- 1Key Laboratory of Chinese Internal Medicine of Ministry of Education and Beijing, Dongzhimen Hospital, Beijing University of Chinese Medicine, Beijing, China
- 2State Key Laboratory of Bioactive Substance and Function of Natural Medicines, Institute of Materia Medica, Chinese Academy of Medical Sciences and Peking Union Medical College, Beijing, China
- 3College of Pharmaceutical Sciences, Qinghai University for Nationalities, Xining, China
Secoyanhusamine A (1), a rare rearranged seco-isoquinoline alkaloid derived from ring oxidative cleavage, was isolated from an aqueous extract of Corydalis yanhusuo tubers, together with its biosynthetic precursor dehydrocorybulbine (2). Secoyanhusamine A (1) was the first example of a highly oxidized isoquinoline inner salt resulting in a 5-(2-azanylethyl)-2-carboxylate-4-oxo-4H-pyran ring system. The biosynthetic pathway of 1 was also postulated. Secoyanhusamine A (1) exhibited potent inhibition against acetylcholinesterase (AChE) with an IC50 value of 0.81 ± 0.13 μM. Molecular simulation docking demonstrated that 1 created a strong interaction with the Asp-74 residue of AChE via attractive charge of the quaternary nitrogen.
Introduction
Acetylcholinesterase (AChE) has been regarded as an attractive target in the treatment of Alzheimer’s disease (AD) (Amat-ur-Rasool et al., 2021), the most common neurodegenerative disease in old age that is characterized clinically by progressive memory loss, cognitive dysfunction, language disorders, and personality changes (Dong et al., 2012; Kepp, 2012). The maintenance of acetylcholine (ACh) levels via inhibition of AChE has shown to be an effective therapy in the amelioration of the AD symptoms. Indeed, several drugs such as donepezil, galantamine, and rivastigmine have been approved defined upon this approach (Tumiatti et al., 2008).
Previous studies have shown that natural products are the great resources of candidate drugs for the treatment of AD (Irajia et al., 2020; Tang et al., 2021). One of the interesting resources of potential active compounds, such as isoquinoline alkaloids, is the plant of the Papaveraceae family (Adsersen et al., 2007; Hu et al., 2009; Hung et al., 2010; Zhou et al., 2012; Hostalkova et al., 2019; Zhang et al., 2019). The most studied isoquinoline alkaloid is berberine, which could improve cognitive impairment by promoting autophagic clearance in the mouse model of AD (Wu et al., 2021; Ye et al., 2021). In addition, other isoquinoline alkaloids with AChE inhibitory activity have the potential to act against AD, for instance, palmatine, jatrorrhizine, and coptisine (Ingkaninan et al., 2006; Jung et al., 2009; Hung et al., 2010; Tsai and Lee, 2010; Xiao et al., 2011; Brunhofer et al., 2012; Huang et al., 2012; Mollataghi et al., 2012; Cao et al., 2018; Liu et al., 2019).
The dried tuber of Corydalis yanhusuo W.T. Wang (Papaveraceae), also known as “Yuan-Hu”, is an important traditional Chinese medicine for the treatment of spasms and menstrual and abdominal pain (He et al., 2007; Chinese Pharmacopoeia, 2020). Recent study illustrated that a Chinese medicine prescription Yuan-Hu Zhi Tong (YZT) comprising of C. yanhusuo tubers could alleviate the AD symptoms in the P301S tau and 3XTg-AD mice model, of which the major bioactive constituents are the isoquinoline alkaloids originated from C. yanhusuo (Iyaswamy et al., 2020). In addition, during our preliminary AChE inhibition activity screening, the YH-D fraction of the C. yanhusuo tubers aqueous extract was hit, with an IC50 value of 8.16 ± 1.06 μg/ml. Inspired by this finding, a bioactivity-guided isolation strategy was used to explore promising AChE inhibitors from this bioactive fraction, which resulted in the isolation of a rare rearranged seco-isoquinoline alkaloid with potent AChE inhibition, named secoyanhusamine A (1) (Figure 1), together with a biosynthetic precursor dehydrocorybulbine (2). We report details of the isolation, structure elucidation, possible biogenetic pathway, and AChE inhibitory activity of 1.
Materials and Methods
General Experimental Procedures
The UV spectrum was acquired on a Cary 300 spectrometer. The IR spectrum was obtained on a Nicolet Impact 400 FT-IR spectrophotometer. 1D- and 2D-NMR spectra were recorded using Bruker 600 MHz spectrometers (600 MHz for 1H and 150 MHz for 13C), and the chemical shifts were reported as δ values using internal standard TMS (measured in MeOH-d4). HR-ESIMS data were obtained on Agilent 1100 Series LC-MSD-Trap-SL and Agilent 6520 Accurate-Mass Q-TOFL CMS spectrometers (Agilent Technologies, Ltd., Santa Clara, CA, United States). Column chromatography (CC) was performed with a macroporous resin (HP20, Mitsubishi Group, Japan), Sephadex LH-20 (Amersham Biosciences, Sweden), and silica gel (200–300 mesh, Qingdao Marine Chemical Inc., People’s Republic of China). Analytical HPLC was performed with an Agilent 1260Ⅱ using a Titank column (Guangzhou FLM Scientific Instrument Co., Ltd.) packed with C18 (250 × 4.6 mm, 5 μm). HPLC separation was performed on a system consisting of a Waters 600 controller, a Waters 600 pump, and a Waters 2487 Dual λ absorbance detector (Waters Corporation, Milford, MA, United States), with a Shiseido MGII ODS C18 column (250 × 10 mm or 250 × 20 mm, 5 μm). TLC was conducted on precoated silica gel GF254 plates. Spots were visualized under UV light (254 or 356 nm) or by spraying with 10% H2SO4 in 95% EtOH followed by heating or with a Dragendorff’s reagent. All chemicals were obtained from commercially available sources and were used without further purification.
Plant Material
See ref. Wang et al., 2020.
Extraction and Isolation
For extraction and preliminary fractionation of the extract, see ref. Wang et al., 2020. The 50% EtOH fraction (YH-D) was evaporated under reduced pressure to yield 270 g of a residue, which was subjected to column chromatography (CC) on MCI, with H2O (40 L), 30% EtOH (100 L), 60% EtOH (100 L), and 95% EtOH (100 L) as successive eluents to yield four major fractions. The 30% EtOH fraction was chromatographed by MPLC over a reversed-phase C18 silica gel eluting with MeOH–H2O (0–95%), to afford five fractions (A−E). Fraction A (19.54 g) was subsequently fractionated by Sephadex LH-20 CC eluting with 10% MeOH–H2O to furnish six subfractions (A1−A6). Subfraction A4 was separated further by ODS C18 MPLC eluting with MeOH–H2O (0–50%), to afford seven fractions (A4-1−A4-7). Subfraction A4-6 was separated further by semipreparative HPLC [RP18, 5 μm, 250 × 10 mm, 254 nm, CH3CN−H2O−TFA (25:75:0.1)] to give 1 (tR = 12.7 min, 2.0 mg) and 2 (tR = 17.5 min, 5.0 mg). The 95% EtOH fraction (YH-E) was subjected to a silica gel CC, eluting with CH2Cl2−MeOH (200:1 → 10:1), to afford nine fractions (A−I). Fraction I was fractionated by Sephadex LH-20 CC eluting with MeOH to yield eight subfractions (I1−I8). Subfraction I7 was separated further by preparative HPLC to give 2 [RP18, 5 μm, 250 × 20 mm, 280 nm, MeOH−H2O−TFA (52:48:0.1), tR = 21.3 min, 250 mg].
Compound Characterization
Secoyanhusamine A (1): a yellow, amorphous powder; UV (MeOH) λmax (log ε) 204 (3.85), 256 (3.75), 293 (2.85), and 393 (2.65) nm; IR νmax 3349, 3098, 2908, 1681, 1636, 1520, 1418, 1392, 1350, 1290, 1204, 1173, 1129, 1102, 1058, 1033, 932, 827, 803, 721, 667, and 609 cm−1; 1H NMR (MeOH-d4, 600 MHz) and 13C NMR (MeOH-d4, 150 MHz) spectral data, see Table 1; HRESIMS m/z 370.1299 [M + H]+ (calcd for C20H20NO6, 370.1285) and 739.2518 [2M + H]+ (calcd. for C40H39N2O12, 739.2498).
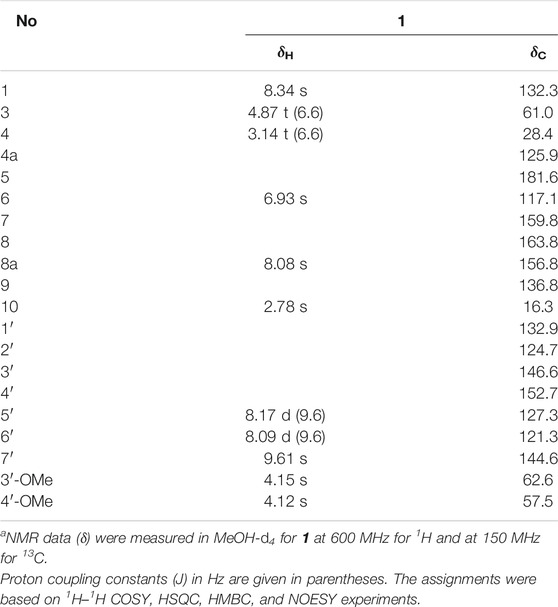
TABLE 1. NMR spectroscopic data for 1a.
Dehydrocorybulbine (2): a yellow, amorphous powder; 1H NMR (DMSO-d6, 400 MHz) δ: 10.04 (1H, brs, 6-OH), 9.86 (1H, s, H-7′), 8.20 (1H, d, J = 9.6 Hz, H-5′), 8.16 (1H, d, J = 9.6 Hz, H-6′), 7.36 (1H, s, H-8), 6.91 (1H, s, H-5), 4.81 (2H, t, J = 5.6 Hz, H2-3), 4.09 (3H, s, 4′-OMe), 4.08 (3H, s, 3′-OMe), 3.86 (3H, s, 7-OMe), 3.05 (2H, t, J = 5.6 Hz, H2-4), and 2.97 (3H, s, Me-10); 13C NMR (DMSO-d6, 150 MHz) δ: 150.0 (C-4′), 149.1 (C-6), 146.3 (C-7), 143.9 (C-7′), 143.7 (C-3′), 136.4 (C-1), 133.2 (C-1′), 131.9 (C-4a), 129.3 (C-9), 125.9 (C-5′), 121.2 (C-6′), 120.6 (C-2′), 117.8 (C-8a), 115.1 (C-8), 114.6 (C-5), 62.0 (3′-OMe), 57.0 (4′-OMe), 56.8 (C-3), 56.2 (7-OMe), 26.6 (C-4), and 17.7 (Me-10) (+)-ESIMS m/z 352 [M]+.
Bioassay for Anti-Cholinesterase Activity
Inhibition of AChE was assessed by a modified version of the colorimetric method of Ellman (Oh et al., 2004). A mixture of 196 μl phosphate buffer (PBS) and acetylcholinesterase (final concentration 0.01 μg/ml) and 2 µl inhibitor (YH-D, compound 1, and positive control donepezil) was added to the 96-well plate, and then the plate was incubated at 4°C for 20 min; 2 µl DTNB and ATCI (final concentration of 116 μM) was added, and the solution was incubated for 20 min at 37°C on a shaker. The optical density was measured at 405 nm immediately, and the percentage inhibition was calculated. Donepezil was used as a positive control.
Results and Discussion
The bioactive fraction YH-D (270 g) was separated systematically by column chromatography, such as MCI and Sephadex LH-20 as well as preparative HPLC, to yield secoyanhusamine A (1).
Secoyanhusamine A (1) was obtained as a yellow amorphous powder. The HRESIMS of 1 gave the positive ion peak at m/z 370.1299 [M + H]+ (calcd. for C20H20NO6, 370.1285) and 739.2518 [2M + H]+ (calcd. for C40H39N2O12, 739.2498), corresponding to an elemental formula of C20H19NO6, which indicated 12 degrees of unsaturation. Its IR spectrum revealed the presence of an inner salt formed via carboxylate (3600-2800 and 1,636 cm−1), carbonyl (1,681 cm−1), and aromatic (1,520 and 1,418 cm−1) functionalities. The 1H NMR and 1H–1H COSY data (Figure 2) of 1 in MeOH-d4 provided the signals of two ortho-coupled protons at δH 8.17 (1H, d, J = 9.6 Hz, H-5′) and 8.09 (1H, d, J = 9.6 Hz, H-6′) and two nitrogen-bearing aromatic protons at δH 9.61 (1H, s, H-7′) and 8.34 (1H, s, H-1), which indicated a 4,7,8-trisubstituted isoquinoline moiety. Furthermore, two aromatic singlet protons at δH 8.08 (1H, s, H-8a) and 6.93 (1H, s, H-6), two adjacent coupling methylenes at δH 4.87 (2H, t, J = 6.6 Hz, H2-3) and 3.14 (2H, t, J = 6.6 Hz, H2-4), two methoxy groups at δH 4.15 (3H, s, OMe-3′) and 4.12 (3H, s, OMe-4′), and a methyl singlet at δH 2.78 (3H, s, H3-10) could be recognized in the 1H NMR spectrum of 1. The 13C NMR data showed 20 carbon resonances corresponding to thirteen aromatic and/or olefinic carbons, two methylenes [δC 61.0 (C-3) and 28.4 (C-4)], two methoxy groups [(δC 62.6 (OMe-3′) and 57.5 (OMe-4′)], and a methyl group (δC 16.3, C-10) as well as two carbonyl carbons [(δC 181.6 (C-5) and 163.8 (C-8)] (Table 1). Since the above functionalities meet 11 degrees of unsaturation, compound 1 was considered as a highly oxidized isoquinoline alkaloid with another ring system.
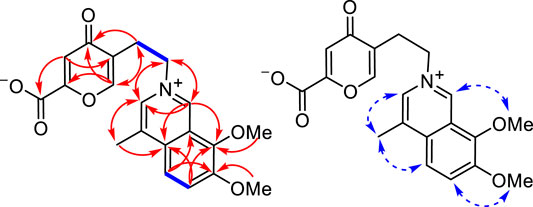
FIGURE 2. Main 1H–1H COSY (left, blue lines), three-bond HMBC (left, red arrows, from 1H to 13C), and NOESY (right, blue arrows) correlations of 1.
The analysis of 1H–1H COSY, HSQC, HMBC, and NOESY NMR data confirmed the above assignments and constructed the structure of 1. The proton resonances and corresponding proton-bearing carbon resonances in the NMR spectra were assigned by the HSQC experiment. The presence of the 4-methyl-7,8-dimethoxy-isoquinoline moiety of 1 was confirmed by the HMBC correlations from H-7′ to C-1′ and C-3′, from H-5′ to C-1′ and C-3′, from H-6′ to C-2′ and C-4′, from H3-10 to C-1′ and C-1, and from the two methoxy protons to C-3′ and C-4′ as well as the NOESY correlations of H3-10/H-1, OMe-4′/H-5′, and OMe-3′/H-7′ (Figure 2). Additionally, the 1H–1H COSY correlations of H2-3/H2-4 coupled with the HMBC cross-peaks from H2-4 to C-5 and C-8a, from H-6 to C-4a and C-8, and from H-8a to C-5 and C-7 served to establish a 5-(2-azanylethyl)-2-carboxylate-4-oxo-4H-pyran ring system (Figure 2). Finally, the observed HMBC cross-peaks from both H-1 and H-7′ to C-3 and from H2-3 to C-1 and C-7′ verified that the 5-(2-azanylethyl)-2-carboxylate-4-oxo-4H-pyran ring was connected to the 4-methyl-7,8-dimethoxy-isoquinoline moiety via the quaternary nitrogen atom (Figure 2). Therefore, the structure of 1 was determined as shown and named secoyanhusamine A. Taking into account the elemental composition and the presence of both alkali amine and acidic carboxylic units, 1 was considered to be an inner salt under a neutral hydrophilic condition. In a consistent manner, no TFA signals were observed in the 13C NMR and 19F NMR spectra of 1 (Supplementary Material, Figures 2, 3) when the purification of 1 was performed by semipreparative HPLC with TFA.
Isoquinoline alkaloids are the major constituents of C. yanhusuo (Hu et al., 2009; Zhou et al., 2012; Lv et al., 2012; Tong et al., 2013; Yang et al., 2014; Wang et al., 2020; Xiao et al., 2020). The 5-(2-azanylethyl)-2-carboxylate-4-oxo-4H-pyran ring moiety was not common in the isoquinoline alkaloids family. However, the remaining 4-methyl-7,8-dimethoxy-isoquinoline moiety of 1 was similar to that of isoquinoline alkaloids isolated from this genus plants (Lv et al., 2012; Tong et al., 2013; Yang et al., 2014; Liu et al., 2016; Wang et al., 2020; Xiao et al., 2020). Thus, 1 is probably generated from the biosynthetic precursor dehydrocorybulbine (2) (250 mg) (Lv et al., 2012), which has been also isolated from this plant in large quantities (Scheme 1). 2 undergoes an electron migration rearrangement followed by oxidative cleavage of ring B to obtain III, which continued to involve O2 and NADPH then cytochrome P450- or FAD-dependent enzymatic Baeyer–Villiger oxidation to form a peroxy-enzyme-activated complex IV (Liao et al., 2005; Ji et al., 2020; Fang et al., 2021), leading to the insertion of oxygen between C-8 and C-8a (V). The intermediate V would then undergo rearrangement to produce 1.
The initial biological screening of the YH-D fraction encouraged further AChE inhibitory evaluation of secoyanhusamine A (1). As a result, secoyanhusamine A (1) showed potent inhibition with an IC50 value of 0.81 ± 0.13 μM, compared to the positive control (donepezil, IC50 = 0.15 ± 0.01 μM). Furthermore, molecular docking simulation was performed on the basis of the previously reported crystal structure of AChE (Cheung et al., 2012). As shown in Figure 3, secoyanhusamine A (1) could be docked perfectively into the catalytic cavity of AChE, while the N-atom and the pyrone ring could strongly interact with the Asp-74 residue of AChE via attractive charge and with the Tyr-341 residue of AChE via Pi–Pi T-shaped, respectively.
Conclusion
In summary, secoyanhusamine A (1), a rare rearranged seco-isoquinoline alkaloid derived from ring oxidative cleavage, was isolated from an aqueous extract of Corydalis yanhusuo tubers and showed significant inhibitory activity against acetylcholinesterase (AChE). The structures of 1 were determined via extensive NMR spectroscopic analysis. Our study provides a new structural architecture of the natural product that can be used in follow-up studies relevant to the development of anti-Alzheimer’s agents.
Data Availability Statement
The original contributions presented in the study are included in the article/Supplementary Material, further inquiries can be directed to the corresponding authors.
Author Contributions
LW, PL, and SL contributed to the conception and design of this research. LW carried out the study and collected important background information. HX and YW provided assistance for compound characterization and data analysis. YW carried out the 1D- and 2D-NMR spectra data. PL and SL performed manuscript revision. All authors contributed to manuscript revision and read and approved the submitted version.
Funding
This research was financially supported by the Beijing Natural Science Foundation (Grant No. JQ18026), the National Natural Science Foundation of China (NNSFC; Grant Nos. 82073978, 81760783, and 81522050), and the Innovation team of Medicinal Material Resource Protection and High Value Utilization in Qinghai Province (Grant No. 2021XJPI02).
Conflict of Interest
The authors declare that the research was conducted in the absence of any commercial or financial relationships that could be construed as a potential conflict of interest.
Publisher’s Note
All claims expressed in this article are solely those of the authors and do not necessarily represent those of their affiliated organizations, or those of the publisher, the editors, and the reviewers. Any product that may be evaluated in this article, or claim that may be made by its manufacturer, is not guaranteed or endorsed by the publisher.
Supplementary Material
The Supplementary Material for this article can be found online at: https://www.frontiersin.org/articles/10.3389/fchem.2021.831173/full#supplementary-material
References
Adsersen, A., Kjølbye, A., Dall, O., and Jäger, A. K. (2007). Acetylcholinesterase and Butyrylcholinesterase Inhibitory Compounds from Corydalis Cava Schweigg. & Kort. J. Ethnopharmacology 113, 179–182. doi:10.1016/j.jep.2007.05.006
Amat-ur-Rasool, H., Ahmed, M., Hasnain, S., and Carter, W. G. (2021). Anti-Cholinesterase Combination Drug Therapy as a Potential Treatment for Alzheimer's Disease. Brain Sci. 11, 184. doi:10.3390/brainsci11020184
Brunhofer, G., Fallarero, A., Karlsson, D., Batista-Gonzalez, A., Shinde, P., Gopi Mohan, C., et al. (2012). Exploration of Natural Compounds as Sources of New Bifunctional Scaffolds Targeting Cholinesterases and Beta Amyloid Aggregation: The Case of Chelerythrine. Bioorg. Med. Chem. 20, 6669–6679. doi:10.1016/j.bmc.2012.09.040
Cao, T. Q., Ngo, Q.-M. T., Seong, S. H., Youn, U. J., Kim, J. A., Kim, J., et al. (2018). Cholinesterase Inhibitory Alkaloids from the Rhizomes of Coptis Chinensis. Bioorg. Chem. 77, 625–632. doi:10.1016/j.bioorg.2018.01.038
Cheung, J., Rudolph, M. J., Burshteyn, F., Cassidy, M. S., Gary, E. N., Love, J., et al. (2012). Structures of Human Acetylcholinesterase in Complex with Pharmacologically Important Ligands. J. Med. Chem. 55, 10282–10286. doi:10.1021/jm300871x
Chinese Pharmacopoeia (2020). Pharmacopoeia of the People's Republic of China. Beijing: China Medical Science and Technology Press, p145–146.
Dong, S., Duan, Y., Hu, Y., and Zhao, Z. (2012). Advances in the Pathogenesis of Alzheimer's Disease: a Re-evaluation of Amyloid cascade Hypothesis. Transl. Neurodegener. 1 (1), 18. doi:10.1186/2047-9158-1-18
Fang, Q., Wu, L., Urwald, C., Mugat, M., Wang, S., Kyeremeh, K., et al. (2021). Genomic Scanning Enabling Discovery of a New Antibacterial Bicyclic Carbamate-Containing Alkaloid. Synth. Syst. Biotechnol. 6, 12–19. doi:10.1016/j.synbio.2021.01.002
He, K., Gao, J. L., and Zhao, G. S. (2007). Advances in Studies on Chemistry, Pharmacology, and Quality Control of. Corydalis Yanhusuo. Chin. Tradit. Herbal. Drugs 12, 1909–1912. doi:10.3321/j.issn:0253-2670.2007.12.050
Hostalkova, A., Marikova, J., Korabecny, J., Hulcova, D., Kunes, J., Novakova, L., et al. (2019). Isoquinoline Alkaloids from Berberis Vulgaris as Potential Lead Compounds for the Treatment of Alzheimer's Disease. J. Nat. Prod. 82, 239–248. doi:10.1021/acs.jnatprod.8b00592
Hu, T. T., Zhang, X., Ma, S. Z., and Yao, X. S. (2009). A New Protoberberine Alkaloid from Corydalis Yanhusuo W. T. Wang. Chin. Chem. Lett. 20, 955–957. doi:10.1016/j.cclet.2009.03.045
Huang, Q.-Q., Bi, J.-L., Sun, Q.-Y., Yang, F.-M., Wang, Y.-H., Tang, G.-H., et al. (2012). Bioactive Isoquinoline Alkaloids from Corydalis Saxicola. Planta Med. 78, 65–70. doi:10.1055/s-0031-1280126
Hung, T., Dang, N., Kim, J., Jang, H.-S., Ryoo, S.-W., Lee, J., et al. (2010). Alkaloids from Roots of Stephania Rotunda and Their Cholinesterase Inhibitory Activity. Planta Med. 76, 1762–1764. doi:10.1055/s-0030-1249814
Ingkaninan, K., Phengpa, P., Yuenyongsawad, S., and Khorana, N. (2010). Acetylcholinesterase Inhibitors from Stephania Venosa Tuber. J. Pharm. Pharmacol. 58, 695–700. doi:10.1211/jpp.58.5.0015
Iraji, A., Khoshneviszadeh, M., Firuzi, O., Khoshneviszadeh, M., and Edraki, N. (2020). Novel Small Molecule Therapeutic Agents for Alzheimer Disease: Focusing on BACE1 and Multi-Target Directed Ligands. Bioorg. Chem. 97, 103649. doi:10.1016/j.bioorg.2020.103649
Iyaswamy, A., Krishnamoorthi, S. K., Liu, Y. W., Song, J. X., Kammala, A. K., Sreenivasmurthy, S. G., et al. (2020). Yuan-Hu Zhi Tong Prescription Mitigates Tau Pathology and Alleviates Memory Deficiency in the Preclinical Models of Alzheimer's Disease. Front. Pharmacol. 11, 584770. doi:10.3389/fphar.2020.584770
Ji, X., Tu, J., Song, Y., Zhang, C., Wang, L., Li, Q., et al. (2020). A Luciferase-like Monooxygenase and Flavin Reductase Pair AbmE2/AbmZ Catalyzes Baeyer-Villiger Oxidation in Neoabyssomicin Biosynthesis. ACS Catal. 10, 2591–2595. doi:10.1021/acscatal.9b05488
Jung, H. A., Min, B.-S., Yokozawa, T., Lee, J.-H., Kim, Y. S., and Choi, J. S. (2009). Anti-Alzheimer and Antioxidant Activities of Coptidis Rhizoma Alkaloids. Biol. Pharm. Bull. 32, 1433–1438. doi:10.1248/bpb.32.1433
Kepp, K. P. (2012). Bioinorganic Chemistry of Alzheimer's Disease. Chem. Rev. 112, 5193–5239. doi:10.1021/cr300009x
Liao, S.-G., Zhan, Z.-J., Yang, S.-P., and Yue, J.-M. (2005). Lathyranoic Acid A: First Secolathyrane Diterpenoid in Nature from Euphorbia Lathyris. Org. Lett. 7 (7), 1379–1382. doi:10.1021/ol050206a
Liu, L., Wu, L. J., and Yang, C. J. (2016). Research Progress on Biologically Active Isoquinoline Alkaloids with Chemical Structures and Drug-like Properties of Genus Corydalis. Pract. Pharm. Clin. Remed. 19 (3), 371–380.
Liu, M., Liu, Q., Chen, M., Huang, X., and Chen, X. (2019). Large‐scale Separation of Acetylcholinesterase Inhibitors from Zanthoxylum Nitidum by pH‐zone‐refining Counter‐current Chromatography Target‐guided by Ultrafiltration High‐performance Liquid Chromatography with Ultraviolet and Mass Spectrometry Screening. J. Sep. Sci. 42, 1194–1201. doi:10.1002/jssc.201801238
Lu, Z., Sun, W., Duan, X., Yang, Z., Liu, Y., and Tu, P. (2012). Chemical Constituents from Corydalis Yanhusuo. Chin. J. Chin. Mater. Med. 37, 235–237. doi:10.4268/cjcmm20120223
Mollataghi, A., Coudiere, E., Hadi, A. H. A., Mukhtar, M. R., Awang, K., Litaudon, M., et al. (2012). Anti-acetylcholinesterase, Anti-α-glucosidase, Anti-leishmanial and Anti-fungal Activities of Chemical Constituents of Beilschmiedia Species. Fitoterapia 83, 298–302. doi:10.1016/j.fitote.2011.11.009
Oh, M. H., Houghton, P. J., Whang, W. K., and Cho, J. H. (2004). Screening of Korean Herbal Medicines Used to Improve Cognitive Function for Anti-cholinesterase Activity. Phytomedicine 11, 544–548. doi:10.1016/j.phymed.2004.03.001
Tang, X.-H., Luo, R.-C., Ye, M.-S., Tang, H.-Y., Ma, Y.-L., Chen, Y.-N., et al. (2021). Harpertrioate A, an A,B,D-seco-Limonoid with Promising Biological Activity against Alzheimer's Disease from Twigs of Harrisonia Perforata (Blanco) Merr. Org. Lett. 23, 262–267. doi:10.1021/acs.orglett.0c03460
Tong, S., Yu, Q., Li, X.-N., and Yan, J. (2013). Preparative Separation of Tertiary Alkaloids from Corydalis Yanhusuo W. T. Wang by Ph-Zone-Refining Counter-current Chromatography. J. Liquid Chromatogr. Relat. Tech. 36, 229–238. doi:10.1080/10826076.2011.649875
Tsai, S.-F., and Lee, S.-S. (2010). Characterization of Acetylcholinesterase Inhibitory Constituents from Annona Glabra Assisted by HPLC Microfractionation. J. Nat. Prod. 73, 1632–1635. doi:10.1021/np100247r
Tumiatti, V., Bolognesi, M. L., Minarini, A., Rosini, M., Milelli, A., Matera, R., et al. (2008). Progress in Acetylcholinesterase Inhibitors for Alzheimer's Disease: an Update. Expert Opin. Ther. Patents 18 (4), 387–401. doi:10.1517/13543776.18.4.387
Wang, L.-Y., Qiu, B.-L., Xia, H., Xia, G.-Y., Xiao, B.-B., Zhang, J.-F., et al. (2020). Yanhusanines A-F, Isoquinoline-Derived Alkaloid Enantiomers from Corydalis Yanhusuo and Their Biological Activity. J. Nat. Prod. 83, 489–496. doi:10.1021/acs.jnatprod.9b01155
Wu, Y., Chen, Q., Wen, B., Wu, N., He, B., and Chen, J. (2021). Berberine Reduces Aβ42 Deposition and Tau Hyperphosphorylation via Ameliorating Endoplasmic Reticulum Stress. Front. Pharmacol. 12, 640758. doi:10.3389/fphar.2021.640758
Xiao, B.-B., Xia, G.-Y., Wang, L.-Y., Qiu, B.-L., Xia, H., Zhong, W.-C., et al. (2020). (±)-Bicoryanhunine A, Dimeric Benzylisoquinoline Alkaloid Atropo-Enantiomers from Corydalis Yanhusuo. Tetrahedron Lett. 61, 151890–151892. doi:10.1016/j.tetlet.2020.151890
Xiao, H.-T., Peng, J., Liang, Y., Yang, J., Bai, X., Hao, X.-Y., et al. (2011). Acetylcholinesterase Inhibitors from Corydalis Yanhusuo. Nat. Product. Res. 25, 1418–1422. doi:10.1080/14786410802496911
Yang, X. B., Yang, X. W., and Liu, J. X. (2014). Study on Material Base of Corydalis Rhizoma. Chin. J. Chin. Mater. Med. 39, 20–27. doi:10.4268/cjcmm20140105
Ye, C., Liang, Y., Chen, Y., Xiong, Y., She, Y., Zhong, X., et al. (2021). Berberine Improves Cognitive Impairment by Simultaneously Impacting Cerebral Blood Flow and β-Amyloid Accumulation in an APP/tau/PS1 Mouse Model of Alzheimer's Disease. Cells 10, 1161. doi:10.3390/cells10051161
Zhang, J., Zhang, C., Xu, F.-C., QueshengZhang, Q. Y., Zhang, Q.-Y., Tu, P.-F., et al. (2019). Cholinesterase Inhibitory Isoquinoline Alkaloids from Corydalis Mucronifera. Phytochemistry 159, 199–207. doi:10.1016/j.phytochem.2018.11.019
Keywords: Corydalis yanhusuo, seco-isoquinoline alkaloid, secoyanhusamine A, acetylcholinesterase inhibitor, Alzheimer’s disease
Citation: Wang L, Xia H, Wu Y, Wang Y, Lin P and Lin S (2022) Secoyanhusamine A, an Oxidatively Ring-Opened Isoquinoline Inner Salt From Corydalis yanhusuo. Front. Chem. 9:831173. doi: 10.3389/fchem.2021.831173
Received: 08 December 2021; Accepted: 24 December 2021;
Published: 01 February 2022.
Edited by:
Xiaoxiao Huang, Shenyang Pharmaceutical University, ChinaCopyright © 2022 Wang, Xia, Wu, Wang, Lin and Lin. This is an open-access article distributed under the terms of the Creative Commons Attribution License (CC BY). The use, distribution or reproduction in other forums is permitted, provided the original author(s) and the copyright owner(s) are credited and that the original publication in this journal is cited, in accordance with accepted academic practice. No use, distribution or reproduction is permitted which does not comply with these terms.
*Correspondence: Pengcheng Lin, cWhscGNAMTI2LmNvbQ==; Sheng Lin, bHN6bm5AYnVjbS5lZHUuY24=