- Key Laboratory of Fine Chemicals in Universities of Shandong, Jinan Engineering Laboratory for Multi-scale Functional Materials, School of Chemistry and Chemical Engineering, Qilu University of Technology (Shandong Academy of Sciences), Jinan, China
Multifunctional electrode materials with inherent conductivity have attracted extensive attention in recent years. Two-dimensional (2D) metal telluride nanomaterials are more promising owing to their strong metallic properties and unique physical/chemical merits. In this review, recent advancements in the preparation of 2D metal tellurides and their application in electrode materials are presented. First, the most available preparation methods, such as hydro/solvent thermal, chemical vapor deposition, and electrodeposition, are summarized. Then, the unique performance of metal telluride electrodes in capacitors, anode materials of Li/Na ion batteries, electrocatalysis, and lithium-sulfur batteries are discussed. Finally, significant challenges and opportunities in the preparation and application of 2D metal tellurides are proposed.
1 Introduction
Increasing concerns about scarce resources and global climate issues have promoted the pursuit of clean and renewable energy for all humanity (Goodenough, 2014; Zeng et al., 2019). Many initiatives have attempted to power industrial civilization with renewable energy while ensuring the economic viability of related technologies, such as supercapacitors (SCs), alkali-ion batteries, lithium-sulfur (Li-S) batteries, etc., (Jaiswal, 2017; Zhang and Guo, 2020; Elmorshedy et al., 2021). Since then, electrode materials have emerged as a popular research topic in recent years. Many studies have attempted to improve the electrochemical characteristics of electrode materials by changing their composition, nano/microstructures, electronic properties, and so on (Zhang et al., 2019a; Zheng et al., 2021). However, as electrodes, one of the most important considerations is the inherent conductivity of the material.
Tellurium (Te), a sulfur element, has a higher conductivity compared with sulfur and selenium. It also has strong metallic characteristics, allowing telluride materials to admit more electrolyte ions and increase diffusion kinetics, enhancing energy storage reaction and offering high rate capability of energy storage devices (Kshetri et al., 2021). Owing to the unusual electrical structures and various two-dimensional (2D) crystals of 2D metal tellurides, these materials have recently received widespread attention as an essential component of metal chalcogenides (Li et al., 2016; Apte et al., 2018; Kononov et al., 2020; Liu et al., 2020). For instance, VTe2 has excellent electrocatalytic activity for hydrogen evolution reactions and is regarded as a high-performance electrode material (Shi et al., 2021). In addition, NiTe, which has better conductivity and faster electron transfer capability compared with semiconductors, can maintain a specific capacity of approximately 307 mAh g−1 at a high rate of 500 mA g−1 as the anode of rechargeable aluminum ion batteries (Yu et al., 2020). Thus, the study of cathode materials for aluminum ion batteries and their use as battery anode materials [e.g., FeTe2 (Park and Kang, 2020) and CoTe2 (Yang et al., 2020)], electrocatalytic materials [e.g., Ni3Te2 (De Silva et al., 2018) and MoTe2 (Zhou et al., 2017a)], and SC materials [e.g., NiTe (Chen et al., 2019) and CoTe2 (Manikandan et al., 2020)] is of great importance.
In recent years, tellurides have become widely used in electrochemistry owing to their 2D layered structure and unique properties (Wang et al., 2019; Li et al., 2020a; Myung et al., 2020; Zhang et al., 2021). On this basis, we provide a comprehensive overview of tellurides in this study, as shown in Figure 1. We first focus on the preparation methods of telluride electrode materials, including chemical vapor deposition (CVD) (Wood et al., 2014; Zhou et al., 2017b; Tang et al., 2019; Hao et al., 2021; Zhang et al., 2021; Zhou et al., 2021), hydrothermal method (Wang et al., 2012; Hou et al., 2013; Oh et al., 2020; Qi et al., 2021), and electrodeposition (Morris and Vanderveen, 1993; Yu et al., 2018), and their electrochemical properties and applications. Subsequently, conceivable perspectives on the challenges and opportunities of 2D telluride electrode materials are proposed to provide insights into future research.
2 Preparation
2D metal tellurides have recently become popular in energy devices such as SCs, photocatalysts, and electrode materials. Thus far, several preparation methods have been developed to synthesize 2D metal telluride nanomaterials, as summarized in Table 1.
2.1 Hydro/solvothermal methods
The most prevalent techniques for synthesizing nanomaterials are the hydrothermal and solvothermal procedures. In contrast to alternative procedures for synthesizing nanostructured materials, the hydrothermal approach offers the benefits of low synthesis temperature and small grain size. This approach may produce a wide range of morphologies, including nanorods (Yu et al., 2020; Jayababu et al., 2021), nanosheets (Lu et al., 2015; Li et al., 2020b), nanowires (Yang et al., 2009; Wan et al., 2011), and nanotubes (Wang et al., 2010). Moreover, in contrast to other synthesis methods, the hydrothermal approach has an excessively high oxygen affinity for positively charged metal and negatively charged Te ions, resulting in the formation of oxides. Reducing agents, such as hydrazine and sodium borohydride, are necessary to avoid oxide impurities in tellurides.
Wang et al. adopted the hydrothermal technique to synthesize Bi2Te3 nanotubes with diameters of 100 nm and lengths of 500–1,000 nm by using sodium borohydride as the reducing agent and EDTA as the surfactant (Figure 2A–D) (Wang et al., 2010). Zhang et al. created carbon-encapsulated porous Sb2Te3 nanoplates with porous architectures via the hydrothermal and carbonization processes, with carbon shell thicknesses ranging from 50 to 80 nm (Figures 2E–J) (Zhang et al., 2019b). Their approach can successfully produce nanomaterials, such as Ag2Te (Zhang et al., 2006), Bi2Te3 (Wang et al., 2010), CdTe (Gong et al., 2011), Cu2Te (Zhang et al., 2006), Cu2-xTe (Jamwal et al., 2016a), HgTe (Salavati-Niasari et al., 2010), NiTe (Zhang et al., 2002), and PbTe (Jamwal et al., 2016b).
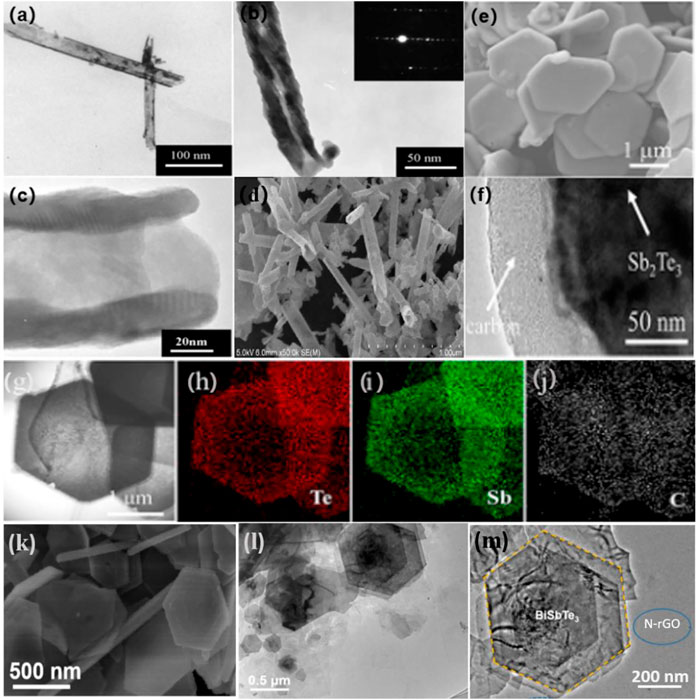
FIGURE 2. (A–D) TEM, SAED pattern, and SEM images of the Bi2Te3 (Wang et al., 2010). Copyright 2010, Elsevier. (E–J) morphologies and Te, Sb, C elemental mapping of the as-prepared Sb2Te3@C sample (Zhang et al., 2019b). Copyright 2019, American Chemical Society. (K) SEM of BiSbTe3 nanosheets. (L) TEM and (M) HRTEM images of BiSbTe3/N-rGO (Zhu et al., 2020). Copyright 2020, Elsevier.
Similar advantages can be attained in solvothermal reactions, such as when one or more precursors are dissolved in a non-aqueous solvent. The BiSbTe3 nanosheets were prepared under solvent-heated conditions by using ethylene glycol as the solvent (Figures 2K–M) (Zhu et al., 2020). The unique nano-plate structure of BiSbTe3 increases the exposure of the electrolyte, leading to high utilization of the composite electrode material during cycling. In addition, the solvothermal method is also commonly used to prepare other metal tellurides, such as Bi2Te3 (Liu et al., 2017a), CuxTe (Liu et al., 2017a), PbTe (Liu et al., 2017a), Ag2Te (Liu et al., 2017a), and Sb2Te3 (Yan et al., 2016).
2.2 Chemical vapor deposition methods
CVD is generally used to create thin film materials. This approach is based on the principle of utilizing gaseous precursor reactants to produce thin films on a substrate by breaking down specific components of the gaseous precursor via atomic and intermolecular chemical interactions (Zhou et al., 2015; Naylor et al., 2016; Zhou et al., 2017b; Yoo et al., 2017). The form and properties of the 2D metal tellurides are influenced by the substrates, precursors, and temperature, among other factors (Zhou et al., 2016; Zhang et al., 2019c; Huang et al., 2019; Kim et al., 2020). CVD method is the most widely used method for the synthesis of tellurides, which has good scalability and can be controlled to prepare large-area films or two-dimensional crystals.
Recently, MoTe2 has received widespread attention owing to its distinct semiconducting and semi-metallic characteristics. Kong et al. investigated a CVD technique for fabricating uniform high-crystalline 2H and 1T'-MoTe2 films (Naylor et al., 2016). Various products were created by varying the precursor, carrier gas, and temperature. Under the same conditions, they observed that MoO3 precursors converted more easily into 2H-MoTe2, whereas MoO and MoOx (x < 3) precursors converted more effectively into 1T'-MoTe2. A year later, Kong et al. improved the preparation process and successfully synthesized large-size homogeneous 1T'-MoTe2 (Zhou et al., 2016). They further determined the significant effect of the molybdenum precursor on the formation of 1T'-MoTe2 (Figure 3A–E). 1T'-MoTe2 was reliably created when MoO3 was utilized as a precursor. Furthermore, the amount of Te used in the synthesis of 1T'-MoTe2 had a substantial impact. If the Te supply is sufficient, then 2H-MoTe2 would be produced; otherwise 1T'-MoTe2 would be produced (Zhou et al., 2016). The established CVD technique also allowed for the large-scale direct synthesis of WTe2 and MoTe2 multilayers and monolayers (Zhou et al., 2017b). The thickness of the WTe2 and MoTe2 atomic layers was adjusted using growth time. (Figure 3F–M).
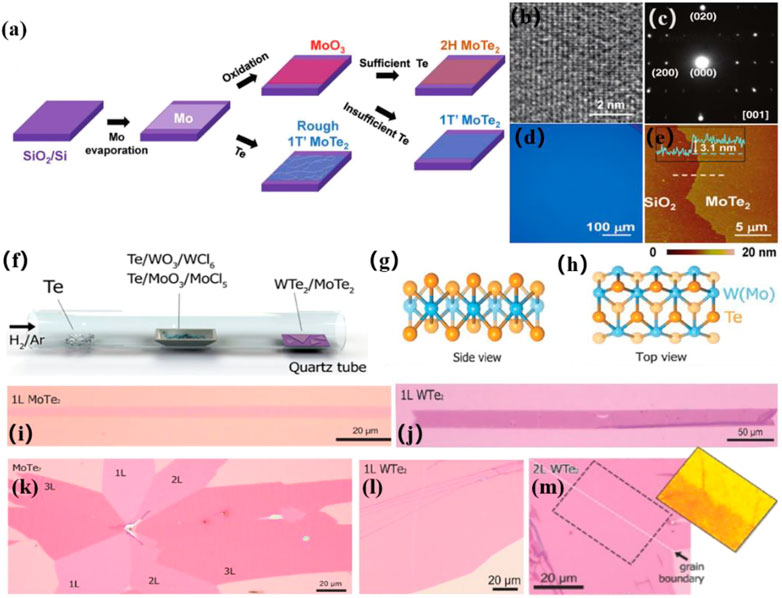
FIGURE 3. (A) Schematic illustration of CVD method to deposit 1T′ and 2H MoTe2 films. (B) high-resolution TEM image, and (C) SAED pattern of 1T′- MoTe2 film grown from MoO3; (D) Typical optical and (E) AFM image of a 1T′- MoTe2 film (Zhou et al., 2016). Copyright 2016, Wiley-VCH. (F) Schematic illustration of CVD method to grow WTe2 and MoTe2 atomic layers. (G,H) Crystalline structures of 1T′-W(Mo)Te2. (I,J) Optical images of MoTe2 and WTe2 monolayer. (K) Optical image of a MoTe2 flake containing 1, 2, and 3 L MoTe2. (L,M) Optical images of a large scale monolayer and bilayer WTe2 films (Zhou et al., 2017b). Copyright 2017, Wiley-VCH.
In addition to 2D WTe2 and MoTe2, many other 2D transition metal tellurides can be grown via CVD methods. Li et al. reported a method that can precisely control 2H-MoTe defects grown by a large-scale phase-change-assisted CVD process using selective etching of I3− solutions (Zhou et al., 2021). Liu et al. reported a facile CVD method to synthesize MoxW1-xTe2 with controlled thickness and chemical composition ratios to investigate its design of material devices from a topological quantum state perspective (Chubilleau et al., 2011). Li et al. reported a strategy using mixed molten salts for enhancing the CVD growth of 2D WTe2 crystals with large grain size and yield, acting as a synergist (Jayababu et al., 2021).
2.3 Electrochemical deposition method
Electrochemical deposition (ECD) is another effective method for obtaining metal tellurides. ECD has the outstanding advantage of easily controlling the morphologies of metal tellurides by using removable templates. Compared with other methods, the ECD method is simple, not limited by grain size and shape, and the prepared crystalline materials have unique properties. Applied electrical potential and deposition rate are two critical parameters of smooth ECD.
Islam et al. suggested an ECD technique for handling bespoke Al2O3 (AAO) stencils that neither needed extensive hole branching nor would damage the aluminum substrate(Figure 4) (Wu et al., 2020). CdTe nanotubes have a high aspect ratio of one-dimensional nanostructures compared to other nanostructures and have amplified optical waveguide properties. Therefore, they are designed as visible light responsive photocatalysts. Figure 4A shows that CdTe was electrochemically deposited onto tailored AAO stencils, which has been sheared by utilizing the full AAO to allow for the acquisition of through-hole and self-supporting features on the Al substrate. The CdTe nanotubes developed in the sulfate bath after the barrier layer has been completely removed, as shown in Figure 4B. The image within the red border is a magnified view of the designated section in the red circle, revealing the hollow ends of the vertically aligned nanotubes. This feature implies the significant advantage of the material to provide electrical contact during cathodic deposition because the aluminum base remains intact even after the barrier layer is completely removed. Meanwhile, broaching barrier layer (BBL) was polarized in dilute H2SO4, compared to neutral KCl solution and immersion in H3PO4 solution, resulting in a totally etched barrier layer that was innocuous to the substrate aluminum.
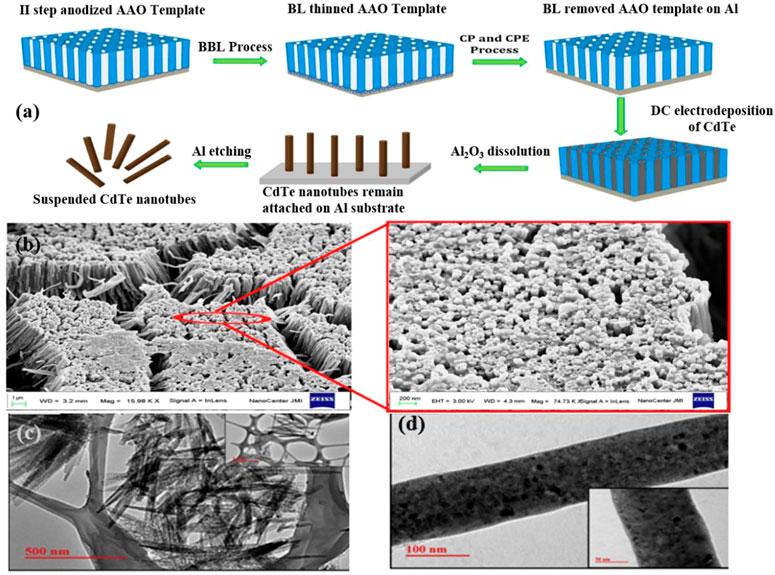
FIGURE 4. (A) Schematic illustration of the steps involved to remove barrier layer from AAO template keeping base Al intact for electrodeposition of CdTe nanotubes; and (B) FE-SEM micrograph of CdTe nanotubes deposited in BL removed AAO template. (C) TEM images illustrating CdTe hollow nanotubes; and (D) solid nanorod obtained using sulfate electrolyte bath (Wu et al., 2020). Copyright 2019, Elsevier.
Choa et al. converted Ag2Te nanotubes to PbTe nanotubes by changing the silver-to-lead atomic ratio through the combined processes of electrostatic spinning, ECD, and cation exchange (Figures 5A–F) (Deshagani et al., 2020). Silver atoms were diffused into the Te layer and transformed into Ag2Te nanofibers by ECD using silver nanofibers synthesized by electrostatic spinning as the starting material. Then, the crystalline transition of AgxTey to PbTe nanocomposites was controlled by the cation exchange from Ag+ cations to Pb2+ cations.
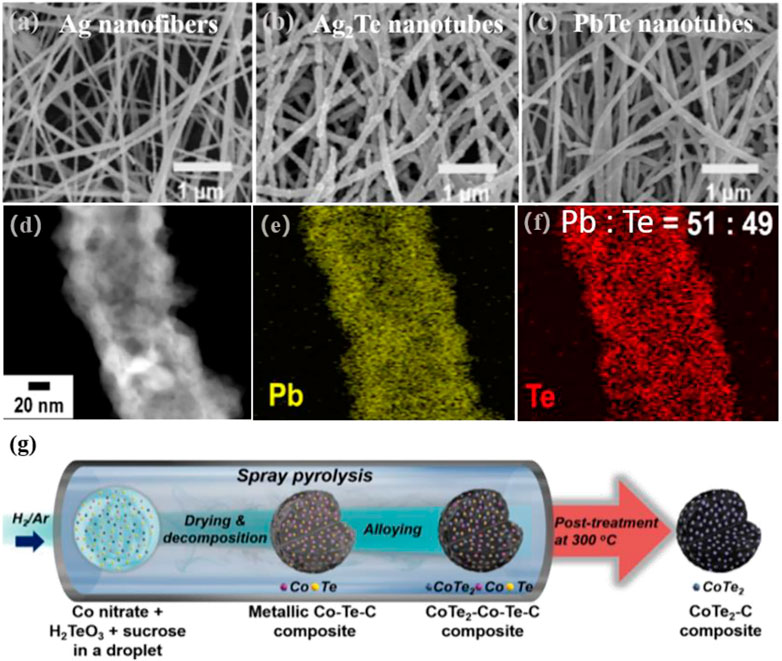
FIGURE 5. SEM images of (A) Ag nanofibers; (B) Ag2Te nanotubes and (C) PbTe nanotubes; (D–F) EDS mapping (Deshagani et al., 2020). Copyright 2018, Elsevier. (G) Schematic illustration of the synthetic process of CoTe2-C composite (Yang et al., 2020). Copyright 2020, Elsevier.
In addition to the abovementioned three methods, many other chemical routes can be used to extensively investigate the synthesis of metal telluride nanomaterials. Examples are microwave synthesis (Ye et al., 2019), spray pyrolysis (Chen et al., 2019), biosynthesis (Poulose et al., 2016), and laser ablation techniques (Jayababu and Kim, 2021). A good method allows for the accurate control of certain features, including spatial structure and distribution, which significantly impact the performance of electrode materials. Kang et al. investigated anode materials for potassium ion batteries using spray pyrolysis to make cobalt telluride-C (CoTe2-C) composite microspheres, as shown in Figure 5G, (Yang et al., 2020). As Te needed to be directly embedded into the composite microspheres, a simple one-step post-treatment technique was used to prepare CoTe2-C composite microspheres. Their approach could be explained by Ostwald maturation induced by the formation of the CoTe2 crystals.
3 Applications as electrodes
3.1 Supercapacitor
Telluride has a substantially greater electrical conductivity and is projected to perform better electrochemically compared with other materials, leading to the widespread research and advancement of metal tellurides built into diverse nanostructures for supercapacitor (SC) applications (Liu et al., 2017b; Rathore et al., 2022). Kim et al. created silver-decorated NiFe alloy telluride nanorods (AMMT HNRs) on nickel foam (NF) (Figure 6A) (Jayababu et al., 2021). The robust electroactivity of the NiFe alloy, the high conductivity of Te and Ag, and the porous layered structure of the telluride all contributed to the AMMT HNRs/NF electrode’s outstanding electrochemical performance. The electrode exhibited a stability of 80.4% over 3,000 cycles. After employing AMMT HNRs/NF and carbon-coated NF as positive and negative electrodes, respectively, and cellulose membranes as separators, high areal energy and power densities were reported in hybrid supercapacitors. (Figure 6B).
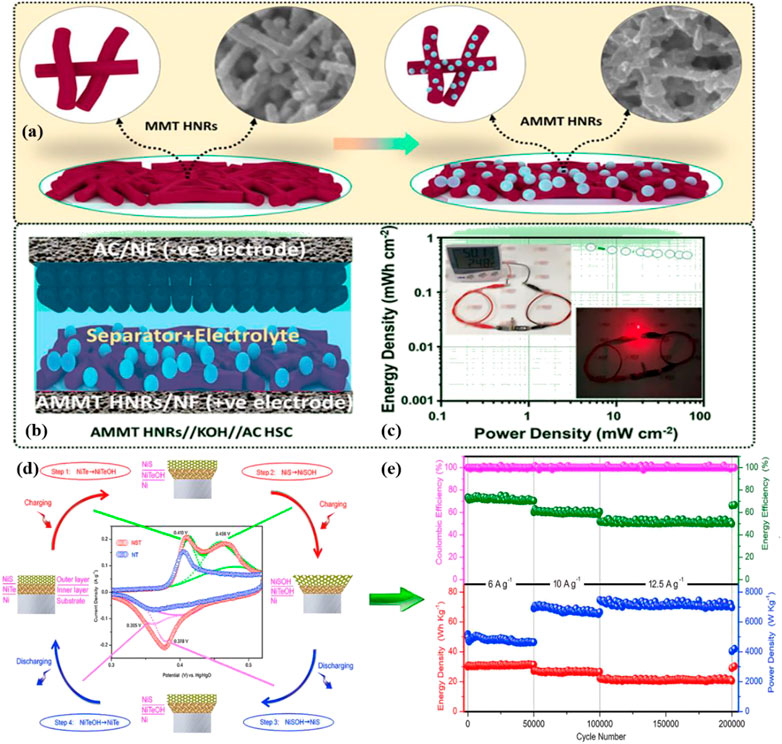
FIGURE 6. (A) Schematic of the MMT HNRs/NF and AMMT HNRs/NF electrodes; (B) Schematic of the hybrid supercapacitors; (C) Energy and power density of the hybrid supercapacitors and real-time suitability tests as a digital multi-sensor power supply (Jayababu et al., 2021). Copyright 2021, American Chemical Society. (D) Step by step illustration of NT and NST electrodes’ electrochemical processes. (E) Electrochemical performance of NiS/NiTe/Ni//AC asymmetric supercapacitor electrodes up to over 200 000 cycles at different current densities (Chen et al., 2019). Copyright 2019, Elsevier.
NiTe, as an SC electrode material, has also attracted the interest of scientists (Park et al., 2018; Song et al., 2021; Yu et al., 2021). The NiTe achieved outstanding electrochemical performance as a coexisting pseudo capacitive material for NiS reported by Wu et al. (Chen et al., 2019). As shown in Figure 6D, four electrochemical reactions occurred on the NiS/NiTe/Ni (NST) electrode. During charging, the volume increased from the inner layer to the outer layer; during discharge, the volume was reduced from the outer layer to the inner layer. The NST was the positive electrode of the asymmetric SC, whereas the active carbon (AC) was the negative electrode. The high capacitance retention and ultra-long cycle life (200000 cycles, Figure 6E) demonstrated the important role of the synergistic structure to structural stability. Additionally, CoTe2 (Manikandan et al., 2020), CuCoTe (Fu and Lee, 2019), VTe2 (Ahmad et al., 2021), and MoTe2 (Jin et al., 2018) have been widely used as electrode materials for high-performance capacitors.
3.2 Anode materials
Metal tellurides have emerged as the most feasible alternative for cutting-edge ion battery anode materials because to their layered crystal structure, high intrinsic conductivity, and high trap density (Zhu et al., 2020; Guo et al., 2021; Hassan et al., 2021). Kang et al. used a structurally distinct FeTe2 and carbon nanocomposite as anode material for potassium ion batteries (Figure 7) (Park and Kang, 2020). The hollow carbon nanospheres that housed the iron telluride nanocrystals (FeTe2-C) offered enough space to accommodate for the nanocrystals’ enormous volume variations during charging and discharging. During cycling, nanocrystal extrusion within the solid hollow carbon nanospheres was controlled, and no FeTe2 from the electrode were lost, showing strong structural integrity (Park and Kang, 2020). Kang et al. also investigated the reaction mechanism of the CoTe2-C composite microsphere as an anode material for potassium ion batteries and the related potassium ion conversion. Where the mechanism of the CoTe2 phase transition reaction can be expressed as:
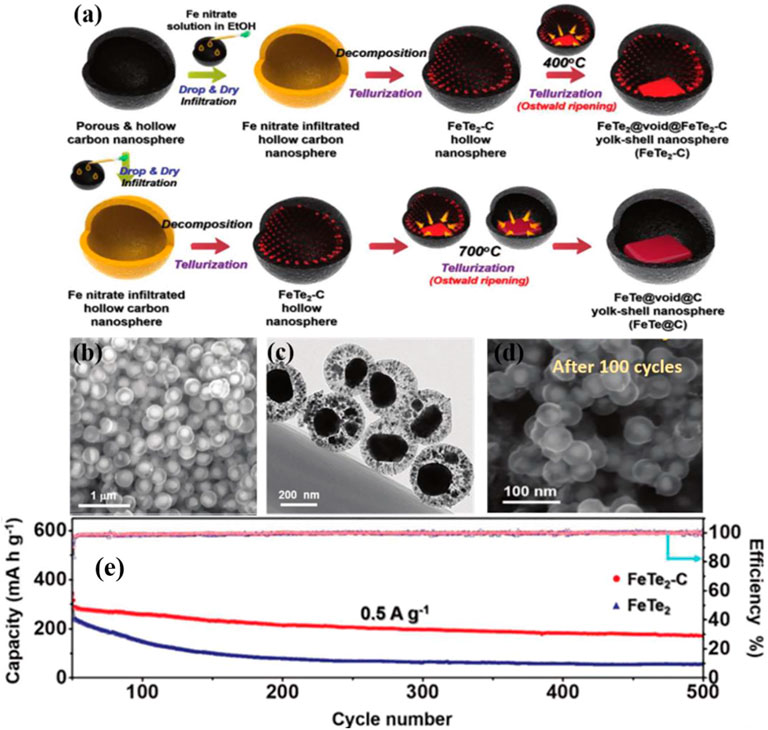
FIGURE 7. (A) Formation illustration of the hollow carbon nanosphere within FeTe2-C nanospheres: (B–D) SEM and TEM images of initial FeTe2-C and FeTe2-C after cycled. (E) long-term cycling performances of FeTe2 and FeTe2-C (Park and Kang, 2020). Copyright 2020, Wiley-VCH.
The surface-driven reactions during rapid potassiumization/depotassiumization significantly promote the charge storage of CoTe2-C in potassium ion cells, leading to excellent rate performance. And the high contribution of the capacitance-controlled behavior of CoTe2-C indicates its good multiplicative performance. At a current density of 0.5 A g−1, the CoTe2-C composite has a 100th cycle discharge capacity of 189.5 mAh g−1 (Chen et al., 2019). In addition, Kim et al. (Fu and Lee, 2019) investigated a CuCo LDHs-coated CuCoTe honeycomb nanosheet as anode for hybrid SCs. They demonstrated the material’s excellent electrochemical performance and high stability (Chen et al., 2019).
3.3 Electrocatalysis
Depicted as 2D materials, tellurides have also been recently described as electrocatalytically active materials with low cost and strong catalytic activity (Luxa et al., 2017; Han et al., 2020). Nath et al. used hydrothermal and electrodeposition methods to create 5 and 8 nm-thick Ni3Te2 films (De Silva et al., 2018). Voltammetric cycling and linear sweep voltammetry were used to examine the OER catalytic activity of the as-synthesized materials in the alkaline electrolytes. Their results showed that Ni3Te2 films have a high catalytic efficiency of 10 mA cm−2 with a notably low overpotential of 180 mV. The overpotential value was much lower than that needed for nanostructured Ni3Se2 (190 mV) (Xu et al., 2019) and Ni3S2 (260 mV) (Li et al., 2022). Nanodendritic MoTe2 was used as an electrocatalyst for hydrogen precipitation reaction (Zhou et al., 2017a). Nanodendrimers were created electrochemically on Mo-doped reduced polyimide/graphene oxide composite substrates. The deposition period increased the size of the nanodendrites. Their findings further showed that nanodendrimers have the potential to be good catalysts for hydrogen precipitation in neutral fluids. Ashiq et al. proposed a highly active Cu7Te4 nanowire synthesized through water oxidation as an electrocatalyst for water oxidation reaction (Zhang et al., 2022). Chu et al. demonstrated the ability of FeTe2 to function as an efficient and durable nitrogen reduction electrocatalyst, with an excellent combination of NH3 yield and Faraday efficiency (Yao et al., 2022). Metal telluride materials, such as Sb2Te3 (He et al., 2022), MoTe2[99], Ni3Te2-CoTe[100], and NiTe-HfTe2[101], have received extensive attention for their potential application in electrocatalysis.
3.4 Li-S batteries
Because of its low cost, environmental friendliness, and high energy density, Li-S batteries are appealing next-generation energy storage technologies. Meanwhile, owing to the intrinsic 2D structure of telluride, it offers an interesting function in Li-S batteries (Wang et al., 2019; Guo et al., 2021; Hassan et al., 2021).
Xie et al. examined diphenyl ditelluride (DPDTe) as bifunctional electrolyte additive for high-efficiency sulfur cathodes and dendrite-free lithium anodes[102]. As shown in Figures 8A–E, the presence of DPDTe enables catalytic mediation by Te radicals, which is accountable for substantially enhancing LIPS redox dynamics and regulating Li2S accumulation. DPDTe can also combine with metallic lithium to generate a uniform, dense, and stable organic–inorganic hybrid SEI to minimize the nucleation overpotential of lithium and promote uniform lithium deposition, thereby successfully limiting the formation of lithium dendrites. The addition of DPDTe can improve sulfur usage and lead to highly reversible lithium stripping/plating, further resulting in good rate capability (611.4 mAh g−1 at 5C) of Li-S batteries.
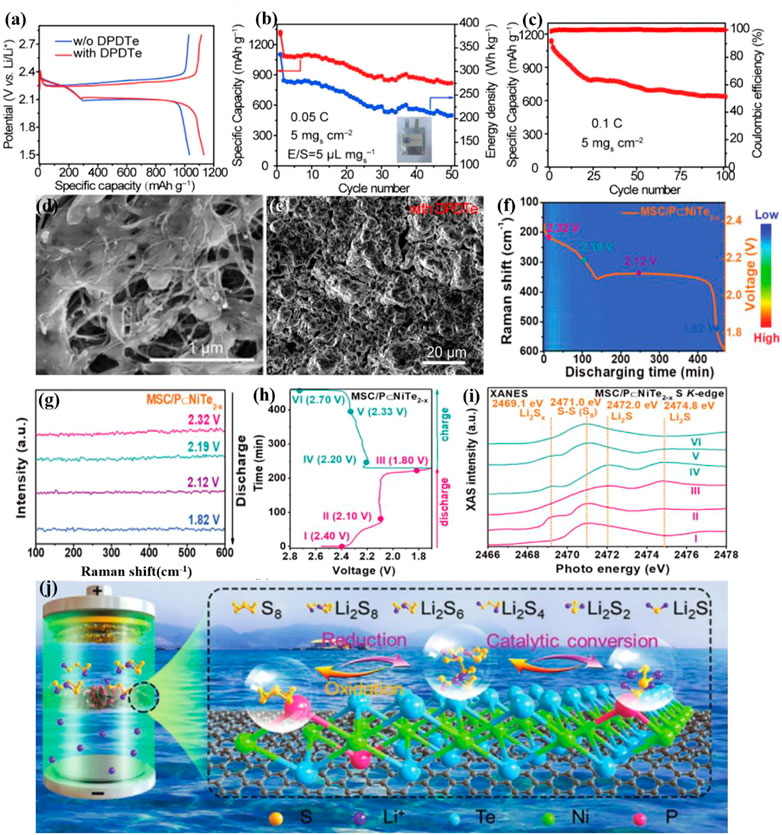
FIGURE 8. (A–C) Electrochemical performance of Li–S pouch cells with/without DPDTe additive, with high sulfur loading of 5 mg cm−2 and E/S ratio of 5.0 μL mg−1. (D) SEM images of the Li2S deposition with DPDTe and (E) the cycled Li anode with DPDTe additive at the 50th cycle[102]. Copyright 2022, Wiley-VCH. (F,G) In situ Raman contour plots and Raman spectra at 0.1 C with MSC/P⊂NiTe2−x; (H,I) Galvanostatic discharge/charge profiles and corresponding ex situ XANES of the S K-edge cathode with MSC/P⊂NiTe2−x separator; (J) Adsorption-catalytic LiPS mechanism with MSC/P⊂NiTe2−x in a Li–S configuration[103]. Copyright 2022, Wiley-VCH.
Zhan et al. explored a phosphorus-doped nickel Te electrocatalyst (P⊂NiTe2-x) grown on carbon-based (MSC) as a functional layer for high-performance Li-S battery separators (MSC/P⊂NiTe2-x)[103]. The increased electrochemical performance implies that the P doping of Te vacancies can enhance Li-S battery conductivity, boost adsorption, and decrease the redox energy barrier of Li-S batteries. MSC nanosheets enable NiTe2 nanoparticles to disperse and diffuse Li+. Ex-situ X-ray absorption spectroscopy and in-situ Raman spectroscopy both demonstrated the ability of MSC/P⊂NiTe2-x to inhibit the shuttle effect and accelerate the redox conversion (Figures 8F–J). Compounding telluride materials in the electrode[91, 104], electrolyte, and diaphragm coatings has become one of the important strategies of breaking through the severe shuttle effect of Li-S batteries.
4 Summary and prospective
Metal tellurides have received extensive attention owing to their great application potential for high-performance electrode materials. In this study, the synthesis methods of tellurides and the research progress of their properties and application in the field of electrodes were reviewed. Three methods for preparing metal tellurides were discussed. Then, the latest progress in terms of the role of telluride in capacitors, anode materials, electrocatalysis, and Li-S batteries was presented. Despite significant progress in the study of 2D tellurides, researchers still face considerable opportunities and challenges.
The CVD approach is now being widely utilized to controllably prepare tellurides. It is feasible to produce 2D tellurides with customizable shape and good crystallinity on a large scale. However, for powder electrochemical materials, the mild chemical interaction between the transition metal and Te under vapor conditions, on the other hand, is a disadvantage of CVD synthesis. Furthermore, while tellurides exhibit remarkable performance, they are difficult to precisely control properties such as pore structure and distribution in the preparation of electrode materials, which greatly affects the volume change of electrode materials during cycling, making it more difficult to improve the capacity, stability and extended cycle life of capacitors and batteries. Future studies should concentrate on: 1) investigating more approaches for the controlled synthesis of tellurides, not only for 2D single-crystal; and 2) designing more composites or building heterostructures to facilitate the electrochemical performances telluride-based electrodes, because like other sulfide generics, tellurides also suffer from extreme volume fluctuations, which result in poor cycling performance.
Author contributions
MG: writing-original draft, investigation; SG: review and; editing, supervision, resources. SX: investigation, visualization, review and; editing; JL: visualization, review and; editing; YW: writing-review and; editing; GZ: resources, supervision.
Funding
This work was supported by the National Natural Science Foundation of China (Grant Nos. 22108133, 51972180, 51572134), the Program for Scientific Research Innovation Team in Colleges and Universities of Jinan (Grant No. 2018GXRC006), and Science, Education and Industry Integration of Basic Research Projects of Qilu University of Technology (Grant No. 2022PY062).
Conflict of interest
The authors declare that the research was conducted in the absence of any commercial or financial relationships that could be construed as a potential conflict of interest.
Publisher’s note
All claims expressed in this article are solely those of the authors and do not necessarily represent those of their affiliated organizations, or those of the publisher, the editors and the reviewers. Any product that may be evaluated in this article, or claim that may be made by its manufacturer, is not guaranteed or endorsed by the publisher.
References
Ahmad, A., Khan, W. U., Shah, A. A., Yasin, N. A., Naz, S., Ali, A., et al. (2021). Synergistic effects of nitric oxide and silicon on promoting plant growth, oxidative stress tolerance and reduction of arsenic uptake in Brassica juncea. Chemosphere 262, 128384. doi:10.1016/j.chemosphere.2020.128384
Apte, A., Krishnamoorthy, A., Hachtel, J. A., Susarla, S., Idrobo, J. C., Nakano, A., et al. (2018). Telluride-based atomically thin layers of ternary two-dimensional transition metal dichalcogenide alloys. Chem. Mat. 30 (20), 7262–7268. doi:10.1021/acs.chemmater.8b03444
Asaba, T., Wang, Y., Li, G., Xiang, Z., Tinsman, C., Chen, L., et al. (2018). Magnetic field enhanced superconductivity in epitaxial thin film WTe2. Sci. Rep. 8 (1), 6520–6527. doi:10.1038/s41598-018-24736-x
Chen, S., Wu, B., Qian, H., Wu, Z., Liu, P., Li, F., et al. (2019). An asymmetric supercapacitor using sandwich-like NiS/NiTe/Ni positive electrode exhibits a super-long cycle life exceeding 200 000 cycles. J. Power Sources 438, 227000. doi:10.1016/j.jpowsour.2019.227000
Chubilleau, C., Lenoir, B., Migot, S., and Dauscher, A. (2011). Laser fragmentation in liquid medium: A new way for the synthesis of PbTe nanoparticles. J. colloid interface Sci. 357 (1), 13–17. doi:10.1016/j.jcis.2011.01.057
De Silva, U., Masud, J., Zhang, N., Hong, Y., Liyanage, W. P. R., Asle Zaeem, M., et al. (2018). Nickel telluride as a bifunctional electrocatalyst for efficient water splitting in alkaline medium. J. Mat. Chem. A Mat. 6 (17), 7608–7622. doi:10.1039/c8ta01760c
Deshagani, S., Ghosal, P., and Deepa, M. (2020). Altered crystal structure of nickel telluride by selenide doping and a poly (N-methylpyrrole) coating amplify supercapacitor performance. Electrochimica Acta 345, 136200. doi:10.1016/j.electacta.2020.136200
Dong, G. H., Zhu, Y. J., and Chen, L. D. (2011). Sb2Te3 nanostructures with various morphologies: Rapid microwave solvothermal synthesis and seebeck coefficients. CrystEngComm 13 (22), 6811–6816. doi:10.1039/c1ce05591g
Dunpall, R., Mlowe, S., and Revaprasadu, N. (2014). Evidence of oriented attachment in the growth of functionalized ZnTe nanoparticles for potential applications in bio-imaging. New J. Chem. 38 (12), 6002–6007. doi:10.1039/c4nj01183j
Elmorshedy, M. F., Elkadeem, M. R., Kotb, K. M., Taha, I. B., and Mazzeo, D. (2021). Optimal design and energy management of an isolated fully renewable energy system integrating batteries and supercapacitors. Energy Convers. Manag. 245, 114584. doi:10.1016/j.enconman.2021.114584
Fu, G., and Lee, J. M. (2019). Ternary metal sulfides for electrocatalytic energy conversion. J. Mat. Chem. A Mat. 7 (16), 9386–9405. doi:10.1039/c9ta01438a
Gong, H., Hao, X., Wu, Y., Cao, B., Xu, H., and Xu, X. (2011). Influence of EDTA2− on the hydrothermal synthesis of CdTe nanocrystallites. J. Solid State Chem. 184 (12), 3269–3272. doi:10.1016/j.jssc.2011.10.018
Goodenough, J. B. (2014). Electrochemical energy storage in a sustainable modern society. Energy Environ. Sci. 7 (1), 14–18. doi:10.1039/c3ee42613k
Grishanov, D. A., Mikhaylov, A. A., Medvedev, A. G., Gun, J., Nagasubramanian, A., Madhavi, S., et al. (2018). Synthesis of high volumetric capacity graphene oxide-supported tellurantimony Na-and Li-ion battery anodes by hydrogen peroxide sol gel processing. J. colloid interface Sci. 512, 165–171. doi:10.1016/j.jcis.2017.10.040
Grishanov, D. A., Mikhaylov, A. A., Medvedev, A. G., Gun, J., Prikhodchenko, P. V., Xu, Z. J., et al. (2018). Graphene oxide‐supported β‐tin telluride composite for sodium‐and lithium‐ion battery anodes. Energy Technol. 6 (1), 127–133. doi:10.1002/ente.201700760
Guo, Y., Cheng, Y., Li, Q., and Chu, K. (2021). FeTe2 as an earth-abundant metal telluride catalyst for electrocatalytic nitrogen fixation. J. Energy Chem. 56, 259–263. doi:10.1016/j.jechem.2020.07.055
Han, Y., Cai, W., Wu, X., Qi, W., Li, B., Li, H., et al. (2020). Electrocatalytic nitrogen fixation on metal tellurides boosted by multiple promoted-synergetic effects of telluride. Cell Rep. Phys. Sci. 1 (11), 100232. doi:10.1016/j.xcrp.2020.100232
Hao, Z., Xu, K., Kang, J., Chen, C., and Zhu, W. (2021). Atomically thin telluride multiheterostructures: Toward spatial modulation of bandgaps. Nanoscale 13 (46), 19587–19592. doi:10.1039/d1nr03746c
Hassan, A., Nisar, L., Iqbal, R., Sadaqat, M., Hussain, F., Ashiq, M. N., et al. (2021). Copper telluride nanowires for high performance electrocatalytic water oxidation in alkaline media. J. Power Sources 491, 229628. doi:10.1016/j.jpowsour.2021.229628
He, J., Bhargav, A., and Manthiram, A. (2022). In situ grown 1T′‐MoTe2 nanosheets on carbon nanotubes as an efficient electrocatalyst and lithium regulator for stable lithium–sulfur full cells. Adv. Energy Mater. 12 (1), 2103204. doi:10.1002/aenm.202103204
Hou, T. C., Yang, Y., Lin, Z. H., Ding, Y., Park, C., Pradel, K. C., et al. (2013). Nanogenerator based on zinc blende CdTe micro/nanowires. Nano Energy 2 (3), 387–393. doi:10.1016/j.nanoen.2012.11.004
Huang, W., Yin, L., Wang, F., Cheng, R., Wang, Z., Sendeku, M. G., et al. (2019). Multibit optoelectronic memory in top‐floating‐gated van der Waals heterostructures. Adv. Funct. Mat. 29 (36), 1902890. doi:10.1002/adfm.201902890
Ishizaki, T., Yata, D., and Fuwa, A. (2003). Electrodeposition of a copper-tellurium compound under diffusion-limiting control. Mat. Trans. 44 (8), 1583–1587. doi:10.2320/matertrans.44.1583
Jaiswal, A. (2017). Lithium-ion battery based renewable energy solution for off-grid electricity: A techno-economic analysis. Renew. Sustain. Energy Rev. 72, 922–934. doi:10.1016/j.rser.2017.01.049
Jamwal, D., Rana, D., Pathak, D., Raizada, P., and Thakur, P. (2016). Array of bis-quaternary ammonium surfactant tailored Cu(2− x)Te quantum dots with amended functional assets. RSC Adv. 6 (17), 13981–13990. doi:10.1039/c5ra24396c
Jamwal, D., Rana, D., Singh, P., Pathak, D., Kalia, S., Thakur, P., et al. (2016). Well-defined quantum dots and broadening of optical phonon line from hydrothermal method. RSC Adv. 6 (104), 102010–102014. doi:10.1039/c6ra19818j
Jayababu, N., Jo, S., Kim, Y., and Kim, D. (2021). Novel conductive Ag-decorated NiFe mixed metal telluride hierarchical nanorods for high-performance hybrid supercapacitors. ACS Appl. Mat. Interfaces 13 (17), 19938–19949. doi:10.1021/acsami.1c00506
Jayababu, N., and Kim, D. (2021). CuCo LDHs coated CuCoTe honeycomb‐like nanosheets as a novel anode material for hybrid supercapacitors. Small 17 (36), 2102369. doi:10.1002/smll.202102369
Jin, T., Han, Q., Wang, Y., and Jiao, L. (2018). 1D nanomaterials: Design, synthesis, and applications in sodium–ion batteries. Small 14 (2), 1703086. doi:10.1002/smll.201703086
Kim, H., Johns, J. E., and Yoo, Y. (2020). Mixed‐dimensional heterostructures: Mixed‐dimensional in‐plane heterostructures from 1D Mo6Te6 and 2D MoTe2 synthesized by Te‐flux‐controlled chemical vapor deposition (Small 47/2020). Small 16 (47), 2070255. doi:10.1002/smll.202070255
Kim, J., Kang, H., Ahn, B., and Seo, H. (2021). Fluorine doping for improved thermoelectric properties of spark plasma sintered bismuth telluride. J. Mater. Sci. Technol. 90, 225–235. doi:10.1016/j.jmst.2021.02.035
Kononov, A., Abulizi, G., Qu, K., Yan, J., Mandrus, D., Watanabe, K., et al. (2020). One-dimensional edge transport in few-layer WTe2. Nano Lett. 20 (6), 4228–4233. doi:10.1021/acs.nanolett.0c00658
Kshetri, T., Singh, T. I., Lee, Y. S., Khumujam, D. D., Kim, N. H., and Lee, J. H. (2021). Metal organic framework-derived cobalt telluride-carbon porous structured composites for high-performance supercapacitor. Compos. Part B Eng. 211, 108624. doi:10.1016/j.compositesb.2021.108624
Li, H., Gu, S., Sun, Z., Guo, F., Xie, Y., Tao, B., et al. (2020). The in-built bionic “MoFe cofactor” in Fe-doped two-dimensional MoTe2 nanosheets for boosting the photocatalytic nitrogen reduction performance. J. Mat. Chem. A Mat. 8 (26), 13038–13048. doi:10.1039/d0ta04251j
Li, J., Tang, X., Song, L., Zhu, Y., and Qian, Y. (2009). From Te nanotubes to CoTe2 nanotubes: A general strategy for the formation of 1D metal telluride nanostructures. J. Cryst. growth 311 (20), 4467–4472. doi:10.1016/j.jcrysgro.2009.08.007
Li, L., Li, H., Li, J., Wu, H., Yang, L., Zhang, W., et al. (2020). Chemical vapor deposition-grown nonlayered α-MnTe nanosheet for photodetectors with ultrahigh responsivity and external quantum efficiency. Chem. Mat. 33 (1), 338–346. doi:10.1021/acs.chemmater.0c03898
Li, T., Wu, J., Qiao, L., Zhu, Q., Fu, Z., Lin, J., et al. (2022). Bimetallic Ni-Hf tellurides as an advanced electrocatalyst for overall water splitting with layered g-C3N4 modification. Mater. Today Energy 26, 101002. doi:10.1016/j.mtener.2022.101002
Li, Y., Duerloo, K. A. N., Wauson, K., and Reed, E. J. (2016). Structural semiconductor-to-semimetal phase transition in two-dimensional materials induced by electrostatic gating. Nat. Commun. 7 (1), 10671–10678. doi:10.1038/ncomms10671
Liu, C., Gao, H., Li, Y., Wang, K., Burton, L. A., and Ren, W. (2020). Manipulation of the Rashba effect in layered tellurides MTe (M= Ge, Sn, Pb). J. Mat. Chem. C Mat. 8 (15), 5143–5149. doi:10.1039/d0tc00003e
Liu, M., Wang, Z., Liu, J., Wei, G., Du, J., Li, Y., et al. (2017). Synthesis of few-layer 1T′-MoTe2 ultrathin nanosheets for high-performance pseudocapacitors. J. Mat. Chem. A Mat. 5 (3), 1035–1042. doi:10.1039/c6ta08206h
Liu, S., Peng, N., Bai, Y., Xu, H., Ma, D. Y., Ma, F., et al. (2017). General solvothermal approach to synthesize telluride nanotubes for thermoelectric applications. Dalton Trans. 46 (13), 4174–4181. doi:10.1039/c7dt00085e
Lu, T. H., Chen, C. J., Basu, M., Ma, C. G., and Liu, R. S. (2015). The CoTe2 nanostructure: An efficient and robust catalyst for hydrogen evolution. Chem. Commun. 51 (95), 17012–17015. doi:10.1039/c5cc06806a
Luxa, J., Vosecký, P., Mazánek, V., Sedmidubsky, D., Pumera, M., Lazar, P., et al. (2017). Layered transition-metal ditellurides in electrocatalytic applications contrasting properties. ACS Catal. 7 (9), 5706–5716. doi:10.1021/acscatal.7b02080
Manikandan, M., Subramani, K., Sathish, M., and Dhanuskodi, S. (2020). Hydrothermal synthesis of cobalt telluride nanorods for a high performance hybrid asymmetric supercapacitor. RSC Adv. 10 (23), 13632–13641. doi:10.1039/c9ra08692g
Morris, G. C., and Vanderveen, R. J. (1993). Cadmium telluride films prepared by pulsed electrodeposition. Sol. energy Mater. Sol. cells 30 (4), 339–351. doi:10.1016/0927-0248(93)90111-f
Myung, N., Park, H. Y., Jee, H. W., Sohn, E. B., Lee, S. J., Paeng, K. J., et al. (2020). Electrosynthesis of MoTe2 thin films: A combined voltammetry-electrochemical quartz crystal microgravimetry study of mechanistic aspects. J. Electrochem. Soc. 167 (11), 116510. doi:10.1149/1945-7111/aba15e
Naylor, C. H., Parkin, W. M., Ping, J., Gao, Z., Zhou, Y. R., Kim, Y., et al. (2016). Monolayer single-crystal 1T′-MoTe2 grown by chemical vapor deposition exhibits weak antilocalization effect. Nano Lett. 16 (7), 4297–4304. doi:10.1021/acs.nanolett.6b01342
Oh, J., Park, H. J., Bala, A., Kim, H. S., Liu, N., Choo, S., et al. (2020). Nickel telluride vertically aligned thin film by radio-frequency magnetron sputtering for hydrogen evolution reaction. Apl. Mater. 8 (12), 121104. doi:10.1063/5.0024588
Park, G. D., and Kang, Y. C. (2020). Conversion reaction mechanism for yolk‐shell‐structured iron telluride‐C nanospheres and exploration of their electrochemical performance as an anode material for potassium‐ion batteries. Small Methods 4 (10), 2000556. doi:10.1002/smtd.202000556
Park, J., Kwon, T., Kim, J., Jin, H., Kim, H. Y., Kim, B., et al. (2018). Hollow nanoparticles as emerging electrocatalysts for renewable energy conversion reactions. Chem. Soc. Rev. 47 (22), 8173–8202. doi:10.1039/c8cs00336j
Poulose, A. C., Veeranarayanan, S., Mohamed, M. S., Aburto, R. R., Mitcham, T., Bouchard, R. R., et al. (2016). Multifunctional Cu2−xTe nanocubes mediated combination therapy for multi-drug resistant MDA MB 453. Sci. Rep. 6 (1), 35961–36013. doi:10.1038/srep35961
Qi, Y., Yang, Z., Peng, S., Wang, M., Bai, J., Li, H., et al. (2021). Self-supported cobalt–nickel bimetallic telluride as an advanced catalyst for the oxygen evolution reaction. Inorg. Chem. Front. 8 (18), 4247–4256. doi:10.1039/d1qi00693b
Rathore, H. K., Hariram, M., Ganesha, M. K., Singh, A. K., Das, D., Kumar, M., et al. (2022). Charge storage mechanism in vanadium telluride/carbon nanobelts as electroactive material in an aqueous asymmetric supercapacitor. J. Colloid Interface Sci. 621, 110–118. doi:10.1016/j.jcis.2022.04.062
Salavati-Niasari, M., Bazarganipour, M., and Davar, F. (2010). Solution-chemical syntheses of nanostructure HgTe via a simple hydrothermal process. J. Alloys Compd. 499 (1), 121–125. doi:10.1016/j.jallcom.2010.03.135
Shi, J., Huan, Y., Zhao, X., Yang, P., Hong, M., Xie, C., et al. (2021). Two-dimensional metallic vanadium ditelluride as a high-performance electrode material. ACS Nano 15 (1), 1858–1868. doi:10.1021/acsnano.0c10250
Song, X., Tian, D., Qiu, Y., Sun, X., Jiang, B., Zhao, C., et al. (2021). Efficient polysulfide trapping and conversion on N‐doped CoTe2 via enhanced dual‐anchoring effect. Small 17 (42), 2102962. doi:10.1002/smll.202102962
Tang, B., Zhou, J., Sun, P., Wang, X., Bai, L., Dan, J., et al. (2019). Phase‐controlled synthesis of monolayer ternary telluride with a random local displacement of tellurium atoms. Adv. Mat. 31 (23), 1900862. doi:10.1002/adma.201900862
Wan, B., Hu, C., Zhou, W., Liu, H., and Zhang, Y. (2011). Construction of strong alkaline hydrothermal environment for synthesis of copper telluride nanowires. Solid state Sci. 13 (10), 1858–1864. doi:10.1016/j.solidstatesciences.2011.07.018
Wang, B. B., Zhu, M. K., Wang, H., Dong, G., and Xie, H. (2012). Study on effects of sodium hydroxide on synthesis of zinc telluride nanocrystals by hydrothermal method. Mater. Sci. Semicond. Process. 15 (2), 131–135. doi:10.1016/j.mssp.2011.09.003
Wang, M., Song, Y., Sun, Z., Shao, Y., Wei, C., Xia, Z., et al. (2019). Conductive and catalytic VTe2@ MgO heterostructure as effective polysulfide promotor for lithium–sulfur batteries. ACS Nano 13 (11), 13235–13243. doi:10.1021/acsnano.9b06267
Wang, Z., Wang, F., Chen, H., Zhu, L., Yu, H. j., and Jian, X. y. (2010). Synthesis and characterization of Bi2Te3 nanotubes by a hydrothermal method. J. alloys Compd. 492 (1-2), L50–L53. doi:10.1016/j.jallcom.2009.11.155
Wei, Y., Chen, J., Wang, S., Zhong, X., Xiong, R., Gan, L., et al. (2020). Wrapping Sb2Te3 with a graphite layer toward high volumetric energy and long cycle Li-ion batteries. ACS Appl. Mat. Interfaces 12 (14), 16264–16275. doi:10.1021/acsami.9b22346
Wei, Y., Huang, L., Chen, J., Guo, Y., Wang, S., Li, H., et al. (2019). Level the conversion/alloying voltage gap by grafting the endogenetic Sb2Te3 building block into layered GeTe to build Ge2Sb2Te5 for Li-ion batteries. ACS Appl. Mat. Interfaces 11 (44), 41374–41382. doi:10.1021/acsami.9b14293
Wood, S. M., Klavetter, K. C., Heller, A., and Mullins, C. B. (2014). Fast lithium transport in PbTe for lithium-ion battery anodes. J. Mat. Chem. A Mat. 2 (20), 7238–7243. doi:10.1039/c4ta01167h
Wu, B., Qian, H., Nie, Z., Luo, Z., Wu, Z., Liu, P., et al. (2020). Ni3S2 nanorods growing directly on Ni foam for all-solid-state asymmetric supercapacitor and efficient overall water splitting. J. Energy Chem. 46, 178–186. doi:10.1016/j.jechem.2019.11.011
Xu, J., Yin, Y., Xiong, H., Du, X., Jiang, Y., Guo, W., et al. (2019). Improving catalytic activity of metal telluride by hybridization: An efficient Ni3Te2-CoTe composite electrocatalyst for oxygen evolution reaction. Appl. Surf. Sci. 490, 516–521. doi:10.1016/j.apsusc.2019.06.080
Yan, X., Zheng, W., Liu, F., Yang, S., and Wang, Z. (2016). Thickness effects for thermoelectric property of antimony telluride nanoplatelets via solvothermal method. Sci. Rep. 6 (1), 37722–37728. doi:10.1038/srep37722
Yang, S., Park, G. D., and Kang, Y. C. (2020). Conversion reaction mechanism of cobalt telluride-carbon composite microspheres synthesized by spray pyrolysis process for K-ion storage. Appl. Surf. Sci. 529, 147140. doi:10.1016/j.apsusc.2020.147140
Yang, Y., Taggart, D. K., Brown, M. A., Xiang, C., Kung, S. C., Yang, F., et al. (2009). Wafer-scale patterning of lead telluride nanowires: Structure, characterization, and electrical properties. ACS Nano 3 (12), 4144–4154. doi:10.1021/nn901173p
Yao, W., Tian, C., Yang, C., Xu, J., Meng, Y., Manke, I., et al. (2022). P‐doped NiTe2 with Te‐vacancies in lithium–sulfur batteries prevents shuttling and promotes polysulfide conversion. Adv. Mater. 34 (11), 2106370. doi:10.1002/adma.202106370
Ye, B., Gong, C., Huang, M., Ge, J., Fan, L., Lin, J., et al. (2019). A high-performance asymmetric supercapacitor based on Ni3S2-coated NiSe arrays as positive electrode. New J. Chem. 43 (5), 2389–2399. doi:10.1039/c8nj05399e
Yoo, Y., DeGregorio, Z. P., Su, Y., Koester, S. J., and Johns, J. E. (2017). In‐plane 2H‐1T′ MoTe2 homojunctions synthesized by flux‐controlled phase engineering. Adv. Mat. 29 (16), 1605461. doi:10.1002/adma.201605461
Yu, B., Huang, A., Srinivas, K., Zhang, X., Ma, F., Wang, X., et al. (2021). Outstanding catalytic effects of 1T′-MoTe2 quantum dots@ 3D graphene in shuttle-free Li–S batteries. ACS Nano 15 (8), 13279–13288. doi:10.1021/acsnano.1c03011
Yu, Z., Ferrer-Argemi, L., Kim, J., Lim, J. H., Myung, N. V., and Lee, J. (2018). Phase-dependent thermal conductivity of electrodeposited antimony telluride films. J. Mat. Chem. C Mat. 6 (13), 3410–3416. doi:10.1039/c8tc00140e
Yu, Z., Jiao, S., Tu, J., Luo, Y., Song, W. L., Jiao, H., et al. (2020). Rechargeable nickel telluride/aluminum batteries with high capacity and enhanced cycling performance. ACS Nano 14 (3), 3469–3476. doi:10.1021/acsnano.9b09550
Zeng, Y., Wang, M., He, W., Fang, P., Wu, M., Tong, Y., et al. (2019). Engineering high reversibility and fast kinetics of Bi nanoflakes by surface modulation for ultrastable nickel–bismuth batteries. Chem. Sci. 10 (12), 3602–3607. doi:10.1039/c8sc04967j
Zhang, H. T., Xiong, Y. M., Luo, X. G., Wang, C., Li, S., and Chen, X. (2002). Hydrothermal synthesis and characterization of NiTe alloy nanocrystallites. J. Cryst. growth 242 (3-4), 259–262. doi:10.1016/s0022-0248(02)01456-2
Zhang, L., Ai, Z., Jia, F., Liu, L., Hu, X., and Yu, J. C. (2006). Controlled hydrothermal synthesis and growth mechanism of various nanostructured films of copper and silver tellurides. Chem. Eur. J. 12 (15), 4185–4190. doi:10.1002/chem.200501404
Zhang, Q., and Guo, S. (2020). Emerging materials methods for renewable energy. Small Methods 4 (6), 2000087. doi:10.1002/smtd.202000087
Zhang, S., Qiu, L., Zheng, Y., Shi, Q., Zhou, T., Sencadas, V., et al. (2021). Rational design of core‐shell ZnTe@ N‐doped carbon nanowires for high gravimetric and volumetric alkali metal ion storage. Adv. Funct. Mat. 31 (3), 2006425. doi:10.1002/adfm.202006425
Zhang, W., Ma, F., Wu, Q., Zeng, Z., Zhong, W., Cheng, S., et al. (2022). A dual–functional organotelluride additive for highly efficient sulfur redox kinetics and lithium regulation in lithium–sulfur batteries. Energy & Environ. Mater. doi:10.1002/eem2.12369
Zhang, W., Zhang, Q., Shi, Q., Xin, S., Wu, J., Zhang, C. L., et al. (2019). Facile synthesis of carbon-coated porous Sb2Te3 nanoplates with high alkali metal ion storage. ACS Appl. Mat. Interfaces 11 (33), 29934–29940. doi:10.1021/acsami.9b09056
Zhang, X., Jin, Z., Wang, L., Hachtel, J. A., Villarreal, E., Wang, Z., et al. (2019). Low contact barrier in 2H/1T′ MoTe2 in-plane heterostructure synthesized by chemical vapor deposition. ACS Appl. Mat. Interfaces 11 (13), 12777–12785. doi:10.1021/acsami.9b00306
Zhang, Y., Tang, Y., Deng, J., Leow, W. R., Xia, H., Zhu, Z., et al. (2019). Correlating the Peukert’s constant with phase composition of electrode materials in fast lithiation processes. ACS Mat. Lett. 1 (5), 519–525. doi:10.1021/acsmaterialslett.9b00320
Zheng, G., Wang, D., Tian, S., Ren, M., and Song, M. (2021). Effect of microstructure and contact interfaces of cobalt MOFs-derived carbon matrix composite electrode materials on lithium storage performance. Energy 222, 119914. doi:10.1016/j.energy.2021.119914
Zhou, J., Liu, F., Lin, J., Huang, X., Xia, J., Zhang, B., et al. (2017). Large‐area and high‐quality 2D transition metal telluride. Adv. Mat. 29 (3), 1603471. doi:10.1002/adma.201603471
Zhou, L., Xu, K., Zubair, A., Liao, A. D., Fang, W., Ouyang, F., et al. (2015). Large-area synthesis of high-quality uniform few-layer MoTe2. J. Am. Chem. Soc. 137 (37), 11892–11895. doi:10.1021/jacs.5b07452
Zhou, L., Yan, S., Lu, T., Shi, Y., Wang, J., and Yang, F. (2014). Indium telluride nanotubes: Solvothermal synthesis, growth mechanism, and properties. J. Solid State Chem. 211, 75–80. doi:10.1016/j.jssc.2013.11.033
Zhou, L., Zubair, A., Wang, Z., Zhang, X., Ouyang, F., Xu, K., et al. (2016). Synthesis of high‐quality large‐area homogenous 1T′ MoTe2 from chemical vapor deposition. Adv. Mat. 28 (43), 9526–9531. doi:10.1002/adma.201602687
Zhou, Y., Jia, L., Feng, Q., Wang, T., Li, X., and Wang, C. (2017). MoTe2 nanodendrites based on Mo doped reduced graphene oxide/polyimide composite film for electrocatalytic hydrogen evolution in neutral solution. Electrochimica Acta 229, 121–128. doi:10.1016/j.electacta.2017.01.147
Zhou, Y., Tao, L., Chen, Z., Lai, H., Xie, W., and Xu, J. (2021). Defect etching of phase‐transition‐assisted CVD‐grown 2H‐MoTe2. Small 17 (32), 2102146. doi:10.1002/smll.202102146
Keywords: 2D metal tellurides, synthesis, electrocatalysis, batteries, electrodes
Citation: Guo M, Gu S, Xu S, Lu J, Wang Y and Zhou G (2022) Design, synthesis and application of two-dimensional metal tellurides as high-performance electrode materials. Front. Chem. 10:1023003. doi: 10.3389/fchem.2022.1023003
Received: 19 August 2022; Accepted: 31 August 2022;
Published: 26 September 2022.
Edited by:
Hongda Li, Guangxi University of Science and Technology, ChinaReviewed by:
Xintong Liu, Beijing Technology and Business University, ChinaZhanglei Ning, Sichuan Normal University, China
Copyright © 2022 Guo, Gu, Xu, Lu, Wang and Zhou. This is an open-access article distributed under the terms of the Creative Commons Attribution License (CC BY). The use, distribution or reproduction in other forums is permitted, provided the original author(s) and the copyright owner(s) are credited and that the original publication in this journal is cited, in accordance with accepted academic practice. No use, distribution or reproduction is permitted which does not comply with these terms.
*Correspondence: Shaonan Gu, c25ndUBxbHUuZWR1LmNu; Guowei Zhou, Z3d6aG91QHFsdS5lZHUuY24=