- 1Department of Hepatobiliary and Pancreas Surgery, China-Japan Union Hospital of Jilin University, Changchun, China
- 2Laboratory Animal Center, College of Animal Science, Jilin University, Changchun, China
- 3Department of Hand and Foot Surgery, The First Hospital of Jilin University, Changchun, China
- 4School of Grain Science and Technology, Jilin Business and Technology College, Changchun, China
- 5School of Pharmacy, Changchun University of Chinese Medicine, Changchun, China
Wounds can be divided into two categories, acute and chronic. Acute wounds heal through the normal wound healing process. However, chronic wounds take longer to heal, leading to inflammation, pain, serious complications, and an economic burden of treatment costs. In addition, diabetes and burns are common causes of chronic wounds that are difficult to treat. The rapid and thorough treatment of chronic wounds, including diabetes wounds and burns, represents a significant unmet medical need. Wound dressings play an essential role in chronic wound treatment. Various biomaterials for wound healing have been developed. Among these, hydrogels are widely used as wound care materials due to their good biocompatibility, moisturizing effect, adhesion, and ductility. Wound healing is a complex process influenced by multiple factors and regulatory mechanisms in which stem cells play an important role. With the deepening of stem cell and regenerative medicine research, chronic wound treatment using stem cells has become an important field in medical research. More importantly, the combination of stem cells and stem cell derivatives with hydrogel is an attractive research topic in hydrogel preparation that offers great potential in chronic wound treatment. This review will illustrate the development and application of advanced stem cell therapy-based hydrogels in chronic wound healing, especially in diabetic wounds and burns.
1 Introduction
Wound formation occurs due to physical or chemical factors or skin and soft tissue damage caused by medical and physiological conditions (Eming et al., 2014). Wound healing results from the interaction of a series of biomolecules and stem cells and is considered one of the most complex dynamic physiological processes in the human body (Hadisi et al., 2018). An acute wound is defined as a skin injury due to trauma or surgery that usually heals in an orderly and timely process without complications (Lazarus et al., 1994). Chronic wounds usually do not regain their functional integrity within 3 months or even show a tendency to heal (Malone-Povolny et al., 2019). They are more likely to be caused by continuous stimulation and not only to heal slowly but also relapse. The Wound Healing Society classifies chronic wounds into four categories, depending on their cause: pressure ulcers, venous ulcers, arterial insufficiency ulcers, and diabetic ulcers (Kirsner, 2016). Compared with the normal wound healing process, the causes and symptoms of chronic wound healing are more complex and severe (Martin and Nunan, 2015). Therefore, chronic wound healing is a significant challenge in modern medicine. There is an urgent need to conduct relevant research on promoting chronic wound healing. Stem cells are a special type of cell with extensive self-renewal ability and further differentiation ability, which can respond to injury and repair tissues by proliferating and differentiating (Watt and Driskell, 2010). They can not only differentiate into different functional cells and promote the whole regeneration process but also stimulate and promote tissue regeneration by secreting functional growth factors (Nourian Dehkordi et al., 2019). In addition, they can regulate angiogenesis, remodeling, cell recruitment, and the immune system (Stappenbeck and Miyoshi, 2009). Therefore, different types of stem cells play important roles in wound healing, including epidermal and dermal stem cells, mesenchymal stem cells (MSCs), endothelial progenitor cells (EPCs), and hematopoietic stem cells (HSCs) (Kucharzewski et al., 2019). Importantly, stem cell therapy shows excellent potential for promoting wound repair.
Many chronic wounds require treatment with wound dressings, which prevent pollution and repeated damage, protect against compression hemostasis and swelling, apply drugs to the wound, and remove necrotic tissue (Zeng et al., 2022). Hydrogel is considered an ideal material for chronic wound dressing because of its three-dimensional structure, good permeability and biocompatibility, easy cleaning, and provision of a moist environment conducive to wound repair (Tavakoli and Klar, 2020). Numerous studies have shown that hydrogel is a new biomaterial with broad application prospects in wound healing (Wang et al., 2021). Hydrogels commonly used in chronic wound healing include polypeptide-based gelatin, silk fibroin, fibrin, polysaccharide-based chitosan, hyaluronic acid, and alginate. Hydrogels are formed through the crosslinking of hydrophilic polymer chains in water (Lu et al., 2018). The many methods for preparing hydrogel include electrostatic interaction and covalent chemical bond crosslinking (Su et al., 2021). Traditional hydrogels lack strength and are prone to permanent breakage, and their internal structure is simple and lacks specialized functions (Zhang and Khademhosseini, 2017). Therefore, hydrogels currently in use are typically modified by adding chemical or molecular substances or optimizing mechanical properties. According to the different needs of chronic wounds, hydrogels with anti-inflammatory, antioxidant, pro-angiogenic, antibacterial, hypoglycemic, heat-sensitive, and even multifunctional properties have been produced.
The specialized structure of hydrogels allows for different functional polymers or bioactive substances to be incorporated to promote wound healing (Wang et al., 2021). An area of rapid growth is combining hydrogels with stem cell-based therapies that load stem cells or stem cell derivatives into hydrogels to promote wound healing (Las Heras et al., 2020). This review summarizes the application of different types of hydrogel-based stem cell therapy to treat chronic wounds, including those formed by diabetes and deep burns.
2 Chronic wounds
When the skin or surface soft tissue is damaged by trauma, injury, burn, ulcer, surgery, chronic disease, or inflammation, it usually hinders basic physiological functions in the human body and requires repair through a process called wound healing (Eming et al., 2014). When the wound healing process is disturbed, it can slow healing and cause pathological changes, forming chronic wounds (Richmond and Harris, 2014). Most chronic wounds are serious skin and tissue injuries, which are difficult to heal, and cause the body to receive external stimuli directly (Wilkinson and Hardman, 2020). In addition, slow wound healing can lead to poor patient resistance, malnutrition, septicemia, and other complications (Guo and Dipietro, 2010). Furthermore, chronic wounds that repeatedly trigger inflammatory responses can lead to immune and metabolic disorders and further damage vascular structural integrity and tissue regeneration (Malone-Povolny et al., 2019). At present, hydrogels are recognized as biological materials with properties well suited to wound dressing. Normal wound healing involves the orderly progression of hemostasis, inflammation, proliferation, and remodeling (Guo and Dipietro, 2010). However, chronic wounds do not complete this process effectively, as shown by prolonged hemostasis or inflammation, resulting in a failure of proliferation and remodeling (Figure 1).
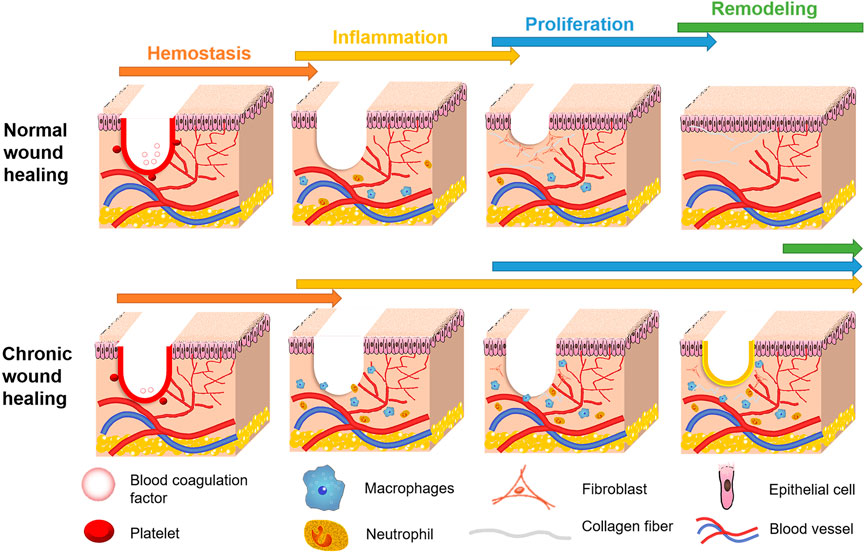
FIGURE 1. Comparison of healing process between chronic wound and normal wound. The U-shaped red line in wound area indicates that the wound is in a hemostatic stage, while the yellow line indicates that the wound is not healing and is in an inflammatory state.
2.1 Factors affecting chronic wound healing
The main stages of subdivision that occur during wound healing include hemostasis, collagen deposition, stromal remodeling, angiogenesis, re-epithelialization, and scar formation (Laurens et al., 2006). Factors that can cause wound healing to fail include hyperglycemia, repeated tissue damage (Randeria et al., 2015), long-term or excessive inflammation, persistent infection, and attenuation of microbial, dermal, and epidermal responses in repair stimulation (Frykberg and Banks, 2015). Skin structure can be divided into three layers: the epidermis (epithelium), dermis, and subcutaneous layer (Vijayavenkataraman et al., 2016). During hemostasis, blood vessels constrict, and platelets accumulate on the subcutaneous surface (Kharaziha et al., 2021). At this stage, fibrin plays a role in wound healing by regulating hemostasis and internal environmental balance (Heher et al., 2018). Fibroblasts in the subcutaneous tissue proliferate during the cell proliferation phase to form contractile granulation tissue and produce collagen deposition (Li and Wang, 2011). Proliferating fibroblasts reshape the extracellular matrix (ECM), while endothelial cells proliferate, migrate, and recombine to form new blood vessels (Tracy et al., 2016). In the re-epithelialization stage, epidermal stem cells proliferate to rebuild the epidermis (Ben Amar and Wu, 2014). At the same time, the body will undergo an immune response, where macrophages, neutrophils, and other immune cells participate in the wound healing process. Therefore, the cells recruited to the wound contribute to wound healing through proliferation, differentiation, or secretion of regulatory substances.
In addition, certain cytokines play an important role in the wound healing process. Macrophages and neutrophils secrete inflammatory cytokines to slow wound healing (Krzyszczyk et al., 2018). For example, vascular endothelial (VEGF), fibroblast (FGF), and platelet-derived (PDGF) growth factors affect hemostasis and angiogenesis (Shen et al., 2016). Moreover, hepatocyte growth factor (HGF) contributes to the re epithelialization of injured tissues (Dally et al., 2017), while both FGF and epidermal growth factor (EGF) regulate the re-epithelialization process (Seeger and Paller, 2015). HGF regulates re-epithelialization by binding to and activating the MET receptor tyrosine kinase (Chmielowiec et al., 2007). The re-epithelialization regulators HGF, FGF, and EGF are also ligands of receptor tyrosine kinases. Receptor tyrosine kinase activation usually stimulates the migration, proliferation, and survival of keratinocytes (Schafer and Werner, 2007). Moreover, the proliferation-promoting transforming growth factor-β (TGF-β) regulates the transcriptional regulator SMAD3 and influences the epithelial-mesenchymal interaction (Werner and Alzheimer, 2006).
MSCs can promote wound healing, especially in the treatment of chronic wounds (Rodgers and Jadhav, 2018). MSCs are thought to migrate to damaged tissue and release growth factors such as EGF, FGF, PDGF, and TGF -β, VEGF, insulin-like growth factor-1, angiopoietin-1 (ANGPT1), and stromal cell-derived factor-1 all influence fibroblast and endothelial cell development (da Silva Meirelles et al., 2009). Moreover, dendritic (DC) and natural killer (NK) cells mediate the tissue injury immune process in addition to inhibiting lymphocytes, and MSCs interact with macrophages and neutrophils to produce important regulatory effects (Seno et al., 2009). For example, MSCs participate in immune regulation through the PD-1:PD-L1/2 pathways (Corcione et al., 2006). In addition, epidermal stem cells, embryonic stem cells (ESCs), and induced pluripotent stem cells (IPSs) may also promote the chronic wound healing process.
2.2 Diabetes and burn wounds
Some conditions and diseases, such as diabetes and deep burns, can significantly affect the rate of wound healing and even cause the formation of chronic wounds. Diabetes is a metabolic disease characterized by hyperglycemia, which can cause chronic damage and dysfunction of various tissues, especially the eyes, kidneys, heart, blood vessels, and nerves (American Diabetes, 2014). Diabetic patients often have a weakened immune system and suffer long-term complications, including chronic wounds. The main features of diabetic wounds are imbalanced inflammatory reaction, increased risk of infection, and insufficient angiogenesis, which are usually affected by complex factors such as immune deficiency, dysfunction, and local infection (Wang et al., 2021). The average wound healing process lasts 12–13 months, and the possibility of recurrence is very high, which seriously affects the comfort and health of patients and increases the cost and difficulty of treatment (Richmond et al., 2013). The wound healing process in people with diabetes is stagnant at the inflammatory stage, characterized by elevated levels of pro-inflammatory cytokines, protease and reactive oxygen species (ROS), and cell dysfunction (Frykberg and Banks, 2015). ROS induces the expression of serine proteases and matrix metalloproteinases (MMPs), leading to ECM and growth factor degradation, further inhibiting wound healing and promoting an inflammatory response (Demidova-Rice et al., 2012). In addition, their accumulation of advanced glycation end products (AGEs) can induce ROS production and induce nuclear factor kappa B (NF-κB) activation through binding to the receptor for advanced glycation endproducts (RAGE) expressed in various skin cells, including keratinocyte, fibroblast, dendritic, endothelial, and mononuclear cells, leading to pathological gene expression (Lohwasser et al., 2006).
The severity of a burn victim’s injury depends on the wound’s depth and the body surface area affected. From minor to the most severe burns requiring the highest level of intensive care and surgery, chronic wounds are considered deep burns (Wasiak et al., 2013). Deep burns involve deeper skin structures, such as blood vessels, nerves, and hair follicles, causing considerable pain and taking a long time to heal (Zhang et al., 2015). How the burns are treated also influences their wound healing time and affects their risk of infection. Superficial burns may develop into deeper wounds if they are dry or infected. The malignant transformation of burns can lead to tissue ischemia, cytokine environment disturbance, and free radical damage, causing further protein degeneration and necrosis (Singh et al., 2007). Common complications from burns caused by abnormal wound healing include a hypertrophic scar, post-burn contracture, and non-healing, where increased smooth muscle alpha-actin (α-SMA) and collagen levels due to myofibroblast activation are markers of hypertrophic scar formation (Hall et al., 2017). Therefore, given the serious impact of chronic wounds caused by diabetes and burn on patient recovery and health, the development of new diabetic wounds and burn dressings are of particular importance.
3 The application of hydrogel combined with stem cells in the treatment of chronic wounds
Numerous methods for treating chronic wounds have been proposed that each target different stages in the wound healing process. These methods include different types of dressing, delivery of cytokines and growth factors, cell therapy, and application of electrical or mechanical stimulation (Frykberg and Banks, 2015). Among them, using artificial construction biomaterials as dressings that promote the wound healing process is considered one of the most effective strategies. Given this, many different types of biomaterial-based wound dressings have recently been developed to simulate the skin’s microenvironment (Bhardwaj et al., 2017). Among them, hydrogels with bionic structure and physical properties have been widely used in wound healing (Xie et al., 2015). Hydrogel has high biocompatibility, encapsulating all types of cells and biological macromolecules that are then released under various external conditions (Naahidi et al., 2017). Hydrogel is an insoluble polyurethane polymer with high hydrophilic content. Its hydrophilicity is determined by the cross-linking degree of polar functional groups. The high porosity and large specific surface area of hydrogels also enable them to retain water and transport materials due to their 3D porous network structure (Hao et al., 2021). Based on their rheological properties, hydrogels are viscoelastic in nature and the hydrogel will not stick to the wound, which can be conveniently smeared and removed without causing secondary damage (Wang et al., 2021). This versatility makes hydrogel a promising biomaterial for delivering therapeutic molecules and indicates that hydrogel dressings incorporating stem cell therapy have good prospects in chronic wound healing. Moreover, different hydrogels have different characteristics that can improve stem cell delivery to the wound depending on their properties (Table 1).
MSCs are a type of pluripotent stem cell with all the attributes commonly associated with stem cells, including the ability to self-renew and multidirectional differentiation (Chen et al., 2022), found in the bone marrow, adipose tissue, umbilical cord, and placenta (Lin et al., 2019). MSCs offer great potential in treating various diseases, especially those related to tissue injury (Bhardwaj et al., 2017), as shown by numerous studies that have used them to promote chronic wound healing (Varderidou-Minasian and Lorenowicz, 2020). MSCs and MSC-like cells exist in almost all tissues, including bone marrow, muscle, fat, hair follicle, and dermis (Bianco et al., 2008). Moreover, MSCs have the advantages of wide distribution and easy separation and culturing in vitro (Shi et al., 2010). However, the realization of clinical treatment requires the delivery of large numbers of stem cells to specific sites in the body with high precision. Due to their low transplantation efficiency, the clinical transformation of MSCs is hard to achieve. Therefore, combining them with biomaterials such as hydrogel has the potential to improve the efficiency and stability of MSCs delivery, enhancing traditional MSC-mediated repair and related cellular functions (Meier et al., 2015). The combination of MSCs and hydrogel has become the leading direction in wound healing research.
3.1 Hydrogels based on bone marrow MSCs promote chronic wound healing
Bone marrow mesenchymal stem cells (BMSCs) are a type of adult stem cell originating from the mesoderm, which can differentiate into various mesenchymal tissues, such as bone, cartilage, adipose, bone marrow, and hematopoietic (Wu et al., 2018). MSCs regulate the wound repair process by differentiating into several stromal or damaged cell types and interacting with many types of tissue and immune cells through bioactive factors, including fibroblasts, endothelial and epithelial cells, macrophages, neutrophils, and lymphocytes (Shi et al., 2010) (Figure 2).
The Detachable hybrid microneedle depot (d-HMND) made of gelatin methacryloyl (GelMA) hydrogel is an efficient drug delivery vehicle that can minimize drug dose and can degrade stably for long-term release delivery cells. The d-HMND has been proposed for BMSCs delivery and demonstrated in vitro and in vivo that MSCs delivered by hydrogel can promote mouse full skin thickness excisional wound regeneration by secreting VEGF (Lee et al., 2020). Besides, in vitro experiments showed that BMSCs encapsulated in a polyethylene glycol diacrylate (PEGdA) and thiogelatin polyethylene glycol (Gel-PEG-Cys) crosslinked hydrogel were shown to have a beneficial effect on wound healing and the antibacterial loaded hydrogel enhanced the proliferation, chemotaxis and haptotaxis of stem cells (Guerra et al., 2017). A fibrin hydrogel carrier was designed to enhance the angiogenesis and anti-inflammatory ability of the encapsulated BMSC globules and promote the secretion of VEGF and prostaglandin E2 (PGE2) and wound healing in three-dimensional endothelial human skin equivalent model (Murphy et al., 2017). In addition, thermosensitive chitosan (HBC) modification to create an eLHBC hydrogel coated in BMSCs was found to have adhesiveness and antibacterial activities and facilitate wound healing by promoting fibroblast migration and secretion of VEGF and FGF in the incisions of rats skin (Tian et al., 2021). Furthermore, BMSCs combined with a novel biocompatible heat-sensitive hydrogel have been shown to enhance α-SMA expression in full-thickness wound of mouse model, significantly promoting wound healing, epithelial cell proliferation, re-epithelialization, and collagen deposition (Lei et al., 2018). In addition, a fibrin hydrogel containing 0.7 × 106 BMSCs was implanted subcutaneously in rats and found to promote wound healing and repair and interact with VEGF to promote endothelial cell proliferation and differentiation, potentially promoting angiogenesis in the injured areas (Tan et al., 2021). A thinned silk nanofiber hydrogel encapsulating BMSCs and injected into the SD rats wound site has been shown to accelerate wound healing by promoting the up-regulation and secretion of ANGPT1 and HGF. And shear thinning hydrogels can protect BMSCs and optimize their behavior during injection. (Li J. et al., 2021). Furthermore, delivery of BMSCs extracellular vesicles (EVs) loaded with miR-29b-3p in a bilayered thiolated alginate/PEG diacrylate (BSSPD) hydrogel showed that hydrogels can sequentially release EVs and miR-29b-3p can rapidly heal wounds and reduce scar formation by inhibiting collagen type I alpha 1 chain (Col1A1) expression in the full-thickness skin defect model of rats and rabbit ears (Shen et al., 2021). Therefore, BMSCs are the most common type of stem cell used to promote wound healing, as they can promote cell proliferation and angiogenesis and inhibit inflammation.
3.2 Hydrogels based on adipose MSCs promote chronic wound healing
Adipose mesenchymal stem cells (ADSCs) are pluripotent stem cells derived from adipose tissue that can be induced to differentiate into various cell types under specific conditions, including adipose, bone, cartilage, islet β, and cardiac muscle (Miana and Gonzalez, 2018). Recently, numerous studies have applied ADSCs to wound healing, where they act on fibroblasts, macrophages, and skin cells by secreting growth factors, including growth differentiation factor 11 (GDF11) and TGF-β, and regulating the immune response, cell proliferation, and angiogenesis during wound healing (Mazini et al., 2020).
A short peptide (GV8) hydrogel can self-assemble and maintain the activity of delivery cells under mild physiological conditions. GV8 peptide hydrogel containing ADSCs secretome has been shown to have good potential in full-thickness excisional wound healing C57/BL6 mice model (Hiew et al., 2021). Here, ADSCs secrete factors that regulate angiogenesis and endothelial cell migration (thymosin beta 4 X-linked [TMSB4X], gremlin 1 [GREM1], and extracellular matrix protein 1 [ECM1]), metalloproteinase inhibition (tissue inhibitors of metalloproteinases 1 [TIMP1]), cell proliferation (EGF containing fibulin extracellular matrix protein 1 [EFEMP1]), immune cell proliferation and morphogenesis (colony-stimulating factor 1 [CSF1]), and growth factor interaction (latent transforming growth factor-beta binding protein 1 [LTBP1]) (Hiew et al., 2021). In addition, platelet-rich plasma hydrogel can provide a stable physical framework and promote the potential of ADSCs in vitro and in vivo, increasing their expression of ANGPT1 and angiopoietin 2 (ANGPT2) and promoting male athymic rats wound healing (Samberg et al., 2019). Similarly, a biomimetic pullulan collagen hydrogel scaffold can deliver ADSCs into the splinted murine wounds environment and enhance their stem cell properties by increasing the expression of octamer-binding transcription factor 4 (Oct4). Moreover, ADSCs accelerate wound healing by significantly enhancing the expression of multiple factors, including stromal cell-derived factor-1 (SDF-1), monocyte chemoattractant protein-1 (MCP-1), fibroblast growth factor 2 (FGF-2), insulin-like growth factor 1 (IGF-1), VEGF-a, endoglin (ENG), HGF, and ANGPT1 (Garg et al., 2014). In addition, UV crosslinked biodegradable hydrogel containing ADSCs can be used as a the dermal layer of a bilayer skin substitute and promote the formation of blood vessels and increase the number of endothelial cells (Eke et al., 2017). Moreover, an adhesive hydrogel consisting of alginate, Arabia gum, and calcium ions has been synthesized and shown excellent mouse wound healing abilities after combined use with 1 × 106 mouse ADSCs (Sun et al., 2016). Furthermore, a hydrogel loaded with the secretory body from the human ADSC line HATMSC2 was found to contain high levels of interleukin-8 (IL-8) and MCP-1, and relatively high expression of the pro-angiogenic microRNAs miR210, miR126, and miR296, indicating that it has good potential in chronic wound treatment (Kraskiewicz et al., 2021). Moreover, the ADSC-derived exosomes applied within an alginate-coated hydrogel significantly promoted wound closure, collagen synthesis, and wound angiogenesis in the full-thickness excisional wound of rat model (Shafei et al., 2020). At present, stem cell therapies based on ADSCs are gradually emerging, and the combination of ADSCs and hydrogel has gradually become mainstream in wound healing research.
3.3 Hydrogels based on other types of stem cells promote wound healing
Umbilical cord mesenchymal stem cells (UCMSCs) are a type of multifunctional stem cell that exists in the neonatal umbilical cord tissue and can differentiate into many types of tissue cells. Studies have shown that functional injectable thermosensitive chitosan hydrogel-coated 5 × 106 human UCMSCs can promote SD rats skin wound healing and inhibit wound inflammation by reducing tumor necrosis factor-α (TNF-α) and interleukin 1 beta (IL-1β) protein levels (Xu et al., 2019). Another study constructed a biodegradable, dual-sensitive hydrogel-coated human UCMSC-derived exosomes that inhibit inflammatory responses and promote female SD rats wound healing and skin regeneration by promoting collagen deposition and regulating TNF-α and IL-1β expression (Li Q. et al., 2021). In addition, human UCMSC-derived exosomes in a silk fibroin and sericin composite hydrogel can promote vascular growth and inhibit the inflammatory response, promoting C57BL/6J mice wound healing (Han et al., 2021). Furthermore, sodium alginate/collagen (SA/Col) hydrogel is injectable, biodegradable, and has low immunogenicity, which can promote the retention and survival of UCMSCs in vivo. Studies have shown that a SA/Col hydrogel containing human UCMSCs significantly upregulates TGF-β1 expression, accelerates keratinocyte maturation, inhibits inflammatory responses by inhibiting NLRP3 signaling, and promotes wound healing and skin regeneration (Zhang Z. et al., 2021).
Human amniotic mesenchymal stem cells (hAMSCs) derived from placental amniotic tissue are adult stem cells with apparent plasticity and multidirectional differentiation potential. Studies have shown that hAMSCs are suitable for skin tissue engineering and regenerative medicine (Farhadihosseinabadi et al., 2018). Moreover, study have shown that an acellular dermal matrix hydrogel decorated with carbon nanodots can enhance chronic wound healing by transporting hAMSCs to clear ROS and upregulate collagen I antibody expression in adult Wistar male rats (Bankoti et al., 2020).
Compared with IPSs or ESCs, tissue-specific mature stem cells such as MSCs are considered more acceptable and safer and have more potential for medical applications (Sharma et al., 2021). Therefore, most studies combining stem cell therapy with hydrogel materials to promote wound healing have used MSCs or their derivatives. However, some studies have shown that functional tissue regeneration can be achieved using mesenchymal cells derived from human ESCs in a hydrogel matrix (Hwang et al., 2008), and ESCs may offer another promising direction in wound healing research. While UCMSCs and hAMSCs are rarely used at present, they also have beneficial effects on promoting wound healing, and like other unstudied stem cell types, would benefit from additional research and development.
4 The application of hydrogel combined with stem cells in the treatment of diabetes and burn chronic wounds
Diabetic wounds no longer have the normal skin regeneration function and are difficult to heal through normal physiological processes (Greenhagen et al., 2019). They usually occur on the limbs, especially the feet, and are prone to infection and recurrence (Krishnan et al., 2007). Severe full-thickness burns involve all skin structures, including blood vessels, nerves, and hair follicles, and may also involve underlying structures such as muscle and bone. Burns are the main cause of extensive body surface hypertrophic scarring (Markeson et al., 2015). Wound dressing with hydrogel stem cells can promote wound healing by secreting cell growth factors and exosomes and cooperating with drugs (Figure 3).
4.1 Hydrogels based on stem cells promote diabetic wound healing
Diabetic chronic wounds are characterized by unbalanced inflammatory responses, oxidative stress effects, hyperglycemia, absence of angiogenesis, and a high risk of bacterial infection (Wang et al., 2021). Therefore, hydrogel-based stem cell therapy as a new biomaterial has attracted much attention in promoting diabetic wound healing because of its water absorption, permeability, biocompatibility, and drug loading ability, which can control drug release and provide a stable and suitable growth environment for chronic wounds (Cascone and Lamberti, 2020). Introducing stem cells or bioactive substances derived from stem cells into hydrogels can regulate the chronic wound microenvironment and accelerate wound healing in diabetic patients.
A new type of chitosan polyurethane hydrogel membrane (HPUC) with excellent antibacterial and hemostatic activity and bioabsorbability has been developed, which promotes bone healing and reduces inflammation by transplantation of BMSCs in rats with diabetes mellitus (DM) (Viezzer et al., 2020). Another study used a self-healing hydrogel containing 2×105 BMSCs to release TGF-β1, VEGF, and basic FGF, to inhibit the activation of M1 macrophages and promote the activation of M2 macrophages, aiding late wound healing of diabetic foot ulcers in SD rats (Bai H. et al., 2020). It has been reported that a hydroxyapatite/chitosan composite (HAP-Cs) hydrogel coated in miR-126–3p overexpressed MSC-derived exosomes that can activate angiogenesis by targeting the mitogen-activated protein kinase (MAPK)/extracellular signal-regulated kinase (ERK) and phosphoinositide 3-kinase (PI3K)/protein kinase B (AKT) signaling pathways and promote diabetic SD rats wound healing (Li et al., 2016). Other studies have shown that MSCs EVs bind to porcine submucosal hydrogel materials through peptides and promote the proliferation and migration of cells in diabetic rats full-thickness skin wounds by activating the wingless/integrated (Wnt)/β-catenin pathway and promoting angiogenesis by activating the hypoxia-induction-factor-1 α (HIF-1α)/VEGF pathway (Ma et al., 2022). Another study showed that loading ADSCs on the gelatin sericin (GS) hydrogel scaffold inhibits ROS damage and promotes wound angiogenesis in Wistar rat diabetic ulcers. GS hydrogels contain laminin, an endothelial basal protein that can improves angiogenesis (Tyeb et al., 2020). An injectable Gel-PEG hydrogel system was used to transport 3 × 105 ADSCs to diabetic wounds, significantly accelerating wound healing by reducing inflammatory cell infiltration and enhancing neovascularization in a full-thickness excisional wound model in db/db diabetic mice (Dong et al., 2018). Furthermore, ADSCs precultured in hyaluronidase-based spongy hydrogel increased numbers of intraepidermal nerve fibers (IENF), promoting the growth of new nerves, reducing the number of macrophages on the wound surface, and promoting the transition from the inflammatory to proliferative stage of the wound surface, promoting the healing of diabetic mice full-thickness wounds (da Silva et al., 2017). In addition, ADSC exocrine loaded on an antibacterial hydrogel wound dressing promoted wound healing in DM rats by preventing infection, fibroblast proliferation, and granulation tissue formation (Shiekh et al., 2020). Another study developed an injectable, self-healing, and antibacterial polypeptide-based FHE hydrogel that stimulates the release of exosomes from reactive ADSCs and promotes human umbilical vein endothelial cell proliferation, migration, and angiogenesis, co-promoting chronic wound healing and complete skin regeneration (Wang et al., 2019). Besides, a clinical study exploring the potential of hydrogel-based allogeneic ADSCs sheets for the treatment of foot ulcers in diabetic patients found that 82% of the patients in the treatment group had complete wound closure without adverse effects within 12 weeks (Moon et al., 2019). In addition, studies have shown that the combined use of human UCMSC-derived exosomes and F-127 hydrogel enhances VEGF and TGFβ-1 expression, promoting wound healing in DM rats (Yang et al., 2020). Moreover, an injectable hydrogel composed of collagen and polyethylene glycol with controlled drug release and adhesion, loaded with umbilical cord stem cell factor (SCF), has also been developed to treat diabetic rat wounds, decreasing TNF-α expression and increasing VEGF expression in the SCF treatment group (Zhang L. et al., 2021). In addition, the combined use of Wharton jelly mesenchymal stem cells (WJMSCs) with PF-127 hydrogel and sodium ascorbate phosphate (SAP) promoted skin wound healing in diabetic rats, and the addition of SAP reduced the apoptotic rate of WJMSCs in hydrogel by reducing oxidative stress and mitochondrial damage (Jiao et al., 2021). Furthermore, using thermosensitive hydrogel as a scaffold to increase the implantation of muscle-derived mesenchymal stem cells (MDSCs) and improved db/db genetically diabetic mouse model wound healing (Lee et al., 2007). These findings indicate that using hydrogels loaded with stem cells and their derivatives as dressings is a promising method for treating chronic diabetic wounds (Table 2). Furthermore, future research should focus on treating diabetes using modified hydrogels loaded with stem cell exosomes with different characteristics.
4.2 Hydrogels based on stem cells promote burn wound healing
Slow wound healing, infection, pain, and hypertrophic scarring remain significant challenges in burn treatment and research (Wang et al., 2018). Severe burns have limited vascular perfusion, which may lead to excessive scarring. Progressive microvascular damage, edema, arteriolar thrombosis, and necrosis due to impaired tissue perfusion can deepen and enlarge the burn wound (Xue et al., 2018). Hydrogel delivery systems not only control the release of bio-therapeutic products such as stem cells in space and time but also mimic the natural ECM microenvironment (Xue et al., 2018). Considering their ease of application and disassembly, dressing change requirements, cost, and patient comfort (Wasiak et al., 2013), the design and modification of hydrogel dressings make them valuable and beneficial for various applications. Stem cells can secrete and chemotaxis growth factors sustainably, respond to local stimuli, influence the wound microenvironment to promote healing, reduce scar formation, improve skin regeneration, regulate inflammatory responses, and reduce the risk of fibrosis and infection (Abdul Kareem et al., 2021). The combined use of stem cells with hydrogels as a wound dressing or skin substitute—another commonly used treatment for burns—protects large wounds and increases the dermal component of the wound to promote healing (Halim et al., 2010).
Studies have shown that a hyaluronic acid hydrogel system can load ADSCs, increase wound angiogenesis and normal tissue remodeling, and significantly increase the expression of growth factors and cytokines that promote female FVB/NJ mice with deep second-degree burns healing, such as PDGF, SDF-1, haemodiafiltration (HDF), metalloproteinase-2 (MMP2), metalloproteinase-9 (MMP9), ANGPT1, ANGPT2, C-C Motif Chemokine Ligand 2 (CCL2), MCP-1, and VEGF-a (Dong et al., 2020). In addition, delivering ADSCs using polyethylene glycol fibrin hydrogels promoted deep partial thickness burn healing and reduced scar production in porcine models by releasing VEGF and increasing collagen fiber densification (Burmeister et al., 2018). Another study used 3D bio-printing to construct an integral 3D-ADscs/NO hydrogel scaffold to enhance the migration and angiogenesis of human umbilical vein endothelial cells (hUVECs) through VEGF expression and promote severe full-thickness burn wound healing in Balb/c mice (Wu et al., 2021). Moreover, autologous stem cells have been isolated from the adipose layer of surgical debridement burn skin (dsADSCs) that can differentiate into the upper cortex, vascularized dermis, and subcutaneous layer in collagenous PEG fibrin-based bilayer hydrogels, which can be used for deep burn skin allografts (Chan et al., 2012). In other studies, 1 × 106 ADSCs loaded into aloe vera hydrogel promoted angiogenesis and granulation tissue formation in rat burn models by increasing the expression of FGF and TGF-β1, promoting debrided full thickness wound healing (Oryan et al., 2019). Furthermore, enzyme crosslinked gelatin hydrogels have been used to encapsulate hADSCs spheroid, which significantly reduced scar formation and promoted neovascularization by releasing PDGF, VEGF, and FGF in male Wistar rats. Besides, it was found that hADSCs spheroid with hydrogel scaffolds could release more growth factors because they had more cell-cell/cell-ECM interactions (Lu et al., 2020).
Current stem cell therapies specifically applied for burn treatment are still based primarily on ADSCs, with the direct encapsulation of stem cells used. Applying other types of stem cells or stem cell-derived bioactive substances, such as exosomes, to deep burns is an important future research direction.
5 Discussion
Chronic wound healing is an important healthcare issue, and research on promoting chronic wound healing is an important area. The treatment of chronic wounds in diabetes and severe burns is also an urgent research topic. Wound dressings used to accelerate wound healing have attracted much attention, and many studies believe that hydrogels are one of the most valuable biomaterials for promoting chronic wound healing due to their unique properties, including biocompatibility, moisture retention, ductility, permeability, and controlled delivery of therapeutic drugs (Zhang et al., 2020). Currently, hydrogels used in most studies are loaded with functional bioactive molecules, such as stem cells, to target treatments for wound-healing indicators such as angiogenesis, collagen deposition, and inhibition of inflammatory responses to treat chronic wounds.
The review has highlighted how hydrogel delivery of stem cells to the injury site is an effective strategy for improving chronic wound healing (Sivaraj et al., 2021). Stem cells, including MSCs, have the potential to undergo multidirectional differentiation, induce cell proliferation and migration to the wound surface, and promote skin repair (Nuschke, 2014). ECM and the wound-healing environment can also be regulated by synthesizing collagen and fibronectin or secreting growth factors (Badiavas and Falanga, 2003). Moreover, MSCs decrease the secretion of pro-inflammatory cytokines (tumor necrosis factor and interferon) and increase the expression of anti-inflammatory cytokines such as interleukin-10 (IL-10) and interleukin-4 (IL-4) (Na et al., 2017). They also act on fibroblasts and macrophages through GDF11 and TGF-β, mediating the immune response, cell proliferation, and angiogenesis (Sharma et al., 2021). VEGF and FGF are also important beneficial MSC mediators of angiogenesis (Werner, Grose). In addition, MSCs preferentially target injured or damaged tissue sites and are easily cultured in vitro, and many studies have used them in clinical applications (Shi et al., 2010). Hydrogels have good biocompatibility, degradability, adhesion, moisture resistance, and antimicrobial properties and can be used as a non-biological scaffold for delivering and continuously releasing stem cells. Bioactive hydrogel loaded with stem cells or stem cell-derived substances can significantly shorten wound healing time by inhibiting infection (Chen et al., 2021), reducing the inflammatory reaction (Garcia et al., 2019), stimulating cell migration and proliferation (Yu et al., 2020), and promoting angiogenesis in the wound healing process (Cerqueira et al., 2014).
Hydrogel-loaded stem cells for wound healing are expected to offer good clinical treatment (Supplementary Figure S1). However, there are few studies on the application of hydrogel stem cells in clinical practice, and the use of stem cells in human body is limited. In view of the characteristics of stem cells, the application of hydrogels is developing towards promoting the activity of stem cells, promoting the secretion of cytokines and the sustained release of stem cells. Moreover, hydrogels can be modified with macromolecules to obtain special properties suitable for chronic wounds, such as self-healing (Zhang et al., 2020), antioxidant (Xu et al., 2020), injectable (Gao et al., 2020), immunomodulatory (Kharaziha et al., 2021), and antibacterial hydrogels (Zhong et al., 2020). Furthermore, more distinctive hydrogel designs are emerging with the development of new synthetic and processing technologies. Currently, there exist many polymeric dynamic hydrogels that respond to changes in the cellular environment, enhancing the potential of stem cell-based wound therapy (Burdick et al., 2016).In addition, stem cell-derived exosomes are also an emerging therapeutic method that avoids the risk of directly introducing cells into the wound and has broad application prospects (Safari et al., 2022). Because the etiology of diabetic ulcer wounds is complex, future directions may include developing new hydrogel materials with multiple effects by combining multiple substances released at different wound healing stages (Bai Q et al., 2020). Conversely, burn research may instead focus on how to prevent scar formation, reduce patient pain (Wang et al., 2018), and develop skin graft substitutes.
However, pressure ulcers in DM patients are still a difficult problem to solve clinically. It has been shown that methylacrylic gelatin frozen hydrogel coated EPCs and acid fibroblast growth factor (aFGF) can treat pressure ulcers in DM rats (Zhu et al., 2022). Besides, there have been studies to promote the treatment of venous leg ulcers by wrapping antisense oligodeoxynucleotides targeting the mRNA of gap junction protein Cx43 in the thermoreversible hydrogel Pluronic F-127 (Gilmartin et al., 2016). In addition to applying hydrogel dressings, wound management strategies include several other common approaches. Compression therapy is the mainstay of venous ulcers, arterial ulcers may require revascularization surgery, and diabetic foot ulcers require ICC pressure relief (Alam et al., 2021). Additionally, hyperbaric oxygen therapy (HBOT) has physiological effects that can promote tissue repair and has multiple approved indications, but data on its treatment remain controversial. Studies suggest that HBOT is best used in diabetic foot ulcers, but its long-term efficacy remains to be determined (Kranke et al., 2015). Therefore, hydrogel combined with stem cells is a new and promising treatment method for chronic wounds.
This review helps to focus more precisely on the research progress of hydrogel combined with stem cells for wound healing (Supplementary Figure S2). Hydrogel dressings based on stem cell therapy for promoting wound healing are an important area for future research, including optimizing the hydrogel delivery system, maintaining loading material activity, and developing new active molecules to promote chronic wound healing. Moreover, the in-depth study of chronic wound mechanisms, including diabetic and deep burn wound healing, will enable us to expand the use of stem cell hydrogel therapy in treating chronic wounds.
Author contributions
Conceptualization, QL and DW; Funding acquisition, DW, YJ and BS; Supervision, DW; Writing—original draft, QL; Writing—review and editing, QL, DW, ZJ, RL, TX, CL, YD, YJ, and BS.
Funding
This work was supported by the Jilin Health Commission Program under Grant 2020J05S, the Fundamental Research Funds for the Central Universities under Grant 2019JCKT-70, the Jilin Education Department Program under Grant JJKH20200950KJ, and the Jilin Scientific and Technological Development Program under Grant 202002006JC, 20210101010JC and 20220505033ZP.
Conflict of interest
The authors declare that the research was conducted in the absence of any commercial or financial relationships that could be construed as a potential conflict of interest.
Publisher’s note
All claims expressed in this article are solely those of the authors and do not necessarily represent those of their affiliated organizations, or those of the publisher, the editors and the reviewers. Any product that may be evaluated in this article, or claim that may be made by its manufacturer, is not guaranteed or endorsed by the publisher.
Supplementary material
The Supplementary Material for this article can be found online at: https://www.frontiersin.org/articles/10.3389/fchem.2022.1038839/full#supplementary-material
References
Abdul Kareem, N., Aijaz, A., and Jeschke, M. G. (2021). Stem cell therapy for burns: Story so far. Biologics 15, 379–397. Epub 20210831. doi:10.2147/BTT.S259124
Ahmad, S., Ahmad, M., Manzoor, K., Purwar, R., and Ikram, S. (2019). A review on latest innovations in natural gums based hydrogels: Preparations & applications. Int. J. Biol. Macromol. 136, 870–890. Epub 20190619. doi:10.1016/j.ijbiomac.2019.06.113
Alam, W., Hasson, J., and Reed, M. (2021). Clinical approach to chronic wound management in older adults. J. Am. Geriatr. Soc. 69 (8), 2327–2334. Epub 20210517. doi:10.1111/jgs.17177
Alven, S., and Aderibigbe, B. A. (2020). Chitosan and cellulose-based hydrogels for wound management. Int. J. Mol. Sci. 21 (24), 9656. Epub 20201218. doi:10.3390/ijms21249656
American Diabetes, A. (2014). Diagnosis and classification of diabetes mellitus. Diabetes Care 37, S81–S90. doi:10.2337/dc14-S081
Badiavas, E. V., and Falanga, V. (2003). Treatment of chronic wounds with bone marrow-derived cells. Arch. Dermatol. 139 (4), 510–516. doi:10.1001/archderm.139.4.510
Bai, H., Kyu-Cheol, N., Wang, Z., Cui, Y., Liu, H., Liu, H., et al. (2020). Regulation of inflammatory microenvironment using a self-healing hydrogel loaded with bm-mscs for advanced wound healing in rat diabetic foot ulcers. J. Tissue Eng. 11, 204173142094724. Epub 20200731. doi:10.1177/2041731420947242
Bai, Q., Han, K., Dong, K., Zheng, C., Zhang, Y., Long, Q., et al. (2020). Potential applications of nanomaterials and technology for diabetic wound. Int. J. Nanomedicine 15, 9717–9743. Epub 20201203. doi:10.2147/IJN.S276001
Bankoti, K., Rameshbabu, A. P., Datta, S., Roy, M., Goswami, P., Roy, S., et al. (2020). Carbon nanodot decorated acellular dermal matrix hydrogel augments chronic wound closure. J. Mat. Chem. B 8 (40), 9277–9294. doi:10.1039/d0tb01574a
Ben Amar, M., and Wu, M. (2014). Re-Epithelialization: Advancing epithelium frontier during wound healing. J. R. Soc. Interface 11 (93), 20131038. Epub 20140122. doi:10.1098/rsif.2013.1038
Bhardwaj, N., Chouhan, D., and Mandal, B. B. (2017). Tissue engineered skin and wound healing: Current strategies and future directions. Curr. Pharm. Des. 23 (24), 3455–3482. doi:10.2174/1381612823666170526094606
Bianco, P., Robey, P. G., and Simmons, P. J. (2008). Mesenchymal stem cells: Revisiting history, concepts, and assays. Cell. Stem Cell. 2 (4), 313–319. doi:10.1016/j.stem.2008.03.002
Burdick, J. A., Mauck, R. L., and Gerecht, S. (2016). To serve and protect: Hydrogels to improve stem cell-based therapies. Cell. Stem Cell. 18 (1), 13–15. doi:10.1016/j.stem.2015.12.004
Burmeister, D. M., Stone, R., Wrice, N., Laborde, A., Becerra, S. C., Natesan, S., et al. (2018). Delivery of allogeneic adipose stem cells in polyethylene glycol-fibrin hydrogels as an adjunct to meshed autografts after sharp debridement of deep partial thickness burns. Stem Cells Transl. Med. 7 (4), 360–372. Epub 20180218. doi:10.1002/sctm.17-0160
Cai, L., Liu, S., Guo, J., and Jia, Y. G. (2020). Polypeptide-based self-healing hydrogels: Design and biomedical applications. Acta Biomater. 113, 84–100. Epub 20200704. doi:10.1016/j.actbio.2020.07.001
Cascone, S., and Lamberti, G. (2020). Hydrogel-based commercial products for biomedical applications: A review. Int. J. Pharm. X. 573, 118803. Epub 20191101. doi:10.1016/j.ijpharm.2019.118803
Cerqueira, M. T., da Silva, L. P., Santos, T. C., Pirraco, R. P., Correlo, V. M., Reis, R. L., et al. (2014). Gellan gum-hyaluronic acid spongy-like hydrogels and cells from adipose tissue synergize promoting neoskin vascularization. ACS Appl. Mat. Interfaces 6 (22), 19668–19679. Epub 20141113. doi:10.1021/am504520j
Chan, R. K., Zamora, D. O., Wrice, N. L., Baer, D. G., Renz, E. M., Christy, R. J., et al. (2012). Development of a vascularized skin construct using adipose-derived stem cells from debrided burned skin. Stem Cells Int. 2012, 1–11. Epub 20120705. doi:10.1155/2012/841203
Chen, R., Hao, Z., Wang, Y., Zhu, H., Hu, Y., Chen, T., et al. (2022). Mesenchymal stem cell-immune cell interaction and related modulations for bone tissue engineering. Stem Cells Int. 2022, 1–19. Epub 20220201. doi:10.1155/2022/7153584
Chen, T. Y., Wen, T. K., Dai, N. T., and Hsu, S. H. (2021). Cryogel/hydrogel biomaterials and acupuncture combined to promote diabetic skin wound healing through immunomodulation. Biomaterials 269, 120608. Epub 20201216. doi:10.1016/j.biomaterials.2020.120608
Chmielowiec, J., Borowiak, M., Morkel, M., Stradal, T., Munz, B., Werner, S., et al. (2007). C-met is essential for wound healing in the skin. J. Cell. Biol. 177 (1), 151–162. Epub 20070402. doi:10.1083/jcb.200701086
Corcione, A., Benvenuto, F., Ferretti, E., Giunti, D., Cappiello, V., Cazzanti, F., et al. (2006). Human mesenchymal stem cells modulate B-cell functions. Blood 107 (1), 367–372. Epub 20050901. doi:10.1182/blood-2005-07-2657
da Silva, L. P., Santos, T. C., Rodrigues, D. B., Pirraco, R. P., Cerqueira, M. T., Reis, R. L., et al. (2017). Stem cell-containing hyaluronic acid-based spongy hydrogels for integrated diabetic wound healing. J. Invest. Dermatol. 137 (7), 1541–1551. Epub 20170301. doi:10.1016/j.jid.2017.02.976
da Silva Meirelles, L., Fontes, A. M., Covas, D. T., and Caplan, A. I. (2009). Mechanisms involved in the therapeutic properties of mesenchymal stem cells. Cytokine Growth Factor Rev. 20 (5-6), 419–427. Epub 20091118. doi:10.1016/j.cytogfr.2009.10.002
Dally, J., Khan, J. S., Voisey, A., Charalambous, C., John, H. L., Woods, E. L., et al. (2017). Hepatocyte growth factor mediates enhanced wound healing responses and resistance to transforming growth factor-β1-driven myofibroblast differentiation in oral mucosal fibroblasts. Int. J. Mol. Sci. 18 (9), 1843. Epub 20170824. doi:10.3390/ijms18091843
Demidova-Rice, T. N., Hamblin, M. R., and Herman, I. M. (2012). Acute and impaired wound healing: Pathophysiology and current methods for drug delivery, Part 1: Normal and chronic wounds: Biology, causes, and approaches to care. Adv. Skin. Wound Care 25 (7), 304–314. doi:10.1097/01.ASW.0000416006.55218.d0
Dong, Y., Cui, M., Qu, J., Wang, X., Kwon, S. H., Barrera, J., et al. (2020). Conformable hyaluronic acid hydrogel delivers adipose-derived stem cells and promotes regeneration of burn injury. Acta Biomater. 108, 56–66. Epub 20200403. doi:10.1016/j.actbio.2020.03.040
Dong, Y., Rodrigues, M., Kwon, S. H., Li, X., Sigen, A., Brett, E. A., et al. (2018). Acceleration of diabetic wound regeneration using an in situ-formed stem-cell-based skin substitute. Adv. Healthc. Mat. 7 (17), e1800432. Epub 20180713. doi:10.1002/adhm.201800432
Eke, G., Mangir, N., Hasirci, N., MacNeil, S., and Hasirci, V. (2017). Development of a uv crosslinked biodegradable hydrogel containing adipose derived stem cells to promote vascularization for skin wounds and tissue engineering. Biomaterials 129, 188–198. Epub 20170318. doi:10.1016/j.biomaterials.2017.03.021
Eming, S. A., Martin, P., and Tomic-Canic, M. (2014). Wound repair and regeneration: Mechanisms, signaling, and translation. Sci. Transl. Med. 6 (265), 265sr266. doi:10.1126/scitranslmed.3009337
Farhadihosseinabadi, B., Farahani, M., Tayebi, T., Jafari, A., Biniazan, F., Modaresifar, K., et al. (2018). Amniotic membrane and its epithelial and mesenchymal stem cells as an appropriate source for skin tissue engineering and regenerative medicine. Artif. Cells Nanomed. Biotechnol. 46, 431–440. Epub 20180424. doi:10.1080/21691401.2018.1458730
Frykberg, R. G., and Banks, J. (2015). Challenges in the treatment of chronic wounds. Adv. Wound Care (New. Rochelle. 4 (9), 560–582. doi:10.1089/wound.2015.0635
Gao, Y., Li, Z., Huang, J., Zhao, M., and Wu, J. (2020). In situ formation of injectable hydrogels for chronic wound healing. J. Mat. Chem. B 8 (38), 8768–8780. Epub 20200907. doi:10.1039/d0tb01074j
Garcia, J. R., Quiros, M., Han, W. M., O'Leary, M. N., Cox, G. N., Nusrat, A., et al. (2019). Ifn-gamma-tethered hydrogels enhance mesenchymal stem cell-based immunomodulation and promote tissue repair. Biomaterials 220, 119403. Epub 20190802. doi:10.1016/j.biomaterials.2019.119403
Garg, R. K., Rennert, R. C., Duscher, D., Sorkin, M., Kosaraju, R., Auerbach, L. J., et al. (2014). Capillary force seeding of hydrogels for adipose-derived stem cell delivery in wounds. Stem Cells Transl. Med. 3 (9), 1079–1089. Epub 20140718. doi:10.5966/sctm.2014-0007
Gilmartin, D. J., Soon, A., Thrasivoulou, C., Phillips, A. R., Jayasinghe, S. N., and Becker, D. L. (2016). Sustained release of Cx43 antisense oligodeoxynucleotides from coated collagen scaffolds promotes wound healing. Adv. Healthc. Mat. 5 (14), 1786–1799. Epub 20160602. doi:10.1002/adhm.201600175
Greenhagen, R. M., Frykberg, R. G., and Wukich, D. K. (2019). Serum vitamin D and diabetic foot complications. Diabet. Foot Ankle 10 (1), 1579631. Epub 20190219. doi:10.1080/2000625X.2019.1579631
Guerra, A. D., Rose, W. E., Hematti, P., and Kao, W. J. (2017). Minocycline enhances the mesenchymal stromal/stem cell pro-healing phenotype in triple antimicrobial-loaded hydrogels. Acta Biomater. 51, 184–196. Epub 20170107. doi:10.1016/j.actbio.2017.01.021
Guo, S., and Dipietro, L. A. (2010). Factors affecting wound healing. J. Dent. Res. 89 (3), 219–229. Epub 20100205. doi:10.1177/0022034509359125
Hadisi, Z., Nourmohammadi, J., and Nassiri, S. M. (2018). The antibacterial and anti-inflammatory investigation of lawsonia inermis-gelatin-starch nano-fibrous dressing in burn wound. Int. J. Biol. Macromol. 107, 2008–2019. Epub 20171013. doi:10.1016/j.ijbiomac.2017.10.061
Halim, A. S., Khoo, T. L., and Mohd Yussof, S. J. (2010). Biologic and synthetic skin substitutes: An overview. Indian J. Plast. Surg. 43, S23–S28. doi:10.4103/0970-0358.70712
Hall, C., Hardin, C., Corkins, C. J., Jiwani, A. Z., Fletcher, J., Carlsson, A., et al. (2017). Pathophysiologic mechanisms and current treatments for cutaneous sequelae of burn wounds. Compr. Physiol. 8 (1), 371–405. Epub 20171212. doi:10.1002/cphy.c170016
Han, C., Liu, F., Zhang, Y., Chen, W., Luo, W., Ding, F., et al. (2021). Human umbilical cord mesenchymal stem cell derived exosomes delivered using silk fibroin and sericin composite hydrogel promote wound healing. Front. Cardiovasc. Med. 8, 713021. Epub 20210819. doi:10.3389/fcvm.2021.713021
Hao, R., Cui, Z., Zhang, X., Tian, M., Zhang, L., Rao, F., et al. (2021). Rational design and preparation of functional hydrogels for skin wound healing. Front. Chem. 9, 839055. Epub 20220124. doi:10.3389/fchem.2021.839055
Heher, P., Muhleder, S., Mittermayr, R., Redl, H., and Slezak, P. (2018). Fibrin-based delivery strategies for acute and chronic wound healing. Adv. Drug Deliv. Rev. 129, 134–147. Epub 20171213. doi:10.1016/j.addr.2017.12.007
Hiew, S. H., Wang, J. K., Koh, K., Yang, H., Bacha, A., Lin, J., et al. (2021). Bioinspired short peptide hydrogel for versatile encapsulation and controlled release of growth factor therapeutics. Acta Biomater. 136, 111–123. Epub 20210920. doi:10.1016/j.actbio.2021.09.023
Hwang, N. S., Varghese, S., Lee, H. J., Zhang, Z., Ye, Z., Bae, J., et al. (2008). In vivo commitment and functional tissue regeneration using human embryonic stem cell-derived mesenchymal cells. Proc. Natl. Acad. Sci. U. S. A. 105 (52), 20641–20646. Epub 20081218. doi:10.1073/pnas.0809680106
Jiao, Y., Chen, X., Niu, Y., Huang, S., Wang, J., Luo, M., et al. (2021). Wharton's jelly mesenchymal stem cells embedded in pf-127 hydrogel plus sodium ascorbyl phosphate combination promote diabetic wound healing in type 2 diabetic rat. Stem Cell. Res. Ther. 12 (1), 559. Epub 20211030. doi:10.1186/s13287-021-02626-w
Kharaziha, M., Baidya, A., and Annabi, N. (2021). Rational design of immunomodulatory hydrogels for chronic wound healing. Adv. Mat. 33 (39), e2100176. Epub 20210712. doi:10.1002/adma.202100176
Kirsner, R. S. (2016). The wound healing society chronic wound ulcer healing guidelines update of the 2006 guidelines--blending old with new. Wound Repair Regen. 24 (1), 110–111. Epub 20160107. doi:10.1111/wrr.12393
Kranke, P., Bennett, M. H., Martyn-St James, M., Schnabel, A., Debus, S. E., and Weibel, S. (2015). Hyperbaric oxygen therapy for chronic wounds. Cochrane Database Syst. Rev. 2015 (6), CD004123. Epub 20150624. doi:10.1002/14651858.CD004123.pub4
Kraskiewicz, H., Hinc, P., Krawczenko, A., Bielawska-Pohl, A., Paprocka, M., Witkowska, D., et al. (2021). Hatmsc secreted factors in the hydrogel as a potential treatment for chronic wounds-in vitro study. Int. J. Mol. Sci. 22 (22), 12241. Epub 20211112. doi:10.3390/ijms222212241
Krishnan, S. T., Quattrini, C., Jeziorska, M., Malik, R. A., and Rayman, G. (2007). Neurovascular factors in wound healing in the foot skin of type 2 diabetic subjects. Diabetes Care 30 (12), 3058–3062. Epub 20070926. doi:10.2337/dc07-1421
Krzyszczyk, P., Schloss, R., Palmer, A., and Berthiaume, F. (2018). The role of macrophages in acute and chronic wound healing and interventions topromote pro-wound healing phenotypes. Front Physiol 9, 419. doi:10.3389/fphys.2018.00419
Kucharzewski, M., Rojczyk, E., Wilemska-Kucharzewska, K., Wilk, R., Hudecki, J., and Los, M. J. (2019). Novel trends in application of stem cells in skin wound healing. Eur. J. Pharmacol. 843, 307–315. Epub 20181208. doi:10.1016/j.ejphar.2018.12.012
Las Heras, K., Igartua, M., Santos-Vizcaino, E., and Hernandez, R. M. (2020). Chronic wounds: Current status, available strategies and emerging therapeutic solutions. J. Control. Release 328, 532–550. Epub 20200922. doi:10.1016/j.jconrel.2020.09.039
Laurens, N., Koolwijk, P., and de Maat, M. P. (2006). Fibrin structure and wound healing. J. Thromb. Haemost. 4 (5), 932–939. doi:10.1111/j.1538-7836.2006.01861.x
Lazarus, G. S., Cooper, D. M., Knighton, D. R., Margolis, D. J., Pecoraro, R. E., Rodeheaver, G., et al. (1994). Definitions and guidelines for assessment of wounds and evaluation of healing. Arch. Dermatol. 130 (4), 489–493. doi:10.1001/archderm.130.4.489
Lee, K., Xue, Y., Lee, J., Kim, H. J., Liu, Y., Tebon, P., et al. (2020). A patch of detachable hybrid microneedle depot for localized delivery of mesenchymal stem cells in regeneration therapy. Adv. Funct. Mat. 30 (23), 2000086. Epub 20200427. doi:10.1002/adfm.202000086
Lee, P. Y., Cobain, E., Huard, J., and Huang, L. (2007). Thermosensitive hydrogel peg-plga-peg enhances engraftment of muscle-derived stem cells and promotes healing in diabetic wound. Mol. Ther. 15 (6), 1189–1194. Epub 20070403. doi:10.1038/sj.mt.6300156
Lei, Z., Singh, G., Min, Z., Shixuan, C., Xu, K., Pengcheng, X., et al. (2018). Bone marrow-derived mesenchymal stem cells laden novel thermo-sensitive hydrogel for the management of severe skin wound healing. Mater. Sci. Eng. C 90, 159–167. Epub 20180417. doi:10.1016/j.msec.2018.04.045
Li, B., and Wang, J. H. (2011). Fibroblasts and myofibroblasts in wound healing: Force generation and measurement. J. Tissue Viability 20 (4), 108–120. Epub 20091207. doi:10.1016/j.jtv.2009.11.004
Li, J., Ding, Z., Zheng, X., Lu, G., Lu, Q., and Kaplan, D. L. (2021). Injectable silk nanofiber hydrogels as stem cell carriers to accelerate wound healing. J. Mat. Chem. B 9 (37), 7771–7781. Epub 20210929. doi:10.1039/d1tb01320c
Li, M., Ke, Q. F., Tao, S. C., Guo, S. C., Rui, B. Y., and Guo, Y. P. (2016). Fabrication of hydroxyapatite/chitosan composite hydrogels loaded with exosomes derived from mir-126-3p overexpressed synovial mesenchymal stem cells for diabetic chronic wound healing. J. Mat. Chem. B 4 (42), 6830–6841. Epub 20161012. doi:10.1039/c6tb01560c
Li, Q., Gong, S., Yao, W., Yang, Z., Wang, R., Yu, Z., et al. (2021). Exosome loaded genipin crosslinked hydrogel facilitates full thickness cutaneous wound healing in rat animal model. Drug Deliv. (Lond). 28 (1), 884–893. doi:10.1080/10717544.2021.1912210
Lin, H., Sohn, J., Shen, H., Langhans, M. T., and Tuan, R. S. (2019). Bone marrow mesenchymal stem cells: Aging and tissue engineering applications to enhance bone healing. Biomaterials 203, 96–110. Epub 20180622. doi:10.1016/j.biomaterials.2018.06.026
Lohwasser, C., Neureiter, D., Weigle, B., Kirchner, T., and Schuppan, D. (2006). The receptor for advanced glycation end products is highly expressed in the skin and upregulated by advanced glycation end products and tumor necrosis factor-alpha. J. Invest. Dermatol. 126 (2), 291–299. doi:10.1038/sj.jid.5700070
Lu, L., Yuan, S., Wang, J., Shen, Y., Deng, S., Xie, L., et al. (2018). the formation mechanism of hydrogels. Curr. Stem Cell. Res. Ther. 13 (7), 490–496. doi:10.2174/1574888X12666170612102706
Lu, T. Y., Yu, K. F., Kuo, S. H., Cheng, N. C., Chuang, E. Y., and Yu, J. S. (2020). Enzyme-crosslinked gelatin hydrogel with adipose-derived stem cell spheroid facilitating wound repair in the murine burn model. Polym. (Basel) 12 (12), 2997. Epub 20201216. doi:10.3390/polym12122997
Ma, S., Hu, H., Wu, J., Li, X., Ma, X., Zhao, Z., et al. (2022). Functional extracellular matrix hydrogel modified with msc-derived small extracellular vesicles for chronic wound healing. Cell. Prolif. 55 (4), e13196. Epub 20220214. doi:10.1111/cpr.13196
Malone-Povolny, M. J., Maloney, S. E., and Schoenfisch, M. H. (2019). Nitric oxide therapy for diabetic wound healing. Adv. Healthc. Mat. 8 (12), e1801210. Epub 20190115. doi:10.1002/adhm.201801210
Markeson, D., Pleat, J. M., Sharpe, J. R., Harris, A. L., Seifalian, A. M., and Watt, S. M. (2015). Scarring, stem cells, scaffolds and skin repair. J. Tissue Eng. Regen. Med. 9 (6), 649–668. Epub 20131029. doi:10.1002/term.1841
Martin, P., and Nunan, R. (2015). Cellular and molecular mechanisms of repair in acute and chronic wound healing. Br. J. Dermatol. 173 (2), 370–378. Epub 20150714. doi:10.1111/bjd.13954
Mazini, L., Rochette, L., Admou, B., Amal, S., and Malka, G. (2020). Hopes and limits of adipose-derived stem cells (ADSCs) and mesenchymal stem cells (MSCs) in Wound Healing. Int. J. Mol. Sci. 21 (4). doi:10.3390/ijms21041306
Meier, R. P., Mahou, R., Morel, P., Meyer, J., Montanari, E., Muller, Y. D., et al. (2015). Microencapsulated human mesenchymal stem cells decrease liver fibrosis in mice. J. Hepatology 62 (3), 634–641. Epub 20141030. doi:10.1016/j.jhep.2014.10.030
Miana, V. V., and Gonzalez, E. A. P. (2018). Adipose tissue stem cells in regenerative medicine. Ecancermedicalscience 12, 822. Epub 20180328. doi:10.3332/ecancer.2018.822
Moon, K. C., Suh, H. S., Kim, K. B., Han, S. K., Young, K. W., Lee, J. W., et al. (2019). Potential of allogeneic adipose-derived stem cell-hydrogel complex for treating diabetic foot ulcers. Diabetes 68 (4), 837–846. Epub 20190124. doi:10.2337/db18-0699
Murphy, K. C., Whitehead, J., Zhou, D., Ho, S. S., and Leach, J. K. (2017). Engineering fibrin hydrogels to promote the wound healing potential of mesenchymal stem cell spheroids. Acta Biomater. 64, 176–186. Epub 20171005. doi:10.1016/j.actbio.2017.10.007
Naahidi, S., Jafari, M., Logan, M., Wang, Y., Yuan, Y., Bae, H., et al. (2017). Biocompatibility of hydrogel-based scaffolds for tissue engineering applications. Biotechnol. Adv. 35 (5), 530–544. Epub 20170527. doi:10.1016/j.biotechadv.2017.05.006
Nourian Dehkordi, A., Mirahmadi Babaheydari, F., Chehelgerdi, M., and Raeisi Dehkordi, S. (2019). Skin tissue engineering: Wound healing based on stem-cell-based therapeutic strategies. Stem Cell. Res. Ther. 10 (1), 111. Epub 20190329. doi:10.1186/s13287-019-1212-2
Nuschke, A. (2014). Activity of mesenchymal stem cells in therapies for chronic skin wound healing. Organogenesis 10 (1), 29–37. Epub 20131210. doi:10.4161/org.27405
Oryan, A., Alemzadeh, E., Mohammadi, A. A., and Moshiri, A. (2019). Healing potential of injectable Aloe vera hydrogel loaded by adipose-derived stem cell in skin tissue-engineering in a rat burn wound model. Cell. Tissue Res. 377 (2), 215–227. Epub 20190328. doi:10.1007/s00441-019-03015-9
Randeria, P. S., Seeger, M. A., Wang, X. Q., Wilson, H., Shipp, D., Mirkin, C. A., et al. (2015). Sirna-based spherical nucleic acids reverse impaired wound healing in diabetic mice by ganglioside Gm3 synthase knockdown. Proc. Natl. Acad. Sci. U. S. A. 112 (18), 5573–5578. Epub 20150420. doi:10.1073/pnas.1505951112
Rasool, A., Ata, S., and Islam, A. (2019). Stimuli responsive biopolymer (chitosan) based blend hydrogels for wound healing application. Carbohydr. Polym. 203, 423–429. Epub 20181002. doi:10.1016/j.carbpol.2018.09.083
Richmond, J. M., and Harris, J. E. (2014). Immunology and skin in health and disease. Cold Spring Harb. Perspect. Med. 4 (12), a015339. Epub 20141201. doi:10.1101/cshperspect.a015339
Richmond, N. A., Maderal, A. D., and Vivas, A. C. (2013). Evidence-based management of common chronic lower extremity ulcers. Dermatol. Ther. 26 (3), 187–196. doi:10.1111/dth.12051
Rodgers, K., and Jadhav, S. S. (2018). The application of mesenchymal stem cells to treat thermal and radiation burns. Adv. Drug Deliv. Rev. 123, 75–81. Epub 20171012. doi:10.1016/j.addr.2017.10.003
Rodriguez-Cabello, J. C., Gonzalez de Torre, I., Ibanez-Fonseca, A., and Alonso, M. (2018). Bioactive scaffolds based on elastin-like materials for wound healing. Adv. Drug Deliv. Rev. 129, 118–133. Epub 20180315. doi:10.1016/j.addr.2018.03.003
Safari, B., Aghazadeh, M., Davaran, S., and Roshangar, L. (2022). Exosome-loaded hydrogels: A new cell-free therapeutic approach for skin regeneration. Eur. J. Pharm. Biopharm. 171, 50–59. Epub 20211115. doi:10.1016/j.ejpb.2021.11.002
Samberg, M., Stone, R., Natesan, S., Kowalczewski, A., Becerra, S., Wrice, N., et al. (2019). Platelet rich plasma hydrogels promote in vitro and in vivo angiogenic potential of adipose-derived stem cells. Acta Biomater. 87, 76–87. Epub 20190118. doi:10.1016/j.actbio.2019.01.039
Schafer, M., and Werner, S. (2007). Transcriptional control of wound repair. Annu. Rev. Cell. Dev. Biol. 23, 69–92. doi:10.1146/annurev.cellbio.23.090506.123609
Seeger, M. A., and Paller, A. S. (2015). The roles of growth factors in keratinocyte migration. Adv. Wound Care (New. Rochelle. 4 (4), 213–224. doi:10.1089/wound.2014.0540
Seno, H., Miyoshi, H., Brown, S. L., Geske, M. J., Colonna, M., and Stappenbeck, T. S. (2009). Efficient colonic mucosal wound repair requires Trem2 signaling. Proc. Natl. Acad. Sci. U. S. A. 106 (1), 256–261. Epub 20081224. doi:10.1073/pnas.0803343106
Shafei, S., Khanmohammadi, M., Heidari, R., Ghanbari, H., Taghdiri Nooshabadi, V., Farzamfar, S., et al. (2020). Exosome loaded alginate hydrogel promotes tissue regeneration in full-thickness skin wounds: An in vivo study. J. Biomed. Mat. Res. A 108 (3), 545–556. Epub 20191120. doi:10.1002/jbm.a.36835
Sharma, P., Kumar, A., Dey, A. D., Behl, T., and Chadha, S. (2021). Stem cells and growth factors-based delivery approaches for chronic wound repair and regeneration: A promise to heal from within. Life Sci. 268, 118932. Epub 20210102. doi:10.1016/j.lfs.2020.118932
Shen, Y., Xu, G., Huang, H., Wang, K., Wang, H., Lang, M., et al. (2021). Sequential release of small extracellular vesicles from bilayered thiolated alginate/polyethylene glycol diacrylate hydrogels for scarless wound healing. ACS Nano 15 (4), 6352–6368. Epub 20210316. doi:10.1021/acsnano.0c07714
Shen, Y. I., Cho, H., Papa, A. E., Burke, J. A., Chan, X. Y., Duh, E. J., et al. (2016). Engineered human vascularized constructs accelerate diabetic wound healing. Biomaterials 102, 107–119. Epub 20160604. doi:10.1016/j.biomaterials.2016.06.009
Shi, Y., Hu, G., Su, J., Li, W., Chen, Q., Shou, P., et al. (2010). Mesenchymal stem cells: A new strategy for immunosuppression and tissue repair. Cell. Res. 20 (5), 510–518. Epub 20100406. doi:10.1038/cr.2010.44
Shiekh, P. A., Singh, A., and Kumar, A. (2020). Exosome laden oxygen releasing antioxidant and antibacterial cryogel wound dressing oxoband Alleviate diabetic and infectious wound healing. Biomaterials 249, 120020. Epub 20200413. doi:10.1016/j.biomaterials.2020.120020
Singh, V., Devgan, L., Bhat, S., and Milner, S. M. (2007). The pathogenesis of burn wound conversion. Ann. Plast. Surg. 59 (1), 109–115. doi:10.1097/01.sap.0000252065.90759.e6
Sivaraj, D., Chen, K., Chattopadhyay, A., Henn, D., Wu, W., Noishiki, C., et al. (2021). Hydrogel scaffolds to deliver cell therapies for wound healing. Front. Bioeng. Biotechnol. 9, 660145. Epub 20210503. doi:10.3389/fbioe.2021.660145
Stappenbeck, T. S., and Miyoshi, H. (2009). The role of stromal stem cells in tissue regeneration and wound repair. Science 324 (5935), 1666–1669. doi:10.1126/science.1172687
Su, J., Li, J., Liang, J., Zhang, K., and Li, J. (2021). Hydrogel preparation methods and biomaterials for wound dressing. Life (Basel) 11 (10), 1016. Epub 20210927. doi:10.3390/life11101016
Sun, L., Huang, Y., Bian, Z., Petrosino, J., Fan, Z., Wang, Y., et al. (2016). Sundew-inspired adhesive hydrogels combined with adipose-derived stem cells for wound healing. ACS Appl. Mat. Interfaces 8 (3), 2423–2434. Epub 20160112. doi:10.1021/acsami.5b11811
Tan, J., Li, L., Wang, H., Wei, L., Gao, X., Zeng, Z., et al. (2021). Biofunctionalized fibrin Gel Co-embedded with bmscs and vegf for accelerating skin injury repair. Mater. Sci. Eng. C 121, 111749. Epub 20201127. doi:10.1016/j.msec.2020.111749
Tavakoli, S., and Klar, A. S. (2020). Advanced hydrogels as wound dressings. Biomolecules 10 (8). doi:10.3390/biom10081169
Tian, M. P., Zhang, A. D., Yao, Y. X., Chen, X. G., and Liu, Y. (2021). Mussel-inspired adhesive and polypeptide-based antibacterial thermo-sensitive hydroxybutyl chitosan hydrogel as bmscs 3d culture matrix for wound healing. Carbohydr. Polym. 261, 117878. Epub 20210301. doi:10.1016/j.carbpol.2021.117878
Tracy, L. E., Minasian, R. A., and Caterson, E. J. (2016). Extracellular matrix and dermal fibroblast function in the healing wound. Adv. Wound Care (New. Rochelle. 5 (3), 119–136. doi:10.1089/wound.2014.0561
Tyeb, S., Shiekh, P. A., Verma, V., and Kumar, A. (2020). Adipose-derived stem cells (adscs) loaded gelatin-sericin-laminin cryogels for tissue regeneration in diabetic wounds. Biomacromolecules 21 (2), 294–304. Epub 20191211. doi:10.1021/acs.biomac.9b01355
Varderidou-Minasian, S., and Lorenowicz, M. J. (2020). Mesenchymal stromal/stem cell-derived extracellular vesicles in tissue repair: Challenges and opportunities. Theranostics 10 (13), 5979–5997. Epub 20200501. doi:10.7150/thno.40122
Viezzer, C., Mazzuca, R., Machado, D. C., de Camargo Forte, M. M., and Gomez Ribelles, J. L. (2020). A new waterborne chitosan-based polyurethane hydrogel as a vehicle to transplant bone marrow mesenchymal cells improved wound healing of ulcers in a diabetic rat model. Carbohydr. Polym. 231, 115734. Epub 20191213. doi:10.1016/j.carbpol.2019.115734
Vijayavenkataraman, S., Lu, W. F., and Fuh, J. Y. (2016). 3d bioprinting of skin: A state-of-the-art review on modelling, materials, and processes. Biofabrication 8 (3), 032001. Epub 20160908. doi:10.1088/1758-5090/8/3/032001
Wang, C., Wang, M., Xu, T., Zhang, X., Lin, C., Gao, W., et al. (2019). Engineering bioactive self-healing antibacterial exosomes hydrogel for promoting chronic diabetic wound healing and complete skin regeneration. Theranostics 9 (1), 65–76. Epub 20190101. doi:10.7150/thno.29766
Wang, H., Xu, Z., Zhao, M., Liu, G., and Wu, J. (2021). Advances of hydrogel dressings in diabetic wounds. Biomater. Sci. 9 (5), 1530–1546. doi:10.1039/d0bm01747g
Wang, Y., Beekman, J., Hew, J., Jackson, S., Issler-Fisher, A. C., Parungao, R., et al. (2018). Burn injury: Challenges and advances in burn wound healing, infection, pain and scarring. Adv. Drug Deliv. Rev. 123, 3–17. Epub 20170920. doi:10.1016/j.addr.2017.09.018
Wasiak, J., Cleland, H., Campbell, F., and Spinks, A. (2013). Dressings for superficial and partial thickness burns. Cochrane Database Syst. Rev. 2013 (3), CD002106. Epub 20130328. doi:10.1002/14651858.CD002106.pub4
Watt, F. M., and Driskell, R. R. (2010). The therapeutic potential of stem cells. Phil. Trans. R. Soc. B 365 (1537), 155–163. doi:10.1098/rstb.2009.0149
Werner, S., and Alzheimer, C. (2006). Roles of activin in tissue repair, fibrosis, and inflammatory disease. Cytokine Growth Factor Rev. 17 (3), 157–171. Epub 20060214. doi:10.1016/j.cytogfr.2006.01.001
Werner, S., and Grose, R. (2003). Regulation of wound healing by growth factors and cytokines. Physiol. Rev. 83 (3), 835–870. doi:10.1152/physrev.2003.83.3.835
Wilkinson, H. N., and Hardman, M. J. (2020). Wound healing: Cellular mechanisms and pathological outcomes. Open Biol. 10 (9), 200223. Epub 20200930. doi:10.1098/rsob.200223
Wu, J., Zhang, W., Ran, Q., Xiang, Y., Zhong, J. F., Li, S. C., et al. (2018). The differentiation balance of bone marrow mesenchymal stem cells is crucial to hematopoiesis. Stem Cells Int. 2018, 1–13. Epub 20180403. doi:10.1155/2018/1540148
Wu, Y., Liang, T., Hu, Y., Jiang, S., Luo, Y., Liu, C., et al. (2021). 3d bioprinting of integral adscs-No hydrogel scaffolds to promote severe burn wound healing. Regen. Biomater. 8 (3), rbab014. Epub 20210425. doi:10.1093/rb/rbab014
Xie, Z., Aphale, N. V., Kadapure, T. D., Wadajkar, A. S., Orr, S., Gyawali, D., et al. (2015). Design of antimicrobial peptides conjugated biodegradable citric acid derived hydrogels for wound healing. J. Biomed. Mat. Res. A 103 (12), 3907–3918. Epub 20150825. doi:10.1002/jbm.a.35512
Xu, H., Huang, S., Wang, J., Lan, Y., Feng, L., Zhu, M., et al. (2019). Enhanced cutaneous wound healing by functional injectable thermo-sensitive chitosan-based hydrogel encapsulated human umbilical cord-mesenchymal stem cells. Int. J. Biol. Macromol. 137, 433–441. Epub 20190702. doi:10.1016/j.ijbiomac.2019.06.246
Xu, Z., Han, S., Gu, Z., and Wu, J. (2020). Advances and impact of antioxidant hydrogel in chronic wound healing. Adv. Healthc. Mat. 9 (5), e1901502. Epub 20200124. doi:10.1002/adhm.201901502
Xue, M., Zhao, R., Lin, H., and Jackson, C. (2018). Delivery systems of current biologicals for the treatment of chronic cutaneous wounds and severe burns. Adv. Drug Deliv. Rev. 129, 219–241. Epub 20180319. doi:10.1016/j.addr.2018.03.002
Yang, J., Chen, Z., Pan, D., Li, H., and Shen, J. (2020). <p>Umbilical cord-derived mesenchymal stem cell-derived exosomes combined pluronic F127 hydrogel promote chronic diabetic wound healing and complete skin regeneration</p>. Int. J. Nanomedicine 15, 5911–5926. Epub 20200811. doi:10.2147/IJN.S249129
Yu, J. R., Janssen, M., Liang, B. J., Huang, H. C., and Fisher, J. P. (2020). A liposome/gelatin methacrylate nanocomposite hydrogel system for delivery of stromal cell-derived factor-1α and stimulation of cell migration. Acta Biomater. 108, 67–76. Epub 20200317. doi:10.1016/j.actbio.2020.03.015
Zeng, Q., Qi, X., Shi, G., Zhang, M., and Haick, H. (2022). Wound dressing: From nanomaterials to diagnostic dressings and healing evaluations. ACS Nano 16 (2), 1708–1733. Epub 20220120. doi:10.1021/acsnano.1c08411
Zhang, A., Liu, Y., Qin, D., Sun, M., Wang, T., and Chen, X. (2020). Research status of self-healing hydrogel for wound management: A review. Int. J. Biol. Macromol. 164, 2108–2123. Epub 20200813. doi:10.1016/j.ijbiomac.2020.08.109
Zhang, C., Chen, Y., and Fu, X. (2015). Sweat gland regeneration after burn injury: Is stem cell therapy a new hope? Cytotherapy 17 (5), 526–535. Epub 20141218. doi:10.1016/j.jcyt.2014.10.016
Zhang, L., Zhou, Y., Su, D., Wu, S., Zhou, J., and Chen, J. (2021). Injectable, self-healing and ph responsive stem cell factor loaded collagen hydrogel as a dynamic bioadhesive dressing for diabetic wound repair. J. Mat. Chem. B 9 (29), 5887–5897. doi:10.1039/d1tb01163d
Zhang, Y. S., and Khademhosseini, A. (2017). Advances in engineering hydrogels. Science 356 (6337), eaaf3627. doi:10.1126/science.aaf3627
Zhang, Z., Li, Z., Li, Y., Wang, Y., Yao, M., Zhang, K., et al. (2021). Sodium alginate/collagen hydrogel loaded with human umbilical cord mesenchymal stem cells promotes wound healing and skin remodeling. Cell. Tissue Res. 383 (2), 809–821. Epub 20201107. doi:10.1007/s00441-020-03321-7
Zhong, Y., Xiao, H., Seidi, F., and Jin, Y. (2020). Natural polymer-based antimicrobial hydrogels without synthetic antibiotics as wound dressings. Biomacromolecules 21 (8), 2983–3006. Epub 20200730. doi:10.1021/acs.biomac.0c00760
Keywords: hydrogel, wound healing, stem cell therapy, diabetes, burn
Citation: Li Q, Wang D, Jiang Z, Li R, Xue T, Lin C, Deng Y, Jin Y and Sun B (2022) Advances of hydrogel combined with stem cells in promoting chronic wound healing. Front. Chem. 10:1038839. doi: 10.3389/fchem.2022.1038839
Received: 07 September 2022; Accepted: 07 November 2022;
Published: 28 November 2022.
Edited by:
Jia Zhou, Shanghai Jiao Tong University, ChinaReviewed by:
Mathias Tremp, Hirslanden Private Hospital Group, SwitzerlandKan Zhu, University of California, Davis, United States
Copyright © 2022 Li, Wang, Jiang, Li, Xue, Lin, Deng, Jin and Sun. This is an open-access article distributed under the terms of the Creative Commons Attribution License (CC BY). The use, distribution or reproduction in other forums is permitted, provided the original author(s) and the copyright owner(s) are credited and that the original publication in this journal is cited, in accordance with accepted academic practice. No use, distribution or reproduction is permitted which does not comply with these terms.
*Correspondence: Ye Jin, anljY3VjbUBmb3htYWlsLmNvbQ==; Baozhen Sun, c3VuYnpAamx1LmVkdS5jbg==
†These authors have contributed equally to this work