pro-apoptotic activity of barbatic acid. Molecules 24 (12), 2270. doi:10.3390/molecules24122270
Rezanka, T., and Guschina, I. A. (2001). Glycoside esters from lichens of central Asia. Phytochemistry 58 (3), 509–516. doi:10.1016/S0031-9422(01)00261-8
Rizzo, S., Tarozzi, A., Bartolini, M., Costa, G. D., Bisi, A., Gobbi, S., et al. (2012). 2-arylbenzofuran-based molecules as multipotent Alzheimer's disease modifying agents. Eur. J. Med. Chem. 58, 519–532. doi:10.1016/j.ejmech.2012.10.045
Saenkham, A., Jaratrungtawee, A., Siriwattanasathien, Y., Boonsri, P., Chainok, K., Suksamrarn, A., et al. (2020). Highly potent cholinesterase inhibition of geranylated xanthones from Garcinia fusca and molecular docking studies. Fitoterapia 146, 104637. doi:10.1016/j.fitote.2020.104637
Salgado, F., Albornoz, L., Cortez, C., Stashenko, E., Urrea-Vallejo, K., Nagles, E., et al. (2018). Secondary metabolite profiling of species of the genus Usnea by UHPLC-ESI-OT-MS-MS. Molecules 23 (1), 54–69. doi:10.3390/molecules23010054
Shukla, V., Joshi, G. P., and Rawat, M. (2010). Lichens as a potential natural source of bioactive compounds: a review. Phytochem. Rev. 9 (2), 303–314. doi:10.1007/s11101-010-9189-6
Singh, B., Gangwar, P., Paliya, B., Bajpai, R., Singh, B. R., Kumar, J., et al. (2016). The genus usnea: A potent phytomedicine with multifarious ethnobotany, phytochemistry and pharmacology. Rsc Adv. 6 (26), 21672–21696. doi:10.1039/c5ra24205c
Singh, M., Kaur, M., Kukreja, H., Chugh, R., Silakari, O., and Singh, D. (2013). Acetylcholinesterase inhibitors as alzheimer therapy: from nerve toxins to neuroprotection. Eur. J. Med. Chem. 70, 165–188. doi:10.1016/j.ejmech.2013.09.050
Singh, S., Khatoon, S., Joshi, Y., Prgyadeep, S., Upreti, D. K., and Singh Rawat, A. K. (2016). A validated HPTLC densitometric method for simultaneous determination of evernic and usnic acids in four usnea species and comparison of their antioxidant potential. J. Chromatogr. Sci. 54, 1670–1677. doi:10.1093/chromsci/bmw118
Su, H., Zhu, X.-B., Yuan, Z.-H., Li, J., Guo, S.-J., Han, L.-J., et al. (2009). Studies on chemical constituents of. Chondrophycus Papillous. Mar. Sci. 33 (05), 33–35.
Sultana, N., and Afolayan, A. J. (2011). A new depsidone and antibacterial activities of compounds from Usnea undulata Stirton. J. Asian Nat. Prod. Res. 13 (12), 1158–1164. doi:10.1080/10286020.2011.622720
Sun, C., Liu, F., Sun, J., Li, J., and Wang, X. (2016). Optimisation and establishment of separation conditions of organic acids from Usnea longissima Ach. by pH-zone-refifining counter-current chromatography: Discussion of the eluotropic sequence. J. Chromatogr. A 1427, 96–101. doi:10.1016/j.chroma.2015.12.016
Tang, Y.-X., Laxi, N., and Bao, H.-Y. (2015). Chemical constituents and their antioxidant activities from thallus of Ramalina conduplicans. Mycosystema 34 (01), 169–176. doi:10.13346/j.mycosystema.130130
Tuan, N. T., Dam, N. P., Van Hieu, M., Trang, D. T. X., Danh, L. T., Men, T. T., et al. (2020). Chemical constituents of the lichen parmotrema tinctorum and their antifungal activity. Chem. Nat. Compd. 56 (2), 315–317. doi:10.1007/s10600-020-03017-y
Ullah, M., Uddin, Z., Song, Y. H., Li, Z. P., Kim, J. Y., Ban, Y. J., et al. (2019). Bacterial neuraminidase inhibition by phenolic compounds from Usnea longissima. S Afric J. Bot. 120, 326–330. doi:10.1016/j.sajb.2018.10.020
Vanga, N. R., Kota, A., Sistla, R., and Uppuluri, M. (2017). Synthesis and anti-inflammatory activity of novel triazole hybrids of (+)-usnic acid, the major dibenzofuran metabolite of the lichen Usnea longissima. Mol. Divers. 21, 273–282. doi:10.1007/s11030-016-9716-5
Wahl, H., Hong, Q., Stube, D., Maier, M. E., Häring, H. U., and Liebich, H. M. (2001). Simultaneous analysis of the di(2-ethylhexyl)phthalate metabolites 2-ethylhexanoic acid, 2-ethyl-3-hydroxyhexanoic acid and 2-ethyl-3-oxohexanoic acid in urine by gas chromatography–mass spectrometry. J. Chromatogr. B 758 (2), 213–219. doi:10.1016/s0378-4347(01)00183-9
Wang, L.-D., Wu, S.-H., Chen, Y.-W., Yu, Y., Yang, L.-Y., Li, S.-L., et al. (2009). Studies on the secondary metabolites of endophytic fungus Epicoccum isolated from Azadirachta indica. Nat. Prod. Res. Dev. 21 (06), 916–918. doi:10.16333/j.1001-6880.2009.06.004
Wang, Y.-N., Liu, M.-F., Hou, W.-Z., Xu, R.-M., Gao, J., Lu, A.-Q., et al. (2017). Bioactive benzofuran derivatives from Cortex Mori Radicis, and their neuroprotective and analgesic activities mediated by mGluR1. Molecules 22, 236–248. doi:10.3390/molecules22020236
Wu, J., Tian, Y., Wang, S., Pistolozzi, M., Jin, Y., Zhou, T., et al. (2016). Design, synthesis and biological evaluation of bambuterol analogues as novel inhibitors of butyrylcholinesterase. Eur. J. Med. Chem. 126, 61–71. doi:10.1016/j.ejmech.2016.08.061
Yang, Y., Nguyen, T. T., Jeong, M. H., Crişan, F., Yu, H., Ha, H. H., et al. (2017). Inhibitory activity of (+)-usnic acid against nonsmall cell lung cancer cell motility. PLoS One 11, e0146575. doi:10.1371/journal.pone.0146575
Yu, X.-L., Yang, X.-Y., Gao, X. -L., Bai, R.-F., Yin, X., Su, G.-Z., et al. (2016). Phenolic constituents from Usnea longgisima. China J. Chin. Mater, Med. 41 (10), 1864–1869. doi:10.4268/cjcmm20161017
Yu, X., Guo, Q., Su, G., Yang, A., Hu, Z., Qu, C., et al. (2016). Usnic acid derivatives with cytotoxic and antifungal activities from the lichen Usnea longissima. J. Nat. Prod. 79 (5), 1373–1380. doi:10.1021/acs.jnatprod.6b00109
Yu, Z.-P., Wu, S.-J., Zhao, W.-Z., Ding, L., Fan, Y., Shiuan, D., et al. (2018). Anti-Alzheimers activity and molecular mechanism of albumin-derived peptides against AChE and BChE. Food Funct. 9 (2), 1173–1178. doi:10.1039/c7fo01462g
Zhao, C., Yao, Z., Yang, D., Ke, J., Wu, Q., Li, J., et al. (2020). Chemical constituents from Fraxinus hupehensis and their antifungal and herbicidal activities. Biomolecules 10 (1), 74–83. doi:10.3390/biom10010074
Zhao, X.-Q., Zhu, J.-L., Wang, L.-W., Li, Y.-B., Zhao, T.-T., Chen, X., et al. (2019). U. diffracta extract mitigates high fat diet and VD3-induced atherosclerosis and biochemical changes in the serum liver and aorta of rats. Biomed. Pharmacother. 120, 109446. doi:10.1016/j.biopha.2019.109446
Zheng, Y.-B., Lu, C.-H., Xu, L., Zheng, Z.-H., Su, W.-J., and Shen, Y.-M. (2012). Chemical components from surface liquid fermented Hericium erinaceus with cytotoxity investigations. Nat. Prod. Res. Dev. 24 (12), 1703–1706. doi:10.16333/j.1001-6880.2012.12.002
Zhu, J., Wang, L.-N., Cai, R., Geng, S.-Q., Dong, Y.-F., and Liu, Y.-M. (2019). Design, synthesis, evaluation and molecular modeling study of 4-N-phenylaminoquinolines for Alzheimer disease treatment. Bioorg. Med. Chem. Lett. 29 (11), 1325–1329. doi:10.1016/j.bmcl.2019.03.050
Keywords: Usnea diffracta Vain, phenols, dibenzofurans, usnic acid, antimicrobial activities, acetylcholinesterase inhibition
Citation: Hao Y-M, Yan Y-C, Zhang Q, Liu B-Q, Wu C-S and Wang L-N (2023) Phytochemical composition, antimicrobial activities, and cholinesterase inhibitory properties of the lichen Usnea diffracta Vain. Front. Chem. 10:1063645. doi: 10.3389/fchem.2022.1063645
Received: 07 October 2022; Accepted: 19 December 2022;
Published: 06 January 2023.
Edited by:
Shao-Hua Wang, Lanzhou University, ChinaReviewed by:
Muhammad Saeed Jan, University of Malakand, PakistanJiaozhen Zhang, Shandong University, China
Copyright © 2023 Hao, Yan, Zhang, Liu, Wu and Wang. This is an open-access article distributed under the terms of the Creative Commons Attribution License (CC BY). The use, distribution or reproduction in other forums is permitted, provided the original author(s) and the copyright owner(s) are credited and that the original publication in this journal is cited, in accordance with accepted academic practice. No use, distribution or reproduction is permitted which does not comply with these terms.
*Correspondence: Li-Ning Wang, liningwang@tjutcm.edu.cn