- 1State Key Laboratory of Electronic Thin Films and Integrated Devices, School of Materials and Energy, University of Electronic Science and Technology of China, Chengdu, China
- 2Chongqing Medical and Pharmaceutical College, Chongqing, China
Single-atom catalysts (SACs) with isolated metal atoms dispersed on supports have attracted increasing attention due to their maximum atomic utilization and excellent catalytic performance in various electrochemical reactions. However, SACs with a high surface-to-volume ratio are fundamentally less stable and easily agglomerate, which weakens their activity. In addition, another issue that restricts the application of SACs is the low metal loading. Defect engineering is the most effective strategy for the precise synthesis of nanomaterials to catch and immobilize single atoms through the modulation of the electronic structure and coordination environment. Herein, in this mini-review, the latest advances in designing SACs by defect engineering have been first highlighted. Then, the heteroatom doping or intrinsic defects of carbon-based support and anion vacancies or cation vacancies of metal-based supports are systematically evaluated. Subsequently, the structure–activity relationships between a single-atom coupled defect structure and electrocatalytic performance are illustrated by combining experimental results and theoretical calculations. Finally, a perspective to reveal the current challenges and opportunities for controllable preparation, in situ characterization, and commercial applications is further proposed.
Introduction
With the increasing depletion of fossil fuels (e.g., coal, oil, and natural gas), exploiting renewable, sustainable, and eco-friendly fuels is quite urgent to alleviate the energy crisis in modern society (Mi et al., 2018; Guo and Zhang, 2020). At present, increasing attention is being devoted to electrochemical energy conversion and storage (e.g., fuel cells, water-splitting devices, storage batteries, artificial carbon, and nitrogen fixation) due to their high energy densities and environmental benefits (Wang et al., 2019; Ai et al., 2021; Chen et al., 2021). The operation of all of these technologies requires appropriate advanced electrocatalysts to reduce the energy barriers and accelerate the kinetics of chemical reactions, such as the hydrogen oxidation reaction (HOR), oxygen reduction reaction (ORR), hydrogen evolution reaction (HER), oxygen evolution reaction (OER), nitrogen reduction reaction (NRR), carbon dioxide reduction reaction (CO2RR), biomass oxidation reaction, and so on (Jiang et al., 2020; Zhou et al., 2020; Li et al., 2021a; Wang et al., 2022b; Wang et al., 2022c; Zhu et al., 2022). Accordingly, the state-of-the-art catalysts mainly depend on platinum group metals (PGMs, Pt, and Au), PGM-based oxides (IrO2 and RuO2), and PGM-based alloys (PtRu and PtPd) (Li et al., 2022; Yan et al., 2022; Zhao et al., 2022). Regrettably, the apparent activity, limited durability, high cost, and low storage of PGM-based electrocatalysts have significantly hampered the commercialization process. The intuitive strategy to overcome this bottleneck issue is to use single-atom catalysts (SACs), which not only profoundly expose the atoms to reduce the usage of PGMs but also trigger the immeasurable performance on account of the size effect and isolation effect (Qiao et al., 2011; Wang et al., 2021). However, the atomically dispersed metal atoms often inevitably suffer from migration and agglomeration on support (Xu et al., 2018). The reason for this is that SACs with a high surface-to-volume ratio are thermodynamically less stable. In addition, the high loading of SACs is also a great challenge for practical applications (Zhu et al., 2017; Sun et al., 2021). Therefore, the development of efficient and robust SACs with high atomic density and dispersion is still a challenge.
From the second law of thermodynamics, defects widely exist in nanomaterials. They could disturb or even break the periodic structure of crystals (Yan et al., 2017; Li et al., 2020). Subsequently, the electronic structure of the nanomaterials is redistributed, and then the adsorption energy of intermediate species is optimized. Consequently, the kinetics of catalytic reactions is accelerated (Yan et al., 2019). In addition to activating the intermediate species, defect engineering can be also used as an effective strategy to alter the surface-interfacial coordination environment of the supports to form strong intercalation for the capture of the metal species, such as particles, clusters, and even single atoms. Moreover, some defects (e.g., vacancies and edge sites) serve as an adsorbed site to catch and disperse single atoms. Such an excellent defective structure, by benefiting the capture of a single atom, leads to an extraordinarily high metal loading (∼20%) in SACs, which exceeds that of the traditional strategy with a very low metal loading of 1–2 wt% (Cheng et al., 2018). Furthermore, the defects and SACs synergistically improve the electrocatalytic performance in terms of selectivity, activity, and durability (Zhang et al., 2019b; Ban et al., 2021; Zhang et al., 2021). However, a deep understanding of the role of defects and SACs in the catalytic process is still lacking, and the interrelation between defects and SACs in synthetic catalysts is poorly recognized. More recently, several in situ spectral and microscopical instruments provide more in-depth information about the nanomaterials at the molecular and atomic levels, such as studying the growth process of electrocatalysts, observing the electrochemical reaction process directly, and analyzing the reaction mechanisms accurately (Zhang et al., 2019a; Wang et al., 2022a). These findings encourage us to further explore designing SACs by defect engineering.
Herein, in this mini-review, we first systematically summarize the latest advances in designing SACs by defect engineering. Subsequently, we turn our attention to addressing how the defective carbon-based supports (e.g., heteroatom doping and intrinsic defects) and defective metal-based supports (e.g., cation vacancies and anion vacancies) affect the formation of SACs. Then, the structure–activity relationships between a single-atom coupled defect structure and electrocatalytic ability are further discussed. At the end of this review, after an in-depth understanding of the single atom and defect structure, the prospects and challenges of defective SACs are proposed in terms of controllable preparation, in situ characterization, and practical application.
Defect engineering on carbon-based materials
Owing to their low prices, excellent electrical conductivity, high specific surface area, and robust stability in both acidic and alkaline electrolytes, carbon-based materials have been selected as the most routine support (Yang et al., 2017; Li et al., 2018). However, because of the different specific surface energy of metal and carbon, the weak interactions between single metal atoms and carbon support could cause the migration and aggregation of single metal atoms, and thus the electrocatalytic performance becomes poor. To solve these issues, significant interest has been devoted to the design and synthesis of defective carbon-based materials to disperse single metal atoms due to their controllable coordination-unsaturated environment and strong metal–support interactions (Tang et al., 2020; Khan et al., 2021). Heteroatom doping and intrinsic defects are the main defective carbons, which not only regulate the surface charge and electronic structure of carbons but also offer anchor sites for the immobilization of single metal atoms. Benefiting from such a unique structure, the isolated metal atoms should be firmly captured, and the catalytic performance of catalysts could be further improved. In this section, the recent studies of single atomic electrocatalysts supported on defective carbon support have been highlighted.
Single atomic metal–nitrogen–carbon has been widely studied as one of the most promising alternatives to PGM-based electrocatalysts for different electrochemical applications. The species, loading, and even the adjacent coordination environment of the single metal atom play a part in their performance (Zhao et al., 2021). Heteroatom doping is the most common defect in nanocarbon materials. Based on different electronegativities and atomic sizes, heteroatom (e.g., N, C, B, P, S, and O) defects can effectively alter the coordination structure of the single metal atom. For example, Gong and co-workers developed a single-Ni-atom-implanted nitrogen-doped carbon (NC) using a host–guest cooperative protection strategy (Gong et al., 2020). X-ray absorption spectroscopy (XAS) revealed that the coordination structure of Ni–N is finely tuned by the C-doping defect from Ni–N4 to Ni–N3C1 and Ni–N2C2. The electrocatalytic CO2 reduction tests exhibited that Ni–N–C with the lowest N coordination number (Ni–N2C2) presents the highest CO Faradaic efficiency of 98% and turnover frequency of 1,622 h−1, far superior to those of Ni–N3C1 and Ni–N4 (Figure 1A). Density functional theory (DFT) calculations showed that the doping defect in Ni–N2C2 could optimize the coordination number of Ni–N and then facilitate the formation of a COOH* intermediate, consequently improving their activity and selectivity. In addition to adjusting the coordination structure of single metal atoms, heteroatom doping can also regulate the electronic structure of single metal atom-based active sites. Li and co-workers synthesized Cu or Fe single atom anchored on an S, N-codoped carbon basal plane by a defect trapping strategy (Figure 1B) (Li et al., 2021a). Both Raman spectra and electron paramagnetic resonance (EPR) spectroscopy displayed that S-doping created numerous defects in NSC compared to that in NC. Experimentally, the Fe-NSC catalyst achieves the maximum NH4+ yield of 7.822 g.N.g−1Fe, Faradaic efficiency of 78%, and lower charge-transfer resistance. XAS and theoretical calculations illustrated that S-doping induces an asymmetric charge distribution and redistribution, thus increasing the interaction between single atom Fe and defect carbon and resulting in efficient electrochemical denitrification. Apart from dispersing monometallic atoms, bimetallic atoms could be also anchored by doping defects. Zhang and co-workers reported a double atomic Co-Pt site supported on an N-doped defective carbon (denoted as A-CoPt-NC) by electrochemical activation (Zhang et al., 2018a). The EXAFS data and transmission electron microscopy (TEM) image showed that the N-doping defect takes part in capturing a single Co atom and then benefits from coordinating with Pt (Figure 1C). The optimal catalysts exhibited ultrahigh ORR performance and 267 times higher mass activity compared to commercial Pt/C. Theoretically, this dramatic improvement in ORR activity is ascribed to the synergetic effects of bimetallic atom (Co-Pt) and defect supports, which alters the d-orbital shift and the coordination structures of atomic metals.
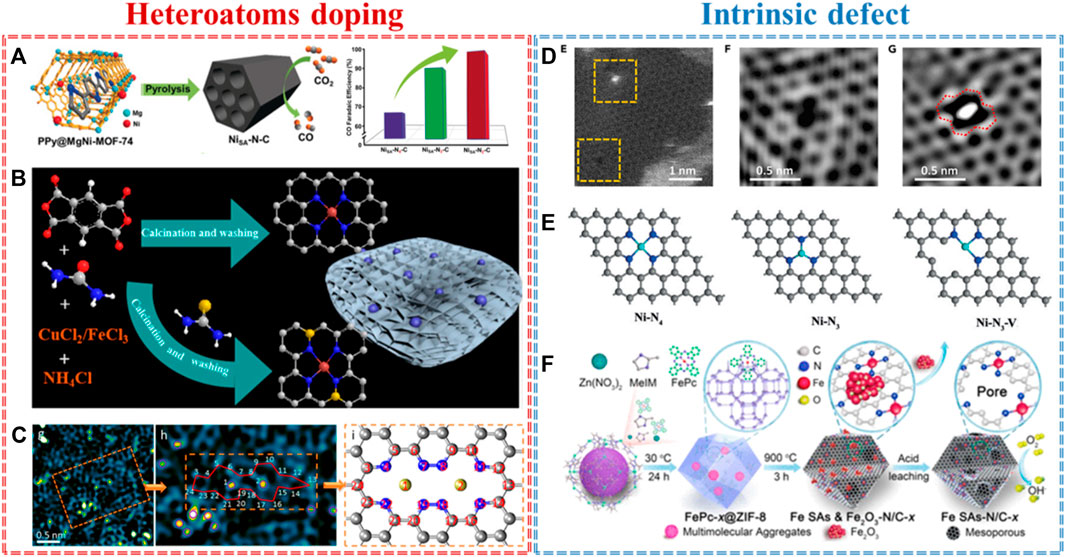
FIGURE 1. Heteroatom doping: (A) single atomic Ni–Nx–C (x = 2, 3, and 4) for CO2RR (Gong et al., 2020), (B) Cu/Fe–N(S)C SACs with defect-rich carbon for electrochemical denitrification (Li et al., 2021), (C) atomic Co-Pt supported on N doped carbon for ORR (Zhang et al., 2018a). Intrinsic defects: (D) graphene defects trap atomic Ni species for HER and OER (Zhang et al., 2018b), (E) vacancy-defect Ni SACs for CO2RR (Rong et al., 2020), and (F) edge-hosted Fe-N4 moieties in Fe–N–C SACs for ORR (Jiang et al., 2018).
After a deeper understanding of doping defects, we found that heteroatoms play an important role in activating adjacent carbon atoms to improve the performance of electrocatalysts, revealing that the truly active sites are carbon atoms themselves. Thus, we have reasons to believe that the intrinsic defects in carbon-based supports are more precious than heteroatom doping. For example, Zhang and co-workers constructed atomically dispersed Ni atoms trapped on graphene defects (A-Ni@DG) by a facile acid-leaching strategy (Zhang et al., 2018b). Both the HAADF-STEM image (Figure 1D) and XAS results showed that the intrinsic defect in graphene not only serves as an anchored site to capture a single Ni atom but also tailors the electronic densities of state (DOSs) of Ni species. Such an excellent structure, by precisely controlling the coordination environment of Ni, leads to exceptionally high performance in electrochemical water-splitting with an ultralow overpotential of 70 mV for HER and 270 mV for OER at 10 mA cm−2, which surpasses that of the state-of-the-art Pt/C and Ir/C catalysts. As well as pure intrinsic defects, vacancy defects are widely studied in SACs. For example, Chen and co-workers prepared a vacancy-defect Ni–N3–V single-atom catalyst by cleaving the weaker Ni–O interaction in the precursors of Ni–N3O–C at a high temperature (Rong et al., 2020). The Ni–N3–V showed an extremely high Faradaic efficiency (over 90%) and a record high turnover frequency (1.35 * 105 h−1), which is much higher than those of Ni–N4 SACs. This enhancement is owed to the vacancy defect in Ni–N–C, which increases the CO* desorption energy of active sites (Figure 1E). Not only vacancy defects but also the edge sites in SACs could affect the coordinate structure of metal-N4. Jiang and co-workers built an atomically dispersed Fe-N4 site embedded in the edge of three-dimensional (3D) hierarchically porous carbon by encapsulating iron (II) phthalocyanine (FePc) into the cavity of ZIF-8 by edge-site engineering (Jiang et al., 2018). The micro–mesoporous carbon is formed by the aggregation of excessive FePc molecules through the Kirkendall effect, and subsequently, the Fe–N4 site is in situ generated on the edge of pore carbon (Figure 1F). The synergistic effect between single metal sites and defective carbons delivers superior ORR performance with a half-wave potential of 0.915 V and a high atom-utilization efficiency up to a 10-fold enhancement compared to any Fe-based catalyst. DFT calculations further demonstrated that edge-N atoms play a vital role in tuning the surface charge of the Fe–N4 structure and then optimizing the adsorption energy of oxygen species to improve the ORR activity. In general, these results demonstrate that heteroatom doping and intrinsic defects benefit the formation of strong interfacial interactions between single metal atoms and the defective carbon, finely controlling the electronic structures and the coordination environment of the single-atom catalysts for electrocatalysis.
Defect engineering on metal-based materials
As summarized earlier, carbon-based defective materials are cheap and a fine support for capturing single atoms. However, the corrosion of carbon under high potentials is an inevitable problem in the electrocatalytic process, which probably induces the collapse of catalysts (Xie et al., 2014; Qiao et al., 2019). Recently, due to facile syntheses and stability in high potentials, increasing attention has been paid to metal-based materials, such as metal oxide, metal nitride, metal sulfide, and metal phosphide. Meanwhile, highly dispersed SACs have been well anchored on metal-based supports by various defects, such as cation vacancies and anion vacancies (Tan et al., 2022; Xiao et al., 2022). In this part, we turn our focus to metal-based defective materials stabilizing single metal atoms.
The most common and popular anion vacancies in metal-based materials are oxygen vacancies. Benefiting from the low formation energy of oxygen vacancies, the physicochemical properties of defective metal oxides may be changed, and the oxygen vacancies could be also used as the anchored sites to capture single atoms. For example, Yin and co-workers demonstrated a co-electrodeposition method to prepare Ir single atom coupling with oxygen vacancies on ultrathin NiCo2O4 porous nanosheets (Ir-NiCo2O4 NSs) (Yin et al., 2020). Figure 2A further verifies the defect structure and single atom of Ir-NiCo2O4 NSs by HAADF-STEM. The lower coordinated Co sites near the oxygen vacancies play an important role in anchoring atomic Ir and then facilitate electron exchange and transfer. In addition, the Ir-Ox sites not only protect the chemical state of Co during the OER but also optimize the adsorption energy of H and O on the Co site, which could ensure the utilization of Co-based activity sites. As a result, Ir-NiCo2O4 NSs showed an excellent OER activity of 240 mV overpotential at 10 mA cm−2 and robust stability of 70 h under the condition of acid electrolyte. Additionally, Tong and co-workers documented that the defect-rich W18O49 support could easily catch the single Fe atom by generating oxygen vacancy for NRR (Tong et al., 2020). Zhang and co-workers fabricated oxygen vacancy MXene to stabilize atomic Pt for HER (Zhang et al., 2022). Except for oxygen vacancy, the sulfur vacancy-based materials also act well for capturing single atoms. Gong and co-workers reported the simultaneous modulation of the mesoscale diffusion and Mo-Fe-C active site formation over monodispersed hollow Fe@MoS2-C sub–microreactors (Gong et al., 2022). A unique microenvironment, used by the sulfur vacancies and intercalated carbon, could catch Fe single atom to form the Fe@MoS2-C sub–microreactor. Owing to the rich sulfur vacancies and mesoscale diffusion, the Fe@MoS2-C exhibited an improved OER performance compared to the Fe-based SACs reported in the data. The theoretical calculation revealed the stability of Mo–Fe–C coordination, the electron transfer channel of “MoOx → Fe → carbon,” and the favorable d-band center in the dual-anchoring model (Figure 2B).
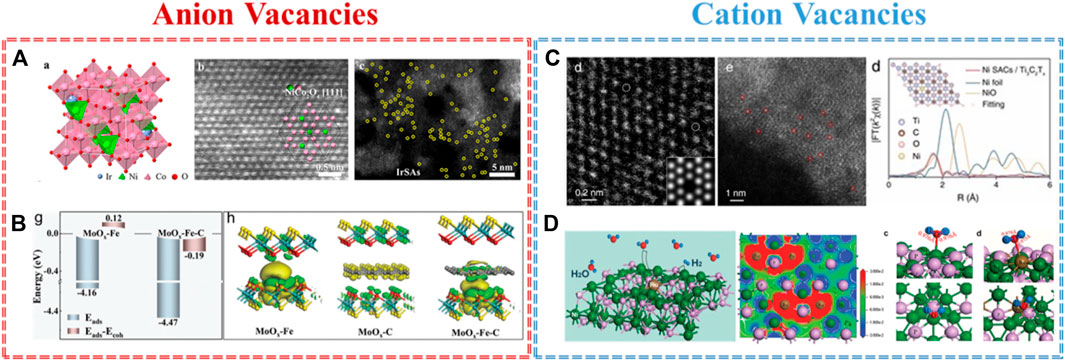
FIGURE 2. Anion vacancies: (A) Ir SACs supported on O vacancy in NiCo2O4 for OER (Yin et al., 2020) and (B) Fe SACs supported on S vacancy in MoS2-C for HER and OER (Gong et al., 2022). Cation vacancies: (C) Ni SACs supported on Ti vacancy in MXene for hydrazine oxidation reaction (Zhou et al., 2022) and (D) Ru SACs supported on Ni vacancy in Ni5P4 for HER (He et al., 2020).
In addition to the anion vacancies, the cation vacancies are also delivered to engineer the high-performance SACs because of their various electron and orbital distributions. For instance, Zhou and co-workers prepared titanium vacancy as structural defects that serve to catch and anchor Ni single atoms by a ‘self-reduction’ strategy (Zhou et al., 2022). The cation vacancy-rich Ti3C2Tx MXene acts simultaneously as a support and reductant during the nucleation of Ni SACs. Figure 2C further confirms the structure of Ni NPs/Ti3C2Tx in terms of the cation vacancies in Ti3C2Tx, the Ni single atom trapped on Ti vacancies, and the coordinate structure of Ni in Ni NPs/Ti3C2Tx. Experimentally, the Ni NPs/Ti3C2Tx catalysts exhibited an ultralow onset potential of -0.03 V (vs. RHE) and a negligible activity loss during a stability test of 24,000 s. Theoretically, this dramatic increase in hydrazine oxidation reaction activity is ascribed to the redistribution of electronic density of states by the strong interaction between the Ni single atom and adjacent C atoms. Subsequently, He and co-workers constructed nickel-vacancy-rich nickel hydroxides as a support precursor to capture the atomic Ru and for the subsequent phosphorization treatment to obtain Ni5P4-Ru (He et al., 2020). The physical characterizations, such as XAS, STEM, and EPR results, affirmed the strong coupling between Ni vacancies and Ru single atoms. Benefiting from the single atom coupled vacancies defect, Ni5P4-Ru achieves an ultralow overpotential of 54 mV at 10 mA cm−2 and long-term stability for OER. Both meticulous spectroscopic analysis and theoretical calculations revealed that single Ru atom coupled Ni vacancies could induce localized structure polarization, which decreases the reaction barrier of water dissociation and optimizes the hydrogen adsorption free energy on Ru sites (Figure 2D). Generally speaking, understanding the positive effect of cation vacancies and anion vacancies in metal-based materials provides further direct evidence and information to guide us toward a rational and precise SAC design at the molecular and atomic levels.
Conclusion and outlook
SACs, as economical alternatives to noble metal-based catalysts, presented excellent catalytic performances for various electrochemical applications because of their well-defined active centers, maximum atomic utilization, explicit coordinate structure, and strong single-atom–support interactions. However, SACs with a high surface-to-volume ratio are thermodynamically less stable. Herein, in this mini-review, we paid attention to the high-efficiency capture and immobilization of SACs by defect engineering. Defects not only tailor the surrounding electronic structure and coordination environment of support but also serve as the anchor sites to catch the isolated single atom and prevent the migration and agglomeration of SACs. Consequently, the electrocatalytic performance is further enhanced by defect engineering and single-atom catalysts simultaneously. Additionally, with the development of synthesis strategies, characterization techniques, and theoretical modelings, the defective SACs have achieved significant progress in recent decades. However, there are always many difficulties and challenges to be explored.
(i) Precisely controlling the formation of SACs by defect engineering
The electrocatalytic performances of SACs are limited by the loading of single atoms. However, the conventional strategies for the construction of SACs are usually with low metal loading due to their extremely high surface energy. Defect engineering could tune the electronic structure of support and then optimize the adsorption energy of anchored sites to facilitate the capture of single metal atoms. Based on the strong interaction between defects and single atoms, an increasing number of isolated metal atoms can be trapped and stabilized on the defective support to achieve a high metal loading. Therefore, the exploration of defective supports benefits carrying out the high metal loading in SACs and provides a new opportunity for maximum utilization of catalysts.
(ii) Exploring the structural evolution of defective SACs via advanced characterization methods under the operational process
Harsh electrocatalytic processes (e.g., long-term operation in high potentials, strongly acidic or alkaline electrolyte) may induce the structural evolution of defective SACs. In addition, the structural evolutions of active sites in previous research studies are almost from theoretical simulations. Thus, it is quite urgent to develop advanced characterization techniques to analyze and study the evolution of active sites in the electrocatalytic reaction directly. Coupling with experimental results and theoretical calculation will provide more invaluable information for further detecting the truly active site under the operational process.
(iii) Large-scale synthesis of defective SACs for practical application
In the traditional fabrication of SACs, the routine precursors are metal–organic frameworks, and the conventional approach is atomic layer deposition. In addition, the generation of defects in supports is a high-energy process. Thus, the synthesis cost, yields, and efficiency of defective SACs are the major challenges, which limit the actual production. Accordingly, the development of an efficient strategy for large-scale synthesis of defective SACs is vital to satisfy the potential demands of their practical application.
Author contributions
The mini-review is designed and executed by WL, JJ, and YZ. The original draft was written by WL and JJ and edited by ZC, XJ, and YZ.
Funding
The authors acknowledge the support received from the University of Electronic Science and Technology of China Scientific Research Foundation (Y030212059003045), Talent Start-up Funds (A1098531023601208), the China Postdoctoral Science Foundation (2021TQ0059 and 2022M710610), and 2021 Talent Introduction Project of Chongqing Medical and Pharmaceutical College (ygz2021104).
Conflict of interest
The authors declare that the research was conducted in the absence of any commercial or financial relationships that could be construed as a potential conflict of interest.
Publisher’s note
All claims expressed in this article are solely those of the authors and do not necessarily represent those of their affiliated organizations, or those of the publisher, the editors, and the reviewers. Any product that may be evaluated in this article, or claim that may be made by its manufacturer, is not guaranteed or endorsed by the publisher.
References
Ai, T., Bao, S., and Lu, J. (2021). Core-shell structured PtxMoy@TiO2 nanoparticles synthesized by reverse microemulsion for methanol electrooxidation of fuel cells. Front. Chem. 9, 667754. doi:10.3389/fchem.2021.667754
Ban, J., Wen, X., Xu, H., Wang, Z., Liu, X., Cao, G., et al. (2021). Dual evolution in defect and morphology of single-atom dispersed carbon based oxygen electrocatalyst. Adv. Funct. Mat. 31 (19), 2010472. doi:10.1002/adfm.202010472
Chen, W., Wu, B., Wang, Y., Zhou, W., Li, Y., Liu, T., et al. (2021). Deciphering the alternating synergy between interlayer Pt single-atom and NiFe layered double hydroxide for overall water splitting. Energy Environ. Sci. 14 (12), 6428–6440. doi:10.1039/D1EE01395E
Cheng, Y., Zhao, S., Johannessen, B., Veder, J.-P., Saunders, M., Rowles, M. R., et al. (2018). Atomically dispersed transition metals on carbon nanotubes with ultrahigh loading for selective electrochemical carbon dioxide reduction. Adv. Mater. 30 (13), 1706287. doi:10.1002/adma.201706287
Gong, F., Liu, M., Gong, L., Ye, S., Jiang, Q., Zeng, G., et al. (2022). Modulation of Mo–Fe–C sites over mesoscale diffusion-enhanced hollow sub-micro reactors toward boosted electrochemical water oxidation. Adv. Funct. Mat. 32, 2202141. doi:10.1002/adfm.202202141
Gong, Y.-N., Jiao, L., Qian, Y., Pan, C.-Y., Zheng, L., Cai, X., et al. (2020). Regulating the coordination environment of MOF-templated single-atom nickel electrocatalysts for boosting CO2 reduction. Angew. Chem. Int. Ed. 59 (7), 2705–2709. doi:10.1002/anie.201914977
Guo, S., and Zhang, Q. (2020). Emerging materials for energy catalysis. Adv. Energy Mat. 10 (11), 2000484. doi:10.1002/aenm.202000484
He, Q., Tian, D., Jiang, H., Cao, D., Wei, S., Liu, D., et al. (2020). Achieving efficient alkaline hydrogen evolution reaction over a Ni5P4 catalyst incorporating single-atomic Ru sites. Adv. Mat. 32 (11), 1906972. doi:10.1002/adma.201906972
Jiang, J., Ding, W., Li, W., and Wei, Z. (2020). Freestanding single-atom-layer Pd-based catalysts: Oriented splitting of energy bands for unique stability and activity. Chem 6 (2), 431–447. doi:10.1016/j.chempr.2019.11.003
Jiang, R., Li, L., Sheng, T., Hu, G., Chen, Y., and Wang, L. (2018). Edge-site engineering of atomically dispersed Fe–N4 by selective C–N bond cleavage for enhanced oxygen reduction reaction activities. J. Am. Chem. Soc. 140 (37), 11594–11598. doi:10.1021/jacs.8b07294
Khan, K., Yan, X., Yu, Q., Bae, S.-H., White, J. J., Liu, J., et al. (2021). Stone-Wales defect-rich carbon-supported dual-metal single atom sites for Zn-air batteries. Nano Energy 90, 106488. doi:10.1016/j.nanoen.2021.106488
Li, J., Li, M., An, N., Zhang, S., Song, Q., Yang, Y., et al. (2021b). Atomically dispersed Fe atoms anchored on S and N co-doped carbon for efficient electrochemical denitrification. Proc. Natl. Acad. Sci. U. S. A. 118(33), e2105628118. doi:10.1073/pnas.2105628118
Li, W., Ding, W., Jiang, J., He, Q., Tao, S., Wang, W., et al. (2018). A phase-transition-assisted method for the rational synthesis of nitrogen-doped hierarchically porous carbon materials for the oxygen reduction reaction. J. Mat. Chem. A Mat. 6 (3), 878–883. doi:10.1039/C7TA09435C
Li, W., Wang, D., Liu, T., Tao, L., Zhang, Y., Huang, Y.-C., et al. (2021a). Doping-modulated strain enhancing the phosphate tolerance on PtFe alloys for high-temperature proton exchange membrane fuel cells. Adv. Funct. Mat. 32 (8), 2109244. doi:10.1002/adfm.202109244
Li, W., Wang, D., Zhang, Y., Tao, L., Wang, T., Zou, Y., et al. (2020). Defect engineering for fuel-cell electrocatalysts. Adv. Mat. 32 (19), 1907879. doi:10.1002/adma.201907879
Li, W., Zhao, L., Jiang, X., Chen, Z., Zhang, Y., and Wang, S. (2022). Confinement engineering of electrocatalyst surfaces and interfaces. Adv. Funct. Mater., 202207727. doi:10.1002/adfm.202207727
Mi, H., Yang, X., Hu, J., Zhang, Q., and Liu, J. (2018). Carbothermal synthesis of nitrogen-doped graphene composites for energy conversion and storage devices. Front. Chem. 6, 501. doi:10.3389/fchem.2018.00501
Qiao, B. T., Wang, A. Q., Yang, X. F., Allard, L. F., Jiang, Z., Cui, Y. T., et al. (2011). Single-atom catalysis of CO oxidation using Pt1/FeOx. Nat. Chem. 3 (8), 634–641. doi:10.1038/Nchem.1095
Qiao, Z., Hwang, S., Li, X., Wang, C. Y., Samarakoon, W., Karakalos, S., et al. (2019). 3D porous graphitic nanocarbon for enhancing the performance and durability of Pt catalysts: A balance between graphitization and hierarchical porosity. Energy Environ. Sci. 12 (9), 2830–2841. doi:10.1039/c9ee01899a
Rong, X., Wang, H.-J., Lu, X.-L., Si, R., and Lu, T.-B. (2020). Controlled synthesis of a vacancy-defect single-atom catalyst for boosting CO2 electroreduction. Angew. Chem. Int. Ed. 59 (5), 1961–1965. doi:10.1002/anie.201912458
Sun, T., Mitchell, S., Li, J., Lyu, P., Wu, X., Pérez-Ramírez, J., et al. (2021). Design of local atomic environments in single-atom electrocatalysts for renewable energy conversions. Adv. Mat. 33 (5), 2003075. doi:10.1002/adma.202003075
Tan, F., Li, W., Wang, J., Min, C., Li, Z., Zhang, B., et al. (2022). Clarifying the critical roles of iron in boosting oxygen reduction: Single Fe atoms anchored on carbon vacancies as efficient active sites. Appl. Catal. B Environ. 305, 121035. doi:10.1016/j.apcatb.2021.121035
Tang, C., Jiao, Y., Shi, B., Liu, J.-N., Xie, Z., Chen, X., et al. (2020). Coordination tunes selectivity: Two-electron oxygen reduction on high-loading molybdenum single-atom catalysts. Angew. Chem. Int. Ed. 59 (23), 9171–9176. doi:10.1002/anie.202003842
Tong, Y., Guo, H., Liu, D., Yan, X., Su, P., Liang, J., et al. (2020). Vacancy engineering of iron-doped W18O49 nanoreactors for low-barrier electrochemical nitrogen reduction. Angew. Chem. Int. Ed. 59 (19), 7356–7361. doi:10.1002/anie.202002029
Wang, H., Liu, F., Yu, R., Xiao, Z., Zhu, Z., Zhou, L., et al. (2022a). Co-gradient Li-rich cathode relieving the capacity decay in Lithium-ion batteries. Nano Energy 100, 107439. doi:10.1016/j.nanoen.2022.107439
Wang, J., Li, Z., Zhu, Z., Jiang, J., Li, Y., Chen, J., et al. (2022b). Tailoring the interactions of heterostructured Ni4N/Ni3ZnC0.7 for efficient CO2 electroreduction. J. Energy Chem. 75, 1–7doi:10.1016/j.jechem.2022.07.037
Wang, Q., Lei, Y., Wang, D., and Li, Y. (2019). Defect engineering in earth-abundant electrocatalysts for CO2 and N2 reduction. Energy Environ. Sci. 12 (6), 1730–1750. doi:10.1039/C8EE03781G
Wang, T., Tao, L., Zhu, X., Chen, C., Chen, W., Du, S., et al. (2022c). Combined anodic and cathodic hydrogen production from aldehyde oxidation and hydrogen evolution reaction. Nat. Catal. 5 (1), 66–73. doi:10.1038/s41929-021-00721-y
Wang, Y., Wang, D., and Li, Y. (2021). Rational design of single-atom site electrocatalysts: From theoretical understandings to practical applications. Adv. Mat. 33 (34), 2008151. doi:10.1002/adma.202008151
Xiao, K., Lin, R.-T., Wei, J.-X., Li, N., Li, H., Ma, T., et al. (2022). Electrochemical disproportionation strategy to in-situ fill cation vacancies with Ru single atoms. Nano Res. 15 (6), 4980–4985. doi:10.1007/s12274-022-4140-x
Xie, X. H., Xue, Y., Li, L., Chen, S. G., Nie, Y., Ding, W., et al. (2014). Surface Al leached Ti3AlC2 as a substitute for carbon for use as a catalyst support in a harsh corrosive electrochemical system. Nanoscale 6 (19), 11035–11040. doi:10.1039/c4nr02080d
Xu, H., Cheng, D., Cao, D., and Zeng, X. C. (2018). A universal principle for a rational design of single-atom electrocatalysts. Nat. Catal. 1 (5), 339–348. doi:10.1038/s41929-018-0063-z
Yan, D., Li, H., Chen, C., Zou, Y., and Wang, S. (2019). Defect engineering strategies for nitrogen reduction reactions under ambient conditions. Small Methods 3 (6), 1800331. doi:10.1002/smtd.201800331
Yan, D., Li, Y., Huo, J., Chen, R., Dai, L., and Wang, S. (2017). Defect Chemistry of nonprecious-metal electrocatalysts for oxygen reactions. Adv. Mat. 29 (48), 1606459. doi:10.1002/adma.201606459
Yan, D., Xia, C., He, C., Liu, Q., Chen, G., Guo, W., et al. (2022). A substrate-induced fabrication of active free-standing nanocarbon film as air cathode in rechargeable zinc–air batteries. Small 18 (7), 2106606. doi:10.1002/smll.202106606
Yang, X.-Y., Chen, L.-H., Li, Y., Rooke, J. C., Sanchez, C., and Su, B.-L. (2017). Hierarchically porous materials: Synthesis strategies and structure design. Chem. Soc. Rev. 46 (2), 481–558. doi:10.1039/C6CS00829A
Yin, J., Jin, J., Lu, M., Huang, B., Zhang, H., Peng, Y., et al. (2020). Iridium single atoms coupling with oxygen vacancies boosts oxygen evolution reaction in acid media. J. Am. Chem. Soc. 142 (43), 18378–18386. doi:10.1021/jacs.0c05050
Zhang, J., Wang, E., Cui, S., Yang, S., Zou, X., and Gong, Y. (2022). Single-atom Pt anchored on oxygen vacancy of monolayer Ti3C2Tx for superior hydrogen evolution. Nano Lett. 22 (3), 1398–1405. doi:10.1021/acs.nanolett.1c04809
Zhang, L., Fischer, J. M. T. A., Jia, Y., Yan, X., Xu, W., Wang, X., et al. (2018a). Coordination of atomic Co–Pt coupling species at carbon defects as active sites for oxygen reduction reaction. J. Am. Chem. Soc. 140 (34), 10757–10763. doi:10.1021/jacs.8b04647
Zhang, L., Jia, Y., Gao, G., Yan, X., Chen, N., Chen, J., et al. (2018b). Graphene defects trap atomic Ni species for hydrogen and oxygen evolution reactions. Chem 4 (2), 285–297. doi:10.1016/j.chempr.2017.12.005
Zhang, L., Zhao, X., Yuan, Z., Wu, M., and Zhou, H. (2021). Oxygen defect-stabilized heterogeneous single atom catalysts: Preparation, properties and catalytic application. J. Mat. Chem. A Mat. 9 (7), 3855–3879. doi:10.1039/D0TA10541D
Zhang, N., Zou, Y., Tao, L., Chen, W., Zhou, L., Liu, Z., et al. (2019a). Electrochemical oxidation of 5-hydroxymethylfurfural on nickel nitride/carbon nanosheets: Reaction pathway determined by in situ sum frequency generation vibrational spectroscopy. Angew. Chem. Int. Ed. 58 (44), 15895–15903. doi:10.1002/anie.201908722
Zhang, Y., Guo, L., Tao, L., Lu, Y., and Wang, S. (2019b). Defect-based single-atom electrocatalysts. Small Methods 3 (9), 1800406. doi:10.1002/smtd.201800406
Zhao, C.-X., Li, B.-Q., Liu, J.-N., and Zhang, Q. (2021). Intrinsic electrocatalytic activity regulation of M–N–C single-atom catalysts for the oxygen reduction reaction. Angew. Chem. Int. Ed. 60 (9), 4448–4463. doi:10.1002/anie.202003917
Zhao, L., Jiang, J., Xiao, S., Li, Z., Wang, J., Wei, X., et al. (2022). PtZn nanoparticles supported on porous nitrogen-doped carbon nanofibers as highly stable electrocatalysts for oxygen reduction reaction. Nano Mater. Sci. doi:10.1016/j.nanoms.2022.04.001
Zhou, S., Zhao, Y., Shi, R., Wang, Y., Ashok, A., Héraly, F., et al. (2022). Vacancy‐rich MXene‐immobilized Ni single atoms as a high‐performance electrocatalyst for the hydrazine oxidation reaction. Adv. Mat. 34, 2204388. doi:10.1002/adma.202204388
Zhou, Y., Xie, Z., Jiang, J., Wang, J., Song, X., He, Q., et al. (2020). Lattice-confined Ru clusters with high CO tolerance and activity for the hydrogen oxidation reaction. Nat. Catal. 3 (5), 454–462. doi:10.1038/s41929-020-0446-9
Zhu, C., Fu, S., Shi, Q., Du, D., and Lin, Y. (2017). Single-atom electrocatalysts. Angew. Chem. Int. Ed. 56 (45), 13944–13960. doi:10.1002/anie.201703864
Keywords: single-atom catalysts, defect engineering, defective carbon-based support, defective metal-based support, electrocatalytic reaction
Citation: Li W, Chen Z, Jiang X, Jiang J and Zhang Y (2022) Recent advances in the design of single-atom electrocatalysts by defect engineering. Front. Chem. 10:1011597. doi: 10.3389/fchem.2022.1011597
Received: 04 August 2022; Accepted: 24 August 2022;
Published: 15 September 2022.
Edited by:
Dafeng Yan, Huazhong University of Science and Technology, ChinaCopyright © 2022 Li, Chen, Jiang, Jiang and Zhang. This is an open-access article distributed under the terms of the Creative Commons Attribution License (CC BY). The use, distribution or reproduction in other forums is permitted, provided the original author(s) and the copyright owner(s) are credited and that the original publication in this journal is cited, in accordance with accepted academic practice. No use, distribution or reproduction is permitted which does not comply with these terms.
*Correspondence: Jinxia Jiang, MjAyMDM2NEBjcW1wYy5lZHUuY24=; Yagang Zhang, eWd6aGFuZ0B1ZXN0Yy5lZHUuY24=