- 1School of Chemistry and Chemical Engineering, Mianyang Teacher’s College, Mianyang, China
- 2Dean’s Office, Mianyang Teacher’s College, Mianyang, China
Six flavonoid derivatives were synthesized and tested for anti-α-glucosidase activities. All derivatives were confirmed using NMR and HRMS and exhibited excellent inhibitory effects on α-glucosidase. Derivative four exhibited the highest anti-α-glucosidase activity (IC50: 15.71 ± 0.21 μM). Structure-activity relationship results showed that bromine group would be the most beneficial group to anti-α-glucosidase activity. Inhibitory mechnism and inhibition kinetics results showed derivative four was a reversible and mixed-type inhibitor. Molecular docking revealed that derivative four was tightly bind to the amino acid residues of active pocket of α-glucosidase and formed hydrogen bond, π-π stacking, and Pi-Donor hydrogen with α-glucosidase. Moreover, the physicochemical parameters of all derivatives were assessed using SwissADME software. This results also showed that the hybridization of flavonoid and phenylpropionic acid would be a useful strategy for the development of α-glucosidase inhibitors.
1 Introduction
Diabetes mellitus is reported as a common chronic metabolic disorder with hyperglycemia. This hyperglycemia can cause microvascular complications such as cardiovascular, renal, and neurological problems (Forouhi and Wareham, 2014; Proenca et al., 2021). Numerous researches reveal that the hydrolysis of carbohydrates is the major inducement of hyperglycemia (Kokil et al., 2015; Kousaxidis et al., 2020). α-Glucosidase located in the small intestine is one important catalytic hydrolase, which can hydrolyze carbohydrates into absorbable glucose. The excess absorbed glucose causes postprandial hyperglycemia, resulting in diabetes (Ali et al., 2017; Proença et al., 2017; Tsoutsouki et al., 2020). Thence, inhibiting α-glucosidase activity might be an effective strategy for controlling postprandial hyperglycemia (Imran et al., 2015; Rasouli et al., 2017; Sohretoglu et al., 2018). Although lots of α-glucosidase inhibitors have been developed, only a few have been used as clinical drugs for the treatment of diabetes, including acarbose, voglibose, and miglitol (Figure 1). But they are reported to have some adverse reactions during the use (Santos et al., 2018; Hedrington and Davis, 2019). This encourages researchers to find more effective and safety α-glucosidase inhibitors.
Natural products are the important medicinal resources, and many clinical drugs are generated directly or indirectly from natural products (Şőhretoğlu and Sari, 2020; Proenca et al., 2021; Mo et al., 2022). Flavonoids present abundantly in natural products that are a class of compounds with biological and pharmacological activities (Dong et al., 2020; Qin et al., 2020; Wang et al., 2020). Besides, antioxidants, antibacterial, anti-inflammatory, anti-tumor, etc., their anti-α-glucosidase and anti-diabetic properties have gotten more and more attention recently (Amador et al., 2020; Liu et al., 2020; Tang et al., 2020). Now, lots of synthesized and isolated flavonoids were obtained as α-glucosidase inhibitors (Jia et al., 2019; Lu et al., 2020; Oueslati et al., 2020; Zhu et al., 2020).
On the other hand, the esterification modification of natural products is effective strategies to obtain better active compounds or ester prodrugs. Previous works revealed that the esterification modification of coumarin and honokiol produced a series of compounds with better anti-α-glucosidase activity (Sheng et al., 2018; Hu et al., 2021). Thence, in this work, flavonoid skeleton was modified with esterification by substituted phenylpropionic acid, followed by the screening of anti-α-glucosidase activity.
2 Results and discussion
2.1 Chemistry
All flavonoid ester derivatives (1∼6) were synthesized according to route outlined in Scheme 1. p-Hydroxybenzaldehyde and 2-Hydroxyacetophenone underwent Claisen-Schmidt condensation to yield 4,2′-dihydroxychalcone, followed by the cyclization reaction to produce 4′-hydroxyflavonoid which reacted with substituted phenylpropionic acid to generate flavonoid derivatives (1∼6), respectively. All synthesized flavonoid ester derivatives were identified by 1H NMR, 13C NMR and HRMS.

SCHEME 1. Synthesis of flavonoid derivatives 1∼6. Reagents and condition: (A) Piperdine, reflux, 160°C; (B) DMSO, I2, 100°C; (C) Substituted phenylpropionic acid, DMSO, EDCI, DCM, rt.
2.2 α-Glucosidase inhibition assay and SAR analysis
All synthesized six flavonoids were screened for inhibitory activity against α-glucosidase and the results were listed in Table 1. The six flavonoid derivatives existed potential anti-α-glucosidase activity with IC50 range of 15.71 ± 0.21–42.06 ± 0.08 μM, which was stronger than that of acarbose (658.26 ± 11.48 μM). Among them, compound 4 showed the strongest inhibitory activity (IC50 = 15.71 ± 0.21 μM). The results showed that flavonoid derivatives could be used as potential α-glucosidase inhibitors. That also was said that hybridization of flavonoid skeleton and phenylpropionic acid would be an effective strategy to discover anti-α-glucosidase inhibitors.
Compared to acarbose, *p < 0.05.
In order to better guide future derivatization, the structure-activity relationship (SAR) was analyzed. For all six derivatives, compound 1 with no substituent group at phenylpropionic acid fraction was selected as template molecule, showing an IC50 value of 42.06 ± 0.08 μM. Introducing methyl group (compound 2, IC50 = 21.55 ± 0.13 μM), chlorine group (compound 3, IC50 = 31.09 ± 0.10 μM), bromine group (compound 4, IC50 = 15.71 ± 0.21 μM), fluorine group (compound 5, IC50 = 19.66 ± 0.04 μM), and trifluoromethyl group (compound 6, IC50 = 27.54 ± 0.02 μM) on phenylpropionic acid fraction caused effective increase in inhibition activity. Thence, introducing substituents would enhance their anti-α-glucosidase activity and the sequence of substitute group was bromine, fluorine, methyl, trifluoromethyl, chlorine group, and hydrogen. (Figure 2).
2.3 Inhibitory mechanism and type assay
To study the inhibitory mechanisms of all derivatives on α-glucosidase, enzyme inhibitory mechnism was detected using compound 4 with the strongest inhibitory. Figure 3A illustrated the plots of enzyme activity vs. enzyme concentration. It could be seen that the plots with compound 4 (0–25 μM) all passed the origin, revealing compound 4 as a reversible inhibitor.
The kinetic type was also studied using Lineweaver-Burk plots. As shown in the Lineweaver-Burk plots of enzyme activity vs. substrate concentration (Figure 3B), all plots with compound 4 (0–25 μM) intersected at the second quadrant, indicating a mix-type inhibition.
2.4 Molecular docking
Molecular docking of compound 4 with α-glucosidase was simulated using SYBYL software, and the binding interactions were analyzed. As shown in Figures 4A–3B, compound 4 bind reliably with the active pocket, the flavonoid section of compound 4 located at entrance of the active pocket, and the bromophenylpropionic acid section located at the interior. Figures 3C,D were the detailed interactions in 3D view and 2D view, respectively. It was seen that the carbonyl moiety formed a hydrogen bond with Arg 312 (2.0 Å), benzene ring of bromophenylpropionic acid section formed a π-π stacking with Phe157 and Pi-Donor hydrogen bond with His239. Moreover, compound 4 also formed hydrophobic interactions with Pro240, His245, and His279.
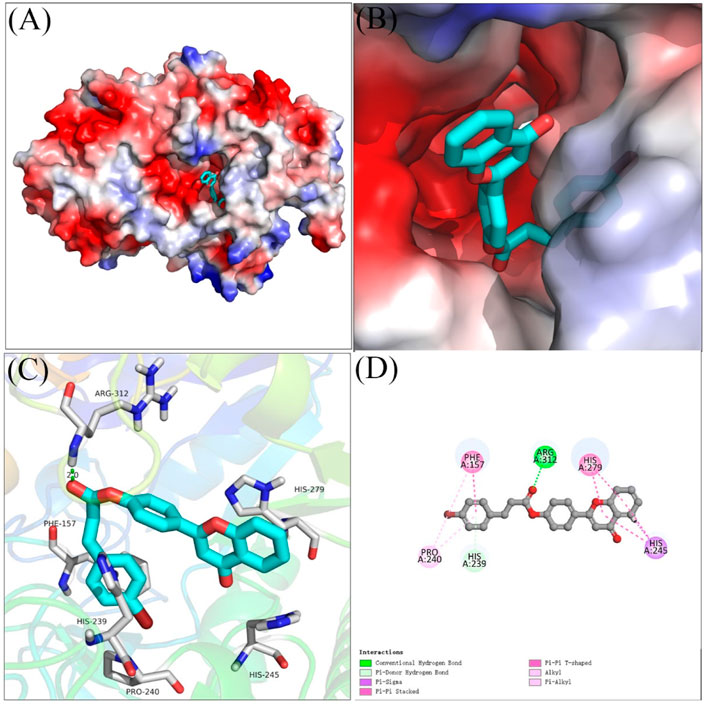
FIGURE 4. The molecular docking of compound 4 with α-glucosidase. (A,B) compound 4 in the active pocket. (C,D) detailed binding between compound 4 and enzyem.
2.5 Physicochemical parameters
The physicochemical parameters of all derivatives were analyzed using SwissADME software. The results showed (Table 2) that all derivatives presented favourable drug-likeness profile. The molecular weight, RB, HBA, HBD, and TPSA of derivatives basically met the standard.
3 Conclusion
In this study, we synthesized six flavonoid derivatives and tested their anti-α-glucosidase activities. All derivatives exhibited excellent inhibitory effects on α-glucosidase. Among them, derivative four exhibited the highest anti-α-glucosidase activity (IC50: 15.71 ± 0.21 μM). Moreover, bromine group was the optimal substituent for activity. Inhibitory mechnism and inhibition kinetics results showed derivative four was a reversible and mixed-type inhibitor. Molecular docking revealed that derivative four was tightly bind to the amino acid residues of active pocket of α-glucosidase. Thence, the hybridization of flavonoid and phenylpropionic acid would be a useful strategy for the development of α-glucosidase inhibitors. In addition, derivative four would be used as a lead compound to develop hypoglycemic drugs.
4 Experimental
4.1 Materials and methods
α-Glucosidase from Saccharomyces cerevisiae (EC 3.2.1.20) and p-Nitrophenyl-α-D-galactopyranoside (p-NPG) were purchased from Sigma-Aldrich. All additional reagents and solvents were readily obtained from a commercial source. NMR spectra were obtained on 500 MHz equipment in CDCl3. High-resolution mass spectral (HRMS) data was recorded on Apex II using the ESI technique.
4.2 General procedure for the synthesis of flavonoid derivatives 1–6
2-hydroxy acetophenone (0.01 M) and appropriate benzaldehydes (0.01 M) were added into piperidine (10 ml) and maintained at 160 C. After reaction was completed, the mixture was treated with ice-cold water and adjusted pH. Then this obtained precipitate was recrystallized in methanol to give 4,2′-dihydroxychalcone. Then, 4,2′-dihydroxychalcone (0.015 M) and iodine (0.015 M) were added into dimethyl sulfoxide, and stirred for 60 min at 140 C. After treated with 20% aqueous sodium thiosulfate, the mixture was extracted with DCM, followed by washing with brine, concentrating in a rotary evaporator, and subsequent recrystallization to obtain 4′-hydroxyflavonoid. 4′-hydroxyflavonoid (0.21 mmol), substituted phenylpropionic acid (0.32 mmol), DMAP (0.42 mmol) and EDCI (0.42 mmol) were added into 10 ml DCM and reacted at room temperature. Then the mixture was quenched by water, extracted with DCM, washed with brine, dried by MgSO4, removed solvent under vacuum, and subsequently purified using column chromatography to yield the corresponding flavonoid derivatives 1 ∼ 6. All 1H NMR, 13C NMR and HRMS data were summarized into SUPPORTING INFORMATION.
4.3 A-glucosidase inhibition and kinetics assay
The α-glucosidase inhibitory activity of compounds (1 ∼ 6) was detected as described in previous reports (Adisakwattana et al., 2009; Adisakwattana et al., 2013; Zhang et al., 2022). 10 μl α-glucosidase solution, 10 μl compound were added into 80 μl phosphate buffer, and the mixture was incubated for 10 min. Then, 100 μl p-NPG solution was added into the mixture, followed by the absorbance change detection at 405 nm. The inhibition rate (%) = [(OD1 - OD0)/OD0] × 100%, where OD1 and OD0 were the absorbance of tested compound and blank, respectively. The IC50 value was calculated from the plot of inhibition rate vs. compound concentration. Acarbose was used as a positive sample. All samples were repeated four times. The enzyme inhibitory mechnism and kinetic type were also determined according to previous reported reports (Adisakwattana et al., 2004; Song et al., 2016).
4.4 Molecular docking
Molecular docking of compound 4 with α-glucosidase was simulated using SYBYL software according to previous researches (Li et al., 2016; Xu et al., 2019). Compound 4 was constructed and energy minimized using software own programs. The α-glucosidase were prepared by hydrogenation and disability rehabilitation. Then, the docking between compound 4 and α-glucosidase was operated in the default format.
4.5 Statistical analysis
Data was presented as mean ± SD. One-way ANOVA was used to analyze the difference between groups. p < 0.05 was considered significant.
Data availability statement
The datasets presented in this study can be found in online repositories. The names of the repository/repositories and accession number(s) can be found in the article/Supplementary Material.
Author contributions
HZ carried out the investigation, and XZ was responsible for project design.
Funding
This work was financially supported by the Start Fund Project of Mianyang Teacher’s College (Nos. QD 2020A01).
Conflict of interest
The authors declare that the research was conducted in the absence of any commercial or financial relationships that could be construed as a potential conflict of interest.
Publisher’s note
All claims expressed in this article are solely those of the authors and do not necessarily represent those of their affiliated organizations, or those of the publisher, the editors and the reviewers. Any product that may be evaluated in this article, or claim that may be made by its manufacturer, is not guaranteed or endorsed by the publisher.
Supplementary material
The Supplementary Material for this article can be found online at: https://www.frontiersin.org/articles/10.3389/fchem.2022.1041328/full#supplementary-material
References
Adisakwattana, S., Chantarasinlapin, P., Thammarat, H., and Yibchok-Anun, S. (2009). A series of cinnamic acid derivatives and their inhibitory activity on intestinal alpha-glucosidase. J. Enzyme Inhib. Med. Chem. 24, 1194–1200. doi:10.1080/14756360902779326
Adisakwattana, S., Pongsuwan, J., Wungcharoen, C., and Yibchok-anun, S. (2013). In vitro effects of cinnamic acid derivatives on protein tyrosine phosphatase 1B. J. Enzyme Inhib. Med. Chem. 28, 1067–1072. doi:10.3109/14756366.2012.715286
Adisakwattana, S., Sookkongwaree, K., Roengsumran, S., Petsom, A., Ngamrojnavanich, N., Chavasiri, W., et al. (2004). Structure-activity relationships of trans-cinnamic acid derivatives on alpha-glucosidase inhibition. Bioorg. Med. Chem. Lett. 14, 2893–2896. doi:10.1016/j.bmcl.2004.03.037
Ali, F., Khan, K. M., Salar, U., Taha, M., Ismail, N. H., Wadood, A., et al. (2017). Hydrazinyl arylthiazole based pyridine scaffolds: Synthesis, structural characterization, in vitro α-glucosidase inhibitory activity, and in silico studies. Eur. J. Med. Chem. 138, 255–272. doi:10.1016/j.ejmech.2017.06.041
Amador, S., Nieto-Camacho, A., Ramírez-Apan, M. T., Martínez, M., and Maldonado, E. (2020). Cytotoxic, anti-inflammatory, and alpha-glucosidase inhibitory effects of flavonoids from Lippia graveolens (Mexican oregano). Med. Chem. Res. 29, 1497–1506. doi:10.1007/s00044-020-02569-6
Dong, Y., Yu, N., Li, X., Zhang, B., Xing, Y., Zhuang, C., et al. (2020). Dietary 5, 6, 7-Trihydroxy-flavonoid aglycones and 1-deoxynojirimycin synergistically inhibit the recombinant maltase-glucoamylase subunit of alpha-glucosidase and lower postprandial blood glucose. J. Agric. Food Chem. 68, 8774–8787. doi:10.1021/acs.jafc.0c01668
Forouhi, N. G., and Wareham, N. J. (2014). Epidemiology of diabetes. Med. (Abingdon) 42 (12), 698–702. doi:10.1016/j.mpmed.2014.09.007
Hedrington, M. S., and Davis, S. N. (2019). Considerations when using alpha-glucosidase inhibitors in the treatment of type 2 diabetes. Expert Opin. Pharmacother. 20, 2229–2235. doi:10.1080/14656566.2019.1672660
Hu, C., Wang, W., Na, Y., Kang, Y., Lin, J., Wu, P., et al. (2021). Novel cinnamic acid magnolol derivatives as potent α-glucosidase and α-amylase inhibitors: Synthesis, in vitro and in silico studies, Bioorg. Chem 116, 105291.
Imran, S., Taha, M., Ismail, N. H., Kashif, S. M., Rahim, F., Jamil, W., et al. (2015). Synthesis of novel flavone hydrazones: In-vitro evaluation of α-glucosidase inhibition, QSAR analysis and docking studies. Eur. J. Med. Chem. 105, 156–170. doi:10.1016/j.ejmech.2015.10.017
Jia, Y., Ma, Y., Cheng, G., Zhang, Y., and Cai, S. (2019). Comparative study of dietary flavonoids with different structures as alpha-glucosidase inhibitors and insulin sensitizers. J. Agric. Food Chem. 6, 10521–10533. doi:10.1021/acs.jafc.9b04943
Kokil, G. R., Veedu, R. N., Ramm, G. A., Prins, J. B., and Parekh, H. S. (2015). Type 2 diabetes mellitus: Limitations of conventional therapies and intervention with nucleic acid-based therapeutics. Chem. Rev. 115 (11), 4719–4743. doi:10.1021/cr5002832
Kousaxidis, A., Petrou, A., Lavrentaki, V., Fesatidou, M., Nicolaou, I., and Geronikaki, A. (2020). Aldose reductase and protein tyrosine phosphatase 1B inhibitors as a promising therapeutic approach for diabetes mellitus. Eur. J. Med. Chem. 207, 112742. doi:10.1016/j.ejmech.2020.112742
Li, Y., Yuan, X., Rong, X., Gao, Y., Qiu, Z., Zhang, Z., et al. (2016). Design, synthesis and biological evaluation of a hybrid compound of berberine and magnolol for improvement of glucose and lipid metabolism. RSC Adv. 6, 81924–81931. doi:10.1039/c6ra15100k
Liu, J., Kong, Y., Miao, J., Mei, X., Wu, S., Yan, Y., et al. (2020). Spectroscopy and molecular docking analysis reveal structural specificity of flavonoids in the inhibition of alpha-glucosidase activity. Int. J. Biol. Macromol. 152, 981–989. doi:10.1016/j.ijbiomac.2019.10.184
Lu, H., Qi, Y., Zhao, Y., and Jin, N. (2020). Effects of hydroxyl group on the interaction of carboxylated flavonoid derivatives with S. Cerevisiae alpha-glucosidase,. Curr. Comput. Aided. Drug Des. 16, 31–44. doi:10.2174/1573409914666181022142553
Mo, Q. G., Zhou, G., Zhu, W. D., Ge, L. L., and Wang, Y. W. (2022). Coumaroyl and feruloyl flavonoid glycosides from the male flowers of Ginkgo biloba L. and their inhibitory activity against alpha-glucosidase. Nat. Prod. Res. 36, 4365–4372. doi:10.1080/14786419.2021.1993216
Oueslati, M. H., Bouajila, J., Gurtat, A., Al-Gamdi, F., and Hichri, F. (2020). Cytotoxic, alpha-glucosidase, and antioxidant activities of flavonoid glycosides isolated from flowers of Lotus lanuginosus vent. (Fabaceae). Pharmacogn. Mag. 16, 22.
Proença, C., Freitas, M., Ribeiro, D., Oliveira, E. F., Sousa, J. L., Tomé, S. M., et al. (2017). α-Glucosidase inhibition by flavonoids: An in vitro and in silico structure-activity relationship study. J. Enzyme Inhib. Med. Chem. 32, 1216–1228. doi:10.1080/14756366.2017.1368503
Proenca, C., Ribeiro, D., Freitas, M., and Fernandes, E. (2021). Flavonoids as potential agents in the management of type 2 diabetes through the modulation of alpha-amylase and alpha-glucosidase activity: A review. Crit. Rev. Food Sci. Nutr. 62, 3137–3207. doi:10.1080/10408398.2020.1862755
Qin, Y., Chen, J., Li, C., Zhu, L., Zhu, X., Zhang, X., et al. (2020). Flavonoid glycosides from the fruits of Embelia ribes and their anti-oxidant and alpha-giucosidase inhibitory activities. J. Asian Nat. Prod. Res. 23, 724–730. doi:10.1080/10286020.2020.1776266
Rasouli, H., Hosseini-Ghazvini, S. M., Adibi, H., and Khodarahmi, R. (2017). Differential α-amylase/α-glucosidase inhibitory activities of plant-derived phenolic compounds: A virtual screening perspective for the treatment of obesity and diabetes. Food Funct. 8, 1942–1954. doi:10.1039/c7fo00220c
Santos, C. M. M., Freitas, M., and Fernandes, E. (2018). A comprehensive review on xanthone derivatives as alpha-glucosidase inhibitors. Eur. J. Med. Chem. 157, 1460–1479. doi:10.1016/j.ejmech.2018.07.073
Sheng, Z., Ge, S., Xu, X., Zhang, Y., Wu, P., Zhang, K., et al. (2018). Design, synthesis and evaluation of cinnamic acid ester derivatives as mushroom tyrosinase inhibitors. Med. Chem. Commun. 9, 853–861. doi:10.1039/c8md00099a
Sohretoglu, D., Sari, S., Barut, B., and Ozel, A. (2018). Discovery of potent alpha-glucosidase inhibitor flavonols: Insights into mechanism of action through inhibition kinetics and docking simulations. Bioorg. Chem. 79, 257–264. doi:10.1016/j.bioorg.2018.05.010
Şőhretoğlu, D., and Sari, S. (2020). Flavonoids as alpha-glucosidase inhibitors: Mechanistic approaches merged with enzyme kinetics and molecular modelling. Phytochem. Rev. 19, 1081–1092. doi:10.1007/s11101-019-09610-6
Song, Y. H., Kim, D. W., Curtis-Long, M. J., Park, C., Son, M., Kim, J. Y., et al. (2016). Cinnamic acid amides from Tribulus terrestris displaying uncompetitive alpha-glucosidase inhibition. Eur. J. Med. Chem. 114, 201–208. doi:10.1016/j.ejmech.2016.02.044
Tang, H., Huang, L., Sun, C., and Zhao, D. (2020). Exploring the structure-activity relationship and interaction mechanism of flavonoids and alpha-glucosidase based on experimental analysis and molecular docking studies. Food Funct. 4, 3332–3350. doi:10.1039/c9fo02806d
Tsoutsouki, J., Wunna, W., Chowdhury, A., and Chowdhury, T. A. (2020). Advances in the management of diabetes: Therapies for type 2 diabetes. Postgrad. Med. J. 96, 610–618. doi:10.1136/postgradmedj-2019-137404
Wang, H., Zhao, J., and Zhang, Y. (2020). The flavonoid-rich ethanolic extract from the green cocoon shell of silkworm has excellent antioxidation, glucosidase inhibition, and cell protective effects in vitro. Food Nutr. Res. 64, 1637. doi:10.29219/fnr.v64.1637
Xu, X. T., Deng, X. Y., Chen, J., Liang, Q. M., Zhang, K., Li, D. L., et al. (2019). Synthesis and biological evaluation of coumarin derivatives as alpha-glucosidase inhibitors. Eur. J. Med. Chem. 189, 112013. doi:10.1016/j.ejmech.2019.112013
Zhang, X., Zheng, Y. Y., Hu, C. M., Wu, X. Z., Lin, J., Xiong, Z., et al. (2022). Synthesis and biological evaluation of coumarin derivatives containing oxime ester as α-glucosidase inhibitors. Arab. J. Chem. 15, 104072. doi:10.1016/j.arabjc.2022.104072
Keywords: ɑ-glucosidase, inhibitor, synthesis, flavonoid, screen
Citation: Zhu H and Zhong X (2022) Synthesis of activity evaluation of flavonoid derivatives as ɑ-glucosidase inhibitors. Front. Chem. 10:1041328. doi: 10.3389/fchem.2022.1041328
Received: 10 September 2022; Accepted: 04 October 2022;
Published: 15 November 2022.
Edited by:
Xi Zheng, Rutgers, The State University of New Jersey, United StatesReviewed by:
Ren Qinggang, Guangdong University of Petrochemical Technology, ChinaJie Zheng, Shangrao Normal University, China
Copyright © 2022 Zhu and Zhong. This is an open-access article distributed under the terms of the Creative Commons Attribution License (CC BY). The use, distribution or reproduction in other forums is permitted, provided the original author(s) and the copyright owner(s) are credited and that the original publication in this journal is cited, in accordance with accepted academic practice. No use, distribution or reproduction is permitted which does not comply with these terms.
*Correspondence: Xin Zhong, zhuhua2006@163.com