- 1Department of Nosocomial Infection Management, Nantong Third People’s Hospital Affiliated to Nantong University, Nantong, Jiangsu, China
- 2Key Laboratory of Neuroregeneration, Ministry of Education and Jiangsu Province, Co-innovation Center of Tissue Engineering and Nerve Injury Repair, Nantong University, Nantong, China
- 3School of Chemistry and Chemical Engineering, Queen’s University Belfast, Belfast, United Kingdom
Gliomas are among the most common malignant tumors in the central nervous system and lead to poor life expectancy. However, the effective treatment of gliomas remains a considerable challenge. The recent development of near infrared (NIR) II (1000–1700 nm) theranostic agents has led to powerful strategies in diagnosis, targeted delivery of drugs, and accurate therapy. Because of the high capacity of NIR-II light in deep tissue penetration, improved spatiotemporal resolution can be achieved to facilitate the in vivo detection of gliomas via fluorescence imaging, and high contrast fluorescence imaging guided surgery can be realized. In addition to the precise imaging of tumors, drug delivery nano-platforms with NIR-II agents also allow the delivery process to be monitored in real-time. In addition, the combination of targeted drug delivery, photodynamic therapy, and photothermal therapy in the NIR region significantly improves the therapeutic effect against gliomas. Thus, this mini-review summarizes the recent developments in NIR-II fluorescence-based theranostic agents for glioma treatment.
Introduction
Gliomas account for 27% of intracranial primary tumors and originate from glial precursor cells (Blümcke et al., 2001; Ostrom et al., 2020; Balana et al., 2022). With high morbidity and mortality rates, gliomas are one of the most common malignant tumors in the central nervous system (Wen and Kesari, 2008; Razpotnik et al., 2017). Currently, gliomas are classified into subtypes, such as glioblastomas, anaplastic gliomas, and oligodendrogliomas. Glioblastomas account for 57.7% of all gliomas (Sailor and Park, 2012; Wesseling and Capper, 2018; d’Angelo et al., 2019). Depending on the location of the primary tumor and degree of malignancy, the treatment of gliomas remains challenging and prognosis is poor. The 5-year survival rate of patients affected with glioblastoma is less than 5% (Khaddour et al., 2020). Like many other malignant cancers, the most common treatment strategy for brain tumor patients is still surgery. Computed tomography (CT), magnetic resonance imaging (MRI) and positron emission tomography (PET) are the most common imaging modalities for surgery preparations (Dasgupta and Haas-Kogan, 2013; McNamara et al., 2014; Silantyev et al., 2019). However, due to their disadvantages such as unfriendly to pregnant women, children, patients with metal implants and patients with claustrophobia, novel imaging strategies especially real time ones need to be developed to improve the complete resection of gliomas. Most brain tumors, especially glioblastoma (GBM), can infiltrate into normal brain tissues, making it almost impossible to distinguish the tumor margins during surgery. In this instance, other precisely tumor-targeting clinical therapeutic methods such as irradiation and chemotherapy usually would be applied combined with surgery to improve the therapeutic efficacy. Phototherapy can also be a complementary to the current clinical treatment for gliomas. However, most of phototherapy is focused on tackling skin-related diseases because of the tissue penetration problem of excitation light, in which near infrared (NIR) fluorescence can help since it has deep tissue penetration. The tight junctions (TJs) of endothelial cells between the central nervous system (CNS) and peripheral blood circulation form the blood brain barrier (BBB) which presents an obstacle to various macromolecule/nanoparticles as contrast agents or therapeutic drugs into the brain. Many novel NIR fluorescent systems have been designed to across BBB and cure glioma-related problems.
Fluorescence imaging offers an opportunity for the precise detection of primary tumors or metastasis (Guilbault, 2020; Ling et al., 2021). Methods, such as photoinduced electron transfer (Daly et al., 2015) and intramolecular charge transfer (Misra and Bhattacharyya, 2018) involve the fabrication of stimuli-responsive fluorescent moieties that can accomplish functions, such as sensing (Schäferling, 2012). Recent developments of fluorescent applications for use in the life sciences have revealed the power of fluorescence for tracking and analyzing biological molecules (Liu H. W. et al., 2018; Huang Z. et al., 2021; Wu et al., 2022), labelling and delivering drugs (Guo et al., 2018; Patsenker and Gellerman, 2020), photodynamic therapy (PDT) (Li L. et al., 2019; Cai and Liu, 2020; Tang et al., 2021), and photothermal therapy (PTT) (Bian et al., 2021). In the case of gliomas, fluorescence has garnered considerable attention for its applicability in tumor diagnosis and therapy. Several fluorophores have been applied to gliomas for imaging, drug delivery, and therapy (Ji et al., 2018; Mishchenko et al., 2020; Ghirardello et al., 2022; Xiang et al., 2022). However, the emissive range of these fluorophores is within the visible light spectrum (380–700 nm), which leads to poor tissue penetration owing to light absorption and scattering and autofluorescence. Considering this, novel molecules and materials that emit light in the near infrared (NIR) range (650–1700 nm), especially within NIR-II (1000–1700 nm), are required to realize in vivo fluorescence imaging, drug delivery, and efficient PDT and PTT therapies (Figure 1). Thus, this review focuses on recent research in NIR-II fluorescence for glioma treatment concerning three areas: imaging, drug delivery, and therapy.
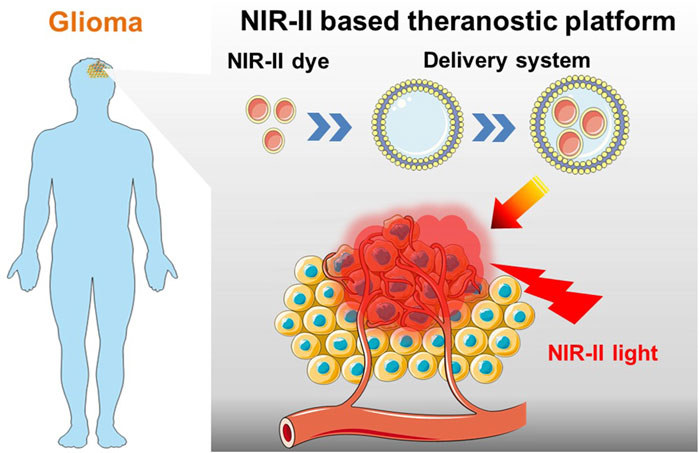
FIGURE 1. Schematic of NIR-II probes equipped with targeted drug delivery nano-platforms for precise diagnosis and effective phototherapy of glioma.
NIR-II fluorescence imaging
The NIR-II region (1000–1700 nm) has better spatiotemporal resolution and deeper tissue penetration than the NIR-I region (700–950 nm) and has attracted considerable interest from several research groups recently (Lu et al., 2020; Zhang et al., 2021). With the help of nanotechnology, through-skull fluorescence imaging in the NIR-IIb region (1500–1700 nm) has the potential to facilitate highly precise imaging-guided tumor surgery (Wang et al., 2022). The development of NIR-II fluorescent agents also significantly broadened the application scope for the fluorescence imaging of gliomas.
Based on their previous research (Liu et al., 2019), Li et al. investigated Er-based lanthanide down-conversion nanoparticles (DCNPs), which produce intense emissions in the NIR-IIb range (1500–1700 nm) and can be used for in vivo through-skull glioma imaging (Ren et al., 2020). A 0.9 nm NaYbF4 interlayer of this core-shell-shell NaErF4:Ce@NaYbF4(0.9 nm)@NaLuF4 material reflects the radiative energy of Er3+ and sequentially drives cross-relaxation via Ce3+ to maximize the transition to produce 1525 nm emissions. To gain biocompatibility, a novel dye-brush polymer was designed and synthesized to encapsulate the DCNPs, resulting in an average hydrodynamic size of 39.9 ± 2.8 nm. The dye-brush polymer absorbed at the same wavelength as the DCNPs (808 nm), which acted as energy harvesters, benefiting the energy cascaded down-conversion process to afford enhanced NIR-IIb fluorescence. The angiopep-2 peptide was chosen as the receptor for this system in combination with the dye-brush polymer-coated DCNPs because it could bind with overexpressed low-density lipoprotein receptor-related protein (LRP) on glioma cells. Because of the relatively large size of this nanoprobe, focused ultrasound sonication was used to control the opening of the blood brain barrier (BBB) to deliver the nanoprobe. Experiments in mice revealed that this DCNP-based nanoprobe was capable of performing the through-skull fluorescence imaging of gliomas. This method is comparable with T2-weighted MRI and can probably be used for intraoperative visualization. To improve the BBB permeability, Liu et al. coated a brain tumor cell membrane with Er-based lanthanide doped nanoparticles to form an NIR-IIb fluorescence imaging agent that targets glioma (Wang et al., 2022b). This nanoparticle, NaYbF4:Gd,Er,Ce@NaYF4, has a diameter of approximately 50 nm and is capable of coating human glioblastoma cell membrane vesicles after modification with phosphatidylcholine to increase the water dispersibility. Animal experiments revealed that this system has good immune escape, BBB crossing abilities, and homologous targeting abilities. Angiopep-2 is another glioma-targeting peptide that has a high binding affinity for low-density lipoprotein receptor-related protein-1. Conjugation with NIR-II fluorescent Ag2S quantum dots allows for the in vivo visualization of gliomas (Xu et al., 2018).
Similar to lanthanide-based nanoparticles and quantum dots, molecular fluorophore-based nanoparticles can also emit in the NIR-II region as nanoprobes to aid in fluorescence-image guided surgery (FIGS). Hong et al. developed a polymethine-based nanoprobe that both absorbs and emits in the NIR-II region. The NIR-II dye is designed to substitute the pyrrole of the indocyanine green (ICG) into thiopyrylium to induce NIR-II excitation and emission (Ding B. et al., 2019). Its nanoparticle version was tested in mice for tumorous microvasculature imaging. The nanoprobe conjugates with cyclic arginine-glycine-aspartate motif (cRGD) via the polyethylene glycol (PEG) chain by a click reaction, and has the potential to realize glioma fluorescence imaging. Instead of using a benzo-bis(1,2,5-thiadiazole) acceptor-centered fluorophore (Sun et al., 2016), Xiao et al. discovered the thiophenthiadiazole-derived fluorophore, which can self-assemble into spherical or vesicular micelles (50–200 nm) after conjugating with the PEG chain through carboxylate groups (Li et al., 2022). These micelles can target solid tumors through the enhanced permeability and retention (EPR) effect. In vivo experiments in mice reveal that this nanoprobe has little immunogenicity, low binding affinity, ultralong blood circulation, and high contrast, which make it a promising novel tool for NIR-II FIGS.
NIR-II fluorescence-based drug delivery
The study and design of artificial delivery routes allows researchers to investigate treatments for critical diseases. Drug delivery systems are used to deliver drugs to the desired tissues and organs, and allow for controllable drug release by multifunctional drug carriers (Li N. et al., 2019; Zhang Y. et al., 2022). Drug delivery can solve many existing problems that limit the use of therapeutic drugs, such as poor solubility, lack of biodistribution and bioavailability, low selectivity, and side effects (Liu et al., 2015; Liu D. et al., 2018; Huang X. et al., 2021; Wei et al., 2021; Zhou et al., 2021; Kalot et al., 2022). Recently, NIR fluorophore-functionalized delivery systems targeting gliomas have been developed that allow the visualization of the delivery process and monitoring of drug behavior.
Grossman et al. tested lipid-based microbubbles (MBs), which are mature ultrasound contrast agents, as carriers (Paradossi et al., 2019). The glioma-targeting peptide, cRGD, which contains a cysteine moiety, was coupled to the MB through a maleimide group together with ICG. After engineering MBs with the dye moiety, ICG, and targeting moieties, the material was further tested in mice and demonstrated accumulation in gliomas without cytotoxicity at a dose of 253 mg/kg. MBs can carry some therapeutic agents, which means they have the potential to become the carriers for glioma drug delivery with coupled with NIR fluorescence imaging. Kim et al. further tested the drug delivery ability of the MBs. As ultrasound waves can oscillate and destroy the MBs, Kim et al. established an MB-based drug delivery system to cross the BBB, which typically limits the delivery of large molecules (400–500 g/mol) (Ha et al., 2019). In their system, Cy5.5 doped albumin nanoparticles decorated the surface of an NHS-functionalized MB. In this case, Cy5.5 was the NIR fluorophore and albumin was the glioma-targeting agent. By applying ultrasound (0.1 W/cm2, 10% duty cycle, 5 min), NIR fluorescent images of these engineered MBs in mouse brain reveal a strong fluorescence accumulation around gliomas compared to the no ultrasound group. This indicates that the BBB was penetrated efficiently, and the NIR fluorophores acted as imaging agents and drug carriers.
Instead of MBs, Shi et al. applied a similar strategy to liposomes, and investigated the precise delivery of (–)-gossypol (AT-101) as a drug for the treatment of glioma (Liu H. et al., 2022). The application of AT-101 is limited because of its hydrophobicity and non-tumor specific biodistribution. In their system, the cRGD (glioma targeting agent) and DiR (NIR fluorescence imaging agent) together with (-)-gossypol are coated on the surface of liposomes. NIR fluorescence enabled the researchers to monitor the release of (-)-gossypol in vivo.
The BBB limits the delivery of many therapeutic agents to glioma. Wang et al. adopted an in situ self-assembly strategy using nanotherapeutic agents to penetrate the BBB (Gao et al., 2020). Their system is based on RGD peptide-modified bisulfite-zinc(II)-dipicolylamine-Arg-Gly-Asp (Bis(DPA-Zn)-RGD), which is positively charged and is capable of targeting tumors. After the accumulation of Bis(DPA-Zn)-RGD in glioma, ultrasmall Au-ICG nanoparticles (∼7 nm) cross the BBB and self-assemble with Bis(DPA-Zn)-RGD.
Instead of tagging a carrier with an NIR fluorescent agent, Zhou et al. discovered an NIR fluorescent agent that could act as a carrier (Li et al., 2020). They discovered a large amino acid-mimicking material, functionalized carbon quantum dots (CQDs). The edges of the CQDs are functionalized with paired a-carboxyl and amino groups, which trigger multivalent interactions with the large neutral amino acid transporter 1 (LAT1). As LAT1 is the overexpressed carrier transporter in cancer cells, these CQDs can target glioma cells selectively. More importantly, a large p-conjugating system enabled the CQDs to transfer drugs through π–π interactions and emit NIR fluorescence as an imaging agent. The material demonstrated efficient BBB penetration during in vivo experiments and could act as both an NIR fluorescence imaging tool and a drug delivery carrier.
Zhang et al. developed an NIR-II fluorescent nano carriers with YVO4:Nd3+ particles as the cores and MnO2 nanosheets as the shells (Lv et al., 2022a). They tested the drug delivery ability of this material in glioma by loading the sonosensitizer, hematoporphyrin monomethyl ether, and glioma targeting agent, lactoferrin, onto the surface. The NIR-II fluorescent nanoparticles acted as carriers, but also enhanced the effect of the sonodynamic therapy (SDT) by providing O2 to the tumor from the MnO2 shell to appease the hypoxia caused by SDT. Animal experiments revealed that this material efficiently facilitated the treatment of glioma by SDT.
NIR-II based phototherapy
There are many NIR-II fluorescent agents available, not only for efficient fluorescence imaging, but also for phototherapy (Hu et al., 2021; Liu et al., 2021; Liu Y. et al., 2022; Zhang L. et al., 2022; Du et al., 2022; Lee et al., 2022; Nirmal et al., 2022; Shi et al., 2022; Sun et al., 2022). The level of deep tissue penetration using the NIR-II window significantly expands the application scope of phototherapy. Zhang et al. developed a graphene quantum dot (∼5 nm) doped with N and B as an NIR-II fluorescence imaging and photothermal therapeutic agent (Wang et al., 2019), respectively. This graphene quantum dot exhibits good photostability and excitation wavelength-dependent emissions in the NIR-II window. The NIR-II emissions attributed to the N and B doping induced a local distortion of electron energy, which created additional energy gaps, and a vacancy deficiency, which redshifted the emission peak. In vivo experiments revealed that this material is efficient for the NIR-II fluorescence imaging of internal organs and blood vessels. In vitro experiments on glioma cells revealed that the material has a high potential for application in PTT.
Kim et al. combined chemotherapeutic organoplatinum (II) metallacycles and NIR-II fluorophores with an FDA-approved amphiphilic polymer of poly (ethyleneoxide)-poly (propylene oxide)-poly (ethylene oxide) (Pluronic F127) to form a nanotheranostic agent (∼110 nm) (Ding F. et al., 2019). The polymer encapsulating two components improves the photostability, fluorescence tissue penetration, solubility, and biocompatibility as well as accumulation in tumor sites via the EPR effect. The glioma treatment was tested in vivo. Other novel materials can be combined to make nanotheranostic systems. Tian et al. developed a system suitable for both MRI and NIR-II fluorescence imaging by loading an Fe-based metal-organic framework with an NIR-II molecular fluorophore and AE105 peptide designed to target overexpressed urokinase plasminogen activator receptor on glioblastoma. In vivo studies reveal that this system can realize efficient PTT and achieve the successful ablation of tumors.
Conjugated polymers are promising materials for NIR-II fluorescence because the gap between the highest occupied molecular orbital (HOMO) and lowest unoccupied molecular orbital (LUMO) can be reduced. However, aggregation-induced quenching limits their applicability to the construction of nano therapeutic and imaging agents. Aggregation-induced emission (AIE) materials can solve this problem. AIE polymer-based nanomaterials with a high quantum yield (∼7.9% in water) have been developed by Deng et al. (2020). The AIE Polymer is encapsulated by lipids and then loaded with a nature killer cell membrane, which can target tumor cells and unzip the tight junction structure of BBB. In vivo animal experiments revealed that this nanomaterial is efficacious for NIR-II fluorescent through-skull imaging and PTT.
Previous AIE nanomaterials emit at approximately 1000 nm. Tang et al. pushed the emission wavelength to 1550 nm, which falls within the NIR-IIb window. To decrease the gap between HOMO and LUMO and gain a large redshift emission, a D-π-A AIE fluorophore was synthesized with planar blocks associated with a twisted skeleton to simultaneously achieve high absorbance and quantum yield (Wang et al., 2022a). The tail emission extends to 1550 nm, which allows NIR-IIb fluorescence imaging in vivo, and the D-π-A AIE fluorophore self-assembles into nano aggregates and is further loaded with the brain-targeting apolipoprotein E peptide. This nanoparticle has good BBB penetration and high PTT efficiency and prolongs the lifespan of mice suffering from orthotopic glioblastoma.
Conclusion and future perspectives
In this review, we have given some representative NIR fluorescent examples for solving problems of glioma diseases in the past 5 years. This review’s focus is on imaging, delivery and therapy. In the fluorescence imaging part, intraoperative glioma visualization with NIR-fluorescence has been highlighted since clinical trials have already been conducted. Application of fluorescence imaging of gliomas in the NIR region has flourished by significantly increasing the spatial and temporal resolution. However, it is noticed that NIR fluorescence as an information processing tool has been underestimated. Very few NIR fluorescent materials involving molecular logic gate concepts have been studied in gliomas. For example, most of the fluorescent probes have been designed as fluorophore at delivery ‘cargo’. In this way, the sensitivity will depend on the efficiency of glioma targeting. In the delivery part, NIR has been widely used as the monitoring tool in drug delivery and shows great efficacy in animal experiments. But this function is realized commonly by separately including fluorophores. The NIR-fluorescent delivery ‘cargo’ would be a promising area to develop. Phototherapy has the non-invasive advantage and minimal side effects which makes it ideal for glioma treatment. The NIR window improves the phototherapy’s efficacy by permitting deep tissue penetration. The NIR-II window even gives through-skull penetration in mouse experiments. Many novel materials have been designed to accomplish more red-shifted emissions.
To date, significant progress has been made towards the in vivo diagnosis of gliomas at the preoperative stage using fluorescence imaging in the NIR-II region. However, several challenges remain, such as the accurate detection of cancer metastasis at the preoperative or even intraoperative stage and improvement of the spatial and temporal resolution. Although the NIR-II spectra is available for through-skull penetration in vivo, the poor capacity of NIR-II nano-systems for crossing the BBB significantly limits the imaging contrast and therapeutic efficacy for glioma detection (Zhang H. et al., 2022; Lv et al., 2022b; Men et al., 2022). By equipping fluorescent probes with targeted delivery systems, the accumulation of NIR agents at glioma tumor sites can be improved. Thus, further investigation on the pathology of gliomas is necessary before new target sites can be found to enhance the targeting efficiency. Furthermore, the biocompability and biodistribution of NIR-II nano-systems should be evaluated and optimized to ensure their safety in clinical studies.
Author contributions
All authors contributed to the design of the study and writing of the manuscript. CY and JL wrote the main manuscript text and prepared figures. JL and CY revised the article critically for important intellectual content and final approval of the version to be submitted. All authors reviewed the manuscript.
Funding
This work was financially supported by Jiangsu Provincial Key Research and Development Program (Project No: BE2022766), Jiangsu Provincial Key Medical Center, Leverhulme Trust (RPG-2019–314), China Scholarship Council, Department of Education and Learning of Northern Ireland and Queen’s University Belfast.
Conflict of interest
The authors declare that the research was conducted in the absence of any commercial or financial relationships that could be construed as a potential conflict of interest.
Publisher’s note
All claims expressed in this article are solely those of the authors and do not necessarily represent those of their affiliated organizations, or those of the publisher, the editors and the reviewers. Any product that may be evaluated in this article, or claim that may be made by its manufacturer, is not guaranteed or endorsed by the publisher.
References
Balana, C., Castañer, S., Carrato, C., Moran, T., Lopez-Paradís, A., Domenech, M., et al. (2022). Preoperative diagnosis and molecular characterization of gliomas with liquid biopsy and radiogenomics. Front. Neurol. 13, 865171–865222. doi:10.3389/fneur.2022.865171
Bian, H., Ma, D., Zhang, X., Xin, K., Yang, Y., Peng, X., et al. (2021). Tailored engineering of novel xanthonium polymethine dyes for synergetic PDT and PTT triggered by 1064 nm laser toward deep-seated tumors. Small 17, 2100398–2100411. doi:10.1002/smll.202100398
Blümcke, I., Becker, A. J., Normann, S., Hans, V., Riederer, B. M., Krajewski, S., et al. (2001). Distinct expression pattern of microtubule-associated protein-2 in human oligodendrogliomas and glial precursor cells. J. Neuropathol. Exp. Neurol. 60, 984–993. doi:10.1093/jnen/60.10.984
Cai, X., Liu, B., d’Angelo, M., Castelli, V., Benedetti, E., Antonosante, A., et al. (2020). Aggregation-Induced Emission: Recent Advances in Materials and Biomedical Applications, 597, 98681–988614. doi:10.1002/anie.20200084510.3389/fbioe.2019.00325Angew. Chem. - Int. Ed.Theranostic nanomedicine for malignant gliomasFront. Bioeng. Biotechnol.
Daly, B., Ling, J., and De Silva, A. P. (2015). Current developments in fluorescent PET (photoinduced electron transfer) sensors and switches. Chem. Soc. Rev. 44, 4203–4211. doi:10.1039/c4cs00334a
Dasgupta, T., and Haas-Kogan, D. A. (2013). The combination of novel targeted molecular agents and radiation in the treatment of pediatric gliomas. Front. Oncol. 3, 110–118. doi:10.3389/fonc.2013.00110
Deng, G., Peng, X., Sun, Z., Zheng, W., Yu, J., Du, L., et al. (2020). Natural-killer-cell-inspired nanorobots with aggregation-induced emission characteristics for near infrared-II fluorescence-guided glioma theranostics. ACS Nano 14, 11452–11462. doi:10.1021/acsnano.0c03824
Ding, B., Xiao, Y., Zhou, H., Zhang, X., Qu, C., Xu, F., et al. (2019a). Polymethine thiopyrylium fluorophores with absorption beyond 1000 nm for biological imaging in the second nearinfrared subwindow. J. Med. Chem. 62, 2049–2059. doi:10.1021/acs.jmedchem.8b01682
Ding, F., Chen, Z., Kim, W. Y., Sharma, A., Li, C., Ouyang, Q., et al. (2019b). A nano-cocktail of an NIR-II emissive fluorophore and organoplatinum(ii) metallacycle for efficient cancer imaging and therapy. Chem. Sci. 10, 7023–7028. doi:10.1039/c9sc02466b
Du, H., Yang, F., Yao, C., Zhong, Z., Jiang, P., Stanciu, S. G., et al. (2022). Multifunctional modulation of high‐performance Zn x Fe 3− x O 4 nanoparticles by precisely tuning the zinc doping content. Small 18, e2201669. doi:10.1002/smll.202201669
Gao, H., Chu, C., Cheng, Y., Zhang, Y., Pang, X., Li, D., et al. (2020). In situ formation of nanotheranostics to overcome the blood-brain barrier and enhance treatment of orthotopic glioma. ACS Appl. Mat. Interfaces 12, 26880–26892. doi:10.1021/acsami.0c03873
Ghirardello, M., Shyam, R., Liu, X., Garcia-Millan, T., Sittel, I., Ramos-Soriano, J., et al. (2022). Carbon dot-based fluorescent antibody nanoprobes as brain tumour glioblastoma diagnostics. Nanoscale Adv. 4, 1770–1778. doi:10.1039/d2na00060a
Guilbault G. G. (Editor) (2020). Practical fluorescence. Second Edition. Boca Raton, Florida, United States: CRC Press. doi:10.1201/9781003066514
Guo, Z., Ma, Y., Liu, Y., Yan, C., Shi, P., Tian, H., et al. (2018). Photocaged prodrug under NIR light-triggering with dual-channel fluorescence: In vivo real-time tracking for precise drug delivery. Sci. China Chem. 61, 1293–1300. doi:10.1007/s11426-018-9240-6
Ha, S. W., Hwang, K., Jin, J., Cho, A. S., Kim, T. Y., Hwang, S. Il, et al. (2019). <p>Ultrasound-sensitizing nanoparticle complex for overcoming the blood-brain barrier: An effective drug delivery system</p>. Int. J. Nanomedicine 14, 3743–3752. doi:10.2147/IJN.S193258
Hu, X., Li, J., Chen, Y., Long, Q., Bai, Y., Li, R., et al. (2022). A self-assembly ICG nanoparticle potentiating targeted photothermal and photodynamic therapy in NSCLC. ACS Biomater. Sci. Eng. 8, 4535–4546. doi:10.1021/acsbiomaterials.2c00620
Huang, X., Ma, Y., Li, Y., Han, F., and Lin, W. (2021a). Targeted drug delivery systems for kidney diseases. Front. Bioeng. Biotechnol. 9, 683247. doi:10.3389/fbioe.2021.683247
Huang, Z., Li, N., Zhang, X., and Xiao, Y. (2021b). Mitochondria-anchored molecular thermometer quantitatively monitoring cellular inflammations. Anal. Chem. 93, 5081–5088. doi:10.1021/acs.analchem.0c04547
Ji, Z., Ai, P., Shao, C., Wang, T., Yan, C., Ye, L., et al. (2018). Manganese-doped carbon dots for magnetic resonance/optical dual-modal imaging of tiny brain glioma. ACS Biomater. Sci. Eng. 4, 2089–2094. doi:10.1021/acsbiomaterials.7b01008
Kalot, G., Godard, A., Busser, B., Bendellaa, M., Dalonneau, F., Paul, C., et al. (2022). Lipoprotein interactions with water-soluble NIR-II emitting aza-BODIPYs boost the fluorescence signal and favor selective tumor targeting. Biomater. Sci. 10, 6315–6325. doi:10.1039/d2bm01271e
Khaddour, K., Johanns, T. M., and Ansstas, G. (2020). The landscape of novel therapeutics and challenges in glioblastoma multiforme: Contemporary state and future directions. Pharmaceuticals 13, 389–426. doi:10.3390/ph13110389
Lee, G., Choi, H. E., Hong, S. H., Choi, M., Han, D. W., Lee, J., et al. (2022). Upconversion nanomaterials and delivery systems for smart photonic medicines and healthcare devices. Adv. Drug Deliv. Rev. 188, 114419. doi:10.1016/j.addr.2022.114419
Li, L., Chen, Y., Chen, W., Tan, Y., Chen, H., and Yin, J. (2019a). Photodynamic therapy based on organic small molecular fluorescent dyes. Chin. Chem. Lett. 30, 1689–1703. doi:10.1016/j.cclet.2019.04.017
Li, N., Huang, Z., Zhang, X., Song, X., and Xiao, Y. (2019b). Reflecting size differences of exosomes by using the combination of membrane-targeting viscosity probe and fluorescence lifetime imaging microscopy. Anal. Chem. 91, 15308–15316. doi:10.1021/acs.analchem.9b04587
Li, S., Su, W., Wu, H., Yuan, T., Yuan, C., Liu, J., et al. (2020). Targeted tumour theranostics in mice via carbon quantum dots structurally mimicking large amino acids. Nat. Biomed. Eng. 4, 704–716. doi:10.1038/s41551-020-0540-y
Li, Y., Gao, J., Wang, S., Du, M., Hou, X., Tian, T., et al. (2022). Self-assembled NIR-II fluorophores with ultralong blood circulation for cancer imaging and image-guided surgery. J. Med. Chem. 65, 2078–2090. doi:10.1021/acs.jmedchem.1c01615
Ling, J., Luo, Y., Sun, C., Dong, Z., Wu, R., Tang, X., et al. (2021). Live intraoperative diagnosis of hepatic metastasis via HDACs targeting molecular theranostic agent. Chem. Eng. J. 406, 126900. doi:10.1016/j.cej.2020.126900
Liu, D., Cito, S., Zhang, Y., Wang, C. F., Sikanen, T. M., and Santos, H. A. (2015). A versatile and robust microfluidic platform toward high throughput synthesis of homogeneous nanoparticles with tunable properties. Adv. Mat. 27, 2298–2304. doi:10.1002/adma.201405408
Liu, D., Zhang, H., Fontana, F., Hirvonen, J. T., and Santos, H. A. (2018a). Current developments and applications of microfluidic technology toward clinical translation of nanomedicines. Adv. Drug Deliv. Rev. 128, 54–83. doi:10.1016/j.addr.2017.08.003
Liu, H. W., Chen, L., Xu, C., Li, Z., Zhang, H., Zhang, X. B., et al. (2018b). Recent progresses in small-molecule enzymatic fluorescent probes for cancer imaging. Chem. Soc. Rev. 47, 7140–7180. doi:10.1039/c7cs00862g
Liu, H., Zhang, R., Zhang, D., Zhang, C., Zhang, Z., Fu, X., et al. (2022a). Cyclic RGD-decorated liposomal gossypol AT-101 targeting for enhanced antitumor effect. Int. J. Nanomedicine 17, 227–244. doi:10.2147/IJN.S341824
Liu, Y., Huang, J., and Liu, J. (2022b). Single laser activated photothermal/photodynamic dual-modal cancer phototherapy by using ROS-responsive targeting flower-like ruthenium nanoparticles. J. Mat. Chem. B 10, 7760–7771. doi:10.1039/d2tb01276f
Liu, Y., Wang, Y., Song, S., and Zhang, H. (2021). Tumor diagnosis and therapy mediated by metal phosphorus-based nanomaterials. Adv. Mat. 33, e2103936. doi:10.1002/adma.202103936
Liu, Z., Ren, F., Zhang, H., Yuan, Q., Jiang, Z., Liu, H., et al. (2019). Boosting often overlooked long wavelength emissions of rare-earth nanoparticles for NIR-II fluorescence imaging of orthotopic glioblastoma. Biomaterials 219, 119364. doi:10.1016/j.biomaterials.2019.119364
Lu, L., Li, B., Ding, S., Fan, Y., Wang, S., Sun, C., et al. (2020). NIR-II bioluminescence for in vivo high contrast imaging and in situ ATP-mediated metastases tracing. Nat. Commun. 11, 4192–4211. doi:10.1038/s41467-020-18051-1
Lv, Z., Jin, L., Cao, Y., Zhang, H., Xue, D., Yin, N., et al. (2022a). A nanotheranostic agent based on Nd3+-doped YVO4 with blood-brain-barrier permeability for NIR-II fluorescence imaging/magnetic resonance imaging and boosted sonodynamic therapy of orthotopic glioma. Light. Sci. Appl. 11, 116. doi:10.1038/s41377-022-00794-9
Lv, Z., Jin, L., Gao, W., Cao, Y., Zhang, H., Xue, D., et al. (2022b). Novel YOF-based theranostic agents with a cascade effect for NIR-II fluorescence imaging and synergistic starvation/photodynamic therapy of orthotopic gliomas. ACS Appl. Mat. Interfaces 14, 30523–30532. doi:10.1021/acsami.2c05354
McNamara, M. G., Lwin, Z., Jiang, H., Templeton, A. J., Zadeh, G., Bernstein, M., et al. (2014). Factors impacting survival following second surgery in patients with glioblastoma in the temozolomide treatment era, incorporating neutrophil/lymphocyte ratio and time to first progression. J. Neurooncol. 117, 147–152. doi:10.1007/s11060-014-1366-9
Men, X., Geng, X., Zhang, Z., Chen, H., Du, M., Chen, Z., et al. (2022). Biomimetic semiconducting polymer dots for highly specific NIR-II fluorescence imaging of glioma. Mat. Today Bio 16, 100383. doi:10.1016/j.mtbio.2022.100383
Mishchenko, T. A., Mitroshina, Y. V., Smyshlyaeva, A. S., Guryev, Y. L., and Vedunova, M. V. (2020). Comparative analysis of the effects of upconversion nanoparticles on normal and tumor brain cells. Acta Naturae 12, 86–94. doi:10.32607/ACTANATURAE.11033
Misra, R., and Bhattacharyya, S. P. (2018). Intramolecular charge transfer: Theory and applications. Weinheim: Wiley VCH. doi:10.1002/9783527801916
Nirmal, G. R., Lin, Z. C., Lin, C. H., Sung, C. T., Liao, C. C., and Fang, J. Y. (2022). Polydopamine/IR820 nanoparticles as topical phototheranostics for inhibiting psoriasiform lesions through dual photothermal and photodynamic treatments. Biomater. Sci. 10, 6172–6189. doi:10.1039/d2bm00835a
Ostrom, Q. T., Patil, N., Cioffi, G., Waite, K., Kruchko, C., and Barnholtz-Sloan, J. S. (2020). CBTRUS statistical report: Primary brain and other central nervous system tumors diagnosed in the United States in 2013-2017. Neuro. Oncol. 22, IV1–IV96. doi:10.1093/neuonc/noaa200
Paradossi, G., Oddo, L., Cerroni, B., Ben-Harush, C., Ariel, E., Di Meco, F., et al. (2019). In vivo toxicity study of engineered lipid microbubbles in rodents. ACS Omega 4, 5526–5533. doi:10.1021/acsomega.8b03161
Patsenker, L., and Gellerman, G. (2020). Fluorescent reporters for drug delivery monitoring. Isr. J. Chem. 60, 504–518. doi:10.1002/ijch.201900137
Razpotnik, R., Novak, N., Curin Šerbec, V., and Rajcevic, U. (2017). Targeting malignant brain tumors with antibodies. Front. Immunol. 8, 1181–1214. doi:10.3389/fimmu.2017.01181
Ren, F., Liu, H., Zhang, H., Jiang, Z., Xia, B., Genevois, C., et al. (2020). Engineering NIR-IIb fluorescence of Er-based lanthanide nanoparticles for through-skull targeted imaging and imaging-guided surgery of orthotopic glioma. Nano Today 34, 100905–100906. doi:10.1016/j.nantod.2020.100905
Sailor, M. J., and Park, J. H. (2012). Hybrid nanoparticles for detection and treatment of cancer. Adv. Mat. 24, 3779–3802. doi:10.1002/adma.201200653
Schäferling, M. (2012). The art of fluorescence imaging with chemical sensors. Angew. Chem. Int. Ed. 51, 3532–3554. doi:10.1002/anie.201105459
Shi, T., Huang, C., Li, Y., Huang, F., and Yin, S. (2022). NIR-II phototherapy agents with aggregation-induced emission characteristics for tumor imaging and therapy. Biomaterials 285, 121535. doi:10.1016/j.biomaterials.2022.121535
Silantyev, A. S., Falzone, L., Libra, M., Gurina, O. I., Kardashova, K. S., Nikolouzakis, T. K., et al. (2019). Current and future trends on diagnosis and prognosis of glioblastoma: From molecular biology to proteomics. Cells 8. doi:10.3390/cells8080863
Sun, J., Feng, E., Shao, Y., Lv, F., Wu, Y., Tian, J., et al. (2022). A selenium-substituted heptamethine cyanine photosensitizer for near infrared photodynamic therapy. Chembiochem., e202200421. doi:10.1002/cbic.202200421
Sun, Y., Qu, C., Chen, H., He, M., Tang, C., Shou, K., et al. (2016). Novel benzo-bis(1, 2, 5-thiadiazole) fluorophores for: In vivo NIR-II imaging of cancer. Chem. Sci. 7, 6203–6207. doi:10.1039/c6sc01561a
Tang, X., Chen, X., Zhang, S., Gu, X., Wu, R., Huang, T., et al. (2021). Silk-inspired in situ hydrogel with anti-tumor immunity enhanced photodynamic therapy for melanoma and infected wound healing. Adv. Funct. Mat. 31, 2101320–2101412. doi:10.1002/adfm.202101320
Wang, H., Mu, Q., Wang, K., Revia, R. A., Yen, C., Gu, X., et al. (2019). Nitrogen and boron dual-doped graphene quantum dots for near infrared second window imaging and photothermal therapy. Appl. Mat. Today 14, 108–117. doi:10.1016/j.apmt.2018.11.011
Wang, J., Liu, Y., Morsch, M., Lu, Y., Shangguan, P., Han, L., et al. (2022a). Brain-targeted aggregation-induced-emission nanoparticles with near infrared imaging at 1550 nm boosts orthotopic glioblastoma theranostics. Adv. Mat. 34, 2106082. doi:10.1002/adma.202106082
Wang, Z., Zhang, M., Chi, S., Zhu, M., Wang, C., and Liu, Z. (2022b22005). Brain tumor cell membrane-coated lanthanide-doped nanoparticles for NIR-IIb luminescence imaging and surgical navigation of glioma. Adv. Healthc. Mat. 11, 2200521–2200610. doi:10.1002/adhm.202200521
Wei, J., Ding, J., Han, B., and Chen, C. (2021). Editorial: Advanced materials for the restoration and reconstruction of dental functions. Front. Bioeng. Biotechnol. 9, 756860. doi:10.3389/fbioe.2021.756860
Wen, P. Y., and Kesari, S. (2008). Malignant gliomas in adults. N. Engl. J. Med. 359, 492–507. doi:10.1056/NEJMra0708126
Wesseling, P., and Capper, D. (2018). WHO 2016 Classification of gliomas. Neuropathol. Appl. Neurobiol. 44, 139–150. doi:10.1111/nan.12432
Wu, S., Yan, Y., Hou, H., Huang, Z., Li, D., Zhang, X., et al. (2022). Polarity-sensitive and membrane-specific probe quantitatively monitoring ferroptosis through fluorescence lifetime imaging. Anal. Chem. 94, 11238–11247. doi:10.1021/acs.analchem.2c01737
Xiang, C., Dirak, M., Luo, Y., Peng, Y., Cai, L., Gong, P., et al. (2022). A responsive AIE-active fluorescent probe for visualization of acetylcholinesterase activity in vitro and in vivo. Mat. Chem. Front. 6, 1515–1521. doi:10.1039/d2qm00239f
Xu, Y., Zhao, Y., Zhang, Y., Cui, Z., Wang, L., Fan, C., et al. (2018). Angiopep-2-conjugated Ag2S quantum dot for NIR-II imaging of brain tumors. Acta chimi. Sin. 76, 393–399. doi:10.6023/A18010039
Zhang, H., Yu, D., Liu, S., Liu, C., Liu, Z., Ren, J., et al. (2022a). NIR-II hydrogen-bonded organic frameworks (HOFs) used for target-specific amyloid-beta photooxygenation in an alzheimer's disease model. Angew. Chem. Int. Ed. Engl. 61, e202109068. doi:10.1002/anie.202109068
Zhang, L., Liu, Y., Huang, H., Xie, H., Zhang, B., Xia, W., et al. (2022b). Multifunctional nanotheranostics for near infrared optical imaging-guided treatment of brain tumors. Adv. Drug Deliv. Rev. 190, 114536. doi:10.1016/j.addr.2022.114536
Zhang, X., Chen, Y., He, H., Wang, S., Lei, Z., and Zhang, F. (2021). ROS/RNS and base dual activatable merocyanine-based NIR-II fluorescent molecular probe for in vivo biosensing. Angew. Chem. Int. Ed. 60, 26337–26341. doi:10.1002/anie.202109728
Zhang, Y., Tian, J., Xiao, F., Zheng, L., Zhu, X., Wu, L., et al. (2022c). B cell-activating factor and its targeted therapy in autoimmune diseases. Cytokine Growth Factor Rev. 64, 57–70. doi:10.1016/j.cytogfr.2021.11.004
Keywords: fluorescence imaging, NIR dyes, delivery system, photodynamic therapy, photothermal therapy, glioma
Citation: Li J, Ling J and Yao C (2022) Recent advances in NIR-II fluorescence based theranostic approaches for glioma. Front. Chem. 10:1054913. doi: 10.3389/fchem.2022.1054913
Received: 27 September 2022; Accepted: 24 October 2022;
Published: 04 November 2022.
Edited by:
Xinfu Zhang, Dalian University of Technology, ChinaReviewed by:
Benhua Wang, Central South University, ChinaXin Yu Tian, Nanjing Drum Tower Hospital, China
Copyright © 2022 Li, Ling and Yao. This is an open-access article distributed under the terms of the Creative Commons Attribution License (CC BY). The use, distribution or reproduction in other forums is permitted, provided the original author(s) and the copyright owner(s) are credited and that the original publication in this journal is cited, in accordance with accepted academic practice. No use, distribution or reproduction is permitted which does not comply with these terms.
*Correspondence: Chaoyi Yao, Y3lhbzAxQHF1Yi5hYy51aw==; Jue Ling, amwyMDE2QG50dS5lZHUuY24=