- 1Department of Surgery, the Second Affiliated Hospital of Soochow University, Suzhou, China
- 2Department of Breast Surgery, Affiliated Maternity and Child Health Care Hospital of Nantong University, Nantong, China
- 3Department of Breast Surgery, Shanghai Changning Maternity and Infant Health Hospital, East China Normal University, Shanghai, China
Breast cancer (BC) is one of the most common cancers in women worldwide; however, the successful treatment of BC, especially triple-negative breast cancer (TNBC), remains a significant clinical challenge. Recently, photothermal therapy (PTT), which involves the generation of heat under irradiation to achieve photothermal ablation of BC with minimal invasiveness and outstanding spatial–temporal selectivity, has been demonstrated as a novel therapy that can overcome the drawbacks of chemotherapy or surgery. Significantly, when combining PTT with chemotherapy and/or photodynamic therapy, an enhanced synergistic therapeutic effect can be achieved in both primary and metastatic BC tumors. Thus, this review discusses the recent developments in nanotechnology-based photothermal therapy for the treatment of BC and its metastasis to provide potential strategies for future BC treatment.
Introduction
Breast cancer (BC) is one of the most common malignancies in women worldwide, and the 5-year survival rate of patients with stage III BC is approximately 50%, with an average of only 4.9 years (Gradishar et al., 2020; Siegel et al., 2021; Afzal et al., 2022; An et al., 2022; Luo et al., 2022). Due to genetic susceptibility, environmental factors, lifestyle, etc., there are significant differences in the incidence and mortality of BC across countries (Ginsburg et al., 2017; Hou et al., 2021). Previous studies have demonstrated that about 0.16 million patients were affected by BC in the United States in 2017 (Torre et al., 2016; Li et al., 2020a). Since 2020, there have been 2.3 million new cases of BC worldwide every year, and the total number of BC patients is predicted to increase by 50% by 2040 (Dias et al., 2021; Shao and Varamini, 2022).
At the molecular level, BC is mainly classified into four different subtypes, luminal A, luminal B, HER2-enriched, and triple-negative breast cancer (TNBC), based on the expression of epidermal growth factor receptor 2 (HER2), estrogen receptor alpha (ER), and progesterone receptor (PR) (Dai et al., 2015; Prat et al., 2015; Azamjah et al., 2019). Clinically, ER- and/or PR-positive and HER2-negative tumors are defined as luminal A, ER- and/or PR-positive, and HER-positive with high Ki67 expression is luminal B; HER2 overexpression with ER- and PR-negative is HER2-enriched; and triple-negative breast cancer (TNBC) is a subtype lacking ER, PR, and HER2 expression but with high Ki67 expression (Cancer Genome Atlas, 2012; Nagarajan and McArdle, 2018; Roswall et al., 2018).
Patients diagnosed with different BC subtypes exhibit high heterogeneity in prognosis. In addition to apocrine carcinoma, lobular carcinoma, and metaplastic carcinoma, approximately 90% of TNBC cases exhibit ductal carcinoma infiltration (Gonzalez-Angulo et al., 2011; Mendes et al., 2015; Salgado et al., 2015). Although the 5-year survival rate of patients with TNBC is more than 60%, the median survival of patients with advanced TNBC is only 1 year (Pal et al., 2014; Pawar and Prabhu, 2019). Aside from the lack of targeted therapy, patients with TNBC usually have a higher risk of metastasis with poorly differentiated grades among all BC subtypes (Chen et al., 2022a; Chen et al., 2022b; Tan et al., 2022). Even with the development of magnetic resonance imaging and positron emission tomography with screening programs, the precise diagnosis of TNBC at early stages remains a significant challenge in the clinic because of the aggressiveness and rapid pathological process into advanced stages. These factors significantly reduce the survival rates of patients with TNBC. Therefore, it is important to find an optimal strategy with desirable therapeutic effects for treating TNBC and improving patient prognosis.
Photothermal therapy
The current therapeutic approaches for BC mainly consist of chemotherapy, hormone therapy, and surgery (Aghanejad et al., 2013; Kadkhoda et al., 2022; Zhong et al., 2022). However, disadvantages such as adverse side effects for patients, drug resistance, and residual tumor cells greatly limit their therapeutic effect and may lead to cancer recurrence (Waks and Winer, 2019; Kadkhoda et al., 2021). In addition, surgery can only remove solid tumors from patients in the early stages, and surgical trauma can induce systemic inflammatory responses to promote micrometastatic growth. Photothermal therapy (PTT) has been demonstrated to be an emerging therapeutic method with low toxicity, minimal invasiveness, and outstanding spatial–temporal selectivity, which could overcome these drawbacks (Shakil et al., 2019; Mao and Liu, 2020; Peng et al., 2021). By irradiating photothermal agents under near-infrared (NIR) light, hyperthermia can be triggered to kill cancer cells in target tissues by energy transfer through electron–phonon and electron–electron relaxation of photothermal agents that increase temperature, with both primary tumors and early local metastasis being potential targets. PTT can effectively suppress BC by activating apoptosis, autophagy, or suppressing cell signaling to induce cell death with a shorter treatment time, which reduces patient pain and possesses desirable therapeutic effects with fewer side effects (Kadkhoda et al., 2022). Moreover, when combining PTT with chemotherapy and/or photodynamic therapy, an enhanced synergistic therapeutic effect can be achieved in both primary and metastatic BC tumors (Zhou et al., 2015b; Guo et al., 2015; Lin et al., 2015; Liu et al., 2019; Deng et al., 2021) (Figure 1).
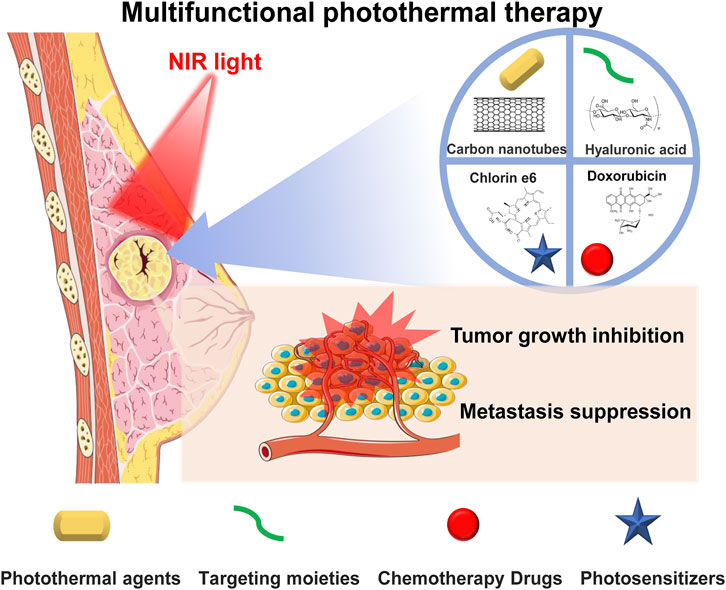
FIGURE 1. Photothermal therapy combined with chemotherapy or/and photodynamic therapy to achieve a synergistic therapeutic effect to inhibit breast cancer primary tumor and metastasis.
Photothermal therapy-triggered apoptosis
Apoptosis is a highly regulated process of cell death distinct from necrosis, which involves intracellular signals, such as DNA damage or growth factor deprivation, and extracellular signals produced during the immune response to cell damage or infection (Pfeffer and Singh, 2018). It has been reported that PTT can also suppress tumors by initiating cell apoptosis via the apoptosis internal pathway, which makes PTT one of the most reliable and powerful methods for cancer treatment (Li et al., 2022).
A recent study has shown that gold nanoparticles (AuNPs) with epidermal growth factor receptor-targeting antibodies may serve as a promising tool for NIR photothermal therapies for cancer via apoptotic pathways. EGFRmAb-conjugated AuNPs exhibited high selectivity and cytotoxicity against cancer cells, where they entered the nucleus. NIR irradiation of AuNPs induced cell apoptosis and DNA damage by inhibiting the PI3K/AKT/mTOR pathway and upregulating the double-strand DNA break repair proteins (Zhang et al., 2018).
Regarding breast cancer treatment, Shang et al. previously reported an AuNP-based theranostic agent synthesized by sequentially coating colloidal polystyrene spheres with polydopamine and AuNPs. This colloidal polydopamine (PDA)/Au agent can not only induce photothermal ablation of breast cancer cells under NIR light but also provide significant enhancement for ultrasound imaging in oncology (Shang et al., 2020).
Shang et al. reported that a combination therapy of PTT and chemotherapy is a promising strategy for breast cancer treatment by equipping gold nanorods (GNRs) with hyaluronic acid/chitosan and doxorubicin via a Schiff base linkage. The hyaluronic acid corona improved the stability of the polysaccharide-based nanoplatforms and allowed for the effective targeting of the CD44 receptor in MCF-7 breast cancer cells. Doxorubicin is released in acidic microenvironments in the tumor to promote pH-responsive drug release behavior. The combined chemo-photothermal therapy exhibited better therapeutic effects than either PTT or doxorubicin individually (Xu et al., 2019).
The overexpression of epidermal growth factor receptor (EGFR) in TNBC enables novel EGFR-targeted therapies for TNBC. Based on clinical ultrasound and photoacoustic imaging, Zhang et al. conjugated gold nanorods with an anti-EGFR antibody to allow for the accurate detection of solid primary tumors and lymph node metastases of TNBC in vivo with efficient NIR photothermal therapy. PTT using anti-EGFR-conjugated gold nanorods can also activate apoptosis in TNBC by upregulating HSP70 and cleaved caspase-3 while suppressing Ki-67 and EGFR (Zhang et al., 2017).
Although PTT can induce cell death pathways, such as apoptosis and necrosis, apoptosis has been regarded as a more effective method to remove cancer cells than necrosis, as it protects the plasma membrane integrity of cells and avoids inflammation. Xing et al. developed coral-shaped Au nanostructures (Au NCs) with a high surface-to-volume ratio to provide better photothermal conversion in the PPT treatment of breast cancer. Under low-power NIR irradiation for 15 min, Au NCs induced apoptosis in MCF-7 cells by upregulating Bax nuclear-encoded proteins and suppressing Bcl-2 protein expression in the apoptotic pathway, which successfully inhibited cancer recurrence in vivo (Xing et al., 2019).
These findings demonstrate that functionalizing photothermal agents with targeted moieties and combining PTT with other effective therapies are gaining increasing attention and have great potential for treating BC.
Photothermal therapy on BC metastasis
Metastasis, including bone, lung, liver, and brain metastases, in BC patients generally results in a poor prognosis, besides removal of the primary tumor (Gong et al., 2017; Ling et al., 2021; Zuo et al., 2022). Among all BC cases, 50% of BC in patients progressed to liver metastases, and there is still no effective therapy to treat liver metastatic estrogen receptor α (ERα)-positive breast cancer, with these patients showing correspondingly poor outcomes (Boudreau et al., 2021; Rashid et al., 2021); however, despite considerable effort, the biological mechanism of metastasis in BC remains unclear. Studies have shown that patients with the HER2-positive or triple-negative subtypes have a significantly higher risk of developing liver metastases than patients with the HR+/HER2-subtype (Kennecke et al., 2010; van de Water et al., 2012; Soni et al., 2015). The reason for this phenomenon may be that HER2 can activate the chemokine receptor CXCR4 and increase the expression of fibroblast growth factor homologous factor (FGF13) to promote the progression of liver metastasis through the CXCL12/CXCR4 pathway in TNBC (Zlotnik et al., 2011; Johnstone et al., 2020). In contrast, long noncoding RNAs, a group of RNAs over 200 nucleotides in length but which lack the ability to code for proteins, have been recently demonstrated to promote invasion and metastasis of cancer cells by initiating the epithelial to the mesenchymal transition process in BC progression (Zhou et al., 2016; Hussen et al., 2021; Hussen et al., 2022). Moreover, as cell adhesion molecules (CAMs) play an important role in cancer cell invasion and metastasis, one study showed that CD44 could regulate PCF11 via the MAPK/ERK pathway or TGF-β signaling pathway to promote metastasis in BC (Ouhtit et al., 2013; Al-Mansoob et al., 2022).
Significantly, hyperthermia has been reported to have a strong capacity to suppress the expression of metastasis-related factors, such as vascular epithelial growth factor, metalloproteinase, and TGF-β1, and hinder the invasion and metastasis of cancer cells (Okuno et al., 2013; Zhou et al., 2015a; Zou et al., 2016; Alamdari et al., 2022). He et al. developed a photothermal nanoplatform by assembling 1,1-dioctadecyl-3,3,3,3-tetramethylindotricarbocyanine iodide (DIR) into an amphiphilic polymer of poly(ethylene glycol)-block-poly (2-diisopropylmethacrylate) for PTT on BC metastasis. This photothermal nanoplatform exhibited strong light-absorbing capability upon 808 nm NIR irradiation, producing hyperthermia to suppress the invasion of metastatic BC cells. Compared to DIR alone, the nanoplatform showed significantly improved accumulation, which facilitated the PTT in inhibiting tumor progression and metastasis in vivo (He et al., 2015).
By simple mixing of an FDA-approved NIR dye, indocyanine green (ICG), with human serum albumin (HSA) and paclitaxel (PTX), biocompatible photothermal nanoparticles can be self-assembled. The ICG moiety can generate mild photothermal heating to improve intracellular uptake in 4T1 murine breast cancer cells upon NIR irradiation to enhance the synergistic therapeutic efficacy. This HSA-ICG–PTX photothermal nanoparticle has excellent capacity to treat primary tumors and, more importantly, suppress lung metastasis (Chen et al., 2015).
Synergistic therapy of photothermal therapy/chemotherapy
Therapies combining PTT with other treatments, such as photodynamic therapy, chemotherapy, and exosome therapy, have attracted the interest of many researchers worldwide owing to their potential to further improve the PTT-based treatment for cueing BC and distant metastasis (Zhou et al., 2015b; Guo et al., 2015; Lin et al., 2015; Liu et al., 2019; Deng et al., 2021). For example, Tian et al. developed a nanoparticle system by loading ICG dye and DOX into porous silicon nanoparticles to achieve chemo-photothermal therapy to inhibit the growth and metastasis of BC. This drug delivery system was equipped with a tumor cell-derived exosome membrane to enhance its accumulation in tumor sites and intracellular uptake by cancer cells. The heat produced by biomimetic nanoparticles under NIR irradiation can effectively accelerate the release of DOX and facilitate tumor ablation to suppress tumor growth and metastasis in a BC tumor-bearing mouse model. This nanosystem may serve as a promising tool for combination therapies for BC (Tian et al., 2020).
Ma et al. developed another drug delivery system based on ICG, HSA, and DOX. This nanoplatform can generate mild hyperthermia to enhance the cellular uptake in cancer cells. Furthermore, it can successfully induce T-cell responses by increasing T-cell permeability and activating cytotoxic T cells to suppress distant metastasis of BC, which demonstrates a promising T-cell response-enhanced chemo-photothermal therapy against BC (Ma et al., 2020).
As P-selectin proteins overexpressed on platelet membranes can bind to CD44 receptors on BC cells, Ye et al. coated PLGA-based photothermal nanoparticles with platelet membranes to enhance the impact of both PTT and chemotherapy. ICG serves as a PTA to provide NIR-induced hyperthermia, while DOX is a chemotherapeutic agent. Favored by the interaction between CD44 receptors on BC cells and P-selectin on nanoplatelets, these PLGA nanoparticles could easily accumulate in MDA-MB231 breast cancer cells and exhibited a strong capacity to inhibit breast cancer metastasis in vivo (Ye et al., 2019).
Synergistic therapy of photothermal therapy/photodynamic therapy
Photodynamic therapy (PDT) has been demonstrated as a minimally invasive method to treat various types of cancer, including breast cancer, melanoma, and lung cancer (Tang et al., 2021; Ding et al., 2022; Jia et al., 2022; Obaid et al., 2022; Pan et al., 2022; Zhang et al., 2022). The photosensitizers can be activated under a light source to generate reactive oxygen species (ROS) to kill cancer cells. In particular, PDT can avoid systemic toxicity with fewer side effects than chemotherapy due to its high spatiotemporal selectivity (Li et al., 2018; Zhang et al., 2019; Nguyen et al., 2022). To achieve synergistic therapeutic effects with different mechanisms for eliminating breast cancer, combination therapies involving both PDT and PTT have been developed (Su et al., 2015; Lee et al., 2022; Xu et al., 2022).
For instance, Li et al. developed a Lyp-1 (CGNKRTRGC)-modified micellar system by stabilizing negatively charged NIR dye-IR820 with cationic PCL-grafted poly (ethylene imine) to produce a combination PTT/PDT therapy for breast cancer. Lyp-1 peptides precisely target the p32 protein overexpressed on breast cancer cells to enhance the targeting effect of the micellar system. Importantly, PTT/PDT/chemotherapy exhibited excellent inhibition of growth and metastasis in a 4T1 cancer model in BALB/c nude mice (Li et al., 2016).
To overcome the drawbacks of drug resistance and insufficient targeting ability in chemotherapy for TNBC, Li et al. developed chlorin e6 (Ce6)-functionalized AuNPs for synergistic PTT/PDT for the treatment of TNBC. By further equipping nanoparticles with cRGD peptides and triphenylphosphonium cationic moieties, the nanosystem can specifically target TNBC cells and mitochondria to enhance their accumulation in tumors, which facilitates hyperthermia and ROS generation under NIR irradiation for synergistic anti-TNBC effects in mice (Li et al., 2021).
Lung metastasis is one of the main causes of breast cancer treatment failure (Guo et al., 2022; Houthuijzen and de Visser, 2022). To achieve desirable metastasis inhibition, Li et al. synthesized theranostic gold nanostars with polydopamine (PDA) and Ce6 conjugation for precise PTT and PDT to inhibit 4T1 tumors and their lung metastasis. These theranostic gold nanostars exhibited excellent stability and photothermal conversion and further possessed simultaneous photoacoustic imaging for an accurate therapeutic strategy (Li et al., 2020b).
Conclusion and future perspectives
Many achievements have been made in the development of intelligent nanosystems with PTT, even when combined with other effective therapies to enhance the therapeutic effect against breast cancer. However, several challenges limiting its efficacy still remain, such as NIR penetration depth, the toxicity of photothermal agents, and thermal resistance. To develop photothermal agents, the cross-sectional area of absorption and photothermal conversion efficiency should be increased. Long-term biosafety, stability, and targeting ability should also be considered to improve the efficacy in vivo. Thus, combination therapy may enable the application of photothermal agents at lower doses; however, the interaction and influence between different components have not yet been elucidated. Furthermore, current strategies have mainly focused on directly killing breast cancer cells in both primary tumors and metastases, whereas the tumor microenvironment plays a significant role in tumor progression. Successful regulation of immune responses in the TME may greatly improve outcomes. In addition, underlying mechanisms and pathways involved in PTT-triggered apoptosis or antimetastasis should be more clearly evaluated. In summary, the design of multifunctional PTT nanotools has been shown to be a promising direction for the successful treatment of BC in the future.
Author contributions
All authors contributed to the design of the study and the writing of the manuscript. JS and HZ undertook the research, wrote the main manuscript text, and prepared figures. WX wrote the manuscript text. JS and G-QJ revised the article critically for important intellectual content and the final approval of the version to be submitted. All authors reviewed the manuscript.
Funding
This work was supported by grants from the National Natural Science Foundation of China (81873730), the medical scientific research project of Jiangsu Commission of Health (Z2020076), and the Preponderant Clinic Discipline Group Project of the Second Affiliated Hospital of Soochow University (XKTJ-XK202009).
Conflict of interest
The authors declare that the research was conducted in the absence of any commercial or financial relationships that could be construed as a potential conflict of interest.
Publisher’s note
All claims expressed in this article are solely those of the authors and do not necessarily represent those of their affiliated organizations, or those of the publisher, the editors, and the reviewers. Any product that may be evaluated in this article, or claim that may be made by its manufacturer, is not guaranteed or endorsed by the publisher.
References
Afzal, S., Hassan, M., Ullah, S., Abbas, H., Tawakkal, F., and Khan, M. A. (2022). Breast cancer; discovery of novel diagnostic biomarkers, drug resistance, and therapeutic implications. Front. Mol. Biosci. 9, 783450. doi:10.3389/fmolb.2022.783450
Aghanejad, A., Jalilian, A. R., Fazaeli, Y., Beiki, D., Fateh, B., and Khalaj, A. (2013). Radiosynthesis and biodistribution studies of [62Zn/62Cu]–plerixafor complex as a novel in vivo PET generator for chemokine receptor imaging. J. Radioanal. Nucl. Chem. 299 (3), 1635–1644. doi:10.1007/s10967-013-2822-2
Al-Mansoob, M., Ahmad, S. M. S., and Ouhtit, A. (2022). PCF11, a novel CD44-downstream transcriptional target, linking its 3'-end polyadenylation function to tumor cell metastasis. Front. Oncol. 12, 878034. doi:10.3389/fonc.2022.878034
Alamdari, S. G., Amini, M., Jalilzadeh, N., Baradaran, B., Mohammadzadeh, R., Mokhtarzadeh, A., et al. (2022). Recent advances in nanoparticle-based photothermal therapy for breast cancer. J. Control. Release 349, 269–303. doi:10.1016/j.jconrel.2022.06.050
An, J., Peng, C., Xie, X., and Peng, F. (2022). New advances in targeted therapy of HER2-negative breast cancer. Front. Oncol. 12, 828438. doi:10.3389/fonc.2022.828438
Azamjah, N., Soltan-Zadeh, Y., and Zayeri, F. (2019). Global trend of breast cancer mortality rate: A 25-year study. Asian pac. J. Cancer Prev. 20 (7), 2015–2020. doi:10.31557/APJCP.2019.20.7.2015
Boudreau, M. W., Duraki, D., Wang, L., Mao, C., Kim, J. E., Henn, M. A., et al. (2021). A small-molecule activator of the unfolded protein response eradicates human breast tumors in mice. Sci. Transl. Med. (603), eabf1383. doi:10.1126/scitranslmed.abf1383
Cancer Genome Atlas, N. (2012). Comprehensive molecular portraits of human breast tumours. Nature 490 (7418), 61–70. doi:10.1038/nature11412
Chen, M., Wu, C., Fu, Z., and Liu, S. (2022). ICAM1 promotes bone metastasis via integrin‐mediated TGF‐β/EMT signaling in triple‐negative breast cancer. Cancer Sci. doi:10.1111/cas.15532
Chen, Q., Liang, C., Wang, C., and Liu, Z. (2015). An imagable and photothermal "Abraxane-like" nanodrug for combination cancer therapy to treat subcutaneous and metastatic breast tumors. Adv. Mat. 27 (5), 903–910. doi:10.1002/adma.201404308
Chen, Y. C., Wu, C. T., Chen, J. H., Tsai, C. F., Wu, C. Y., Chang, P. C., et al. (2022). Diltiazem inhibits breast cancer metastasis via mediating growth differentiation factor 15 and epithelial-mesenchymal transition. Oncogenesis 11 (1), 48. doi:10.1038/s41389-022-00423-5
Dai, X., Li, T., Bai, Z., Yang, Y., Liu, X., Zhan, J., et al. (2015). Breast cancer intrinsic subtype classification, clinical use and future trends. Am. J. Cancer Res. 5 (10), 2929–2943.
Deng, X., Shao, Z., and Zhao, Y. (2021). Solutions to the drawbacks of photothermal and photodynamic cancer therapy. Adv. Sci. (Weinh). 8 (3), 2002504. doi:10.1002/advs.202002504
Dias, C. J., Helguero, L., and Faustino, M. A. F. (2021). Current photoactive molecules for targeted therapy of triple-negative breast cancer. Molecules 26 (24), 7654. doi:10.3390/molecules26247654
Ding, M., Liu, W., and Gref, R. (2022). Nanoscale MOFs: From synthesis to drug delivery and theranostics applications. Adv. Drug Deliv. Rev., 114496. doi:10.1016/j.addr.2022.114496
Ginsburg, O., Bray, F., Coleman, M. P., Vanderpuye, V., Eniu, A., Kotha, S. R., et al. (2017). The global burden of women’s cancers: A grand challenge in global health. Lancet 389 (10071), 847–860. doi:10.1016/s0140-6736(16)31392-7
Gong, Y., Liu, Y. R., Ji, P., Hu, X., and Shao, Z. M. (2017). Impact of molecular subtypes on metastatic breast cancer patients: A SEER population-based study. Sci. Rep. 7, 45411. doi:10.1038/srep45411
Gonzalez-Angulo, A. M., Timms, K. M., Liu, S., Chen, H., Litton, J. K., Potter, J., et al. (2011). Incidence and outcome of BRCA mutations in unselected patients with triple receptor-negative breast cancer. Clin. Cancer Res. 17 (5), 1082–1089. doi:10.1158/1078-0432.CCR-10-2560
Gradishar, W. J., Anderson, B. O., Abraham, J., Aft, R., Agnese, D., Allison, K. H., et al. (2020). Breast cancer, version 3.2020, NCCN clinical practice guidelines in oncology. J. Natl. Compr. Canc. Netw. 18 (4), 452–478. doi:10.6004/jnccn.2020.0016
Guo, C., Yu, H., Feng, B., Gao, W., Yan, M., Zhang, Z., et al. (2015). Highly efficient ablation of metastatic breast cancer using ammonium-tungsten-bronze nanocube as a novel 1064 nm-laser-driven photothermal agent. Biomaterials 52, 407–416. doi:10.1016/j.biomaterials.2015.02.054
Guo, J., Shen, Y., Hu, S., Rui, T., Liu, J., and Yuan, Y. (2022). Neobavaisoflavone inhibits antitumor immunosuppression via myeloid-derived suppressor cells. Int. Immunopharmacol. 111, 109103. doi:10.1016/j.intimp.2022.109103
He, X., Bao, X., Cao, H., Zhang, Z., Yin, Q., Gu, W., et al. (2015). Tumor-penetrating nanotherapeutics loading a near-infrared probe inhibit growth and metastasis of breast cancer. Adv. Funct. Mat. 25 (19), 2831–2839. doi:10.1002/adfm.201500772
Hou, K., Ning, Z., Chen, H., and Wu, Y. (2021). Nanomaterial technology and triple negative breast cancer. Front. Oncol. 11, 828810. doi:10.3389/fonc.2021.828810
Houthuijzen, J. M., and de Visser, K. E. (2022). The lung fibroblast as "soil fertilizer" in breast cancer metastasis. Immunity 55 (8), 1336–1339. doi:10.1016/j.immuni.2022.07.010
Hussen, B. M., Kheder, R. K., Abdullah, S. T., Hidayat, H. J., Rahman, H. S., Salihi, A., et al. (2022). Functional interplay between long non-coding RNAs and Breast CSCs. Cancer Cell Int. 22 (1), 233. doi:10.1186/s12935-022-02653-4
Hussen, B. M., Shoorei, H., Mohaqiq, M., Dinger, M. E., Hidayat, H. J., Taheri, M., et al. (2021). The impact of non-coding RNAs in the epithelial to mesenchymal transition. Front. Mol. Biosci. 8, 665199. doi:10.3389/fmolb.2021.665199
Jia, W., Liu, R., Wang, Y., Hu, C., Yu, W., Zhou, Y., et al. (2022). Dual-responsive nanoparticles with transformable shape and reversible charge for amplified chemo-photodynamic therapy of breast cancer. Acta Pharm. Sin. B 12 (8), 3354–3366. doi:10.1016/j.apsb.2022.03.010
Johnstone, C. N., Pattison, A. D., Harrison, P. F., Powell, D. R., Lock, P., Ernst, M., et al. (2020). FGF13 promotes metastasis of triple-negative breast cancer. Int. J. Cancer 147 (1), 230–243. doi:10.1002/ijc.32874
Kadkhoda, J., Akrami-Hasan-Kohal, M., Tohidkia, M. R., Khaledi, S., Davaran, S., and Aghanejad, A. (2021). Advances in antibody nanoconjugates for diagnosis and therapy: A review of recent studies and trends. Int. J. Biol. Macromol. 185, 664–678. doi:10.1016/j.ijbiomac.2021.06.191
Kadkhoda, J., Tarighatnia, A., Tohidkia, M. R., Nader, N. D., and Aghanejad, A. (2022). Photothermal therapy-mediated autophagy in breast cancer treatment: Progress and trends. Life Sci. 298, 120499. doi:10.1016/j.lfs.2022.120499
Kennecke, H., Yerushalmi, R., Woods, R., Cheang, M. C., Voduc, D., Speers, C. H., et al. (2010). Metastatic behavior of breast cancer subtypes. J. Clin. Oncol. 28 (20), 3271–3277. doi:10.1200/JCO.2009.25.9820
Lee, G., Lee, J. H., Choi, W., Kim, C., and Hahn, S. K. (2022). Hyaluronate-black phosphorus-upconversion nanoparticle complex for non-invasive theranosis of skin cancer. Biomacromolecules. doi:10.1021/acs.biomac.2c00506
Li, B., Zhou, Q., Wang, H., Zha, Y., Zheng, P., Yang, T., et al. (2021). Mitochondria-targeted magnetic gold nanoheterostructure for multi-modal imaging guided photothermal and photodynamic therapy of triple-negative breast cancer. Chem. Eng. J. 403, 126364. doi:10.1016/j.cej.2020.126364
Li, M., Cheng, B., Zeng, W., Chen, S., Tu, M., Wu, M., et al. (2020). Analysis of the risk factors for mortality in adult COVID-19 patients in wuhan: A multicenter study. Front. Med. 7, 545. doi:10.3389/fmed.2020.00545
Li, S., Lui, K. H., Lau, W. S., Chen, J., Lo, W. S., Li, X., et al. (2022). MSOT-guided nanotheranostics for synergistic mild photothermal therapy and chemotherapy to boost necroptosis/apoptosis. ACS Appl. Mat. Interfaces 14 (29), 33712–33725. doi:10.1021/acsami.2c07592
Li, W., Peng, J., Tan, L., Wu, J., Shi, K., Qu, Y., et al. (2016). Mild photothermal therapy/photodynamic therapy/chemotherapy of breast cancer by Lyp-1 modified Docetaxel/IR820 Co-loaded micelles. Biomaterials 106, 119–133. doi:10.1016/j.biomaterials.2016.08.016
Li, X., Kwon, N., Guo, T., Liu, Z., and Yoon, J. (2018). Innovative strategies for hypoxic-tumor photodynamic therapy. Angew. Chem. Int. Ed. 57 (36), 11522–11531. doi:10.1002/anie.201805138
Li, Z., Yang, F., Wu, D., Liu, Y., Gao, Y., Lian, H., et al. (2020). Ce6-Conjugated and polydopamine-coated gold nanostars with enhanced photoacoustic imaging and photothermal/photodynamic therapy to inhibit lung metastasis of breast cancer. Nanoscale 12 (43), 22173–22184. doi:10.1039/d0nr05386d
Lin, Z., Liu, Y., Ma, X., Hu, S., Zhang, J., Wu, Q., et al. (2015). Photothermal ablation of bone metastasis of breast cancer using PEGylated multi-walled carbon nanotubes. Sci. Rep. 5, 11709. doi:10.1038/srep11709
Ling, J., Luo, Y., Sun, C., Dong, Z., Wu, R., Tang, X., et al. (2021). Live intraoperative diagnosis of hepatic metastasis via HDACs targeting molecular theranostic agent. Chem. Eng. J. 406, 126900. doi:10.1016/j.cej.2020.126900
Liu, Y., Bhattarai, P., Dai, Z., and Chen, X. (2019). Photothermal therapy and photoacoustic imaging via nanotheranostics in fighting cancer. Chem. Soc. Rev. 48 (7), 2053–2108. doi:10.1039/c8cs00618k
Luo, X., Zhang, Q., Chen, H., Hou, K., Zeng, N., and Wu, Y. (2022). Smart nanoparticles for breast cancer treatment based on the tumor microenvironment. Front. Oncol. 12, 907684. doi:10.3389/fonc.2022.907684
Ma, H., Yang, X., Ke, J., Wang, C., Peng, L., Hu, F., et al. (2020). Smart assembled human serum albumin nanocarrier enhanced breast cancer treatment and antitumor immunity by chemo- photothermal therapy. ACS Biomater. Sci. Eng. 6 (5), 3217–3229. doi:10.1021/acsbiomaterials.0c00286
Mao, Y., and Liu, X. (2020). Bioresponsive nanomedicine: The next step of deadliest cancers' theranostics. Front. Chem. 8, 257. doi:10.3389/fchem.2020.00257
Mendes, T. F., Kluskens, L. D., and Rodrigues, L. R. (2015). Triple negative breast cancer: Nanosolutions for a big challenge. Adv. Sci. (Weinh). 2 (11), 1500053. doi:10.1002/advs.201500053
Nagarajan, D., and McArdle, S. E. B. (2018). Immune landscape of breast cancers. Biomedicines 6 (1), 20. doi:10.3390/biomedicines6010020
Nguyen, V. N., Zhao, Z., Tang, B. Z., and Yoon, J. (2022). Organic photosensitizers for antimicrobial phototherapy. Chem. Soc. Rev. 51 (9), 3324–3340. doi:10.1039/d1cs00647a
Obaid, G., Bano, S., Thomsen, H., Callaghan, S., Shah, N., Swain, J. W. R., et al. (2022). Remediating desmoplasia with EGFR-targeted photoactivable multi-inhibitor liposomes doubles overall survival in pancreatic cancer. Adv. Sci. (Weinh). 9, e2104594. doi:10.1002/advs.202104594
Okuno, T., Kato, S., Hatakeyama, Y., Okajima, J., Maruyama, S., Sakamoto, M., et al. (2013). Photothermal therapy of tumors in lymph nodes using gold nanorods and near-infrared laser light. J. Control. Release 172 (3), 879–884. doi:10.1016/j.jconrel.2013.10.014
Ouhtit, A., Madani, S., Gupta, I., Shanmuganathan, S., Abdraboh, M. E., Al-Riyami, H., et al. (2013). TGF-β2: A novel target of CD44-promoted breast cancer invasion. J. Cancer 4 (7), 566–572. doi:10.7150/jca.6638
Pal, S., Luchtenborg, M., Davies, E. A., and Jack, R. H. (2014). The treatment and survival of patients with triple negative breast cancer in a London population. Springerplus 3, 553. doi:10.1186/2193-1801-3-553
Pan, D., Zheng, X., Zhang, L., Li, X., Zhu, G., Gong, M., et al. (2022). Synergistic disruption of metabolic homeostasis through hyperbranched poly(ethylene glycol) conjugates as nanotherapeutics to constrain cancer growth. Adv. Mat. 34 (10), e2109036. doi:10.1002/adma.202109036
Pawar, A., and Prabhu, P. (2019). Nanosoldiers: A promising strategy to combat triple negative breast cancer. Biomed. Pharmacother. 110, 319–341. doi:10.1016/j.biopha.2018.11.122
Peng, Z., Yuan, L., XuHong, J., Tian, H., Zhang, Y., Deng, J., et al. (2021). Chiral nanomaterials for tumor therapy: Autophagy, apoptosis, and photothermal ablation. J. Nanobiotechnology 19 (1), 220. doi:10.1186/s12951-021-00965-7
Pfeffer, C. M., and Singh, A. T. K. (2018). Apoptosis: A target for anticancer therapy. Int. J. Mol. Sci. 19 (2), 448. doi:10.3390/ijms19020448
Prat, A., Pineda, E., Adamo, B., Galvan, P., Fernandez, A., Gaba, L., et al. (2015). Clinical implications of the intrinsic molecular subtypes of breast cancer. Breast 24 (2), S26–S35. doi:10.1016/j.breast.2015.07.008
Rashid, N. S., Grible, J. M., Clevenger, C. V., and Harrell, J. C. (2021). Breast cancer liver metastasis: Current and future treatment approaches. Clin. Exp. Metastasis 38 (3), 263–277. doi:10.1007/s10585-021-10080-4
Roswall, P., Bocci, M., Bartoschek, M., Li, H., Kristiansen, G., Jansson, S., et al. (2018). Microenvironmental control of breast cancer subtype elicited through paracrine platelet-derived growth factor-CC signaling. Nat. Med. 24 (4), 463–473. doi:10.1038/nm.4494
Salgado, R., Denkert, C., Demaria, S., Sirtaine, N., Klauschen, F., Pruneri, G., et al. (2015). The evaluation of tumor-infiltrating lymphocytes (TILs) in breast cancer: Recommendations by an international TILs working group 2014. Ann. Oncol. 26 (2), 259–271. doi:10.1093/annonc/mdu450
Shakil, M. S., Hasan, M. A., and Sarker, S. R. (2019). Iron oxide nanoparticles for breast cancer theranostics. Curr. Drug Metab. 20 (6), 446–456. doi:10.2174/1389200220666181122105043
Shang, B., Zhang, X., Ji, R., Wang, Y., Hu, H., Peng, B., et al. (2020). Preparation of colloidal polydopamine/Au hollow spheres for enhanced ultrasound contrast imaging and photothermal therapy. Mater. Sci. Eng. C 106, 110174. doi:10.1016/j.msec.2019.110174
Shao, H., and Varamini, P. (2022). Breast cancer bone metastasis: A narrative review of emerging targeted drug delivery systems. Cells 11 (3), 388. doi:10.3390/cells11030388
Siegel, R. L., Miller, K. D., Fuchs, H. E., and Jemal, A. (2021). Cancer statistics, 2021. Ca. A Cancer J. Clin. 71 (1), 7–33. doi:10.3322/caac.21654
Soni, A., Ren, Z., Hameed, O., Chanda, D., Morgan, C. J., Siegal, G. P., et al. (2015). Breast cancer subtypes predispose the site of distant metastases. Am. J. Clin. Pathol. 143 (4), 471–478. doi:10.1309/AJCPYO5FSV3UPEXS
Su, S., Tian, Y., Li, Y., Ding, Y., Ji, T., Wu, M., et al. (2015). Triple-punch" strategy for triple negative breast cancer therapy with minimized drug dosage and improved antitumor efficacy. ACS Nano 9 (2), 1367–1378. doi:10.1021/nn505729m
Tan, B., Zhou, K., Liu, W., Prince, E., Qing, Y., Li, Y., et al. (2022). RNA N6-methyladenosine reader YTHDC1 is essential for TGF-beta-mediated metastasis of triple negative breast cancer. Theranostics 12 (12), 5727–5743. doi:10.7150/thno.71872
Tang, X., Chen, X., Zhang, S., Gu, X., Wu, R., Huang, T., et al. (2021). Silk‐inspired in situ hydrogel with anti‐tumor immunity enhanced photodynamic therapy for melanoma and infected wound healing. Adv. Funct. Mat. 31 (17), 2101320. doi:10.1002/adfm.202101320
Tian, R., Wang, Z., Niu, R., Wang, H., Guan, W., and Chang, J. (2020). Tumor exosome mimicking nanoparticles for tumor combinatorial chemo-photothermal therapy. Front. Bioeng. Biotechnol. 8, 1010. doi:10.3389/fbioe.2020.01010
Torre, L. A., Siegel, R. L., Ward, E. M., and Jemal, A. (2016). Global cancer incidence and mortality rates and trends-an update. Cancer Epidemiol. Biomarkers Prev. 25 (1), 16–27. doi:10.1158/1055-9965.EPI-15-0578
van de Water, W., Markopoulos, C., van de Velde, C. J., Seynaeve, C., Hasenburg, A., Rea, D., et al. (2012). Association between age at diagnosis and disease-specific mortality among postmenopausal women with hormone receptor-positive breast cancer. JAMA 307 (6), 590–597. doi:10.1001/jama.2012.84
Waks, A. G., and Winer, E. P. (2019). Breast cancer treatment: A review. JAMA 321 (3), 288–300. doi:10.1001/jama.2018.19323
Xing, Y., Kang, T., Luo, X., Zhu, J., Wu, P., and Cai, C. (2019). Coral-shaped Au nanostructures for selective apoptosis induction during photothermal therapy. J. Mat. Chem. B 7 (40), 6224–6231. doi:10.1039/c9tb01503e
Xu, W., Wang, J., Qian, J., Hou, G., Wang, Y., Ji, L., et al. (2019). NIR/pH dual-responsive polysaccharide-encapsulated gold nanorods for enhanced chemo-photothermal therapy of breast cancer. Mater. Sci. Eng. C 103, 109854. doi:10.1016/j.msec.2019.109854
Xu, Z., Chen, J., Li, Y., Hu, T., Fan, L., Xi, J., et al. (2022). Yolk-shell Fe3O4@Carbon@Platinum-Chlorin e6 nanozyme for MRI-assisted synergistic catalytic-photodynamic-photothermal tumor therapy. J. Colloid Interface Sci. 628, 1033–1043. doi:10.1016/j.jcis.2022.08.006
Ye, H., Wang, K., Wang, M., Liu, R., Song, H., Li, N., et al. (2019). Bioinspired nanoplatelets for chemo-photothermal therapy of breast cancer metastasis inhibition. Biomaterials 206, 1–12. doi:10.1016/j.biomaterials.2019.03.024
Zhang, L., Li, Y., Che, W., Zhu, D., Li, G., Xie, Z., et al. (2019). AIE multinuclear Ir(III) complexes for biocompatible organic nanoparticles with highly enhanced photodynamic performance. Adv. Sci. (Weinh). 6 (5), 1802050. doi:10.1002/advs.201802050
Zhang, M., Kim, H. S., Jin, T., Woo, J., Piao, Y. J., and Moon, W. K. (2017). Near-infrared photothermal therapy using anti-EGFR-gold nanorod conjugates for triple negative breast cancer. Oncotarget 8 (49), 86566–86575. doi:10.18632/oncotarget.21243
Zhang, Y., Cong, L., He, J., Wang, Y., Zou, Y., Yang, Z., et al. (2018). Photothermal treatment with EGFRmAb–AuNPs induces apoptosis in hypopharyngeal carcinoma cells via PI3K/AKT/mTOR and DNA damage response pathways. Acta Biochim. Biophys. Sin. (Shanghai). 50 (6), 567–578. doi:10.1093/abbs/gmy046
Zhang, Y., Tian, S., Huang, L., Li, Y., Lu, Y., Li, H., et al. (2022). Reactive oxygen species-responsive and Raman-traceable hydrogel combining photodynamic and immune therapy for postsurgical cancer treatment. Nat. Commun. 13 (1), 4553. doi:10.1038/s41467-022-32160-z
Zhong, P., Yang, B., Pan, F., and Hu, F. (2022). Temporal trends in Black-White disparities in cancer surgery and cancer-specific survival in the United States between 2007 and 2015. Cancer Med. doi:10.1002/cam4.5141
Zhou, F., Li, X., Naylor, M. F., Hode, T., Nordquist, R. E., Alleruzzo, L., et al. (2015). InCVAX--a novel strategy for treatment of late-stage, metastatic cancers through photoimmunotherapy induced tumor-specific immunity. Cancer Lett. 359 (2), 169–177. doi:10.1016/j.canlet.2015.01.029
Zhou, M., Hou, Y., Yang, G., Zhang, H., Tu, G., Du, Y. E., et al. (2016). LncRNA-hh strengthen cancer stem cells generation in twist-positive breast cancer via activation of hedgehog signaling pathway. Stem Cells 34 (1), 55–66. doi:10.1002/stem.2219
Zhou, M., Zhao, J., Tian, M., Song, S., Zhang, R., Gupta, S., et al. (2015). Radio-photothermal therapy mediated by a single compartment nanoplatform depletes tumor initiating cells and reduces lung metastasis in the orthotopic 4T1 breast tumor model. Nanoscale 7 (46), 19438–19447. doi:10.1039/c5nr04587h
Zlotnik, A., Burkhardt, A. M., and Homey, B. (2011). Homeostatic chemokine receptors and organ-specific metastasis. Nat. Rev. Immunol. 11 (9), 597–606. doi:10.1038/nri3049
Zou, L., Wang, H., He, B., Zeng, L., Tan, T., Cao, H., et al. (2016). Current approaches of photothermal therapy in treating cancer metastasis with nanotherapeutics. Theranostics 6 (6), 762–772. doi:10.7150/thno.14988
Keywords: breast cancer, photothermal therapy, accurate therapy, targeted delivery platform, NIR dye, combination therapeutic strategy
Citation: Sun J, Zhao H, Xu W and Jiang G-Q (2022) Recent advances in photothermal therapy-based multifunctional nanoplatforms for breast cancer. Front. Chem. 10:1024177. doi: 10.3389/fchem.2022.1024177
Received: 21 August 2022; Accepted: 02 September 2022;
Published: 19 September 2022.
Edited by:
Zuhai Lei, Fudan University, ChinaReviewed by:
Qinghua Wu, Anhui University of Chinese Medicine, ChinaBang-Ping Jiang, Guangxi Normal University, China
Copyright © 2022 Sun, Zhao, Xu and Jiang. This is an open-access article distributed under the terms of the Creative Commons Attribution License (CC BY). The use, distribution or reproduction in other forums is permitted, provided the original author(s) and the copyright owner(s) are credited and that the original publication in this journal is cited, in accordance with accepted academic practice. No use, distribution or reproduction is permitted which does not comply with these terms.
*Correspondence: Jingjun Sun, c3VuamluZ2p1bjE5ODRAMTYzLmNvbQ==; Guo-Qin Jiang, amlhbmdfZ3VvcWluQDE2My5jb20=
†These authors have contributed equally to this work