- 1Key Laboratory of Optoelectronics Devices and Systems of Ministry of Education, College of Physics and Optoelectronic Engineering, Shenzhen University, Shenzhen, China
- 2Institute for Advanced Study, Shenzhen University, Shenzhen, China
- 3Institute of Microscale Optoelectronics, Shenzhen University, Shenzhen, China
- 4Additive Manufacturing Institute, College of Mechatronics and Control Engineering, Shenzhen University, Shenzhen, China
Inorganic perovskites have been recognized as highly potent materials for the display and medical industries due to their outstanding features. However, there haven’t been many reports on their implications as a photocatalyst for the removal of heavy metals. Photocatalysis has been regarded as a significant approach for the removal of pollutants because of its great sustainability, improved efficiency, and reduced energy consumption. Here, we applied inorganic cesium lead halides (Br and I) with zinc oxide heterostructure as a photocatalyst for the first time. The heterostructure has been synthesized by the traditional hot injection strategy and its photocatalytic activity was systematically investigated. Interestingly, the CsPbX3/ZnO heterostructure as a photocatalyst has a homogeneous geometry and possesses an excellent degradation efficiency of over 50% under xenon UV-Visible light. The CsPbX3/ZnO catalyst carries superior oxidation/reduction properties and ionic conductivity due to the synergistic photogenerated charge carrier and interaction between CsPbX3 and ZnO. The recycling experiment showed the good stability of the catalysts. These findings suggest that inorganic lead halide heterostructure has the potential to be used for heavy metal degradation and water pollution removal catalysts.
1 Introduction
Lead tri-halide perovskites have been considered as a fascinating class of materials for next generation applications due to their remarkable characteristics, including enhanced optical features, high extinction constant, tunable bandgap, versatile surface chemistry, long-range electron-hole diffusion, and high carrier mobility (Akkerman et al., 2015; Gull et al., 2020). This class of materials possesses the general formula of ABX3 where A is a cation (organic/inorganic), B is a divalent metal (Pb2+, Sn2+, Ge2+), and X is an anion (Cl1-, Br1-, I1- or mixture) (Kulkarni et al., 2019; Yang et al., 2019). In recent years, semiconductor materials have been widely used as photocatalyst in the environmental and energy sectors due to their novel physiochemical features and cost-effectiveness (Batool et al., 2020; Shen et al., 2021b) Commonly used metal-based semiconducting photocatalysts include TiO2 (Fujishima et al., 2000) (Zhang et al., 2021b), Fe2O3 (Hitam and Jalil, 2020), CdS (Cheng et al., 2018), MoS2 (Shen et al., 2020), ZnS (Lee and Wu, 2017), and ZnO (Johar et al., 2015).
Zinc Oxide (ZnO) has a bandgap of 3.37 eV and can treat heavy metals due to its high photocatalytic efficiency and excitation binding energy-producing electron-hole pair (ehp) under UV or visible irradiation (Huang et al., 2015; Le et al., 2019). The electron and hole combine with the adsorbed oxygen (O.) on the photocatalyst surface and water (H2O) to generate O2 and hydroxyl (OH), which help in the oxidation of organic products into end products (CO2 and H2O) (Senapati et al., 2012). Photocatalysis has been regarded as a significant approach for pollutant removal due to its high sustainability, improved efficiency, and low energy consumption (Shen et al., 2021a; Li et al., 2022b) The investigation of photocatalysts with remarkable features such as extraordinary sunlight absorption, significant generation, and separation of charge carriers with enhanced redox potential is quite useful for achieving efficient photocatalytic removal of pollutant (Idrees et al., 2016; Li et al., 2022c). Photocatalysts, a green technology that uses solar energy, have a significant impact on environmental restoration. Therefore, more time is required to investigate potential photocatalysts (Li et al., 2022a; Cai et al., 2022; Li et al., 2022d). In this aspect, lead tri-halide with unique properties could also be used in the photochemical conversion, if the issues of stability, inefficient photocatalytic activity, and rigorous ehp recombination rate could be optimized (Zhao et al., 2020). There have recently been a few reports on the use of inorganic lead tri-halide perovskites, their derivatives, and composites as photocatalysts. Among the cesium-based lead halides, it was observed that pristine bromide-based compounds with a wide-bandgap are difficult to photocatalyze; however, after different treatments such as making a heterostructure or changing the typical ligands are an optimal choice (Xu et al., 2017). Moreover, photoreduction of CO2 and hydrogen evolution using a mixture of Br/Cl and Br/I has been reported (Guan et al., 2019). In comparison to these inorganic halides, cesium lead iodide (CsPbI3) had a narrow bandgap of 1.73 eV, high emission intensity, and existed in two major phases known as alpha phase (α-CsPbI3) and delta phase (δ-CsPbI3) (Lai et al., 2017). Lin et al., recently reported the use of CsPbI3 as a photocatalyst by making heterostructure with tungsten disulfide (WS2), where they used γ-CsPbI3 nanocrystals fabricated with several layered tungsten disulfides for the complete degradation of methylene blue (MB) into less toxic inorganic products with high photocatalytic degradation efficiency (Zhang et al., 2019). The coupling of inorganic perovskites with other compounds is considered as useful strategy for addressing the issues of instability, photocatalytic activity deficiency, and controlled ehp recombination. Ma et al. reported on the photocatalytic activity of graphitic carbon nitride (g-C3N4) combined with CsPbI3 for photocatalytic degradation of the organic dye rhodamine B, and they also reported the use of Pt as a co-catalyst for hydrogen generation by water splitting (Liu and Ma, 2021). Up to now, there are no reports about the photocatalytic degradation of inorganic pollutants from water streams by the CsPbX3/ZnO heterostructure.
Here, for the first time, we used CsPbX3/ZnO heterostructure with ZnO as a photocatalyst to study the photocatalytic activity of CsPbX3. (Zhang et al., 2021a). Although Xu et al., recently reported a CsPbX3/ZnO heterostructure for light-emitting diodes. Wang et al., also used ZnO as a basis for transporting electrons to solar cells (Deng et al., 2020). To date, however, no reports have been found regarding the use of ZnO and CsPbX3 for photocatalytic applications. This study is aimed at modifying CsPbI3, and CsPbBr3 using ZnO with the desired properties for the efficient photo degradation of heavy metals. We investigated the photocatalytic response in CsPbI3, CsPbBr3 forming heterostructures with ZnO and the results proved that δ-CsPbI3/ZnO heterostructure is quite beneficial in the photo degradation of heavy metals under visible light with an efficiency of more than 50%, owing to its hexagonal structure.
2 Materials and method
2.1 Materials
Cesium carbonate (Cs2CO3, 99.99%) was bought from Macklin. Lead iodide (PbI2, 99.99%), Lead bromide (PbBr2, 99.99%), Octadecene (ODE, technical grade 90%), Oleic acid (OA, technical grade 90%), oleylamine (OAm, technical grade 70%), Zinc Stearate (ZnSt2, 98%), Toluene (98%) and Hexane (99.9%) were purchased from Sigma Aldrich and used as it is received without any further modification.
2.2 Photocatalysts synthesis
2.2.1 Formation of cesium and lead halide precursors by hot injection method
Protesescu’s hot injection strategy was used for the synthesis of CsPbX3/ZnO heterostructure, with minor modifications (Protesescu et al., 2015). The stepwise schematic presentation is shown in Figure 1. Firstly, 0.6 g of cesium carbonate (Cs2CO3), 2 ml OA, and 20 ml ODE were added to a 50 ml 3-neck round bottom flask and stirred continuously under vacuum for 30 min at 125°C, absolving the flask with nitrogen (N2) for 10 min, it was placed back under vacuum. An alternative implication of vacuum and N2 to completely remove moisture and oxygen has been applied as shown in Figure 1A. The PbI2/Br2 precursors were then synthesized by degassing 0.8 g of PbI2 and 0.6 g of PbBr2 in 20 ml ODE for 1 h under constant stirring and heating at 125°C in a 50 ml flask. The flask was then filled with a 1:1 mixture of OA and OAm (4 ml each, pre-heated at ∼70°C) and vacuumed again for 15–30 min, until the salt of lead halides was completely dissolved and the solution was no longer releasing gas (15–30 min), as shown in Figure 1B.
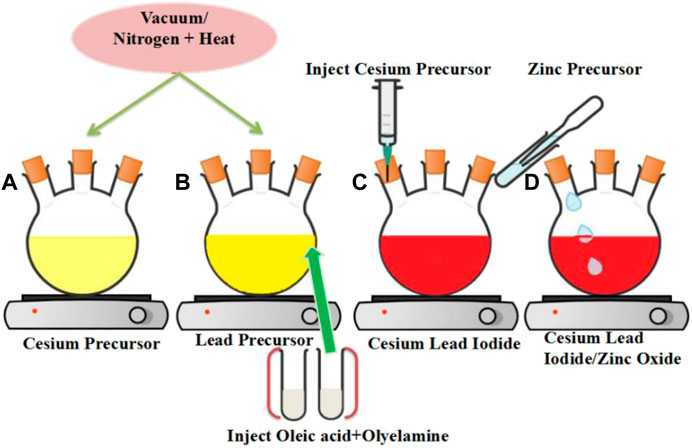
FIGURE 1. Schematic representation for the synthesis of the CsPbI3/ZnO heterostructure (A) Cesium precursor (B) Lead precursor (C) formation of Cesium Lead Iodide (D) formation of Cesium Lead Iodide/Zinc Oxide heterostructure.
2.3 Formation of cesium lead halide/zinc oxide heterostructure
For the formation of cesium lead halides, the temperature of the lead halide precursor was raised to 150°C, and 4 ml cesium precursor was injected swiftly under a nitrogen environment, as shown in Figure 1C. Later, for the photocatalytic activity investigation of as synthesized CsPbX3 with Zinc oxide, 0.4 mg ml−1 zinc stearate in ODE was injected into the above mixture as a zinc source for the formation of CsPbX3/ZnO heterostructure as shown in Figure 1D, aliquotes were taken at different times, and the reaction was quenched by dipping the flask into the ice bath. Finally, the solution was centrifuged for 3 min at 10,000 rpm, the supernatant was discarded, and precipitates were dispersed in toluene/hexane for further investigation.
2.4 Characterization techniques
X-ray diffraction patterns (XRD) spectra was collected X-ray diffractometer (Bruker D8 advance, Germany) with Cu Kα radiation (k = 1.54 nm) in the range of 2θ = 20°–70°. The valence properties of all existing elements in CsPbX3/ZnO NPs were determined by employing X-ray photoelectron (XPS: ESCALAB 250Xi-system) Ultraviolet-visible (UV-visible) spectra were investigated by a Lambda 950 spectrophotometer in the wavelength range of 300–800 nm. Fourier Transform Infrared (FTIR) has been carried out by Shimadzu-8400S infrared spectrometer. The morphology of the material was investigated by transmission electron microscope (JEOL JSM-7800F) and energy-dispersive spectra (EDS) was obtained by using an integrated Oxford INCA X-ACT equipped with SEM. On a multi-channel battery system (LANHE-CT2001A), the electrocatalytic activity of prototype coin cells was studied in a voltage range of 0.1–3.0 V at a constant current density.
2.5 Photocatalytic activity measuring experiment
The photocatalytic activity of CsPbX3/ZnO heterostrucutre as a photocatalyst was irradiated to a 300W xenon lamp in ambient conditions. For which ZnO (0.4 mgmL−1 ZnSt2 in ODE) was injected to CsPbX3 solution, under constant magnetic stirring and heating to achieve equilibrium. For the photocatalytic inquiry, two different temperatures have been used i.e., 100°C and 160°C. After injecting zinc precursor into the halide perovskites, samples were prepared using 15 µL (CsPbX3/ZnO) in 1 ml hexane at different time intervals starting from 2 min till 30min. Photocatalytic activity under UV-visible spectrophotometer in the wavelength range of 300–800 nm has been observed. To explore the photocatalytic activity of ZnO as a photocatalyst, the absorbance spectra of halide perovskites with and without zinc oxide were compared.
Degradation efficiency (%) has been calculated by using Eq. 1.
where Co is degradation concentration without ZnO and Ct degradation concentration with ZnO at different intervals in the above relation (Huang et al., 2015; Sajid et al., 2020).
3 Results and discussions
3.1 Characterization of CsPbX3/ZnO heterostructure
Studies have shown that inorganic cesium lead halide perovskite is considered as a hot area for scientists and researchers due to its outstanding performance in different applications. Different reports highlight its usage as photocatalysts for CO2 reduction (Wang et al., 2019), hydrogen evolution (Zhao et al., 2021) and degradation of different pollutants from the environment and industry (Zhao et al., 2020; Li et al., 2023). To investigate the as-synthesized system of CsPbX3/ZnO heterostructure, different investigations have been carried out. Figure 2A is the XRD pattern of the CsPbI3/ZnO heterostructure. The XRD pattern confirmed the presence of both CsPbI3 and ZnO, which are highlighted with different symbols. All of the characteristic peaks correspond to JCPDS card number. 18–0376 and 36–1,451, which represent the delta and wurtzite phases, respectively. The diffraction peaks located at 21.6°, 22.7°,31.3°, and 39.3° indicate the presence of CsPbI3 (highlighted with #), whereas the peaks at 31.8°,34.4°, 36.2°, 47.5°, 56.5°, 62.8°, 66.3°, and 67.9° correspond to the ZnO (represented with *) (Zhang et al., 2007). Figures 2B,C show the morphology of CsPbI3 and CsPbI3/ZnO heterostructures having the hexagonal geometry of CsPbI3 with an average particle size of 28.3 nm that has been enlarged to 34.5 nm after the addition of photocatalyst ZnO, proving the inclusion of ZnO with the retention of the same hexagonal morphology. The dots on the surface of Figure 2C justifying the formation of the CsPbI3/ZnO heterostructure.
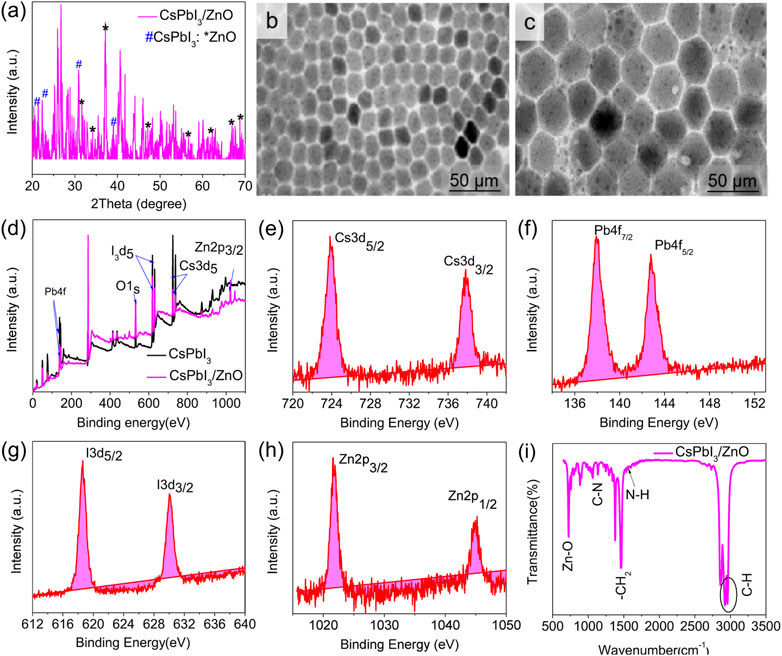
FIGURE 2. (A) X-ray diffraction (XRD) pattern of CsPbI3/ZnO heterostructure, (B,C) TEM images of CsPbI3 and CsPbI3/ZnO heterostructure. (D) The general XPS spectrum and (E–H) HR-XPS spectrum of CsPbI3/ZnO heterostructure. (I) FTIR spectra of CsPbI3/ZnO heterostructure.
XPS analysis was conducted to investigate the chemical states of elements presented in the as-synthesized CsPbI3/ZnO heterostructure (Figure 2D). The general XPS survey spectrum proves the existence of Cs, Pb, I, Zn, and O, confirming the formation of CsPbI3/ZnO. Figures 2E–H presents the HR-XPS deconvoluted peaks of all existing elements.
The HR-XPS Cs spectrum deconvoluted into peaks at 723.86 and 737.83 eV, ascribed to the Cs3d of Cs3d5/2 and Cs3d3/2, respectively (Figure 2E). The peaks at 137.96 and 142.80 eV in the core level spectrum of Pb4f are attributed to Pb4f7/2 and Pb4f5/2, respectively (Figure 2F). Similarly, the core-level I3d spectrum position at 618.61 eV indicated the presence of I3d5/2 and 630 eV I3d3/2 (Figure 2G), while the peaks at 1,021.9 and 1,044.94 eV were attributed to the formation of Zn2p3/2 and Zn2p1/2 (Zn-O), respectively (Figure 2H) (Lu et al., 2018; Pirhashemi et al., 2019).
The surface functionality of the materials was recorded by FTIR spectrum in the range of 650–3,500 cm−1. Figure 2I shows the FTIR spectra of the CsPbI3/ZnO heterostructure in which the peak at 716 cm−1 shows the bonding between Zn-O, whereas the rest of the peaks show the presence of different ligands used for the formation of heterostructure. Generally, the peak at 1,153 cm−1 represents C-N, 1,473 cm−1 shows -CH2, 1,641 cm−1 to N-H, and 2,913 and 2,955 cm−1 correspond to the presence of C-H attributed due to the aliphatic chains of octadecyl, oleylamine and oleic acid (An et al., 2018) (Nipane et al., 2013). EDS was used to investigate the elemental percentages in the synthesized compound, as shown in Figure 3.
The EDS spectrum confirms the existence and equal distribution of all the elements in the synthesized compound, and their atomic percentage is listed in Table 1.
3.2 Photocatalytic activity of CsPbX3/ZnO heterostructure
The basic photocatalytic mechanism starts with the generation of electrons and holes generated as a result of light irradiation with a wavelength greater than or equal to their bandgap; photo-induced electrons move from the valence band (VB) to the conduction band (CB), and corresponding holes move to the valence band. Hence, a redox reaction was carried out using these isolated electron-hole pairs (Ren et al., 2022). The as-synthesized product was optically characterized using UV-visible spectroscopy to analyze the behavior of inorganic halide perovskites with ZnO as a photocatalyst. The designed composite heterostructure is made up of interfaces made of various materials that are tightly bonded and have indistinguishable interface junctions (Zhao et al., 2018). The benefit of using zinc is that it has the capability to replace lead during the formation of heterostructure, indicating the potential to be used as a heavy metal degradation and water pollution removal catalyst (Kar et al., 2022).
The UV-visible absorption spectra of two different inorganic lead halide perovskite (CsPbI3 and CsPbBr3) with ZnO as a photocatalyst for two temperatures, low (100°C) and high (160°C), with activity times ranging from 2 to 30 min, as shown in Figures 4A–D. Figures 4A,B shows that CsPbI3 possesses the highest photocatalytic activity, whereas Figures 4C,D represents that CsPbBr3 has no effect on ZnO even at elevated temperatures. It was observed that pristine CsPbBr3 has negligible photocatalytic activity due to obvious surface defects that introduce shallow transition levels and act as charge recombination sites, and also the material’s wider bandgap, which limits its use as a photocatalytic material. In comparison to CsPbBr3, CsPbI3 has a narrow bandgap of 1.73 eV and higher emission intensity, exhibiting the potential for excessive photo-generated ehp for photocatalysis (Tang et al., 2019; Zhang et al., 2019; Wang et al., 2021). ZnO’s good electron mobility, high absorption tendency, and cost-effectiveness make it an ideal photocatalyst. Coupling ZnO with halide perovskites enhances the generation of ehp by reducing the formation energy of the perovskites (Senapati et al., 2012; Jiang et al., 2019; Deng et al., 2020). Figures 4A,B show an absorbance peak from 370–385 nm due to zinc adsorption, confirming the presence of ZnO (Das et al., 2020; Karami et al., 2020), whereas, in Figures 4C,D show no change in the spectra, indicating that there is no zinc adsorption. Figures 5A,B depicts the obvious zinc adsorption in the CsPbI3/ZnO heterostructure by depicting the obvious absorbance peaks from 370–385 nm at both temperatures as compared to controlled samples of CsPbI3 and ZnO (Figure 5E), while Figures 5C,D represent no discernible change in the spectra. So, here we calculated the degradation efficiency with time in synthesized samples of CsPbI3/ZnO heterostructure at high temperature yielding a degradation efficiency of 52% by using Eq. 1 shown in Figure 4E.
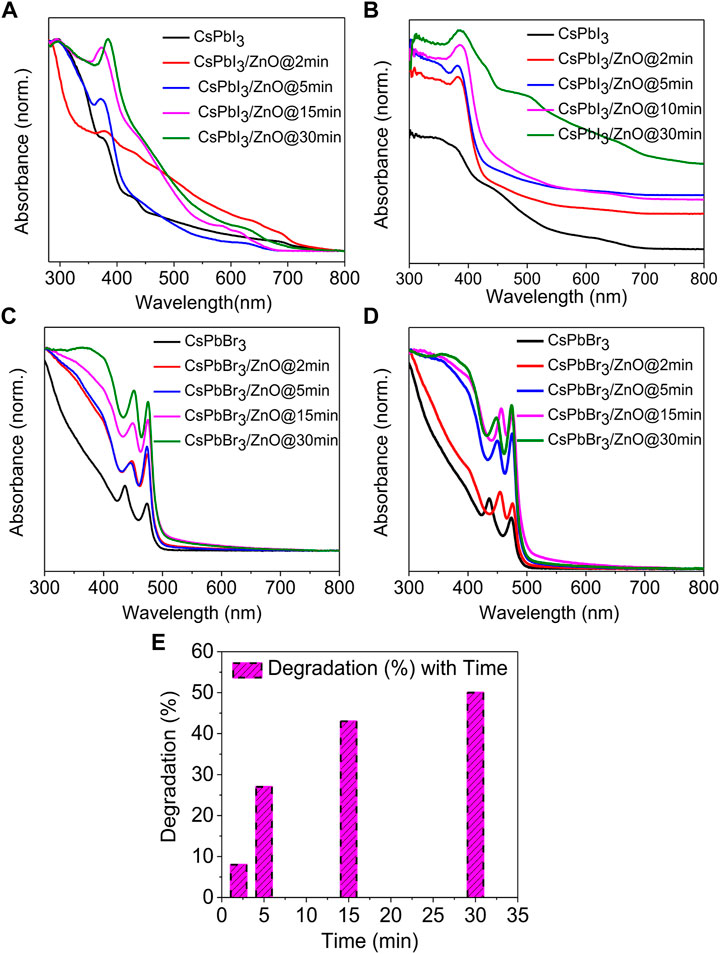
FIGURE 4. UV-visible absorbance spectra of CsPbI3 and CsPbI3/ZnO as a function of time at (A) 100°C and (B) 160°C. CsPbBr3 and CsPbBr3/ZnO at (C) 100°C and (D) 160°C. (E) Degradation efficiency with time for CsPbI3/ZnO heterostructure.
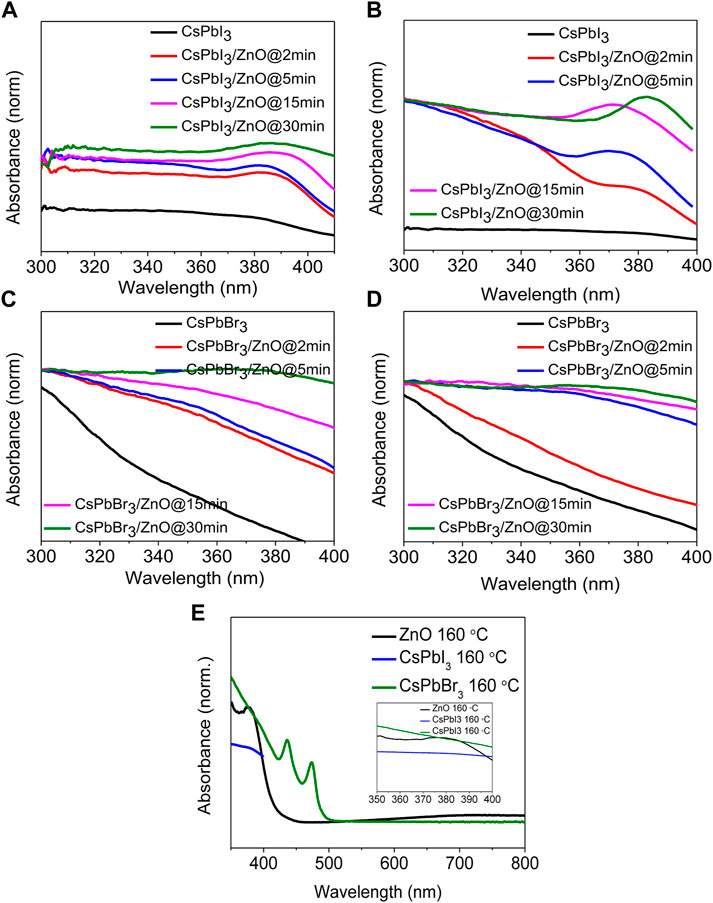
FIGURE 5. Absorbance spectra of inorganic CsPbI3 and CsPbI3/ZnO heterostructures at (A) 100°C and (B) 160 C. Absorbance spectra of inorganic CsPbBr3 and CsPbBr3/ZnO heterostructures at (C) 100 C and (D) 160 C. (E) Absorbance spectra for the controlled samples of CsPbI3 and ZnO. Inset shows the close view.
The cycling stability of a photocatalyst is important for practical usage. Therefore, recycling experiments are carried out for CsPbI3/ZnO. The results are shown in Figure 5C suggested that the catalyst’s performance is negligible after four continuous cycles of photocatalyst reutilization. This study confirms that CsPbI3/ZnO is highly stable to heavy metal phtodegradation. The post-xps analysis indicted that the peaks for all the core elements are greatly reduced with decreasing intensity, confirming the photodegradation of heavy metals (d).
3.3 Cyclic voltammograms and AC impedance analysis of the CsPbI3/ZnO heterostructure
The electrochemical performance of CsPbI3/ZnO was assessed to confirm its potential as a photocatalyst. In Figure 6E, the oxidative and reductive properties of the CsPbI3/ZnO heterostructure were investigated using the characteristic cyclic voltammogram test at a scanning rate of 0.1 mV s−1. The CV curve for the first cycle presented characteristic discharge peaks at 0.82 and 1.41 V, which are the reduction peaks that confirm the successful reaction of CsPbI3/ZnO heterostructure. Moreover, cathodic peaks confirm electrolyte decomposition and the formation of an SEI passivation layer on the surface of anode material during discharge. Two oxidative peaks were observed in the anodic sweep at 1.25 V, corresponding to a gradual Zn+ withdrawal process in CsPbI3/ZnO. The CV test confirms the oxidation and reduction potentials of CsPbI3/ZnO heterostructure. The electrochemical impedance spectra with a wide-range of frequency as shown in Figure 6F. The Nyquist curve consists of a semicircle and an oblique line from high to medium-frequency and high to low-frequency regions, respectively. The semicircle in the high-frequency region defined the extent of resistance to electron transfer, confirming the negligible ion transformation and bulk polarization, while the straight line represents the high electrical conductivity of the CsPbI3/ZnO catalyst. The result showed that the CsPbI3/ZnO catalyst had high electrical conductivity and was conductive to zinc ion diffusion.
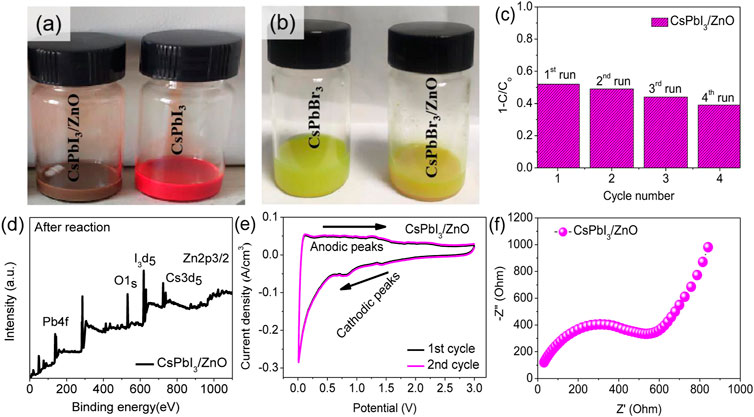
FIGURE 6. Adsorption of (A) CsPbI3/ZnO and CsPbI3, and (B) CsPbBr3/ZnO and CsPbBr3 heterostructure. (C) Cyclic stability of CsPbI3/ZnO under UV light. (D) Post-XPS analysis of CsPbI3/ZnO (E) Cyclic voltammograms of CsPbI3/ZnO at a scanning rate of 0.1 mV s−1 and (F) Nyquist plot at room temperature for CsPbI3/ZnO heterostructure.
3.4 Photocatalytic mechanism
Figure 7 depicts the photocatalytic mechanism of CsPbX3/ZnO heterostructure. Generally, the photocatalytic process initiates with the generation of electron hole pairs (ehp) in the presence of light with an energy greater than or equal to the bandgap (Wang et al., 2022). The electrons from the valence band (VB) are excited towards the conduction band (CB) of perovskites, thereby generating photo-active species including e− and h+. Such photogenerated e− would either combine with the h+ or be arbitrarily shifted to the surface of the photocatalysts, further trapped by O2 to generate O2.-.for the further generation of . OH (Wei et al., 2021). The formation of heterostructure has been regarded as an effective strategy for the retardation of the e/h recombination by keeping the utmost redox potential of a photocatalyst (Shen et al., 2022). Particularly, in this case of perovskite heterostructure, the generation of ehp occurs on the surface of CsPbX3 when an electron (eˉ) in the CsPbX3/ZnO shifts (excites) from the valence band (VB, HOMO) to the conduction band (CB, LUMO) and leaves holes (h+) in the HOMO region, known as the generation of ehp. In the CsPbX3 perovskite, these as-produced ehp react with H2O and O2, generating excessive hydroxyl radicals (.OH) and oxygen molecules (O2−) (Zhang et al., 2019). After the introduction of ZnO, holes can be shifted from the halide perovskite to the surface of ZnO, generating (.OH). The overall mechanism can be summarized as follows:
Thus, the produced radicals help in the reduction of heavy metals into environment-friendly end products like CO2 and H2O (Das et al., 2020; Khan and Pathak, 2020). Hence, these synthesized CsPbX3/ZnO heterostructures are quite useful in removing heavy metals and toxic products from the environment, soil, and different industry.
4 Conclusion
In conclusion, we have successfully synthesized the CsPbX3/ZnO heterostructure with zinc oxide as a photocatalyst using a standard hot injection method. The as-synthesized material was characterized by using different structural characterizations to confirm the successful formation and its morphological structure. The CsPbI3/ZnO heterostructure exhibits a degradation efficiency of 52%, which is higher than the degradation efficiency of CsPbI3 with various other dyes. The CV and EIS analysis show high oxidation and reduction characteristics, as well as superior resistance to electron transfer, confirming the electrical conductivity. Thus, this synthesized heterostructure has the potential to be used for heavy metal degradation as well as a water cleaning catalyst.
Data availability statement
The original contributions presented in the study are included in the article/Supplementary Material, further inquiries can be directed to the corresponding author.
Author contributions
SG: Conception and design of the study, drafting of the manuscript, Acquisition of data SB: Conception and design of study, Review and edit manuscript GL: Review and editing, Supervision. MI: Review and edit manuscript.
Acknowledgments
The authors would like to acknowledge the postdoctoral research fellowship program of Shenzhen University and appreciate the technical support at the University for assistance in using the equipment.
Conflict of interest
The authors declare that the research was conducted in the absence of any commercial or financial relationships that could be construed as a potential conflict of interest.
Publisher’s note
All claims expressed in this article are solely those of the authors and do not necessarily represent those of their affiliated organizations, or those of the publisher, the editors and the reviewers. Any product that may be evaluated in this article, or claim that may be made by its manufacturer, is not guaranteed or endorsed by the publisher.
References
Akkerman, Q. A., D’innocenzo, V., Accornero, S., Scarpellini, A., Petrozza, A., Prato, M., et al. (2015). Tuning the optical properties of cesium lead halide perovskite, 137, 10276–10281.Nanocrystals by anion exchange reactionsJ. Am. Chem. Soc,
An, R., Zhang, F., Zou, X., Tang, Y., Liang, M., Oshchapovskyy, I., et al. (2018). Photostability and photodegradation processes in colloidal CsPbI3 perovskite quantum dots. ACS Appl. Mat. Interfaces 10, 39222–39227. doi:10.1021/acsami.8b14480
Batool, S., Idrees, M., Javed, M. S., Saleem, M., and Kong, J. (2020). Engaging tailored capacity of layered WS2 via sulphur bonding coupled with polyetherimide (WS2@NC) nanocomposite for high power and improved lithium-ion storage. Mater. Chem. Phys. 246, 122832. doi:10.1016/j.matchemphys.2020.122832
Cai, M., Wang, C., Liu, Y., Yan, R., and Li, S. (2022). Boosted photocatalytic antibiotic degradation performance of Cd0. 5Zn0. 5S/carbon dots/Bi2WO6 S-scheme heterojunction with carbon dots as the electron bridge. Sep. Purif. Technol. 300, 121892. doi:10.1016/j.seppur.2022.121892
Cheng, L., Xiang, Q., Liao, Y., and Zhang, H. (2018). CdS-based photocatalysts. Energy Environ. Sci. 11, 1362–1391. doi:10.1039/c7ee03640j
Das, S., Paul, T., Maiti, S., and Chattopadhyay, K. K. (2020). Ambient processed CsPbX3 perovskite cubes for photocatalysis. Mater. Lett. 267, 127501. doi:10.1016/j.matlet.2020.127501
Deng, W., Li, J., Jin, J., Mishra, D. D., Xin, J., Lin, L., et al. (2020). Fast and low temperature processed CsPbI3 perovskite solar cells with ZnO as electron transport layer. J. Power Sources 480, 229134. doi:10.1016/j.jpowsour.2020.229134
Fujishima, A., Rao, T. N., and Tryk, D. A. (2000). Titanium dioxide photocatalysis. J. Photochem. Photobiol. C Photochem. Rev. 1, 1–21. doi:10.1016/s1389-5567(00)00002-2
Guan, Z., Wu, Y., Wang, P., Zhang, Q., Wang, Z., Zheng, Z., et al. (2019). Perovskite photocatalyst CsPbBr3-xIx with a bandgap funnel structure for H2 evolution under visible light. Appl. Catal. B Environ. 245, 522–527. doi:10.1016/j.apcatb.2019.01.019
Gull, S., Yang, Z., Wu, W., Rao, Q., Zhang, J., and Li, W. (2020). Multi-phased cesium lead iodide quantum dots with large Stokes shift. Mater. Lett. 271, 127765. doi:10.1016/j.matlet.2020.127765
Hitam, C., and Jalil, A. (2020). A review on exploration of Fe2O3 photocatalyst towards degradation of dyes and organic contaminants. J. Environ. Manag. 258, 110050. doi:10.1016/j.jenvman.2019.110050
Huang, N., Shu, J., Wang, Z., Chen, M., Ren, C., and Zhang, W. (2015). One-step pyrolytic synthesis of ZnO nanorods with enhanced photocatalytic activity and high photostability under visible light and UV light irradiation. J. Alloys Compd. 648, 919–929. doi:10.1016/j.jallcom.2015.07.039
Idrees, M., Batool, S., Hussain, Q., Ullah, H., Al-Wabel, M. I., Ahmad, M., et al. (2016). High-efficiency remediation of cadmium (Cd2+) from aqueous solution using poultry manure-and farmyard manure-derived biochars. Sep. Sci. Technol. 51, 2307–2317. doi:10.1080/01496395.2016.1205093
Jiang, Y., Liao, J.-F., Xu, Y.-F., Chen, H.-Y., Wang, X.-D., and Kuang, D.-B. (2019). Hierarchical CsPbBr3 nanocrystal-decorated ZnO nanowire/macroporous graphene hybrids for enhancing charge separation and photocatalytic CO2 reduction. J. Mat. Chem. A Mat. 7, 13762–13769. doi:10.1039/c9ta03478a
Johar, M. A., Afzal, R. A., Alazba, A. A., and Manzoor, U. (2015). Photocatalysis and bandgap engineering using ZnO nanocomposites. Adv. Mater. Sci. Eng., 1–22. 2015, doi:10.1155/2015/934587
Kar, M., Chakraborty, R., Patel, U., Ray, S., Acharya, T., Goswami, C., et al. (2022). Impact of Zn-doping on the composition, stability, luminescence properties of silica coated all-inorganic cesium lead bromide nanocrystals and their biocompatibility. Mater. Today Chem. 23, 100753. doi:10.1016/j.mtchem.2021.100753
Karami, M., Ghanbari, M., Amiri, O., and Salavati-Niasari, M. (2020). Enhanced antibacterial activity and photocatalytic degradation of organic dyes under visible light using cesium lead iodide perovskite nanostructures prepared by hydrothermal method. Sep. Purif. Technol. 253, 117526. doi:10.1016/j.seppur.2020.117526
Khan, S. H., and Pathak, B. (2020). Zinc oxide based photocatalytic degradation of persistent pesticides: A comprehensive review. Environ. Nanotechnol. Monit. Manag. 13, 100290. doi:10.1016/j.enmm.2020.100290
Kulkarni, S. A., Mhaisalkar, S. G., Mathews, N., and Boix, P. P. (2019). Perovskite nanoparticles: Synthesis, properties, and novel applications in photovoltaics and LEDs. Small Methods 3, 1800231. doi:10.1002/smtd.201800231
Lai, M., Kong, Q., Bischak, C. G., Yu, Y., Dou, L., Eaton, S. W., et al. (2017). Structural, optical, and electrical properties of phase-controlled cesium lead iodide nanowires. Nano Res. 10, 1107–1114. doi:10.1007/s12274-016-1415-0
Le, A. T., Pung, S.-Y., Sreekantan, S., Matsuda, A., and Huynh, D. P. (2019). Mechanisms of removal of heavy metal ions by ZnO particles. Heliyon 5, e01440. doi:10.1016/j.heliyon.2019.e01440
Lee, G.-J., and Wu, J. J. (2017). Recent developments in ZnS photocatalysts from synthesis to photocatalytic applications—a review. Powder Technol. 318, 8–22. doi:10.1016/j.powtec.2017.05.022
Li, S., Cai, M., Liu, Y., Zhang, J., Wang, C., Zang, S., et al. (2022a). In situ construction of a C3N5 nanosheet/Bi2WO6 nanodot S-scheme heterojunction with enhanced structural defects for the efficient photocatalytic removal of tetracycline and Cr(vi). Inorg. Chem. Front. 9, 2479–2497. doi:10.1039/d2qi00317a
Li, S., Cai, M., Wang, C., Liu, Y., Li, N., Zhang, P., et al. (2022b). Rationally designed Ta3N5/BiOCl S-scheme heterojunction with oxygen vacancies for elimination of tetracycline antibiotic and Cr(VI): Performance, toxicity evaluation and mechanism insight. J. Mater. Sci. Technol. 123, 177–190. doi:10.1016/j.jmst.2022.02.012
Li, S., Wang, C., Cai, M., Liu, Y., Dong, K., and Zhang, J. (2022c). Designing oxygen vacancy mediated bismuth molybdate (Bi2MoO6)/N-rich carbon nitride (C3N5) S-scheme heterojunctions for boosted photocatalytic removal of tetracycline antibiotic and Cr(VI): Intermediate toxicity and mechanism insight. J. Colloid Interface Sci. 624, 219–232. doi:10.1016/j.jcis.2022.05.151
Li, S., Wang, C., Cai, M., Yang, F., Liu, Y., Chen, J., et al. (2022d). Facile fabrication of TaON/Bi2MoO6 core-shell S-scheme heterojunction nanofibers for boosting visible-light catalytic levofloxacin degradation and Cr(VI) reduction. Chem. Eng. J. 428, 131158. doi:10.1016/j.cej.2021.131158
Li, S., Cai, M., Liu, Y., Wang, C., Yan, R., and Chen, X. (2023). Constructing Cd0. 5Zn0. 5S/Bi2WO6 S-scheme heterojunction for boosted photocatalytic antibiotic oxidation and Cr(VI) reduction. Adv. Powder Mater. 2, 100073. doi:10.1016/j.apmate.2022.100073
Liu, Y., and Ma, Z. (2021). Combining g-C3N4 with CsPbI3 for efficient photocatalysis under visible light. Colloids Surfaces A Physicochem. Eng. Aspects 628, 127310. doi:10.1016/j.colsurfa.2021.127310
Lu, C., Li, H., Kolodziejski, K., Dun, C., Huang, W., Carroll, D., et al. (2018). Enhanced stabilization of inorganic cesium lead triiodide (CsPbI3) perovskite quantum dots with tri-octylphosphine. Nano Res. 11, 762–768. doi:10.1007/s12274-017-1685-1
Nipane, D., Thakare, S., and Khati, N. (2013). Synthesis of novel ZnO having cauliflower morphology for photocatalytic degradation study. J. Catal., 1–8. 2013, doi:10.1155/2013/940345
Pirhashemi, M., Elhag, S., Adam, R. E., Habibi-Yangjeh, A., Liu, X., Willander, M., et al. (2019). n–n ZnO–Ag2CrO4 heterojunction photoelectrodes with enhanced visible-light photoelectrochemical properties. RSC Adv. 9, 7992–8001. doi:10.1039/c9ra00639g
Protesescu, L., Yakunin, S., Bodnarchuk, M. I., Krieg, F., Caputo, R., Hendon, C. H., et al. (2015). Nanocrystals of cesium lead halide perovskites (CsPbX3, X= Cl, Br, and I): Novel optoelectronic materials showing bright emission with wide color gamut. Nano Lett. 15, 3692–3696. doi:10.1021/nl5048779
Ren, K., Yue, S., Li, C., Fang, Z., Gasem, K. A., Leszczynski, J., et al. (2022). Metal halide perovskites for photocatalysis applications. J. Mat. Chem. A Mat. 10, 407–429. doi:10.1039/d1ta09148d
Sajid, M. M., Shad, N. A., Javed, Y., Khan, S. B., Zhang, Z., Amin, N., et al. (2020). Preparation and characterization of Vanadium pentoxide (V2O5) for photocatalytic degradation of monoazo and diazo dyes. Surfaces Interfaces 19, 100502. doi:10.1016/j.surfin.2020.100502
Senapati, S., Srivastava, S. K., and Singh, S. B. (2012). Synthesis, characterization and photocatalytic activity of magnetically separable hexagonal Ni/ZnO nanostructure. Nanoscale 4, 6604–6612. doi:10.1039/c2nr31831h
Shen, X., Yang, J., Zheng, T., Wang, Q., Zhuang, H., Zheng, R., et al. (2020). Plasmonic pn heterojunction of Ag/Ag2S/Ag2MoO4 with enhanced Vis-NIR photocatalytic activity for purifying wastewater. Sep. Purif. Technol. 251, 117347. doi:10.1016/j.seppur.2020.117347
Shen, X., Yang, Y., Song, B., Chen, F., Xue, Q., Shan, S., et al. (2021a). Magnetically recyclable and remarkably efficient visible-light-driven photocatalytic hexavalent chromium removal based on plasmonic biochar/bismuth/ferroferric oxide heterojunction. J. Colloid Interface Sci. 590, 424–435. doi:10.1016/j.jcis.2021.01.095
Shen, X., Zhang, Y., Shi, Z., Shan, S., Liu, J., and Zhang, L. (2021b). Construction of C3N4/CdS nanojunctions on carbon fiber cloth as a filter-membrane-shaped photocatalyst for degrading flowing wastewater. J. Alloys Compd. 851, 156743. doi:10.1016/j.jallcom.2020.156743
Shen, X., Song, B., Shen, X., Shen, C., Shan, S., Xue, Q., et al. (2022). Rationally designed S-scheme heterojunction of C3N4/Bi2MoO6/carbon fiber cloth as a recyclable, macroscopic and efficient photocatalyst for wastewater treatment. Chem. Eng. J. 445, 136703. doi:10.1016/j.cej.2022.136703
Tang, C., Chen, C., Xu, W., and Xu, L. (2019). Design of doped cesium lead halide perovskite as a photo-catalytic CO 2 reduction catalyst. J. Mat. Chem. A Mat. 7, 6911–6919. doi:10.1039/c9ta00550a
Wang, Q., Tao, L., Jiang, X., Wang, M., and Shen, Y. (2019). Graphene oxide wrapped CH3NH3PbBr3 perovskite quantum dots hybrid for photoelectrochemical CO2 reduction in organic solvents. Appl. Surf. Sci. 465, 607–613. doi:10.1016/j.apsusc.2018.09.215
Wang, J.-C., Li, N., Idris, A. M., Wang, J., Du, X., Pan, Z., et al. (2021). Surface defect engineering of CsPbBr3 nanocrystals for high efficient photocatalytic CO2 reduction. Sol. RRL 5, 2100154. doi:10.1002/solr.202100154
Wang, C., Li, S., Cai, M., Yan, R., Dong, K., Zhang, J., et al. (2022). Rationally designed tetra (4-carboxyphenyl) porphyrin/graphene quantum dots/bismuth molybdate Z-scheme heterojunction for tetracycline degradation and Cr(VI) reduction: Performance, mechanism, intermediate toxicity appraisement. J. Colloid Interface Sci. 619, 307–321. doi:10.1016/j.jcis.2022.03.075
Wei, K., Faraj, Y., Yao, G., Xie, R., and Lai, B. (2021). Strategies for improving perovskite photocatalysts reactivity for organic pollutants degradation: A review on recent progress. Chem. Eng. J. 414, 128783. doi:10.1016/j.cej.2021.128783
Xu, Y.-F., Yang, M.-Z., Chen, B.-X., Wang, X.-D., Chen, H.-Y., Kuang, D.-B., et al. (2017). A CsPbBr3 perovskite quantum dot/graphene oxide composite for photocatalytic CO2 reduction. J. Am. Chem. Soc. 139, 5660–5663. doi:10.1021/jacs.7b00489
Yang, D., Cao, M., Zhong, Q., Li, P., Zhang, X., and Zhang, Q. (2019). All-inorganic cesium lead halide perovskite nanocrystals: Synthesis, surface engineering and applications. J. Mat. Chem. C Mat. 7, 757–789. doi:10.1039/c8tc04381g
Zhang, H., Feng, J., Wang, J., and Zhang, M. (2007). Preparation of ZnO nanorods through wet chemical method. Mater. Lett. 61, 5202–5205. doi:10.1016/j.matlet.2007.04.030
Zhang, Q., Tai, M., Zhou, Y., Zhou, Y., Wei, Y., Tan, C., et al. (2019). Enhanced photocatalytic property of γ-CsPbI3 perovskite nanocrystals with WS2. ACS Sustain. Chem. Eng. 8, 1219–1229. doi:10.1021/acssuschemeng.9b06451
Zhang, L., Liu, Y., He, Y., Zhang, X., Geng, C., Yang, R., et al. (2021a). Stable CsPbX3/ZnO Heterostructure nanocrystals for light-emitting application. J. Phys. Chem. Lett. 12, 10953–10957. doi:10.1021/acs.jpclett.1c03446
Zhang, Y., Liu, X., Yusoff, M., and Razali, M. H. (2021b). Photocatalytic and antibacterial properties of a 3D flower-like TiO2 nanostructure photocatalyst.Scanning, 2021, 3839235, doi:10.1155/2021/3839235
Zhao, G., Rui, K., Dou, S. X., and Sun, W. (2018). Heterostructures for electrochemical hydrogen evolution reaction: A review. Adv. Funct. Mat. 28, 1803291. doi:10.1002/adfm.201803291
Zhao, Y., Shi, H., Hu, X., Liu, E., and Fan, J. (2020). Fabricating CsPbX3/CN heterostructures with enhanced photocatalytic activity for penicillins 6-APA degradation. Chem. Eng. J. 381, 122692. doi:10.1016/j.cej.2019.122692
Keywords: cesium lead halide/zinc oxide, heterostructure, hot injection strategy, photocatalyst, degradation
Citation: Gull S, Batool S, Li G and Idrees M (2022) Synthesis of cesium lead halide perovskite/zinc oxide (CsPbX3/ZnO, X= Br, I) as heterostructure photocatalyst with improved activity for heavy metal degradation. Front. Chem. 10:1020484. doi: 10.3389/fchem.2022.1020484
Received: 18 August 2022; Accepted: 20 September 2022;
Published: 05 October 2022.
Edited by:
Shijie Li, Zhejiang Ocean University, ChinaReviewed by:
Yanping Liu, Zhejiang Ocean University, ChinaXiaofeng Shen, Zhejiang University of Science and Technology, China
Copyright © 2022 Gull, Batool, Li and Idrees. This is an open-access article distributed under the terms of the Creative Commons Attribution License (CC BY). The use, distribution or reproduction in other forums is permitted, provided the original author(s) and the copyright owner(s) are credited and that the original publication in this journal is cited, in accordance with accepted academic practice. No use, distribution or reproduction is permitted which does not comply with these terms.
*Correspondence: Muhammad Idrees, m.idrees8223@gmail.com Guijun Li, gliad@connect.ust.hk