- Guangxi Key Laboratory of Bioactive Molecules Research and Evaluation, Key Laboratory of Biological Molecular Medicine Research (Guangxi Medical University), Education Department of Guangxi Zhuang Autonomous Region, Pharmaceutical College and School of Basic Medical Sciences, Guangxi Medical University, Nanning, China
The analysis of glyphosate is essential to agricultural production, environment protection and public health. Herein, we proposed a fast and convenient “on-off-on” fluorescence platform for sensitive detection of glyphosate via Cu2+ modulated g-C3N4 nanosheets. The fluorescence of the system was quenched by Cu2+. With the presence of glyphosate, the fluorescence could be restored due to the formation of Cu2+- glyphosate complex. The proposed method was cost-effective with label-free and enzyme-free. Moreover, it exhibits high sensitivity with a low detection limit of 0.01 μg/ml. Furthermore, the proposed method has been successfully monitored glyphosate in real samples.
1 Highlights
• A fast and convenient “on-off-on” fluorescence platform based on Cu2+ modulated g-C3N4 nanosheets for sensitive detection of glyphosate was developed.
• This method is cost-effective and does not need any labeling, enzyme or other complex processes.
• The proposed method exhibits high sensitivity and has a good analysis performance in complex samples.
2 Introduction
The use of pesticides is highly merited to improve crop yields and products quality. Among many pesticides, glyphosate has become one of the most widely applied herbicides because of its high efficiency, broad-spectrum, non-selective, and low toxicity (Valle et al., 2019). Nevertheless, the misuse of glyphosate can cause its high residues in soil, water, and food, and then produce some problems regarding environmental pollution and health hazards. Moreover, glyphosate exposure has adverse effects on the endocrine system, central nervous system and cell cycle (Gasnier et al., 2009; Wozniak et al., 2020). Besides that, glyphosate has been listed as a potential carcinogen (Guyton et al., 2015). Therefore, constructing a facile, low-cost, and high-efficiency method for glyphosate detection is of great importance for public health and environmental protection.
Currently, some traditional approaches have been applied to analyze glyphosate such as capillary electrophoresis (Muñoz et al., 2019), gas chromatography (Royer et al., 2000; Ding et al., 2015), high-performance liquid chromatography (Sun et al., 2017; Surapong and Burakham, 2021), Chromatography-mass spectrometry (Schütze et al., 2021; Pérez-Mayán et al., 2022), and enzyme-linked immunosorbent assay (González-Martínez et al., 2005). However, these methods tend to require sophisticated instrumentation, tedious pretreatments, long testing times or tedious operation. To overcome such limitations, several techniques have been proposed (Xu et al., 2018; Qin et al., 2020; Ding et al., 2021; Wu et al., 2022; Zhao et al., 2022). Among them, fluorescence methods have been receiving great attention owing to their superior analytical performances such as simplistic, rapid, and sensitive. Especially, fluorescent probes can be applied to develop label-free fluorescent platforms for target analysis (Liu et al., 2020; Zhang et al., 2020; Liu et al., 2021).
As a promising kind of 2D nanomaterials, g-C3N4 nanosheets (CN NNS) have attracted much attention because of their low cost, easy synthesis, excellent catalytic performance, metal-free, water solubility and excellent biocompatibility (Dong et al., 2016). Up to now, CN NNS have emerged in biosensor, imaging and photocatalysis (Guo et al., 2011; Salehnia et al., 2017; Zhang et al., 2021; Zheng et al., 2021). Intriguingly, CN NNS not only have excellent fluorescence properties, but also the fluorescence can be quenched by some metal ions including Fe3+, Hg2+, Eu3+, and Cr6+(Rong et al., 2015a; Zhuang et al., 2017; Ti et al., 2021; Wang et al., 2021). Recently, Chen group reported a label-free fluorescence sensor for detection of Fe3+ and ascorbic acid via CN NNS (Guo et al., 2018). Duan group used CN NNS to establish a facile fluorescence approach for 6-Thioguanine and Hg2+ (Duan et al., 2018). This property can be used to develop novel sensor strategies for metal ion detection or some other targets detection which were mediated by these metal ions.
In the present work, a facile and effective “on-off-on” fluorescence sensor based on CN NNS was developed for glyphosate. The CN NNS were prepared by one-step process. The fluorescence of the CN NNS could be quenched by Cu2+. Then, the fluorescence gradually increased with the addition of glyphosate due to Cu2+ preferentially coordinated with glyphosate. The quantitation of glyphosate could be achieved according to the change of fluorescence. Thus, the proposed approach not only provided a novel sensor platform for glyphosate but also exhibited a potential application in environmental safety and biological fields.
3 Materials and methods
3.1 Reagents and materials
Glyphosate, dicyanamide, copper chloride (CuCl2), and other pesticides (such as carbendazim, carbaryl, parathion, malathion, chlorpyrifos, diazinon, omethoate) were gained from Aladdin Reagent Co., Ltd. (Shanghai, China). Tris was purchased from Solarbio Science and Technology Co., Ltd. (Beijing, China). Ultrapure water was gained from a Milli-Q Integral 15 system (Millipore) and used throughout the work. Water samples were obtained from Yongjiang river in Nanning City and the lake water of Guangxi Medical University campus.
3.2 Preparation of CN NNS nanosheets
The CN NNS were synthesized according to the previously reported literature (Rong et al., 2015b). Briefly, the alumina crucible containing 10 g of ground dicyandiamide was placed in a muffle furnace, heated to 550°C at a heating rate of 3°C/min, reacted for 2 h, and then cooled to 25°C at the same rate. As a result, the bulk CN NNS was acquired. After that, 1 g of bulk CN NNS was put into 100 mL10 M HNO3 and refluxed for 16 h at 25°C. Then, the refluxed product was collected through centrifugation at 10000 rpm and washed to neutrality with water. The obtained precipitate was dispersed in 50 ml water for 6 h through a 2D nanomaterial stripper. Finally, the CN NNS solution was stored at 4°C.
3.3 Analysis of glyphosate
First, the stock solution of glyphosate was diluted with water to different concentrations. Then, 2 μL of 0.2 mM Cu2+, 10 μL of different concentrations of glyphosate, 5 μL CN NNS and 183 μL 20 mM Tris-HCl buffer (pH 6.0) reacted at room temperature for 10 min with a total volume of 200 μL. Subsequently, the fluorescence spectra of the samples were collected using an FL-8500 fluorescence spectrometer (PerkinElmer, United States) with the excitation wavelength at 308 nm.
3.4 Determination of glyphosate in real samples
First, the samples of river water and lake water were filtered with 0.22 μm membrane to remove solid impurities, respectively. After that, the analysis of glyphosate in the real water samples were carried out as described above processing procedure (2.3 Analysis of glyphosate).
4 Results and discussion
4.1 Characterization
The morphology of the obtained CN NNS was characterized through transmission electron microscopy (TEM). Figure 1A exhibited a lamellar structure and a well-dispersed state by TEM image. The XRD image displayed a broad diffraction peak (002) at 27.6° in Figure 1B, which was consistent with previous reports (Sun et al., 2020). As shown in Figure 1C, FT-IR spectrum of the obtained CN NNS was analyzed. The broad bands peaked at 3,000 to 3,500 cm−1 were ascribed to N-H stretching. The bands peaked at 1,000 to 1750 cm−1 were attributed to C=N stretching and C=O stretching. A characteristic peak of appeared at 804 cm−1, which was due to the vibration of the triazine ring. The FT-IR results indicated the presence of carboxyl, amino and hydroxy groups.
4.2 Optical properties of CN NNS nanosheets
In this work, the UV-Vis absorption spectroscopy and fluorescence spectra are used to reveal the optical properties of CN NNS. The UV-Vis absorption possessed a characteristic absorption peak at 308 nm in Figure 2A. In addition, Figure 2B shows the solution emitted a strong fluorescence emission at 426 nm with the excitation wavelength at 308 nm. Compared with daylight, brilliant blue fluorescence of the solution was clearly observed under the irradiation of 365 nm UV light (Figure 2C). Meanwhile, the fluorescence stability of the CN NNS dispersion was also researched. As displayed in Figure 2D, there is no obvious change in the intensity after the solution was stored for more than 2 months, implying outstanding stability of the dispersion.
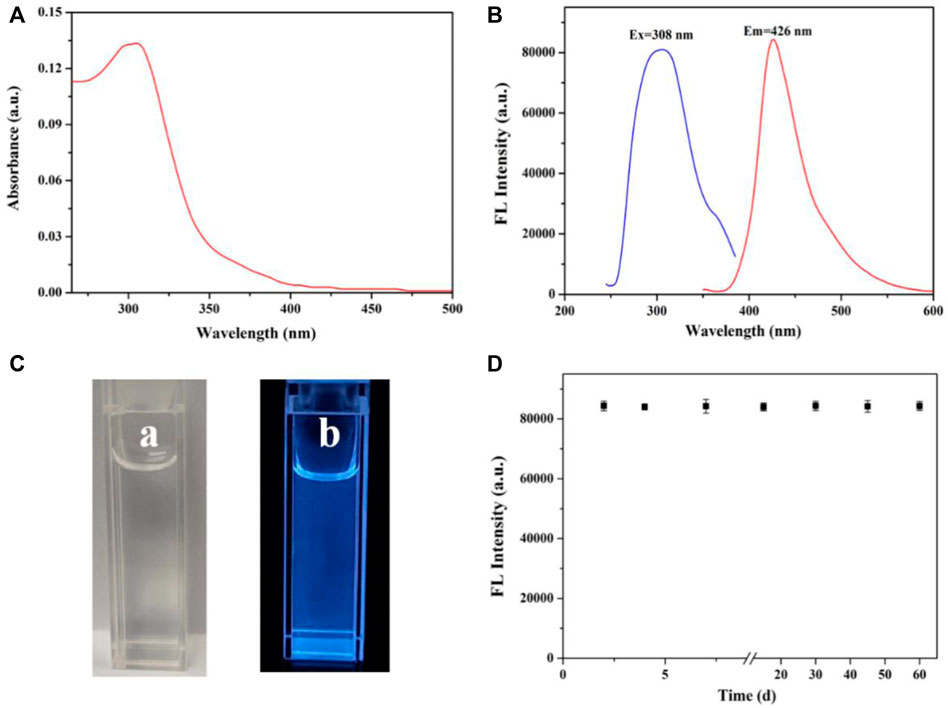
FIGURE 2. (A) UV-Vis spectra of CN NNS; (B) The fluorescence excitation and emission spectra of CN NNS; (C) The photos of the dispersion under room light (A) and 365 nm UV light (B); (D)The fluorescence photostability of the dispersion.
4.3 Principle of the proposed sensor
The principle of this novel enzyme-free fluorescence sensor for glyphosate assay based on CN NNS is illustrated in Figure 3. Firstly, the blue CN NNS were prepared by simple synthesis using dicyanamide. In the absence of glyphosate, the fluorescence of system was greatly quenched by Cu2+, lending to a low fluorescence signal. In contrast, upon the addition of glyphosate, Cu2+ preferentially coordinated with glyphosate to form glyphosate-Cu2+complex due to the stronger interaction than CN NNS-Cu2+. As a result, a high fluorescence signal was obtained. Thus, the concentrations of glyphosate could be detected by the fluorescence change.
4.4 Feasibility of the sensor
In order to verify the feasibility of this sensor strategy, the fluorescence emission spectra of the reaction solutions were detected. Figure 4 showed that the CN NNS had very high current fluorescence (curve a). After the introduction of glyphosate, no obvious change in fluorescence intensity at 426 nm was observed in curve a and curve b, manifesting that glyphosate had no effect on CN NNS. However, when Cu2+ was added to the system, the fluorescence intensity decreased significantly (curve c) due to the formation of the Cu2+-CN NNS complex. After glyphosate and Cu2+ were added, we observed that the fluorescence intensity of system increased significantly (curve d) because glyphosate exhibits a stronger combination ability with Cu2+. Above results illustrated that the fluorescence method using CN NNS and Cu2+ was feasible for glyphosate detection.
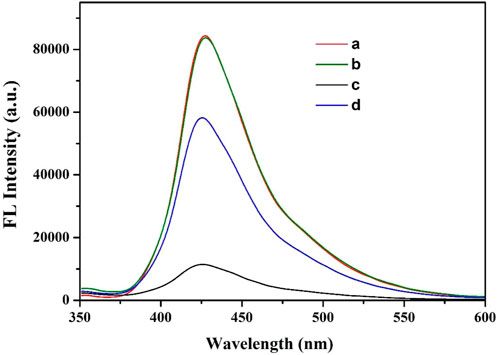
FIGURE 4. Fluorescence emission spectra of different sample solutions. Sample a: CN NNS; Sample b: CN NNS + glyphosate; Sample c: CN NNS + Cu2+; Sample d: CN NNS +Cu2++ glyphosate. The concentrations of CN NNS, Cu2+ and glyphosate were 5 μg/mL, 2 μM and 5 μg/mL, respectively.
4.5 Optimization of assay conditions
For achieving the best assay performances, several main factors including the concentration of Cu2+, pH of reaction system, and the reaction time were optimized.
The concentration of Cu2+ was first investigated. As seen in Figure 5A, the value of F/F0 increases with Cu2+ concentration from 0.5 μM to 2 μM. The maximum F/F0 value was obtained at 2 μM. Afterwards, the value of F/F0 decreased gradually. Thus, 2 μM was the optimal concentrations of Cu2+. In addition, the pH of reaction system was also optimized. The value of F/F0 increased on increasing pH from 4.0 to 6.0, and the F/F0 value reached a maximum when the pH was 6.0. However, after the pH exceeds 6.0, the F/F0 value gradually decreases as the pH value increases (Figure 5B). Thus, 6.0 was used as the optimum pH of the reaction system. Finally, effect of incubation time was also examined. The value of F/F0 increased with the increase reaction time and sustained a stable value at 10 min (Figure 5C). So, the optimal incubation time was 10 min.
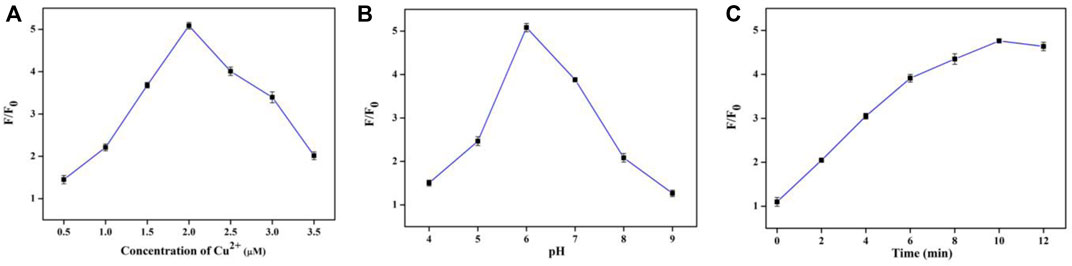
FIGURE 5. Optimization of assay conditions. The effect of Cu2+ concentration (A), pH value (B), and incubation time (C).
4.6 Fluorescence assay for glyphosate
Based on the optimal conditions, various concentrations of glyphosate were analyzed. As illustrated in Figure 6A, the fluorescence intensity at 426 nm increases as the concentration of glyphosate increased from 0 to 8.0 μg/ml. The fluorescence intensity and the glyphosate concentration in the ranged from 0.02 μg/ml to 6.0 μg/ml shows a good linear relationship (inset of Figure 6B). The linear equation was F = 8,814.14C + 13143.35 (C: the concentration of glyphosate, F: the fluorescence intensity at 426 nm, R2 = 09968). The limit of detection is 0.01 μg/ml, which is substantially lower than the maximum residue of 0.7 μg/ml in drinking water by the US EPA and GB5749-2022 (Drinking Water Standards and Health Advisories., 2018; Standardization Administration of China., 2022). The sensitivity is comparable to or better than most other methods for glyphosate assay (Chang et al., 2016; Hou et al., 2020; Tai et al., 2022).
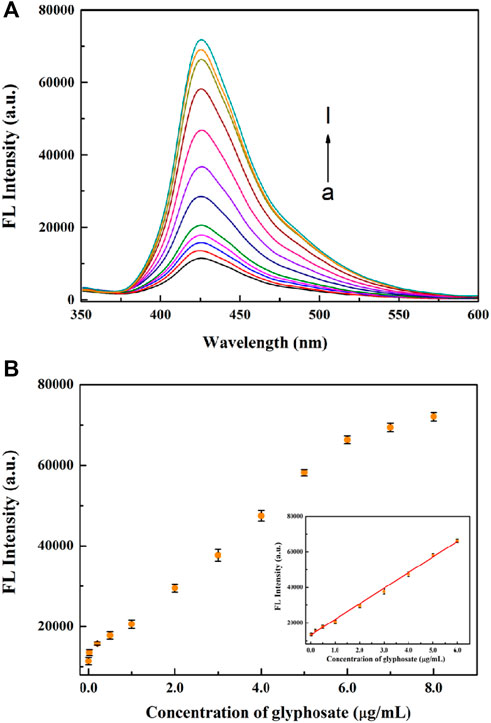
FIGURE 6. (A) The response of fluorescence emission spectra with different concentrations of glyphosate. The concentrations of glyphosate from a to l were 0, 0.02, 0.2, 0.5, 1, 2, 3, 4, 5, 6, 7 and 8 μg/ml, respectively. (B) The relationship between the fluorescence intensity and glyphosate concentrations. Inset: linear curve for glyphosate (0.02–6 μg/ml).
4.7 Selectivity study
The selectivity of this sensor was verified by detecting other pesticides including carbendazim, carbaryl, parathion, malathion, chlorpyrifos, diazinon and omethoate. As displayed in Figure 7, compared to other pesticides, only glyphosate could induce a remarkable fluorescence enhancement. These results indicated that the present assay has good selectivity for glyphosate detection.
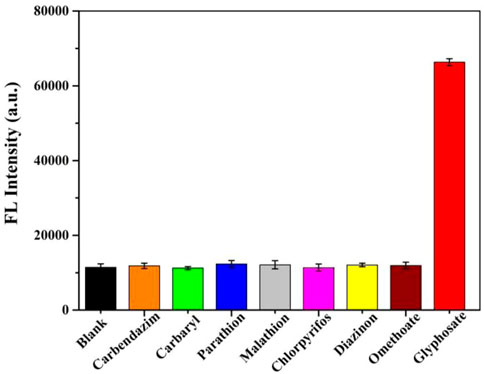
FIGURE 7. Selectivity of the present method for glyphosate. The concentration was 5 μg/ml for glyphosate, and the concentrations were 100 μg/ml for other pesticides.
4.8 Analysis of real samples
To evaluate the applicability of the present method in real samples, the recovery experiments were estimated in river water and lake water samples. The samples were spiked with different concentrations of glyphosate and detected. As can be seen in Table 1, The recoveries ranged from 96.5 to 104.4%. These results indicated the proposed method had great potential for glyphosate detection in complicated real samples.
5 Conclusion
In summary, we constructed a novel “on-off-on” sensor for sensitive detection of glyphosate via CN NNS as fluorescence probe. The fluorescence change of system can be obtained by the combination between copper ions and nanosheets or glyphosate, achieving the detection of glyphosate. The developed method was convenient, low-cost and rapid without tedious procedures. It exhibits a high sensitivity with a detection limit of 0.01 μg/ml. Besides that, a satisfactory performance in actual samples was also obtained. Therefore, the developed approach is expected to possess potential ;application in environmental safety and biological fields.
Data availability statement
The original contributions presented in the study are included in the article/supplementary material, further inquiries can be directed to the corresponding author.
Author contributions
YQ and GY conceived this work. YQ and RH contributed to the experimental efforts and collected the data. YQ and GY drafted and reviewed the manuscript.
Funding
This work was supported by Guangxi Scientific and Technological Base and Personnel Project (Grant No. GUIKEAD22035051); Open Project of Key Laboratory of Longevity and Aging-related Diseases (Guangxi Medical University), Ministry of Education (Grant No. KLLAD202103); The Basic Ability Enhancement Program for Young and Middle-aged Teachers of Guangxi (Grant No. 2022KY0098).
Conflict of interest
The authors declare that the research was conducted in the absence of any commercial or financial relationships that could be construed as a potential conflict of interest.
Publisher’s note
All claims expressed in this article are solely those of the authors and do not necessarily represent those of their affiliated organizations, or those of the publisher, the editors and the reviewers. Any product that may be evaluated in this article, or claim that may be made by its manufacturer, is not guaranteed or endorsed by the publisher.
References
Chang, Y., Zhang, Z., Hao, J., Yang, W., and Tang, J. (2016). A simple label free colorimetric method for glyphosate detection based on the inhibition of peroxidase-like activity of Cu(Ⅱ). Sensors Actuators B Chem. 228, 410–415. doi:10.1016/j.snb.2016.01.048
Ding, J., Guo, H., Liu, W., Zhang, W., and Wang, J. (2015). Current progress on the detection of glyphosate in environmental samples. J. Sci. Appl. Biomed. 3 (6), 88–95.
Ding, S., Lyu, Z., Li, S., Ruan, X., Fei, M., Zhou, Y., et al. (2021). Molecularly imprinted polypyrrole nanotubes based electrochemical sensor for glyphosate detection. Biosens. Bioelectron. X. 191, 113434. doi:10.1016/j.bios.2021.113434
Dong, Y., Wang, Q., Wu, H., Chen, Y., Lu, C-H., Chi, Y., et al. (2016). Graphitic carbon nitride materials: Sensing, imaging and therapy. Small 12 (39), 5376–5393. doi:10.1002/smll.201602056
Drinking Water Standards and Health Advisories (2018). United States environmental protection Agency. Washington, DC: US EPA.
Duan, J., Zhang, Y., Yin, Y., Li, H., Wang, J., and Zhu, L. (2018). A novel “on-off-on” fluorescent sensor for 6-thioguanine and Hg2+ based on g-C3N4 nanosheets. Sensors Actuators B Chem. 257, 504–510. doi:10.1016/j.snb.2017.10.071
Gasnier, C., Dumont, C., Benachour, N., Clair, E., Chagnon, M. C., and Seralini, G. E. (2009). Glyphosate-based herbicides are toxic and endocrine disruptors in human cell lines. Toxicology 262 (3), 184–191. doi:10.1016/j.tox.2009.06.006
González-Martínez, M. A., Brun, E. M., Puchades, R., Maquieira, A., Ramsey, K., and Rubio, F. (2005). Glyphosate immunosensor. Application for water and soil analysis. Anal. Chem. 77 (13), 4219–4227. doi:10.1021/ac048431d
Guo, X., Yue, G., Huang, J., Liu, C., Zeng, Q., and Wang, L. (2018). Label-free simultaneous analysis of Fe(III) and ascorbic acid using fluorescence switching of ultrathin graphitic carbon nitride nanosheets. ACS Appl. Mat. Interfaces 10 (31), 26118–26127. doi:10.1021/acsami.8b10529
Guo, Y., Deng, L., Li, J., Guo, S., Wang, E., and Dong, S. (2011). Hemin-graphene hybrid nanosheets with intrinsic peroxidase-like activity for label-free colorimetric detection of single-nucleotide polymorphism. ACS Nano 5 (2), 1282–1290. doi:10.1021/nn1029586
Guyton, K. Z., Loomis, D., Grosse, Y., Grosse, F. E., Benbrahim-Tallaa, L., Guha, N., et al. (2015). Carcinogenicity of tetrachlorvinphos, parathion, malathion, diazinon, and glyphosate. Lancet Oncol. 16 (5), 490–491. doi:10.1016/S1470-2045(15)70134-8
Hou, J., Wang, X., Lan, S., Zhang, C., Hou, C., He, Q., et al. (2020). A turn-on fluorescent sensor based on carbon dots from Sophora japonica leaves for the detection of glyphosate. Anal. Methods 12, 4130–4138. doi:10.1039/d0ay01241f
Liu, H., Zhang, P., Zhang, C., Chen, Jian., and Jiang, J-H. (2020). Self-assembly of a dual-targeting and self-calibrating ratiometric polymer nanoprobe for accurate hypochlorous acid imaging. ACS Appl. Mat. Interfaces 12 (41), 45822–45829. doi:10.1021/acsami.0c13857
Liu, P., Li, B., Zheng, J., Liang, Q., Wu, C., Huang, L., et al. (2021). A novel N-nitrosation-based ratiometric fluorescent probe for highly selective imaging endogenous nitric oxide in living cells and zebrafish. Sensors Actuators B Chem. 329, 129147. doi:10.1016/j.snb.2020.129147
Muñoz, R., Guevara-Lara, A., Santos, J. L. M., Miranda, J. M., and Rodriguez, J. A. (2019). Determination of glyphosate in soil samples using CdTe/CdS quantum dots in capillary electrophoresis. Microchem. J. 146, 582–587. doi:10.1016/j.microc.2019.01.059
Pérez-Mayán, L., Castro, G., Ramil, M., Cela, R., and Rodríguez, I. (2022). Approaches to liquid chromatography tandem mass spectrometry assessment of glyphosate residues in wine. Anal. Bioanal. Chem. 414, 1445–1455. doi:10.1007/s00216-021-03775-w
Qin, Y., Wu, G., Guo, Y., Ke, D., Yin, J., Wang, D., et al. (2020). Engineered glyphosate oxidase coupled to spore-based chemiluminescence system for glyphosate detection. Anal. Chim. Acta X. 1133, 39–47. doi:10.1016/j.aca.2020.07.077
Rong, M., Lin, L., Song, X., Wang, Y., Zhong, Y., Yan, J., et al. (2015a). Fluorescence sensing of chromium (VI) and ascorbic acid using graphitic carbon nitride nanosheets as a fluorescent "switch. Biosens. Bioelectron. X. 68, 210–217. doi:10.1016/j.bios.2014.12.024
Rong, M., Lin, L., Song, X., Zhao, T., Zhong, Y., Yan, J., et al. (2015b). A label-free fluorescence sensing approach for selective and sensitive detection of 2, 4, 6-trinitrophenol (TNP) in aqueous solution using graphitic carbon nitride nanosheets. Anal. Chem. 87 (2), 1288–1296. doi:10.1021/ac5039913
Royer, A., Beguin, S., Tabet, J. C., Hulot, S., Reding, M. A., and Communal, P. Y. (2000). Determination of glyphosate and aminomethylphosphonic acid residues in water by gas chromatography with tandem mass spectrometry after exchange ion resin purification and derivatization. Application on vegetable matrixes. Anal. Chem. 72 (16), 3826–3832. doi:10.1021/ac000041d
Salehnia, F., Hosseini, M., and Ganjali, M. R. (2017). A fluorometric aptamer based assay for cytochrome C using fluorescent graphitic carbon nitride nanosheets. Microchim. Acta 184, 2157–2163. doi:10.1007/s00604-017-2130-6
Schütze, A., Morales-Agudelo, P., Vidal, M., Calafat, A. M., and Ospina, M. (2021). Quantification of glyphosate and other organophosphorus compounds in human urine via ion chromatography isotope dilution tandem mass spectrometry. Chemosphere 274, 129427. doi:10.1016/j.chemosphere.2020.129427
Standardization Administration of China (2022). Standardization Administration of China. GB 5749-2022. Available at: https://openstd.samr.gov.cn/bzgk/gb/newGbInfo?hcno=99E9C17E3547A3C0CE2FD1FFD9F2F7BE.
Sun, L., Kong, D., Gu, W., Guo, X., Tao, W., Shan, Z., et al. (2017). Determination of glyphosate in soil/sludge by high performance liquid chromatography. J. Chromatogr. A 1502, 8–13. doi:10.1016/j.chroma.2017.04.018
Sun, Y., Zhang, Y., Zhang, H., Liu, M., and Liu, Y. (2020). Integrating highly efficient recognition and signal transition of g-C3N4 embellished Ti3C2 MXene hybrid nanosheets for electrogenerated chemiluminescence analysis of protein kinase activity. Anal. Chem. 92, 10668–10676. doi:10.1021/acs.analchem.0c01776
Surapong, N., and Burakham, R. (2021). Magnetic molecularly imprinted polymer for the selective enrichment of glyphosate, glufosinate, and aminomethylphosphonic acid prior to high-performance liquid chromatography. ACS Omega 6 (41), 27007–27016. doi:10.1021/acsomega.1c03488
Tai, S., Qian, Z., Ren, H., Barimah, A. O., Peng, C., and Wei, X. (2022). Highly selective and sensitive colorimetric detection for glyphosate based on β-CD@DNA-CuNCs enzyme mimics. Anal. Chim. Acta X. 1222, 339992. doi:10.1016/j.aca.2022.339992
Ti, M., Li, Y., Li, Z., Zhao, D., Wu, L., Yuan, L., et al. (2021). A ratiometric nanoprobe based on carboxylated graphitic carbon nitride nanosheets and Eu3+ for the detection of tetracyclines. Analyst 146 (3), 1065–1073. doi:10.1039/d0an01826k
Valle, A. L., Mello, F. C. C., Alves-Balvedi, R. P., Rodrigues, L. P., and Goulart, L. R. (2019). Glyphosate detection: Methods, needs and challenges. Environ. Chem. Lett. 17, 291–317. doi:10.1007/s10311-018-0789-5
Wang, X., Xiong, H., Chen, T., Xu, Y., Bai, G., Zhang, J., et al. (2021). A phosphorus-doped g-C3N4 nanosheets as an efficient and sensitive fluorescent probe for Fe3+ detection. Opt. Mat. (Amst). 119, 111393. doi:10.1016/j.optmat.2021.111393
Wozniak, E., Reszka, E., Jablonska, E., Balcerczyk, A., Broncel, M., and Bukowska, B. (2020). Glyphosate affects methylation in the promoter regions of selected tumor suppressors as well as expression of major cell cycle and apoptosis drivers in PBMCs (in vitro study). Toxicol. Vitro 63, 104736. doi:10.1016/j.tiv.2019.104736
Wu, J., Chen, X., Zhang, Z., and Zhang, J. (2022). “Off-on” fluorescence probe based on green emissive carbon dots for the determination of Cu2+ ions and glyphosate and development of a smart sensing film for vegetable packaging. Microchim. Acta 189 (3), 131. doi:10.1007/s00604-022-05241-5
Xu, M., Gao, Y., Li, Y., Li, X., Zhang, H., Han, X. X., et al. (2018). Indirect glyphosate detection based on ninhydrin reaction and surface-enhanced Raman scattering spectroscopy. Spectrochimica Acta Part A Mol. Biomol. Spectrosc. 197, 78–82. doi:10.1016/j.saa.2018.01.014
Zhang, X., Yang, P., and Jiang, S. P. (2021). The edge-epitaxial growth of yellow g-C3N4 on red g-C3N4 nanosheets with superior photocatalytic activities. Chem. Commun. 57, 3119–3122. doi:10.1039/d1cc00209k
Zhang, Y., Zhang, Y., Yue, Y., Chao, J., Huo, F., and Yin, C. (2020). Based on morpholine as luminescence mechanism regulation and organelle targeting dual function Cys NIR specific biological imaging probe. Sensors Actuators B Chem. 320, 128348. doi:10.1016/j.snb.2020.128348
Zhao, Y., Yan, Y., Liu, C., Zhang, D., Wang, D., Ispas, A., et al. (2022). Plasma-assisted fabrication of molecularly imprinted NiAl-LDH layer on Ni nanorod arrays for glyphosate detection. ACS Appl. Mat. Interfaces 14 (31), 35704–35715. doi:10.1021/acsami.2c08500
Zheng, Y., Liu, Y., Wei, F., Xiao, H., Mou, J., Wu, H., et al. (2021). Functionalized g-C3N4 nanosheets for potential use in magnetic resonance imaging-guided sonodynamic and nitric oxide combination therapy. Acta Biomater. 121, 592–604. doi:10.1016/j.actbio.2020.12.011
Keywords: glyphosate, G-C3N4 nanosheets, Cu2+, fluorescence, on-off-on
Citation: Qin Y, Huang R and Ye G-J (2022) An “on-off-on” fluorescence probe for glyphosate detection based on Cu2+ modulated g-C3N4 nanosheets. Front. Chem. 10:1036683. doi: 10.3389/fchem.2022.1036683
Received: 05 September 2022; Accepted: 14 September 2022;
Published: 30 September 2022.
Edited by:
He Xiaojun, Wenzhou Medical University, ChinaReviewed by:
Yu Huo, Sichuan Normal University, ChinaYou Zhang, Dongguan University of Technology, China
Hua Yao, Nanning Normal University, China
Copyright © 2022 Qin, Huang and Ye. This is an open-access article distributed under the terms of the Creative Commons Attribution License (CC BY). The use, distribution or reproduction in other forums is permitted, provided the original author(s) and the copyright owner(s) are credited and that the original publication in this journal is cited, in accordance with accepted academic practice. No use, distribution or reproduction is permitted which does not comply with these terms.
*Correspondence: Gao-Jie Ye, gaojieygj@163.com