- College of Physics and Electronic Engineering, Chongqing Normal University, Chongqing, China
Heusler compounds exhibit many interesting properties, such as high thermopower, magnetocaloric properties, and even topological insulator states. Heusler Mn2CoAl alloy has been experimentally and theoretically proposed as a promising spin-gapless semiconductor with novel electronic, magnetic, spintronic, transport, and topological properties. Furthermore, the spin-gapless semiconducting-like behaviors are also predicted in Mn2CoAl films by measuring the transport and magnetic properties. This mini-review systematically summarizes the interesting properties of Mn2CoAl bulk and Mn2CoAl-based films. This mini-review is hoped to guide further experimental investigations and applications in the particular scientific community.
Introduction
On the one side, in 2008, Liu et al. (2008) investigated the structural, electronic, and magnetic properties of Mn2CoZ (Z = Al, Si, Ge, Sn, and Sb) alloys with Hg2CuTi-type structure with the help of first-principles calculations, and they stated that all the Mn2CoZ alloys belong to ferrimagnetic half-metals (Fang et al., 2002; Dowben and Skomski, 2004; Müller et al., 2009; Ashton et al., 2017). It is noteworthy that the spin-gapless semiconducting state of Mn2CoAl has not been mentioned by Liu et al. (2008). Moreover, Liu et al. (2008) stated that the Mn2CoZ (Z = Al, Si, Ge, Sn, and Sb) alloys follow the Slater-Pauling rule Mt =NV−24 (Mt denotes the total magnetic moment and the NV is the valence electrons in both spin channels). Then, Liu et al. (2008) successfully synthesized the Hg2CuTi-type Mn2CoZ alloys, in which the two Mn atoms exhibit different magnetic behaviors. In the same year, Xing et al. (2008) also proposed the half-metallic ferrimagnetism of the Heusler alloys Mn2CoZ (Z = Al, Ga, Si, Ge) using the first-principles plane-wave pseudopotential method. Moreover, they (Xing et al., 2008) pointed out that the half-metallic states in Mn2CoZ can maintain in a large range of lattice constants, reflecting robust half-metallic behaviors. In 2011, Meinert, Schmalhorst, and Reiss (Meinert et al., 2011) studied the complex magnetic interactions between the constituents and the Curie temperatures using first-principles calculations. Surprisingly, the Curie temperatures of Mn2CoAl/Ga/In are all above 800 K.
On the other side, in 2008, Wang, (2008) proposed the concept of a spin-gapless semiconductor. The spin-gapless semiconductor is a new class of zero-gap materials. Spin-gapless semiconductors for practical use should feature 1) completely spin polarized carriers; 2) high mobility of carriers; 3) zero or negligibly small excitation energy of electrons from the valence to the conduction band; 4) easy switching between electron and hole modes by tuning the Fermi level, owing to the ambipolar nature of the band gap (For type II spin-gapless semiconductors). Wang, (2008) proposed that by introducing magnetic ions into the parent nonmagnetic gapless compounds, such as PbPdO2, the spin-gapless semiconducting state can appear. Based on his work, a series of spin-gapless semiconductors (Li et al., 2009; Gao et al., 2015; Wang et al., 2016a; Galanakis et al., 2016; Gao et al., 2016; He et al., 2017; Wang, 2017; Deng et al., 2018a; Huang et al., 2019a; Nadeem et al., 2020; Yue et al., 2020; Yang et al., 2021) with parabolic or linear dispersion between energy and momentum are proposed. More interestingly, topological signatures, such as Dirac point and nodal line states, can be found in spin-gapless semiconductors. For example, He et al. (2017) proposed that NiCl3 monolayer is a near-room-temperature Dirac spin-gapless semiconductor when the spin-orbital coupling (SOC) is absent. When SOC is added, it becomes an intrinsic Chern insulator with a large non-trivial band gap of ∼24 meV, at which the quantum anomalous Hall effect could be observed. In 2018, Zhang et al. (2018) proposed the nodal ring spin-gapless semiconducting state in a 2D HK lattice via first-principle calculations. In 2022, Ding et al. (2022) summarized almost all the predicted nodal ring/line spin-gapless semiconductors in 2D and 3D materials (Guan et al., 2013; Li and Yang, 2013; Ding and Wang, 2015; Wang et al., 2016b; Rasool et al., 2016; Wang et al., 2017c; Liu et al., 2017; Deng et al., 2018b; Wang et al., 2018; Huang et al., 2019b; Wu et al., 2020b; Guo et al., 2020; Li et al., 2021; Wang et al., 2021; Ji et al., 2022; Wu et al., 2022) in the past 3 years. Remarkably, they (Ding et al., 2022) also provided three valuable suggestions for the future theoretical design of nodal ring/line spin-gapless semiconductors. Moreover, some quaternary Heusler SGSs (Bainsla et al., 2015; Rani et al., 2019) have been prepared, and their interesting gapless behavior has been confirmed. For the verification of the spin-gapless semiconducting property, their specific transport behavior has been widely measured and accepted as strong evidence.
In 2013, the spin-gapless semiconducting state in Mn2CoAl has been experimentally confirmed by Ouardi et al. (2013a) according to the transport behaviors. They reported that Mn2CoAl with robust spin polarization is a promising material for room-temperature semiconductor spintronics. In this mini-review, the properties of Mn2CoAl bulk, Mn2CoAl [001] surface, Mn2CoAl/GaAs heterostructures, Mn2CoAl/Ag/Mn2CoAl current-perpendicular-to-plane spin valves, MgO/Mn2CoAl/Pd trilayers, and various types of Mn2CoAl films are reviewed in details. This mini-review aims to provide an improved understanding of the properties for Mn2CoAl that have been reported in the last 14 years.
Structural, electronic, elastic, and thermodynamic properties for Mn2CoAl bulk
The crystal structure of the Heusler alloys can be viewed as a cubic structure with four interpenetrating f.c.c. Sublattices A, B, C, D (see Figure 1A). Normally, full-Heusler alloys X2YZ can host two types of structures (XA and L21 type structures). As shown in Figure 1A, we exhibited the XA type and L21 type Mn2CoAl. MnMnCoAl and MnCoMnAl represent the XA type Mn2CoAl and L21 type Mn2CoAl, respectively. As mentioned above, Liu et al. (2008) have determined that the crystal structure of Heusler Mn2CoAl should be XA type (i.e., Hg2CuTi) instead of L21. We would like to point out that other researchers have widely investigated the competition between XA and L21 atomic ordering in full Heusler alloys (Wang et al., 2017a; Wang et al., 2017b; Han et al., 2019a; Han et al., 2019b; Wu et al., 2019; Wu et al., 2020a) in recent years.
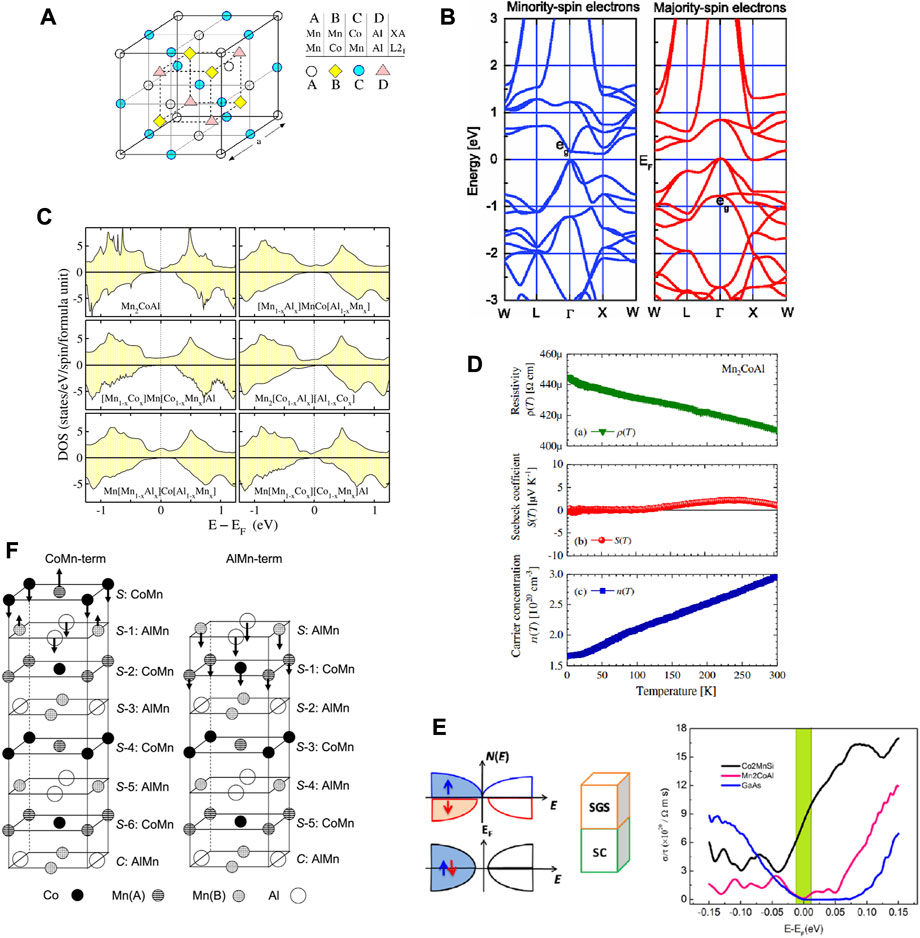
FIGURE 1. (A) Crystal structures of XA and L21 type Mn2CoAl. (B) Band structures of XA Mn2CoAl. (C) The density of states of Mn2CoAl by considering atomic swaps. (D) Measured resistivity, S(T), and n for Mn2CoAl sample under different temperatures. (E) Schematic diagrams of spin-gapless semiconductor (SGS)/semiconductor (SC) spin injection scheme and the calculated room temperature conductivities for half-metal Co2MnSi, spin-gapless semiconductor Mn2CoAl, and semiconductor GaAs. (F) The (001) Mn2CoAl surface with Co-Mn and Al-Mn terminated surfaces. Reproduced from Refs. (Ouardi et al., 2013a; Li and Jin, 2013; Galanakis et al., 2014; Xu et al., 2019) with permissions.
Based on the determined XA structure, the spin-polarized band structures for Mn2CoAl are exhibited in Figure 1B. Obviously, in the minority-spin channel, a direct band gap can be found around the Fermi level, whereas, in the majority-spin channel, an indirect zero-band gap can be observed. Note that, such band structures allow for tunable spin transport. Mn2CoAl hosts a high Curie temperature of ∼720 K and a total Mt of 2 μB. In 2015, Chen et al. (2015) investigated the spin-gapless semiconducting states and the dynamical stability of Mn2CoAl, and they found that the spin-gapless semiconducting states and the dynamical stability can maintain with a pressure less than 25 GPa. The calculated elastic modulus, shear modulus, Yong’s modulus, and Pugh’s ration for Mn2CoAl under equilibrium lattice constants are 185.778, 77.782, 204.768 GPa, and 2.39, respectively. Moreover, with the help of the quasiharmonic Debye model, the pressure and temperature dependences of normalized volume V/V0, bulk modulus B, thermal expansivity, Gruneisen parameter, heat capacity, and Debye temperature are evaluated by Chen et al. (2015) for Mn2CoAl up to 25 GPa. The above-listed data can be viewed as a reference for the follow-up experimental studies.
Conditions for spin-gapless semiconducting behavior in Mn2CoAl bulk
In 2014, Galanakis et al. (2014) examined the conditions for the spin-gapless semiconducting state in Mn2CoAl via first-principle calculations. They (Galanakis et al., 2014) showed that the spin-gapless semiconducting states in Mn2CoAl can be kept by applying the tetragonalization of the lattice. However, the spin-gapless semiconducting states in Mn2CoAl are disappeared by considering the atomic swaps. Swapping the atoms induces a physics nature transition from a spin-gapless semiconducting state to a half-metallic state (as shown in Figure 1C). Furthermore, they (Galanakis et al., 2014) also pointed out that the appearance of Co-surplus will lead to half-metallic states.
Experimentally verified for the spin-gapless semiconducting behavior in Mn2CoAl bulk
In 2013, the transport measurements of Mn2CoAl bulk were performed by Ouardi et al. (Ouardi et al., 2013b; Ouardi et al., 2019) via a physical properties measurement system. They pointed out that Mn2CoAl bulk hosts a nonmetallic resistivity (see Figure 1D). Note that the resistivity of Mn2CoAl bulk is two orders of magnitude higher than that of Co2FeSi metal. From Figure 1D, one finds that the carrier concentration (n) is temperature independent, and the Seebeck coefficient (S(T)) is vanishing. The reason for the appearance of a vanishing S(T) is the compensation of the electron and hole. Note that the temperature-independent n is a main feature for the gapless systems.
Combining the transport and magnetic properties, one can conclude that Mn2CoAl bulk should be a spin-gapless semiconductor. Ouardi et al. (Ouardi et al., 2013b; Ouardi et al., 2019) also reported the magnetoresistance results and the anomalous Hall conductivity of Mn2CoAl bulk, suggesting Mn2CoAl is a novel spintronic material.
Note that the transport and magnetic properties cannot be viewed as the only criteria to judge the spin-gapless semiconducting states in Heusler alloys. The microstructure observations (Xu et al., 2019) should also be considered to validate the spin-gapless semiconducting states in Heusler alloys.
New spin injection scheme based on Mn2CoAl
Spin injection efficiency based on conventional and/or half-metallic ferromagnets/semiconductors is greatly limited by the Schmidt barrier due to conductivity mismatch. In 2015, Xu et al. (2019) proposed that the spin-gapless semiconductor, such as Mn2CoAl, can be used to replace the conventional and/or half-metallic ferromagnets and form a spin-gapless semiconductor/semiconductor heterostructure (as shown in Figure 1E).
From Figure 1E, we listed the calculated room temperature conductivities for half-metal Co2MnSi, spin-gapless semiconductor Mn2CoAl, and semiconductor GaAs. The figure shows that the conductivity of spin-gapless semiconductor Mn2CoAl is much lower than that of half-metal Co2MnSi. More importantly, the conductivity of spin-gapless semiconductor Mn2CoAl is very close to that of semiconductor GaAs. Hence, using spin-gapless semiconductors as the magnetic injectors can reduce the conductive mismatch and enhance the spin injection efficiency.
We would like to point out that the thermodynamic stability, magnetism, and half metallicity of Mn2CoAl/GaAs (0 0 1) interface have been studied by Feng et al. (Feng et al., 2015a) via first principle calculations in 2015. In this same year, Feng et al. (Feng et al., 2015b) studied the effect of disorder on the electronic and magnetic properties of Mn2CoAl/GaAs heterostructures from theory.
Electronic structures, magnetism, and half-metallicity for [001] Mn2CoAl surface
In 2013, Li and Jin (2013) studied the electronic, magnetic, and half-metallic properties of the Mn2CoAl [001] surface by first-principles calculation. As shown in Figure 1F, two types of surface terminations, i.e., AlMn terminated and CoMn terminated surfaces, are considered by Li and Jin (2013). They Li and Jin (2013) reported that the AlMn-terminated Mn2CoAl surface hosts half-metallic behavior, whereas the CoMn-terminated Mn2CoAl surface does not have the half-metallic behavior.
In 2018, Meng et al. (Wei et al., 2018) investigated the interfacial electronic, magnetic, and spin transport properties of Mn2CoAl/Ag/Mn2CoAl current-perpendicular-to-plane spin valves. Interestingly, they (Wei et al., 2018) pointed out that the MnCoT-terminated interface enjoys the largest interface spin polarization of 78% and magnetoresistance ratio of 2,886%.
Experimental Mn2CoAl based films
To this date, some investigations are performed on Mn2CoAl-based films in the experiment. Hence, this section reviews some interesting properties focusing on the Mn2CoAl-based films. In 2018, Arima et al. (2018) studied the electronic structures and the anomalous Hall conductivity of Si-substituted Mn2CoAl epitaxial films. Based on the calculated density of states in Figure 2A, one finds that the spin-gapless semiconducting-like behavior can be maintained in Mn2CoAl1-xSix with x < 0.2. Mn2CoAl1-xSix films (0 ≤ x ≤ 0.3) were grown on MgAl2O4 (100) substrates by molecular beam epitaxy. We collect the θ-2θ x-ray diffraction of the Mn2CoAl1-xSix films in Figure 2B. Arima et al. (2018) stated that the electrical conductivity of Mn2CoAl0.8Si0.2 film is 2340 S/cm, which is closes to that of Mn2CoAl bulk (2440 S/cm).
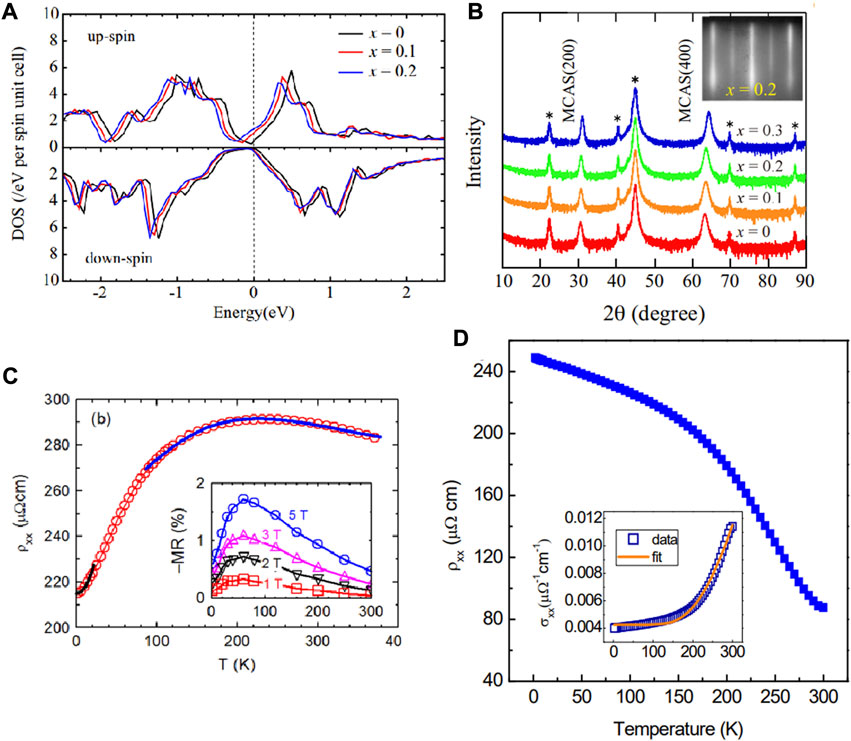
FIGURE 2. (A) Density of states for Mn2CoAl1-xSix (x = 0, 0.1, and 0.2). (B) The θ-2θ x-ray diffraction of the Mn2CoAl1-xSix films. (C) Resistivity versus temperature (red circles). Insert shows magnetoresistance at several magnetic fields. (D) The relationship between the resistivity and temperature was measured at zero magnetic fields. The inset shows the fitting of conductivity. Reproduced from Refs. (Jamer et al., 2013; Xu et al., 2014; Arima et al., 2018) with permissions.
In 2013, Jamer et al. (2013) prepared Mn2CoAl films on GaAs (001) substrates using molecular beam epitaxy. From Figure 2C, one finds the low-temperature resistivity of a 69 nm thick Mn2CoAl film is about 220 μΩcm, and a metallic-like behavior at low temperatures. They (Jamer et al., 2014) also reported that the Mn2CoAl films on GaAs (001) substrates exhibited varying amounts of disorder under different annealing temperatures, resulting in the magnetism changing. As the annealing temperature increases, the Mt increases.
In 2014, Xu et al. (2014) prepared Mn2CoAl films on the thermally oxidized Si substrates by magnetron sputtering deposition. They found that the films host a semiconducting-like resistivity and linear magnetoresistance in the whole region (see Figure 2D). Xu et al. (2014) also reported the unusually low anomalous Hall conductivity, saturation magnetization (1.94 μB at 5 K), and the Curie temperature (∼550 K). Usually, the results mentioned above are the transport signatures of spin-gapless semiconductors. In 2018, Chen et al. (2018) prepared Mn2CoAl films on MgO (001) substrates using molecular beam epitaxy. Their electro- and magneto-transport results showed that the Mn2CoAl hosts spin gapless semiconducting mechanisms at low temperatures. In 2017, Ludbrook and the collaborators (Ludbrook et al., 2017) showed that the MgO/Mn2CoAl/Pd trilayers could exhibit a novel topological Hall effect in temperatures between 3 K and 280 K. The topological Hall effect is evidence of skyrmions.
Summary
In this mini-review, the electronic, magnetic, and transport properties of Mn2CoAl bulk, Mn2CoAl [001] surface, Mn2CoAl/GaAs heterostructures, Mn2CoAl/Ag/Mn2CoAl current-perpendicular-to-plane spin valves, MgO/Mn2CoAl/Pd trilayers, and various types of Mn2CoAl films (with MgAl2O4, GaAs, MgO, and thermally oxidized Si substrates) are reviewed in details. A new spin injection scheme based on Mn2CoAl and normal semiconductors is also summarized in this mini-review.
Author contributions
The author confirms being the sole contributor of this work and has approved it for publication.
Acknowledgments
YY is grateful for support from the key project of education planning supported by the Chongqing municipal education commission (No. 2021-GX-013) and the Basic Research and Frontier Exploration Project of Chongqing Municipality (cstc2018jcyjAX0820).
Conflict of interest
The author declares that the research was conducted in the absence of any commercial or financial relationships that could be construed as a potential conflict of interest.
Publisher’s note
All claims expressed in this article are solely those of the authors and do not necessarily represent those of their affiliated organizations, or those of the publisher, the editors and the reviewers. Any product that may be evaluated in this article, or claim that may be made by its manufacturer, is not guaranteed or endorsed by the publisher.
References
Arima, K., Kuroda, F., Yamada, S., Fukushima, T., Oguchi, T., and Hamaya, K. (2018). Anomalous Hall conductivity and electronic structures of Si-substituted Mn2CoAl epitaxial films. Phys. Rev. B 97 (5), 054427. doi:10.1103/physrevb.97.054427
Ashton, M., Gluhovic, D., Sinnott, S. B., Guo, J., Stewart, D. A., and Hennig, R. G. (2017). Two-dimensional intrinsic half-metals with large spin gaps. Nano Lett. 17 (9), 5251–5257. doi:10.1021/acs.nanolett.7b01367
Bainsla, L., Mallick, A., Raja, M., Nigam, A., Varaprasad, B., Takahashi, Y., et al. (2015). Spin gapless semiconducting behavior in equiatomic quaternary CoFeMnSi Heusler alloy. Phys. Rev. B 91, 104408. doi:10.1103/physrevb.91.104408
Chen, P., Gao, C., Chen, G., Mi, K., Liu, M., Zhang, P., et al. (2018). The low-temperature transport properties of Heusler alloy Mn2CoAl. Appl. Phys. Lett. 113 (12), 122402. doi:10.1063/1.5046396
Chen, X. R., Zhong, M. M., Feng, Y., Zhou, Y., Yuan, H. K., and Chen, H. (2015). Structural, electronic, elastic, and thermodynamic properties of the spin-gapless semiconducting Mn2CoAl inverse Heusler alloy under pressure. Phys. Status Solidi B 252 (12), 2830–2839. doi:10.1002/pssb.201552389
Deng, Y. X., Chen, S. Z., Zeng, Y., Feng, Y., Zhou, W. X., Tang, L. M., et al. (2018). Spin gapless semiconductor and half-metal properties in magnetic penta-hexa-graphene nanotubes. Org. Electron. 63, 310–317. doi:10.1016/j.orgel.2018.09.046
Deng, Y. X., Chen, S. Z., Zeng, Y., Feng, Y., Zhou, W. X., Tang, L. M., et al. (2018). Spin gapless semiconductor and half-metal properties in magnetic penta-hexa-graphene nanotubes. Org. Electron. 63, 310–317. doi:10.1016/j.orgel.2018.09.046
Ding, G., Wang, J., Chen, H., Zhang, X., and Wang, X. (2022). Investigation of nodal line spin-gapless semiconductors using first-principles calculations. J. Mat. Chem. C Mat. 10, 6530–6545. doi:10.1039/d2tc00305h
Ding, Y., and Wang, Y. (2015). Structural, electronic, and magnetic properties of adatom adsorptions on black and blue phosphorene: A first-principles study. J. Phys. Chem. C 119 (19), 10610–10622. doi:10.1021/jp5114152
Dowben, P. A., and Skomski, R. (2004). Are half-metallic ferromagnets half metals? J. Appl. Phys. 95 (11), 7453–7458. doi:10.1063/1.1682911
Fang, C. M., De Wijs, G. A., and De Groot, R. A. (2002). Spin-polarization in half-metals (invited). J. Appl. Phys. 91 (10), 8340–8344. doi:10.1063/1.1452238
Feng, Y., Tian, C. L., Yuan, H. K., Kuang, A. L., and Chen, H. (2015). The effect of disorder on the electronic and magnetic properties of Mn2CoAl/GaAs heterostructures. J. Phys. D. Appl. Phys. 48 (44), 445003. doi:10.1088/0022-3727/48/44/445003
Feng, Y., Zhou, T., Chen, X., Yuan, H., and Chen, H. (2015). Thermodynamic stability, magnetism and half metallicity of Mn2CoAl/GaAs (0 0 1) interface. J. Phys. D. Appl. Phys. 48 (28), 285302. doi:10.1088/0022-3727/48/28/285302
Galanakis, I., Özdoğan, K., Şaşıoğlu, E., and Blügel, S. (2014). Conditions for spin-gapless semiconducting behavior in Mn2CoAl inverse Heusler compound. J. Appl. Phys. 115 (9), 093908. doi:10.1063/1.4867917
Galanakis, I., Özdoğan, K., and Şaşıoğlu, E. (2016). Spin-filter and spin-gapless semiconductors: The case of Heusler compounds. AIP Adv. 6 (5), 055606. doi:10.1063/1.4943761
Gao, G., Ding, G., Li, J., Yao, K., Wu, M., and Qian, M. (2016). Monolayer MXenes: Promising half-metals and spin gapless semiconductors. Nanoscale 8 (16), 8986–8994. doi:10.1039/c6nr01333c
Gao, Q., Xie, H. H., Li, L., Lei, G., Deng, J. B., and Hu, X. R. (2015). First-principle study on some new spin-gapless semiconductors: The Zr-based quaternary Heusler alloys. Superlattices Microstruct. 85, 536–542. doi:10.1016/j.spmi.2015.05.049
Guan, J., Chen, W., Li, Y., Yu, G., Shi, Z., Huang, X., et al. (2013). An effective approach to achieve a spin gapless semiconductor–half-metal–metal transition in zigzag graphene nanoribbons: Attaching A floating induced dipole field via π–π interactions. Adv. Funct. Mat. 23 (12), 1507–1518. doi:10.1002/adfm.201201677
Guo, S. D., Mu, W. Q., Zhu, Y. T., and Chen, X. Q. (2020). Coexistence of intrinsic piezoelectricity and ferromagnetism induced by small biaxial strain in septuple-atomic-layer VSi 2 P 4. Phys. Chem. Chem. Phys. 22 (48), 28359–28364. doi:10.1039/d0cp05273f
Han, Y., Chen, Z., Kuang, M., Liu, Z., Wang, X., and Wang, X. (2019). 171 scandium-based full heusler compounds: A comprehensive study of competition between XA and L21 atomic ordering. Results Phys. 12, 435–446. doi:10.1016/j.rinp.2018.11.079
Han, Y., Wu, M., Feng, Y., Cheng, Z., Lin, T., Yang, T., et al. (2019). Competition between cubic and tetragonal phases in all-d-metal heusler alloys, X2−x Mn1+x V (X = Pd, Ni, Pt, Ag, Au, Ir, Co; x = 1, 0): A new potential direction of the heusler family. IUCrJ 6 (3), 465–472. doi:10.1107/s2052252519004007
He, J., Li, X., Lyu, P., and Nachtigall, P. (2017). Near-room-temperature chern insulator and Dirac spin-gapless semiconductor: Nickel chloride monolayer. Nanoscale 9 (6), 2246–2252. doi:10.1039/c6nr08522a
Huang, H. M., Cao, M. L., Jiang, Z. Y., Xiong, Y. C., Zhang, X., Luo, S. J., et al. (2019). High spin polarization in formamidinium transition metal iodides: First principles prediction of novel half-metals and spin gapless semiconductors. Phys. Chem. Chem. Phys. 21 (29), 16213–16222. doi:10.1039/c9cp00958b
Huang, H. M., Cao, M. L., Jiang, Z. Y., Xiong, Y. C., Zhang, X., Luo, S. J., et al. (2019). High spin polarization in formamidinium transition metal iodides: First principles prediction of novel half-metals and spin gapless semiconductors. Phys. Chem. Chem. Phys. 21 (29), 16213–16222. doi:10.1039/c9cp00958b
Jamer, M. E., Assaf, B. A., Devakul, T., and Heiman, D. (2013). Magnetic and transport properties of Mn2CoAl oriented films. Appl. Phys. Lett. 103 (14), 142403. doi:10.1063/1.4823601
Jamer, M. E., Assaf, B. A., Sterbinsky, G. E., Arena, D. A., and Heiman, D. (2014). Atomic moments in Mn2CoAl thin films analyzed by X-ray magnetic circular dichroism. J. Appl. Phys. 116 (21), 213914. doi:10.1063/1.4903771
Ji, Y., Tan, X., Yue, X., Sun, Y., Wang, Y., Liang, H., et al. (2022). Strain-induced spin-gapless semiconductors and pure thermal spin-current in magnetic black arsenic-phosphorus monolayers. Phys. Chem. Chem. Phys. 24 (22), 13897–13904. doi:10.1039/d2cp01108e
Li, H., Hayashi, K., Nagashima, Y., Yoshioka, S., Dong, J., Li, J. F., et al. (2021). Effects of disorder on the electronic structure and thermoelectric properties of an inverse full-heusler Mn2CoAl alloy. Chem. Mat. 33 (7), 2543–2547. doi:10.1021/acs.chemmater.0c04902
Li, J., and Jin, Y. (2013). Half-metallicity of the inverse heusler alloy Mn2CoAl (0 0 1) surface: A first-principles study. Appl. Surf. Sci. 283, 876–880. doi:10.1016/j.apsusc.2013.07.036
Li, X., and Yang, J. (2013). Bipolar magnetic materials for electrical manipulation of spin-polarization orientation. Phys. Chem. Chem. Phys. 15 (38), 15793–15801. doi:10.1039/c3cp52623b
Li, Y., Zhou, Z., Shen, P., and Chen, Z. (2009). Spin gapless semiconductor− metal− half-metal properties in nitrogen-doped zigzag graphene nanoribbons. ACS Nano 3 (7), 1952–1958. doi:10.1021/nn9003428
Liu, G. D., Dai, X. F., Liu, H. Y., Chen, J. L., Li, Y. X., Xiao, G., et al. (2008). Mn2CoZ(Z=Al, Ga, In, Si, Ge, Sn, Sb)compounds: Structural, electronic, and magnetic properties. Phys. Rev. B 77 (1), 014424. doi:10.1103/physrevb.77.014424
Liu, J. H., Kan, X., Amin, B., Gan, L. Y., and Zhao, Y. (2017). Theoretical exploration of the potential applications of Sc-based MXenes. Phys. Chem. Chem. Phys. 19 (48), 32253–32261. doi:10.1039/c7cp06224a
Ludbrook, B. M., Dubuis, G., Puichaud, A. H., Ruck, B. J., and Granville, S. (2017). Nucleation and annihilation of skyrmions in Mn2CoAl observed through the topological Hall effect. Sci. Rep. 7 (1), 13620. doi:10.1038/s41598-017-13211-8
Meinert, M., Schmalhorst, J. M., and Reiss, G. (2011). Exchange interactions and Curie temperatures of Mn2CoZ compounds. J. Phys. Condens. Matter 23 (11), 116005. doi:10.1088/0953-8984/23/11/116005
Müller, G. M., Walowski, J., Djordjevic, M., Miao, G. X., Gupta, A., Ramos, A. V., et al. (2009). Spin polarization in half-metals probed by femtosecond spin excitation. Nat. Mat. 8 (1), 56–61. doi:10.1038/nmat2341
Nadeem, M., Hamilton, A. R., Fuhrer, M. S., and Wang, X. (2020). Quantum anomalous Hall effect in magnetic doped topological insulators and ferromagnetic spin-gapless semiconductors—a perspective review. Small 16 (42), 1904322. doi:10.1002/smll.201904322
Ouardi, S., Fecher, G. H., Felser, C., and Kübler, J. (2019). Erratum: Realization of spin gapless semiconductors: The heusler compound Mn2CoAl [phys. Rev. Lett. 110, 100401 (2013)]. Phys. Rev. Lett. 122 (5), 059901. doi:10.1103/physrevlett.122.059901
Ouardi, S., Fecher, G. H., Felser, C., and Kübler, J. (2013). Realization of spin gapless semiconductors: The heusler CompoundMn2CoAl. Phys. Rev. Lett. 110 (10), 100401. doi:10.1103/physrevlett.110.100401
Ouardi, S., Fecher, G. H., Felser, C., and Kübler, J. (2013). Realization of spin gapless semiconductors: The heusler CompoundMn2CoAl. Phys. Rev. Lett. 110 (10), 100401. doi:10.1103/physrevlett.110.100401
Rani, D., Enamullah, , Bainsla, L., Suresh, K., and Alam, A. (2019). Spin-gapless semiconducting nature of Co-rich Co1+xFe1−xCrGa. Phys. Rev. B 99, 104429. doi:10.1103/physrevb.99.104429
Rasool, M. N., Hussain, A., Javed, A., Khan, M. A., and Iqbal, F. (2016). Structural stability, electronic and magnetic behaviour of spin-polarized YCoVZ (Z= Si, Ge) and YCoTiZ (Z= Si, Ge) Heusler alloys. Mater. Chem. Phys. 183, 524–533. doi:10.1016/j.matchemphys.2016.09.011
Wang, A., Peng, J., Ren, N., Ding, L., Yu, X., Wang, Z., et al. (2021). Spin-gapless states in two-dimensional molecular ferromagnet Fe2 (TCNQ) 2. J. Phys. Chem. Lett. 12 (33), 7921–7927. doi:10.1021/acs.jpclett.1c01869
Wang, A., Zhang, X., Feng, Y., and Zhao, M. (2017). Chern insulator and Chern half-metal states in the two-dimensional spin-gapless semiconductor Mn2C6S12. J. Phys. Chem. Lett. 8 (16), 3770–3775. doi:10.1021/acs.jpclett.7b01187
Wang, X., Cheng, Z., Wang, J., and Liu, G. (2016). A full spectrum of spintronic properties demonstrated by a C1 b-type Heusler compound Mn 2 Sn subjected to strain engineering. J. Mat. Chem. C Mat. 4 (36), 8535–8544. doi:10.1039/c6tc02526a
Wang, X., Cheng, Z., Wang, J., Wang, X. L., and Liu, G. (2016). Recent advances in the Heusler based spin-gapless semiconductors. J. Mat. Chem. C Mat. 4 (30), 7176–7192. doi:10.1039/c6tc01343k
Wang, X., Cheng, Z., and Wang, W. (2017). L21 and XA ordering competition in hafnium-based full-heusler alloys Hf2VZ (Z= Al, Ga, In, Tl, Si, Ge, Sn, Pb). Materials 10 (10), 1200. doi:10.3390/ma10101200
Wang, X., Cheng, Z., Yuan, H., and Khenata, R. (2017). L2 1 and XA ordering competition in titanium-based full-Heusler alloys. J. Mat. Chem. C Mat. 5 (44), 11559–11564. doi:10.1039/c7tc03909c
Wang, X. H., Yang, A. J., Koratkar, N., Chu, J. F., Lv, P. L., Rong, M. Z., et al. (2018). Effects of adatom and gas molecule adsorption on the physical properties of tellurene: A first principles investigation. Phys. Chem. Chem. Phys. 20 (6), 4058–4066. doi:10.1039/c7cp07906k
Wang, X. L. (2017). Dirac spin-gapless semiconductors: Promising platforms for massless and dissipationless spintronics and new (quantum) anomalous spin Hall effects. Natl. Sci. Rev. 4 (2), 252–257. doi:10.1093/nsr/nww069
Wang, X. L. (2008). Proposal for a new class of materials: Spin gapless semiconductors. Phys. Rev. Lett. 100 (15), 156404. doi:10.1103/physrevlett.100.156404
Wei, M. S., Cui, Z., Ruan, X., Zhou, Q. W., Fu, X. Y., Liu, Z. Y., et al. (2018). Interface characterization of current-perpendicular-to-plane spin valves based on spin gapless semiconductor Mn2CoAl. Appl. Sci. 8 (8), 1348. doi:10.3390/app8081348
Wu, D. D., Ji, Y. T., Du, G. F., Yue, X. Y., Wang, Y. Y., Li, Q. J., et al. (2022). Spin-gapless semiconducting Cl-intercalated phosphorene bilayer: A perfect candidate material to identify its ferroelectric states by spin-seebeck currents. J. Mat. Chem. C Mat. 10 (8), 3188–3195. doi:10.1039/d1tc05932g
Wu, M., Han, Y., Bouhemadou, A., Cheng, Z., Khenata, R., Kuang, M., et al. (2019). Site preference and tetragonal distortion in palladium-rich Heusler alloys. IUCrJ 6 (2), 218–225. doi:10.1107/s2052252518017578
Wu, M., Zhou, F., Khenata, R., Kuang, M., and Wang, X. (2020). Phase transition and electronic structures of all-d-metal Heusler-type X2MnTi compounds (X= Pd, Pt, Ag, Au, Cu, and Ni). Front. Chem. 8, 546947. doi:10.3389/fchem.2020.546947
Wu, X., Feng, Y., Li, S., Zhang, B., and Gao, G. (2020). 2D Mn2C6Se12 and Mn2C6S6Se6: Intrinsic room-temperature Dirac spin gapless semiconductors and perfect spin transport properties. J. Phys. Chem. C 124 (29), 16127–16135. doi:10.1021/acs.jpcc.0c04786
Xing, N., Li, H., Dong, J., Long, R., and Zhang, C. (2008). First-principle prediction of half-metallic ferrimagnetism of the Heusler alloys Mn2CoZ (Z= Al, Ga, Si, Ge) with a high-ordered structure. Comput. Mater. Sci. 42 (4), 600–605. doi:10.1016/j.commatsci.2007.09.007
Xu, G. Z., Du, Y., Zhang, X. M., Zhang, H. G., Liu, E. K., Wang, W. H., et al. (2014). Magneto-transport properties of oriented Mn2CoAl films sputtered on thermally oxidized Si substrates. Appl. Phys. Lett. 104 (24), 242408. doi:10.1063/1.4884203
Xu, X. D., Chen, Z. X., Sakuraba, Y., Perumal, A., Masuda, K., Kumara, L. S. R., et al. (2019). Microstructure, magnetic and transport properties of a Mn2CoAl Heusler compound. Acta Mater. 176, 33–42. doi:10.1016/j.actamat.2019.06.047
Yang, T., Cheng, Z., Wang, X., and Wang, X. L. (2021). Nodal ring spin gapless semiconductor: New member of spintronic materials. J. Adv. Res. 28, 43–49. doi:10.1016/j.jare.2020.06.016
Yue, Z., Li, Z., Sang, L., and Wang, X. (2020). Spin-gapless semiconductors. Small 16 (31), 1905155. doi:10.1002/smll.201905155
Keywords: Mn2CoAl, spin-gapless materials, Heusler, DFT, density functional theory, film, substrate system
Citation: Yang Y (2022) Mini-review of interesting properties in Mn2CoAl bulk and films. Front. Chem. 10:1054337. doi: 10.3389/fchem.2022.1054337
Received: 26 September 2022; Accepted: 06 October 2022;
Published: 19 October 2022.
Edited by:
Guangzhao Wang, Yangtze Normal University, ChinaReviewed by:
Xiaoming Zhang, Hebei University of Technology, ChinaTie Yang, Southwest University, China
Copyright © 2022 Yang. This is an open-access article distributed under the terms of the Creative Commons Attribution License (CC BY). The use, distribution or reproduction in other forums is permitted, provided the original author(s) and the copyright owner(s) are credited and that the original publication in this journal is cited, in accordance with accepted academic practice. No use, distribution or reproduction is permitted which does not comply with these terms.
*Correspondence: Ying Yang, cGh5c2ljc3l5QDEyNi5jb20=