- Department of Pharmacology, School of Pharmacy, Qingdao University Medical College, Qingdao, China
Hydrogen peroxide (H2O2) as a crucial reactive oxygen species (ROS) plays a crucial role in redox signaling in physiological and pathological processes of living cells. Its normal production is closely related to signal transduction of living cells. Overproduction of H2O2 in vivo has been proved to be related to many diseases. Some were developed to reveal the roles of H2O2. However, current fluorescent probes for the detection of H2O2 are restricted in their short emission wavelengths and small Stokes shifts that significantly decrease the sensitivity of detection and cellular visualization. In this work, a novel fluorescent probe BC-B was designed and synthesized with pinacol phenylboronic acid ester as a recognition group and near-infrared fluorophore BC-OH as a reporter group. BC-B probe exhibits a large Stokes shift (122 nm) and near-infrared emission (672 nm), showing an excellent selectivity and sensitivity in detection of H2O2 with the limit of 0.003 μmol/L. Confocal fluorescence imaging further demonstrates that BC-B can be used for detecting endogenous H2O2 in living cells.
Introduction
H2O2 is an important reactive oxygen species (ROS) in living systems (Halliwell et al., 2000; Stone and Yang, 2006). Endogenous H2O2 is mainly produced by NADPH oxidase complexes. Compared with other ROS, H2O2 has a higher concentration and is more stable in vivo. Normal physiological level of H2O2 plays a vital role in cell damage (Jantas et al., 2020), differentiation (Fujita et al., 2021), apoptosis (Quillet-Mary et al., 1997), iron death (Qi et al., 2021) and oxidative stress (Yang L. et al., 2020). Abnormal level of H2O2 is implicated in numerous diseases, such as inflammation (McDonald et al., 2020), neurodegenerative diseases (Fukui and Kato, 2021), diabetes (Wang et al., 2021a), ulcerative colitis (Wang et al., 2020) and cancer (Zoumpourlis et al., 1991; Wang et al., 2021b). Therefore, it is of great significance to develop a high effective method with good sensitivity and selectivity to monitor intracellular H2O2 in biological systems.
Compared with other methods, Fluorescence probe technology used for detection of intracellular H2O2 has the advantages of non-invasiveness and good biocompatibility (An et al., 2020; Zhang et al., 2020; Luo et al., 2021; Xu et al., 2021; Zheng et al., 2021) over the traditional detection assays including chromatography (Tarvin et al., 2011), mass spectrometry (Cocheme et al., 2011), colorimetry (Zou et al., 2019) and electrochemistry (Liu et al., 2014). Many fluorescent probes for H2O2 are developed based on small molecular fluorophores such as rhodamine (Gu et al., 2020), coumarin (Du et al., 2010), naphthalimide (Sun et al., 2013), and BODIPY (Purdey et al., 2018; Wei et al., 2021). However, these fluorescent probes usually have short emission wavelength (<650 nm) and small Stokes shift (<100 nm), which limit their detection of H2O2 in cells or deep tissues. Therefore, it is still necessary to develop a fluorescence probe with large Stokes shift (>100 nm) and near infrared emission (>650 nm) for the detection of intracellular H2O2.
In this study, we designed and synthesized a novel fluorescent probe BC-B using a malononitrile isophorone derivative (BC-OH) that has large Stokes shift and near infrared emission (Yang X. et al., 2020; Ren et al., 2020; Shu et al., 2020; Shiraishi et al., 2021) as a fluorophore and a phenylboronic acid pinacol ester group as a recognition group. BC-B probe exhibits selective and potent detection of H2O2 with a large Stokes shift (122 nm) near infrared emission (672 nm), giving rise to desirable imaging of endogenous H2O2 in living cells.
Materials and methods
Materials and instruments
All reagents were purchased from reagent companies and used directly, if not otherwise specified. The water used in the experiment was double distilled water. UV-Vis spectra were measured with Shimadzu UV 2600. Fluorescence spectra were measured by F-7000 fluorescence spectrophotometer. High-resolution mass spectra of compounds were measured by Agilent Q-TOF6510 spectrograph. The NMR spectra of compounds were recorded by Bruker Advance 500 spectrometer. The pH was measured by PHS-3C. Absorbance for MTT assay was determined by TECAN Austria Gmbh A-5082. Confocal imagings were carried out by Nikon A1R MP.
Synthesis of BC-B
As shown in Scheme 1, compound 1 (2 g, 10.72 mmol) and p-hydroxy benzaldehyde (2 g, 16.3 mmol) were dissolved in 50 ml CH3CN before 2 ml acetic acid and 2 ml piperidine were added. The reaction mixture was heated to 120°C and kept in reflux for 12 h under the protection of argon. The reaction mixture was cooled and the solvent was removed under reduced pressure to give the crude product. Finally, the crude product was purified by silica gel column chromatography to give BC-OH as a red solid.
Under the protection of argon, the fluorophore BC-OH (261 mg, 0.9 mmol), 4-bromomethyl phenylboronic acid pinacol ester (88 mg, 0.296 mmol), anhydrous potassium carbonate (369 mg, 0.89 mmol) and NaI (440 mg, 2.93 mmol) were added into 15 ml anhydrous CH3CN, and reacted at room temperature for 24 h. After completion of the reaction, the solvent was removed by evaporation under reduced pressure. DCM and saturated sodium chloride solution were used to extract repeatedly. The organic phase was collected and dried by anhydrous sodium sulfate. The filtrate was collected after filtration, and the organic solvent was removed under reduced pressure to give crude product, which was further purified by silica gel column chromatography (DCM: PE = 1: 1, v/v), to obtain orange solid (BC-B) (73 mg, yield 48.74%). 1H NMR (500 MHz, DMSO) δ 7.70 (dd, J = 16.2, 8.3 Hz, 4H), 7.47 (d, J = 7.9 Hz, 2H), 7.29 (d, J = 4.6 Hz, 2H), 7.07 (d, J = 8.6 Hz, 2H), 6.85 (s, 1H), 5.22 (s, 2H), 1.31 (s, 14H), 1.03 (s, 6H), 0.89 (s, 2H). 13C NMR (126 MHz, DMSO) δ 160.01, 156.90, 140.66, 138.06, 135.05, 130.09, 129.42, 127.90, 127.33, 122.36, 115.80, 113.70, 84.16, 75.83, 69.58, 42.79, 38.66, 32.16, 27.93, 25.15.
Cytotoxicity test
Cytotoxicity was evaluated in HeLa cells using the MTT assay. HeLa cells were inoculated in culture plate. As adhered to the walls, HeLa cells were incubated with different concentration of BC-B (0, 3, 10, 30 μM) for 24 h. Then, MTT (10 μL) was added and HeLa cells were further cultured for 4 h. Finally, the plate was shaken for about 30 min, and each well was analyzed by the microplate reader (TECAN Austria GmbH A-5082) and detected at the absorbance of 492 nm.
Confocal imaging of H2O2 in cells
Confocal imaging experiments were divided into three groups, each of which is parallel for three times (Ex = 561 nm). In the first group, the HeLa cells were incubated with 2 ml BC-B (10 μmol/L) for 110 min. In the second group, HeLa cells were incubated with 2 ml H2O2 (50 μmol/L) for 30 min and washed with PBS for three times, then incubated with 2 ml BC-B (10 μmol/L) for 110 min. The third group of HeLa cells was incubated with 2 ml H2O2 (50 μmol/L) for 30 min and washed with PBS for three times, then incubated with 2 ml of NAC (1 mmol/L) (NAC is N-Acetyl-l-cysteine, a remover of endogenous H2O2) for 1 h, washed with PBS three times, and then incubated with 2 ml of BC-B (10 μmol/L) for 110 min. In endogenous H2O2 imaging, PMA(1 g/mL)(PMA is Phorbol 12-myristate 13-acetate, a mature H2O2 inducer) is used to replace H2O2 added in the second and third groups of exogenous experiments, stimulate cells to produce endogenous hydrogen peroxide, and study its imaging by BC-B. All cells were washed three times with PBS buffer, and the fluorescence images of cells were observed with confocal fluorescence microscope by Nikon A1R MP.
Results and discussion
Spectral response of BC-B to H2O2
At first, the fluorescence response of BC-B to H2O2 was measured in PBS solution at pH7.4 with excitation of 550 nm. As shown in Figure 1A, the BC-B did not emit fluorescence at 672 nm, suggesting that the phenylboronic acid pinacol ester group of the probe BC-B quenched the fluorescence of BC-OH fluorophore. While H2O2 (50 μmol/L) was added into the BC-B (10 μmol/L) system, the system emitted strong fluorescence at 672 nm with excitation of 550 nm. The emission wavelength of BC-B with H2O2 is the same to that of the fluorophore BC-OH. The results suggested that the probe BC-B can react with H2O2 to release BC-OH fluorophore, showing strong fluorescence. The H2O2 (50 μmol/L) solution pretreated with reactive oxygen scavenger NAC (1 mmol/L) for 5 min was added into BC-B solution (10 μmol/L) and incubated for 110 min, the fluorescence intensity of the system at 672 nm is significantly weaker than that of probe BC-B with H2O2 group, but stronger than that of the free probe BC-B group. When the probe BC-B was incubated with NAC (1 mmol/L) for 110 min, the fluorescence intensity was the same to that of BC-B. As the probe BC-B reacted with H2O2, the UV absorption peak appeared at 550 nm. The UV absorption spectral is similar to that of BC-OH (Supplementary Figure S1). These data further illustrated that H2O2 made BC-B probe release BC-OH fluorophore and showing strong fluorescence, we used HPLC to examine the mechanism of the probe BC-B (Scheme 2 and Supplementary Figure S2).
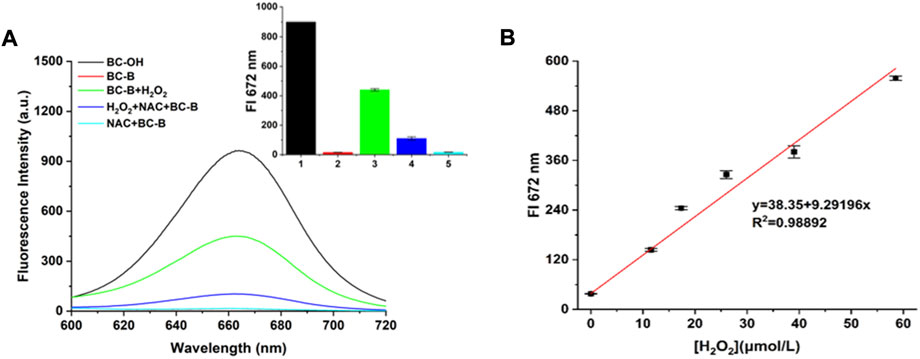
FIGURE 1. Fluorescence spectra of BC-B probe in response to H2O2. (A) The fluorescence spectra of different groups upon excitation using 550 nm, (Black line: BC-OH fluorophore, Red line: BC-B probe, Green line: BC-B probe + H2O2, Blue line: BC-B probe + NAC + H2O2, Blue-green line: BC-B probe + NAC. Inset: fluorescence intensity at 672 nm of different groups. (B) The linearity between the fluorescence intensity of BC-B probe and the concentration of H2O2 from 0 to 60 μmol/L, Ex: 550 nm.
The fluorescence intensity of BC-B system at 672 nm increased with the concentration of H2O2, and a good linearity was built between the fluorescence intensity of BC-B probe and the concentration of H2O2 (y = 38.35 + 9.29196x, R2 = 0.98892) (Supplementary Figure S3A). The limit of detection was calculated to be 3 nmol/L according to the equation LOD = 3σ/k. These results show BC-B has high sensitivity to H2O2 and can quantitatively detect H2O2 in vitro.
Time- and pH-dependent effect of BC-B probe on detecting H2O2
First, the time-dependence of BC-B for detecting H2O2 was evaluated through measuring the fluorescence of BC-B after incubated with or without H2O2 for different time. As shown in Figure 2A and Supplementary Figure S3B, the free probe BC-B shows a little fluorescence in 120 min, indicating that BC-B probe is very stable in PBS solution. As H2O2 was added into the solution of BC-B, the fluorescence intensity (672 nm) of the system increased gradually during the incubation time of 0–110 min, and reached the maximum value at 110 min. Then, we measured the fluorescence changes of BC-B in presence or absence of H2O2 at different pH ranging from 3.4 to 10.5. As shown in Figure 2B and Supplementary Figure S3C, the fluorescence intensity of BC-B (10 μmol/L) at 672 nm was relatively stable with excitation at 550 nm. After incubation of BC-B with H2O2 (20 μmol/L) for 110 min, there was no fluorescence response under acidic conditions, while a good fluorescence response under alkaline conditions (pH = 7.4), this indicated that the BC-B has an ability to detect H2O2 under alkaline conditions.
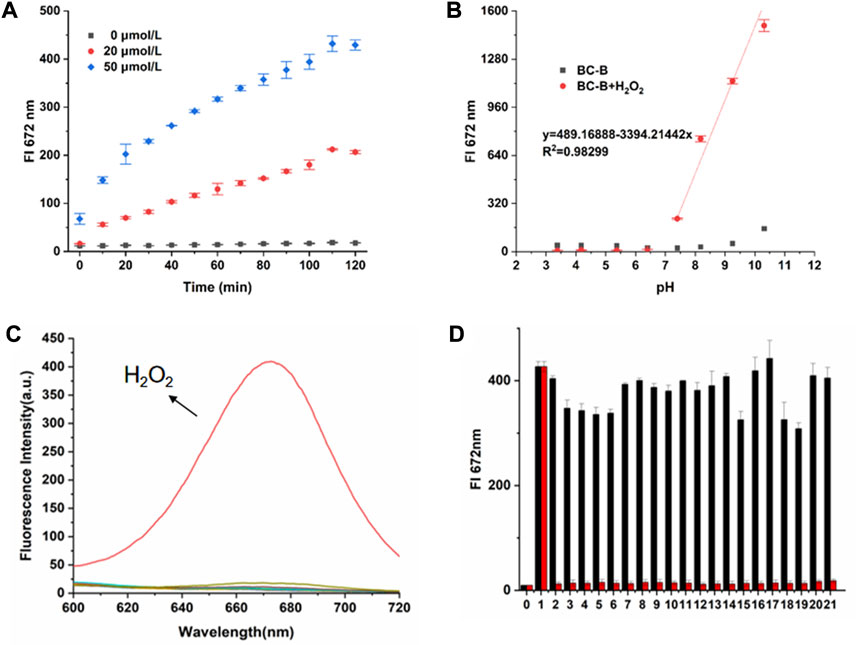
FIGURE 2. Spectral response of BC-B to H2O2. (A) Changes of fluorescence intensity at 672 nm (Ex = 550 nm) during 0–120 min incubation in BC-B system or BC-B system containing H2O2 (20 μmol/L, 50 μmol/L). (B) The changes of fluorescence intensity at 672 nm in BC-B system or BC-B system containing H2O2 after incubation at different pH for 110 min. (C) The fluorescence spectra of the BC-B system containing H2O2 or other analytes after incubation for 110 min (Ex = 550 nm). (D) Selectivity of probe BC-B towards various analytes (Ex = 550 nm). Red: BC-B + H2O2 or other analytes; Black: BC-B + H2O2 and other analytes. (0. BC-B, 1. BC-B + H2O2, 2. NaClO, 3. FeCl3, 4. MgCl2, 5. NaBr, 6. CaCl2, 7. NH4Cl, 8. KI, 9. MgSO4, 10. NaHCO3, 11. L-Pro, 12. L-Arg, 13. β-Ala, 14. glucose, 15. L-Cys, 16. NaNO2, 17. Na2S, 18. NAC, 19. GSH, 20. SNP, 21. C4H10O2).
Selectivity and anti-interference capacity of BC-B
The selectivity of analytes is an important parameter to evaluate property of fluorescent probes. It can be seen from Figure 2C that H2O2 induces a significant increase in the fluorescence intensity of BC-B, while the fluorescence intensity of other active species changes slightly, which indicates that the BC-B can specifically respond to H2O2. Then, add other active species to the solution of BC-B and H2O2 for interference test. Compared with the BC-B probe using H2O2, the fluorescence of these test samples has almost no change, showing good anti-interference ability. These results revealed that BC-B has excellent selectivity for H2O2 and has potential application in visualization of H2O2 in complex cell environment (Figure 2D).
Confocal imaging of HeLa cells for detection of H2O2 level by BC-B
Cell survival percentage was calculated by MTT assay. As shown in Figure 3, the survival percentage of the 0 μmol/L BC-B was considered equal to 1 and the survival percentage of other samples was calculated according to the 0 μmol/L BC-B. High viability values of HeLa cells were obtained when treated with 30 μmol/L BC-B. Cell viability values measured by the MTT assay demonstrate that BC-B has a little cytotoxicity, and has the potential to be used to visualize H2O2 in HeLa.
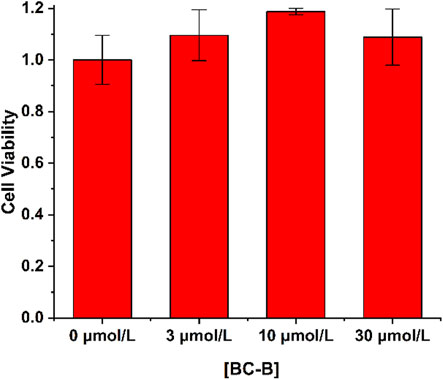
FIGURE 3. The cytotoxicity of BC-B (0–30 μmol/L) against HeLa cells was analyzed by using MTT assay.
Based on the excellent sensitivity, selectivity and biocompatibility of BC-B for H2O2, the ability of BC-B to visualize intracellular H2O2 was tested. As shown in Figure 4, compared with the BC-B group, the fluorescence intensity of the red channel in the BC-B + H2O2 group was significantly enhanced, this result indicates that BC-B can be used to visualize exogenous H2O2 in HeLa cells. After treating H2O2 incubated HeLa cells with active oxygen scavenger NAC, the fluorescence intensity of HeLa cells (NAC + BC-B group) incubated by BC-B was significantly weak comparing to that of BC-B group, which indicates that BC-B can be used to visualize endogenous H2O2 in HeLa cells.
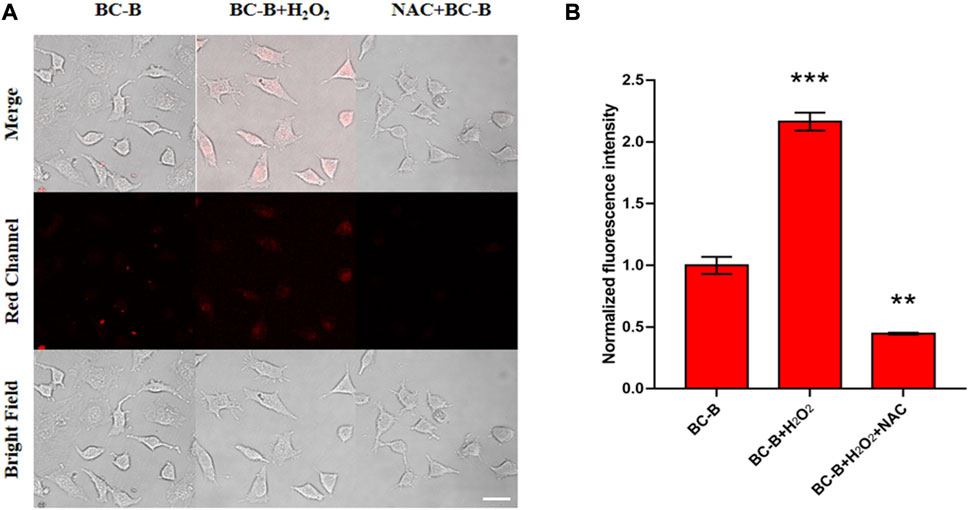
FIGURE 4. Fluorescence images of exogenous H2O2 in HeLa cells. (A) First column (Control group): the cells were treated with BC-B (10 μM) for 110 min. Second column (H2O2 group): the cells were treated with H2O2 (50 μM) for 30 min and then BC-B (10 μM) for 110 min. Third column (BC-B + NAC group): the cells were incubated with H2O2 (50 μM) for 30 min, NAC (1 mM) for 1 h, and then BC-B (10 μM) for 110 min. (B) Bar graph representing the normalized fluorescence intensity of the three groups in panel A, Ex: 561 nm, Em: 663–738 nm; scale bars: 50 μm.
In order to detect endogenous H2O2, PMA is used to stimulate the excessive production of H2O2 in cells. As expected, PMA-treated cells showed brighter fluorescence than untreated cells (Figure 5A). In addition, as shown in the third column of Figure 5A, the bright fluorescence is inhibited after adding NAC. The normalized fluorescence intensity shows the fluorescence change of the three groups (Figure 5B). Therefore, BC-B can image the endogenous H2O2 produced by living cells.
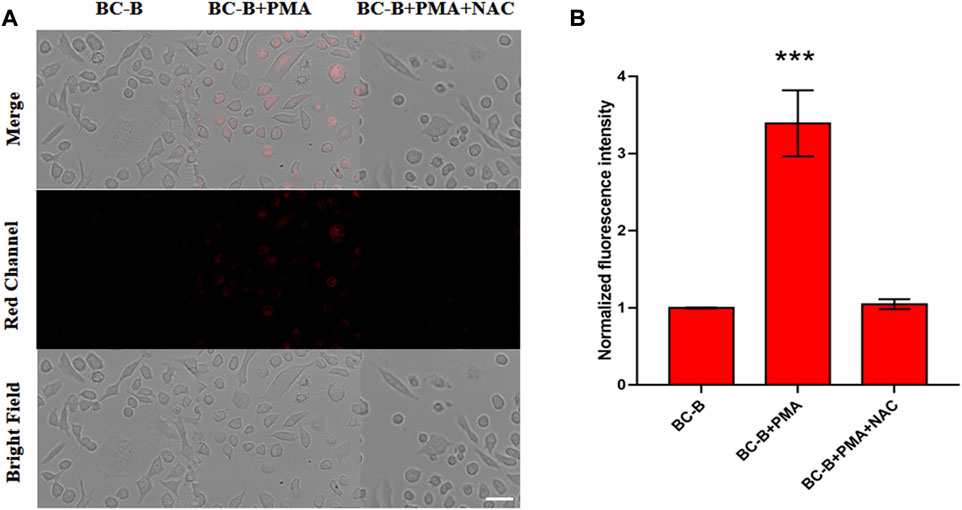
FIGURE 5. Fluorescence images of endogenous H2O2 in HeLa cells. (A) First column (control): the cells were treated with BC-B (10 μM) for 110 min. Second column (PMA group): the cells were treated with PMA (1 μg/ml) for 30 min and then BC-B (10 μM) for 110 min. Third column (PMA + NAC group): the cells were incubated with PMA (1 μg/ml) for 30 min, NAC (1 mM) for 1 h, and then BC-B (10 μM) for 110 min. (B) Bar graph representing the normalized fluorescence intensity of the three groups in panel A, Ex: 561 nm, Em: 663–738 nm; scale bars: 50 μm.
Conclusion
In this study, a near-infrared fluorescent probe BC-B was designed and synthesized for detection of intracellular H2O2 level. The BC-B probe composed of BC-OH as a fluorophore and a phenylboronic acid pinacol ester as a recognition group is featured with a large Stokes shift, low toxicity and high selectivity. Confocal imaging revealed that BC-B probe was capable of detecting and visualizing endogenous or exogenous levels of H2O2 in living HeLa cells.
Data availability statement
The original contributions presented in the study are included in the article/Supplementary Material, further inquiries can be directed to the corresponding author.
Author contributions
NW and YZ designed research; BA and SP performed research; All authors contributed to the writing and review of the manuscript.
Funding
This research was supported by grants awarded to NW from China Postdoctoral Science Foundation (2021M691698) and Shandong Provincial Natural Science Foundation of China (ZR2017BH082).
Conflict of interest
The authors declare that the research was conducted in the absence of any commercial or financial relationships that could be construed as a potential conflict of interest.
Publisher’s note
All claims expressed in this article are solely those of the authors and do not necessarily represent those of their affiliated organizations, or those of the publisher, the editors and the reviewers. Any product that may be evaluated in this article, or claim that may be made by its manufacturer, is not guaranteed or endorsed by the publisher.
Supplementary material
The Supplementary Material for this article can be found online at: https://www.frontiersin.org/articles/10.3389/fchem.2022.1025723/full#supplementary-material
References
An, B., Zhang, H., Peng, J., Zhu, W., Wei, N., and Zhang, Y. (2020). A highly sensitive ratiometric fluorescent probe for imaging endogenous hydrogen sulfide in cells. New J. Chem. 44, 20253–20258. doi:10.1039/d0nj04786d
Cocheme, H. M., Quin, C., Mcquaker, S. J., Cabreiro, F., Logan, A., Prime, T. A., et al. (2011). Measurement of H2O2 within living Drosophila during aging using a ratiometric mass spectrometry probe targeted to the mitochondrial matrix. Cell. Metab. 13, 340–350. doi:10.1016/j.cmet.2011.02.003
Du, L., Ni, N., Li, M., and Wang, B. (2010). A fluorescent hydrogen peroxide probe based on a 'click' modified coumarin fluorophore. Tetrahedron Lett. 51, 1152–1154. doi:10.1016/j.tetlet.2009.12.049
Fujita, H., Mae, K., Nagatani, H., Horie, M., and Nagamori, E. (2021). Effect of hydrogen peroxide concentration on the maintenance and differentiation of cultured skeletal muscle cells. J. Biosci. Bioeng. 131, 572–578. doi:10.1016/j.jbiosc.2020.12.010
Fukui, K., and Kato, Y. (2021). Hydrogen peroxide induces neurite degeneration and its phenomenon relates to neurodegenerative disorders. Free Radic. Biol. Med. 165, 49. doi:10.1016/j.freeradbiomed.2020.12.399
Gu, T., Mo, S., Mu, Y., Huang, X., and Hu, L. (2020). Detection of endogenous hydrogen peroxide in living cells with para-nitrophenyl oxoacetyl rhodamine as turn-on mitochondria-targeted fluorescent probe. Sensors Actuators B Chem. 309, 127731. doi:10.1016/j.snb.2020.127731
Halliwell, B., Clement, M. V., and Long, L. H. (2000). Hydrogen peroxide in the human body. FEBS Lett. 486, 10–13. doi:10.1016/s0014-5793(00)02197-9
Jantas, D., Chwastek, J., Malarz, J., Stojakowska, A., and Lason, W. (2020). Neuroprotective effects of methyl caffeate against hydrogen peroxide-induced cell damage: Involvement of caspase 3 and cathepsin D inhibition. Biomolecules 10, 1530. doi:10.3390/biom10111530
Liu, R., Han, Y., Nie, F., Li, R., and Zheng, J. (2014). Direct electrochemistry of hemoglobin and its biosensing for hydrogen peroxide on TiO2–polystyrene nanofilms. J. Iran. Chem. Soc. 11, 1569–1577. doi:10.1007/s13738-014-0428-4
Luo, J., Zhang, H., Guan, J., An, B., Peng, J., Zhu, W., et al. (2021). Detection of lipase activity in human serum based on a ratiometric fluorescent probe. New J. Chem. 45, 9561–9568. doi:10.1039/d1nj01155c
Mcdonald, K. R., Hernandez-Nichols, A. L., Barnes, J. W., and Patel, R. P. (2020). Hydrogen peroxide regulates endothelial surface N-glycoforms to control inflammatory monocyte rolling and adhesion. Redox Biol. 34, 101498. doi:10.1016/j.redox.2020.101498
Purdey, M. S., Mclennan, H. J., Sutton-Mcdowall, M. L., Drumm, D. W., Zhang, X., Capon, P. K., et al. (2018). Biological hydrogen peroxide detection with aryl boronate and benzil BODIPY-based fluorescent probes. Sensors Actuators B Chem. 262, 750–757. doi:10.1016/j.snb.2018.01.198
Qi, S., Zhang, H., Wang, X., Lv, J., Liu, D., Shen, W., et al. (2021). Development of a NIR fluorescent probe for highly selective and sensitive detection of cysteine in living cells and in vivo. Talanta 234, 122685. doi:10.1016/j.talanta.2021.122685
Quillet-Mary, A., Jaffrezou, J. P., Mansat, V., Bordier, C., Naval, J., and Laurent, G. (1997). Implication of mitochondrial hydrogen peroxide generation in ceramide-induced apoptosis. J. Biol. Chem. 272, 21388–21395. doi:10.1074/jbc.272.34.21388
Ren, H., Huo, F., Zhang, Y., Zhao, S., and Yin, C. (2020). An NIR ESIPT-based fluorescent probe with large Stokes shift for specific detection of Cys and its bioimaging in cells and mice. Sensors Actuators B Chem. 319, 128248. doi:10.1016/j.snb.2020.128248
Shiraishi, Y., Yamada, C., Takagi, S., and Hirai, T. (2021). Fluorometric and colorimetric detection of hypochlorous acid and hypochlorite by a naphthalimide–dicyanoisophorone conjugate. J. Photochem. Photobiol. A Chem. 406, 112997. doi:10.1016/j.jphotochem.2020.112997
Shu, W., Zang, S., Wang, C., Gao, M., Jing, J., and Zhang, X. (2020). An endoplasmic reticulum-targeted ratiometric fluorescent probe for the sensing of hydrogen sulfide in living cells and zebrafish. Anal. Chem. 92, 9982–9988. doi:10.1021/acs.analchem.0c01623
Stone, J. R., and Yang, S. (2006). Hydrogen peroxide: A signaling messenger. Antioxid. Redox Signal. 8, 243–270. doi:10.1089/ars.2006.8.243
Sun, W., Ma, Z., Li, J., Li, W., Du, L., and Li, M. (2013). Bifunctional fluorescent probes for hydrogen peroxide and diols based on a 1, 8-naphthalimide fluorophore. Sci. China Chem. 56, 1440–1445. doi:10.1007/s11426-013-4870-4
Tarvin, M., Mccord, B., Mount, K., and Miller, M. L. (2011). Analysis of hydrogen peroxide field samples by HPLC/FD and HPLC/ED in DC mode. Forensic Sci. Int. 209, 166–172. doi:10.1016/j.forsciint.2011.01.024
Wang, W.-X., Jiang, W.-L., Liu, Y., Li, Y., Zhang, J., and Li, C.-Y. (2020). Near-infrared fluorescence probe with a large Stokes shift for visualizing hydrogen peroxide in ulcerative colitis mice. Sensors Actuators B Chem. 320, 128296. doi:10.1016/j.snb.2020.128296
Wang, W. X., Jiang, W. L., Mao, G. J., Tan, M., Fei, J., Li, Y., et al. (2021a). Monitoring the fluctuation of hydrogen peroxide in diabetes and its complications with a novel near-infrared fluorescent probe. Anal. Chem. 93, 3301–3307. doi:10.1021/acs.analchem.0c05364
Wang, W. X., Jiang, W. L., Mao, G. J., Tan, Z. K., Tan, M., and Li, C. Y. (2021b). A novel near-infrared theranostic probe for accurate cancer chemotherapy in vivo by a dual activation strategy. Chem. Commun. 57, 13768–13771. doi:10.1039/d1cc05864a
Wei, Y. F., Wang, X., Shi, W. J., Chen, R., Zheng, L., Wang, Z. Z., et al. (2021). A novel methylenemalononitrile-BODIPY-based fluorescent probe for highly selective detection of hydrogen peroxide in living cells. Eur. J. Med. Chem. 226, 113828. doi:10.1016/j.ejmech.2021.113828
Xu, L., Zhang, Y., Zhao, L., Han, H., Zhang, S., Huang, Y., et al. (2021). A neoteric dual-signal colorimetric fluorescent probe for detecting endogenous/exogenous hydrogen peroxide in cells and monitoring drug-induced hepatotoxicity. Talanta 233, 122578. doi:10.1016/j.talanta.2021.122578
Yang, L., Zhang, Y., Ren, X., Wang, B., Yang, Z., Song, X., et al. (2020a). Fluorescent detection of dynamic H2O2/H2S redox event in living cells and organisms. Anal. Chem. 92, 4387–4394. doi:10.1021/acs.analchem.9b05270
Yang, X., Han, X., Zhang, Y., Liu, J., Tang, J., Zhang, D., et al. (2020b). Imaging Hg (2+)-induced oxidative stress by NIR molecular probe with "Dual-Key-and-Lock" strategy. Anal. Chem. 92, 12002–12009. doi:10.1021/acs.analchem.0c02509
Zhang, H., Qiao, Z., Wei, N., Zhang, Y., and Wang, K. (2020). A rapid-response and near-infrared fluorescent probe for imaging of nitroxyl in living cells. Talanta 206, 120196. doi:10.1016/j.talanta.2019.120196
Zheng, A., Liu, H., Gao, X., Xu, K., and Tang, B. (2021). A mitochondrial-targeting near-infrared fluorescent probe for revealing the effects of hydrogen peroxide and heavy metal ions on viscosity. Anal. Chem. 93, 9244–9249. doi:10.1021/acs.analchem.1c01511
Zou, J., Cai, H., Wang, D., Xiao, J., Zhou, Z., and Yuan, B. (2019). Spectrophotometric determination of trace hydrogen peroxide via the oxidative coloration of DPD using a Fenton system. Chemosphere 224, 646–652. doi:10.1016/j.chemosphere.2019.03.005
Keywords: hydrogen peroxide, fluorescent probe, near-infrared, large Stokes shift, cellular visualization
Citation: An B, Pang S, Zhang Y and Wei N (2022) A novel near-infrared fluorescent probe for visualization of intracellular hydrogen peroxide. Front. Chem. 10:1025723. doi: 10.3389/fchem.2022.1025723
Received: 25 August 2022; Accepted: 12 October 2022;
Published: 21 October 2022.
Edited by:
Taiping Qing, Xiangtan University, ChinaReviewed by:
Liyi Zhou, Central South University Forestry and Technology, ChinaFabiao Yu, Hainan Medical University, China
Copyright © 2022 An, Pang, Zhang and Wei. This is an open-access article distributed under the terms of the Creative Commons Attribution License (CC BY). The use, distribution or reproduction in other forums is permitted, provided the original author(s) and the copyright owner(s) are credited and that the original publication in this journal is cited, in accordance with accepted academic practice. No use, distribution or reproduction is permitted which does not comply with these terms.
*Correspondence: Ningning Wei, weiningning@qdu.edu.cn