- School of Chemistry and Bioengineering, Hunan University of Science and Engineering, Yongzhou, China
Tea is a popular beverage all around the world. Tea composition, quality monitoring, and tea identification have all been the subject of extensive research due to concerns about the nutritional value and safety of tea intake. In the last 2 decades, research into tea employing electrochemical biosensing technologies has received a lot of interest. Despite the fact that electrochemical biosensing is not yet the most widely utilized approach for tea analysis, it has emerged as a promising technology due to its high sensitivity, speed, and low cost. Through bibliometric analysis, we give a systematic survey of the literature on electrochemical analysis of tea from 1994 to 2021 in this study. Electrochemical analysis in the study of tea can be split into three distinct stages, according to the bibliometric analysis. After chromatographic separation of materials, electrochemical techniques were initially used only as a detection tool. Many key components of tea, including as tea polyphenols, gallic acid, caffeic acid, and others, have electrochemical activity, and their electrochemical behavior is being investigated. High-performance electrochemical sensors have steadily become a hot research issue as materials science, particularly nanomaterials, and has progressed. This review not only highlights these processes, but also analyzes and contrasts the relevant literature. This evaluation also provides future views in this area based on the bibliometric findings.
Introduction
Tea is one of the most popular natural health drinks and is deeply ingrained in people’s lives. Tea trees are grown in around 30 nations throughout the world, yielding roughly 2.5 million tons of tea every year. The introduction of tea trees, on the other hand, can be easily influenced by soil and climate conditions (Feng et al., 2010; Wambu et al., 2017; Kottawa-Arachchi et al., 2019; Beringer et al., 2020). Furthermore, the nutritional composition and taste of tea fluctuate significantly due to variances in processing processes. Tea leaves are classified in a variety of ways based on various factors (Liu et al., 2015). Green tea, yellow tea, white tea, oolong tea, black tea, and dark tea are the most often used criterion for categorizing tea based on the degree of fermentation (de Carvalho Couto et al., 2021). Green tea is produced without the use of fermentation. Yellow tea is fermented to a degree of 10–20 percent. White tea is fermented to a percentage of 10–30%. Oolong tea is fermented to a degree of 20–60%. Black tea is fermented to a degree of 80–90 percent. Dark tea is a post-fermented tea, meaning it has undergone the most fermentation. The material components in tea leaves are changed into diverse forms as a result of varying degrees of fermentation (Zheng et al., 2016; Marx et al., 2017; Seth et al., 2019). Unfermented green tea, for example, preserves more of the natural components of the fresh leaves. These nutrients offer unique therapeutic properties in the human body, including anti-aging, anti-cancer, anti-inflammatory, and antiseptic properties (Kochman et al., 2021). Oolong tea processing, on the other hand, employs alternating mechanical force and stacking (Ng et al., 2018). The external force damages the cellular tissue of the leaf edge, while the polyphenols are oxidized and undergo other chemical changes as a result of the mechanical activity.
Tea leaves contain about 700 chemicals that have been extracted and identified. The secondary metabolic components of tea, such as tea polyphenols, amino acids, alkaloids, aromatic chemicals, pigment molecules, and so on, are primarily responsible for its distinctive flavor (Chapagain and Hoekstra, 2007; McCants, 2008; Tanui et al., 2012; Mzembe et al., 2016; Wang et al., 2020; Ying et al., 2020; Zhang et al., 2020; Zhou et al., 2020). They have a close association with specific pharmacological effects and impact the quality and flavor of tea (Koch et al., 2018; Wei et al., 2018). Based on the characteristics of the components, tea component analysis may be separated into two primary types: flavor component and quality component (Xu et al., 2019a). The flavor component is linked to the color, aroma, and taste of tea, and its detection focuses on determining the flavor qualities of tea quality (Xu et al., 2019b). The detection of the quality component is primarily for quality control and inspection purposes (Wang et al., 2019). The most often utilized techniques are colorimetric and spectroscopic approaches (Zhi et al., 2017). However, in the recent decade, the rapid development of electrochemical sensing techniques has enticed many scientists to experiment with electrochemical biosensing approaches to assess tea components. High sensitivity, wide linear response, outstanding stability, and reproducibility are all advantages of electrochemical sensors. Furthermore, the low cost of electrochemical measurements is a significant advantage. Electrochemical sensors consist of an electrochemical cell with at least two electrodes to form a closed electrical circuit and a transducer where charge transport (always electronic) takes place, whereas charge transport in the analyte sample can be electronic, ionic, or mixed (Power et al., 2018; Karimi-Maleh et al., 2021a). Electroanalytical methods are a class of analytical chemistry techniques that measure the potential (volts) and/or current (amperes) in an electrochemical cell containing the analyte to investigate it (Naveen et al., 2017; Karimi-Maleh et al., 2021b; Karimi-Maleh et al., 2021c; Karimi-Maleh et al., 2021d; Mani et al., 2021; Karimi-Maleh et al., 2022). Two important electrochemical methods used in the parameter evaluation of tea products are voltammetry, which measures current as potential varies, and electronic sensing, which includes the electronic nose (E-nose), electronic tongue (E-tongue), and electronic eye (E-eye). ASV, CV, SWV, and staircase voltammetry, in particular, use various types of electrodes, such as inert carbon electrodes, glassy carbon electrodes, and paraffin-impregnated graphite electrodes, to help determine the quality of tea products. We searched the WOS core database using the keywords electrochemistry and tea and discovered a total of 865 papers dedicated to the study of tea using electrochemistry from 1994 to 2021, as shown in Figure 1. It’s worth noting that similar research has gotten a lot of attention in the recent decade.
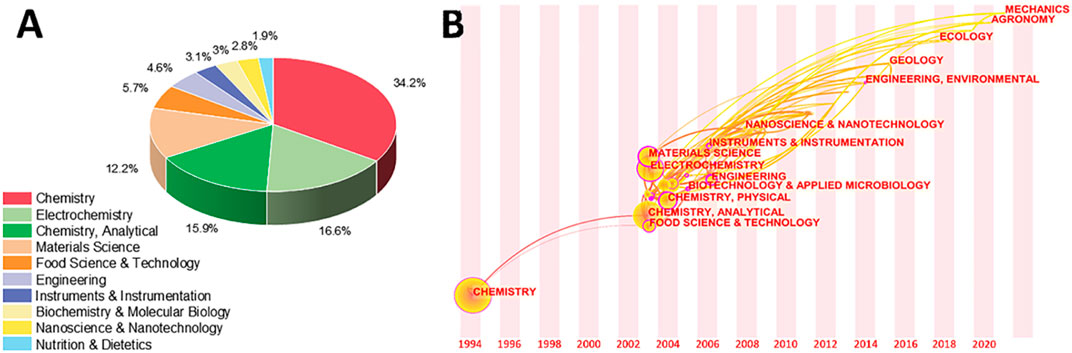
FIGURE 2. (A) The amount of contribution of electrochemical sensing techniques for tea research in different categories. (B) Variation of category with year in the literature of electrochemical sensing techniques for tea analysis.
We conducted a bibliometric analysis of these 865 studies to see if electrochemical biosensing may replace existing analytical approaches in tea quality control. For the bibliometric analysis and visual presentation, CiteSpace was employed (Chen, 2004; Chen, 2006; Chen and Song, 2019). The analysis report compares electrochemical techniques for tea analysis in terms of procedural and thematic changes. We also kept an eye on the cutting-edge of electrochemical sensing technologies in the field of tea analysis. We included both the analysis and data comparison of specific publications in traditional review writing, in addition to the overall pulse of bibliometric analysis. The use of a mix of electrochemical biosensing techniques and nanomaterial technologies was highlighted in particular. The most representative of these pieces was also thoroughly examined.
Literature Information Analysis
Changes in the Literature Category of Electrochemical Sensing Technology for Tea Analysis
Changes in electrical signals are the basis for signal production in electrochemical biosensing systems. Changes in current and resistance can be used as signals. Electrochemical oxidation-reduction is present in the bulk of these signal alterations. Under a result, the vast majority of tea research using electrochemical sensing technologies is classified as chemistry, electrochemistry, or analytical chemistry (Figure 2A). It’s important to note that each published paper does not fall into a single category. As a result, understanding other categories can assist in determining the target themes and areas to cross. What was unexpected, as indicated in Figure 2A, was the importance of materials science in this area. This is because the production of very sensitive electrochemical biosensors frequently necessitates the use of novel materials, according to a later study for the literature (Guth et al., 2009; Wang et al., 2015a; Alqarni et al., 2020; Ranga et al., 2021). This also helps to explain why instruments and instrumentation account for 4.6 percent of all category statistics. Similarly, nanotechnology and nanotechnologies accounted for 2.8 percent of the total. Traditional fields of tea research include food science and technology, biochemistry and molecular biology, and nutrition and dietetics. The development of electrochemical sensing technology has clearly been employed for tea research in various areas, as evidenced by this pie chart.
Figure 2B depicts a history of when these categories first appeared on this subject and how they interacted. The most significant categories arrived between 2003 and 2008, with the exception of chemistry, which debuted in 1994. This shows that the electrochemical sensing analysis approaches for tea research surge began in 2003, which is consistent with the results in Figure 1. The illustration also highlights the current multidisciplinary interaction of electrochemical analytical tools for tea research with ecology, geology, and agronomy. This indicates that the technology discussed in this article is well-established and has application potential, allowing it to be expanded into other areas for research. Abhradip and Chandan (Pal and Das, 2020a), for example, used electrochemical sensors to assess the solid tea waste extract’s ability to inhibit boiler quality steel under acidic circumstances.
Author Country Distribution and Cooperation
Despite the fact that the tea tree is a widely produced plant, tea consumption in various countries has been influenced by cultural factors. As a result, in the scientific study of tea, there is relative independence between countries. Although many nations have actively investigated the development of electrochemical sensing technology in tea, as illustrated in Figure 3A, international cooperation is rare. Only a small percentage of articles have authors who are from different nations. The United States and the United Kingdom play a significant role in international cooperation on this issue. China and India, on the other hand, the two countries with the highest number of publications on this subject, have focused their research mostly on their own countries. Despite the fact that certain countries are geographically close, such as France and Germany, they continue to perform independent studies on this topic. Surprisingly, despite tea’s reputation as an Eastern beverage, the most influential publications in early studies were from the United States. The radical chemistry of epigallocatechin gallate and epigallocatechin was reported by Hagerman et al. (Hagerman et al., 2003). To validate the generation of hydroxyl radicals, the electrochemical redox potentials of both molecules were measured. For phenolic component identification, Luo et al. (Luo et al., 2003) employed liquid chromatography with coulometric electrochemical detection. In this investigation, tea blends were employed as a true sample. Figure 3B depicts the timelines of the various countries participated in this research. Many countries got actively involved in research on this topic between 2008 and 2010. Until recently, this topic continues to draw scholars from a variety of countries, who began to participate in the research. Cameroon, the Netherlands, and Finland, for example, have all published studies on this topic in the last 2 years. Dongmo et al. (Dongmo et al., 2020) from the University of Dschang developed an electrochemical biosensor for catechol detection in tea samples utilizing amino-grafting of montmorillonite. University of Yaoundé researchers Deutchoua et al. (Djitieu Deutchoua et al., 2019) devised two electrochemical techniques for determining antioxidant properties. As authentic samples, tea extracts were employed in this study. Overall, research on electrochemical sensing technology in tea is dominated by China, the United States, India, Brazil, Iran, and Japan. They were responsible for more than 70% of the academic papers.
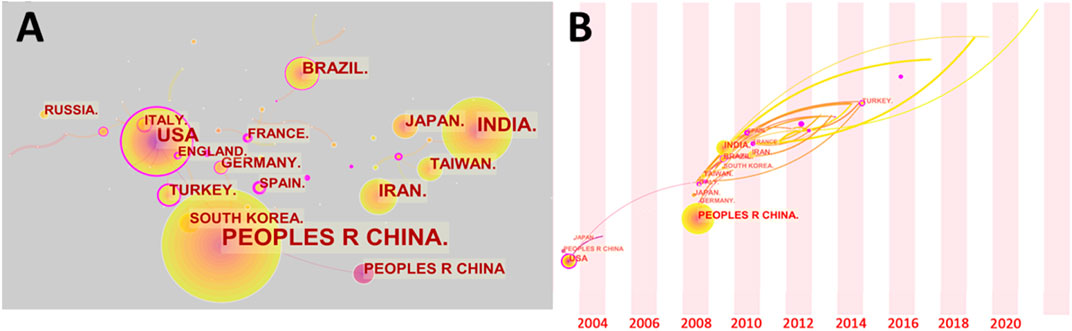
FIGURE 3. (A) Author’s country collaboration network map. (B) Time of involvement of different countries in electrochemical sensing research on tea.
Research Content Analysis
Cluster Analysis of Research Content
Cluster analysis of the content reveals some of the most important study avenues for this subject. Electrochemical detection, electrophoretic deposition, electrochemical investigation, hierarchical pore structure, caffeic acid, electrochemical analysis, techo-economic analysis, spent tea, carbon paste electrode, oxidative DNA damage, and mixed monolayers structure were among the 11 top themes identified by bibliometric clustering of research on electrochemical sensing for tea (Figure 4). The results show that the information on electrochemical procedures, which includes analytical techniques and sensor preparation techniques, is the most important aspect of this topic. Malakootian et al. (Malakootian et al., 2020) used a carbon paste electrode modified with Eu3+-doped NiO to detect Pb (II) and Cd (II) in black tea.
The clustering analysis results included caffeic acid, which is particularly important in tea, in addition to the development of electrochemical techniques and sensors. For the detection of caffeic acid in tea leaves, many of these studies propose an electrochemical sensing device. Chang and colleagues, for example, suggested a ratiometric electrochemical sensor for the detection of caffeic acid (Yin et al., 2021). Caffeic acid is electro-oxidized with two electrons in a diffusion-controlled method. Caffeic acid’s hydroxyl groups undergo two-electron transfer and release two protons, resulting in the quick production of the matching quinone. The surface modification presented in this paper can help improve caffeic acid diffusion at the electrode. For caffeic acid detection, Arajo et al. (Araújo et al., 2020a) developed a screen-printed electrode modified with carbon nanotubes (Figure 5). However, limited research has been done to assess the antioxidant effects of caffeic acid in tea (Lima et al., 2020). Furthermore, because caffeic acid has substantial electrochemical activity, it has been utilized as a signal in various experiments to demonstrate successful caffeic acid production (Li et al., 2020).
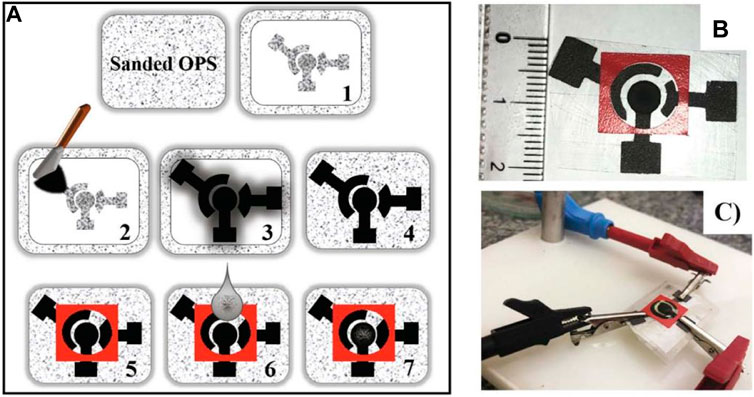
FIGURE 5. (A) Schematic representation, (B,C) images of SPE based caffeic acid biosensor (Araújo et al., 2020a). Copyright: Elsevier B.V.
Tea extracts are frequently utilized to examine the consequences of oxidative DNA damage due to their high antioxidant activity. Yury et al. (Kuzin et al., 2016), for example, described a GCE that had been electropolymerized with methylene blue. For analysis, the DNA solution was first combined with an oxidant before being immobilized on the modified GCE. By interrupting the DNA-methylene blue interactions, the voltammetric signal can be utilized to assess the degree of DNA damage (Kuzin et al., 2016). The presence of an antioxidant can help to slow down this process. They put this methodology to the test to see if it could determine the antioxidant capabilities of green tea extract. In addition to voltammetry, impedimetric technology (Kuzin et al., 2015). can be used for a similar purpose. Uliana and colleagues looked into whether tea may preserve DNA from dye-induced damage (Uliana et al., 2014). They proved that the tea solution could prevent adenine and guanine from reacting with the dye using electrochemical analysis. The current intensity of the adenine molecule had fallen by 60% of its initial value after an interaction duration of 180 s. Green tea appears to be able to minimize DNA molecule damage, according to the findings. Sumkova and Labuda (Šimková and Labuda, 2009) also suggested an electrochemical biosensor for detecting DNA damage and integrated the sensor into a commercial flow-through cell. This apparatus has been used to evaluate the antioxidative effects of ta extracts with great effectiveness.
Tea that has been discarded can be recycled as a valuable biological resource. High-temperature carbonization of wasted tea has been used to make electrodes or electrocatalysts in several investigations (Choi et al., 2016; Deng et al., 2016; Ahsan et al., 2020; Gao et al., 2021). In these investigations, electrochemical techniques were utilized as a characterisation tool to assess the performance of biochar. Gao et al. (Gao et al., 2021), for example, produced a biomorphic carbon electrode from discarded black tea and then used it to store potassium ions. Ahsan and colleagues constructed a cobalt-based electrocatalyst using discarded tea leaves as a template and then employed it for hydrogen and oxygen evolution and oxygen reduction (Ahsan et al., 2020).
Keywords Analysis
Keyword analysis can also reveal which research hotspots are being followed. The use of citation burst to analyze keywords might reveal how the study topic’s focus has shifted over time. The top 27 keywords with the greatest bursts of electrochemical sensing techniques for tea analysis are shown in Figure 6. The term bursts began in 2003, which was the year that additional research findings were published. Catechin, HPLC, performance liquid chromatography, green tea electrochemical detection, and flavonoid are some of the terms used in the beginning. The identification of catechin and flavonoids in tea using liquid chromatography is the main focus here. Electrochemical analysis was used as a technique for identifying samples after chromatographic separation of mixed samples at this point, rather than as a stand-alone sensing technique for tea detection. Long et al. (Hong et al., 2003), for example, suggested a method for detecting natural phenolic compounds in tea using liquid chromatography and multi-channel electrochemical detection. Similarly, Kotani et al. (Kotani et al., 2003) used an HPLC with electrochemical detection to identify catechins. Coulometric detection is frequently the most widely used electrochemical technology when paired with liquid chromatography (Chu et al., 2004; Kotani et al., 2007; Novak et al., 2010a; Shao et al., 2010; Narumi et al., 2014). Electrophoretic (Kartsova and Ganzha, 2006; Kartsova and Alekseeva, 2008) and voltammetric (Novak et al., 2010b; Hocker et al., 2017) approaches had previously been employed in conjunction with chromatographic methods to examine tea.
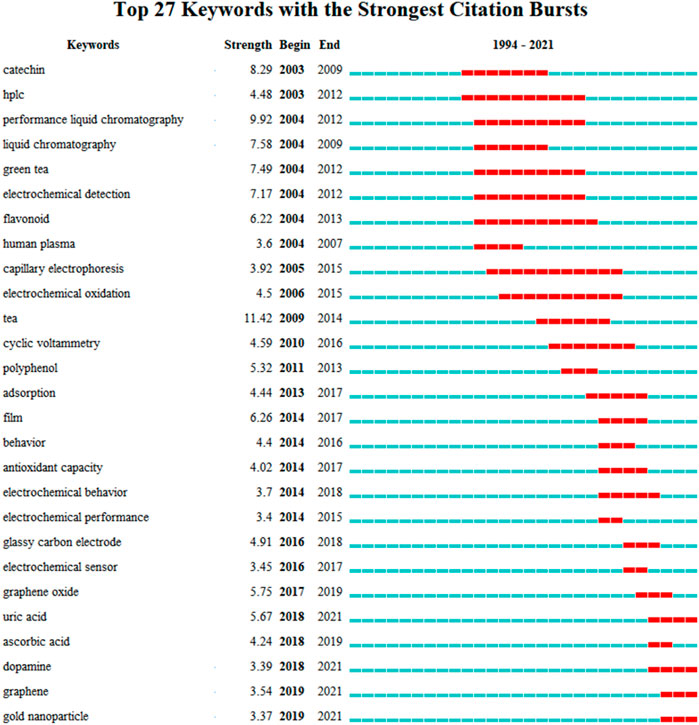
FIGURE 6. Top 27 keywords with the strongest bursts of electrochemical sensing techniques for tea analysis.
Around 2010, due to the development of electrochemical analysis techniques, a lot of work started to focus on the study of electrochemical behavior and antioxidant capacity measurement. It is worth noting that the studies of electrochemical behavior are not primarily an investigation of the electrochemical properties of tea components. According to the specific literature revealed, these works mainly focused on the use of extracts of tea leaves as inhibitors for the corrosion protection of metals. Changes in the electrochemical behavior of metals can be used as a characterization of the degree of corrosion. For example, Rauf et al. (Rauf and Mahdi, 2012) evaluated the effects of green tea on corrosion. Electrochemical frequency modulation and cyclic polarization scans were used for characterization. Unfortunately, the results showed that green tea did not show particularly excellent corrosion resistance. However, in a report by Tang et al. (Tang et al., 2018) green tea extract had good anti-corrosion efficacy on carbon steel. Pal and Das (Pal and Das, 2020b) also claimed that solid waste extract from tea factories is an excellent inhibitor.
The preparation of electrochemical sensors for tea detection has been the hottest topic since 2016. GCE has been chosen as the most commonly used commercial electrode. Different nanomaterials have been synthesized for the modification of GCE. Among them, graphene and its derivatives and gold nanoparticles have been studied the most.
Electrochemical Biosensor Performance Comparison
According to the results of the preceding two sub-sections’ analyses, the most essential aspect of electrochemical biosensing in the research of tea is the detection of tea components. We used bibliometrics to summarize these specific works. The major characteristics (linear detection range, LDR, and limit of detection, LOD) of various electrochemical sensors for tea composition detection are summarized in Table 1.
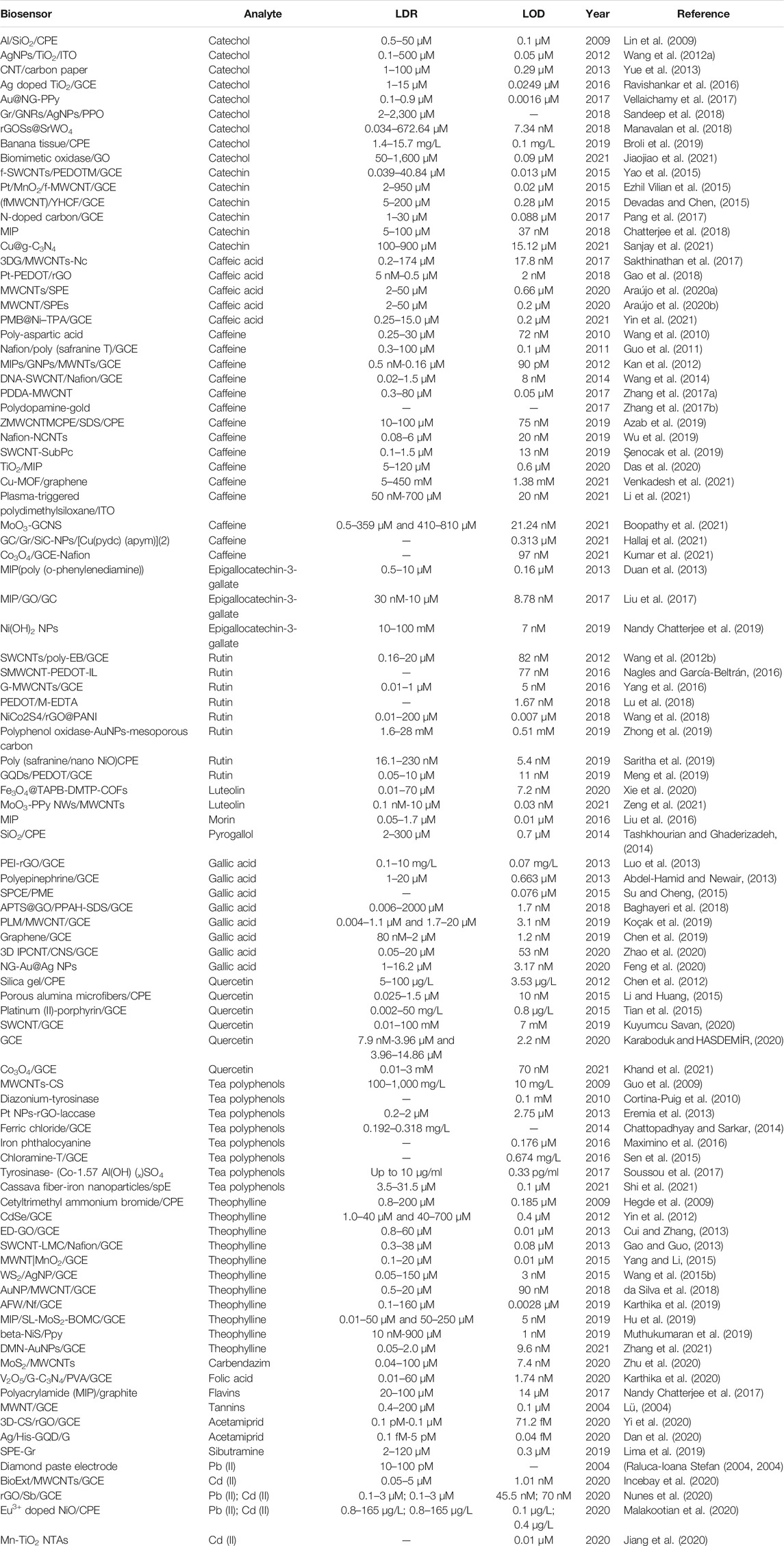
TABLE 1. Comparison of the performance of electrochemical biosensors for the detection of different tea components.
From Table 1, it can be seen that catechol, catechin, caffeine, rutin, gallic acid, quercetin, and tea polyphenols were the most detected tea components by electrochemical sensing technique. Acetamiprid, theophylline, Pb (II) and Cd (II) are the most frequently detected hazardous substances. This is due to the fact that excessive levels of pesticides and heavy metals in tea can lead to food safety problems. Overall, with the development of electrochemical sensing analysis technology, the detection range of different analytes has been enhanced while the detection limits have been reduced. For example, catechol had a detection limit of 0.1 M in the report in 2009 (Lin et al., 2009), which was reduced to 7.34 nM in 2018 (Manavalan et al., 2018). Among these, the widespread use of carbon nanomaterials has proven to be a game-changer. Due to the synergistic role of matrix or composite, carbonaceous materials (Graphene, CNTs, Carbon Nanofibres, and Mesoporous carbon, etc.) and conducting polymer materials act as reliable catalysts with metal oxide nanoparticles for the production of nonenzymatic sensors. It is worth noting that electrochemical sensors are a very sensitive detection technology. The sensors described above already meet the needs of detection, so the pursuit of high sensitivity does not necessarily have practical value. The focus of future electrochemical sensor research should be on how to improve stability and repeatability. Also, miniaturization of electrochemical sensors to fit field detection is an important direction.
Key Authors and Papers Analysis
Author Co-citation Analysis
Figure 7 shows the relationship network of authors’ co-cited information. From this figure, it can be seen that the research work of those authors has had an impact on the field. It is worth noting that the work here is not necessarily limited to the study of electrochemical biosensing for tea, but rather exemplifies the type of work that has had a greater impact on the topic. Lee et al.'s (Lee et al., 2002) work on the pharmacokinetics of catechins and epigal-locatechin-3-gallate in human-consumed tea gave early insights into electrochemical studies on tea. Yang et al. (Yang et al., 2001) explored the antioxidant activity of catechins in microsomal lipid peroxidation at an early stage and also influenced the study of electrochemical techniques for tea detection. Studies on antioxidants in tea have also been mainly influenced by Kilmartin et al. (Kilmartin et al., 2001) because their work suggested for the first time that cyclic voltammetry is an excellent technique for the evaluation of antioxidant activity.
In the design and fabrication of electrochemical biosensors, the electrochemical methodology established by Bard and Faulkner (Bard and Faulkner, 2002) is the most important foundation. Wang’s textbook on analytical chemistry is also the basis for electrochemical sensor design (Wang, 2000). The study of the electron transfer process of glucose oxi-dase in glucose biosensors has laid the foundation for many subsequent mechanistic studies of biosensors (Liu et al., 2005). Meanwhile, the technique for rutin and quercetin detection in plants proposed by Chen et al. (Chen et al., 2000) was applied to the operation of a biosensor for tea analysis. Similarly, the technique for gallic acid detection proposed by Abdel-Hamid and Newair (Abdel-Hamid and Newair, 2013) also affects many of the later tests for substances in tea. The work conducted by Ziyatdinova et al. (Ziyatdinova et al., 2012) is also instructive for the detection of flavonoids. The discovery of graphene has become a very important material in the assembly of electrochemical sensors. The graphene-based bioenzyme sensors proposed by Yang et al. (Yang et al., 2011) have influenced the study of sensors targeting the detection of tea components. Electrochemical techniques are used in this work not only for the detection of analytes, but also as a method for the synthesis of nanomaterials.
Reference Co-citation Analysis
Figure 8 illustrates the literature co-citation analysis relationship graph for electrochemical biosensors in tea analysis. As can be seen from the figure, the relationship between all the literature can be divided into five clusters, one of which contains a very large number of co-cited articles.
Cluster A has a dense network of pairs, representing work that is largely within a broad theme and is very closely linked. Important papers in this cluster include the work on gallic acid by Abdel-Hamind and Newair (Abdel-Hamid and Newair, 2013), previously mentioned in the authors’ co-citation analysis. Luo et al. (Luo et al., 2013) also proposed a method for gallic acid detection. Novak et al. (Novak et al., 2009) reported the electrochemical determination of epicatechin gallate using GCE. Masoum et al. (Masoum et al., 2014), Moccelini et al. (Moccelini et al., 2009) and Wang et al. (Wang et al., 2010) proposed electrochemical methods for catechin detection. Amare and Admassie (Amare and Admassie, 2012) proposed an electrochemical method for caffeine detection. Šeruga et al. (Šeruga et al., 2011) reported the method for polyphenols detection. All these techniques mentioned above are for the analysis of important components in tea.
Behind the detection of these substances is the passion of scientists for antioxidant substances. The explanation of the principle for antioxidant substances reported by Huang et al. (Huang et al., 2005) links cluster A and cluster B. In cluster B, the evaluation of the capacity of antioxidants using cyclic voltammetry reported by Kilmartin et al. (Kilmartin et al., 2001) is one of the most important works. A review of tea and tea polyphenols for cancer chemoprevention (Lambert and Yang, 2003) connects Cluster B and Cluster C. This links electrochemical sensing analysis to the specific health uses of tea components. Cluster C focuses on studies on the electrochemical behavior of tea components and human health, such as the relationship between the electrochemical oxidation of catechins and their antioxidant activity in microsomal lipid peroxidation (Yang et al., 2001).
Clusters D and E are two relatively independent groups. Cluster D focuses on tea as a source of carbon materials and has been used for energy storage. This cluster was accidentally included in the scope of this review because of the many electrochemical characterizations required in energy storage studies. This is a frequent occurrence in bibliometrics in sample statistics due to the sharing of similar keywords across different research directions. Cluster E is about the kinetic study of the electrochemistry of the tetraethylammonium/water interface. Since the abbreviation for tetraethylammonium is TEA, this literature was also accidentally included in the sample for this review.
Conclusion and Perspectives
This bibliometrics-based review summarizes the progress of electrochemical analysis for tea component sensing. The following conclusions can be drawn based on the focus of research in different time periods:
1) Between 1994 and 2010, electrochemical techniques were often used as a detection step after the separation of samples by chromatographic techniques.
2) After the study of tea components gradually came to our attention, the electrochemical behavior of those components that have electrochemical activity began to be investigated.
3) Since some important components in tea have very pronounced electrochemical redox behavior, electro-chemical sensors are starting to become a technique to detect the concentration of these components.
4) As materials science, especially nanomaterials, has become a hot topic, the use of nanomaterials to improve the performance of electrochemical sensors has become the focus of this field. A large number of papers have been published from 2010 onwards.
5) Electrochemical techniques allow not only the detection of specific components in tea but also the evaluation of their antioxidant properties. Therefore, different methodologies based on electrochemical biosensing have been established for measuring the antioxidant properties of tea.
Based on the bibliometric survey of trends in this area, we believe that future direction is likely to focus on the following areas:
1) The use of novel nanomaterial composites, particularly carbon materials and noble metal nanoparticles, will continue to be popular in the design and fabrication of biosensors.
2) Antioxidant property detection biosensors based on DNA ligand technology may become the norm for evaluating tea’s antioxidant properties.
3) Because electrochemical sensors allow for rapid assessment of antioxidant properties, this technique can be applied to a wide range of in vitro biological experiments.
4) Miniaturization of electrochemical biosensors is an important step toward applying this technology in the field for food detection and quality control.
Author Contributions
Conceptualization, JS; writing—original draft preparation, CW, YS, JS, and DD; writing—review and editing, JS; supervision, JS. All authors have read and agreed to the published version of the manuscript.
Conflict of Interest
The authors declare that the research was conducted in the absence of any commercial or financial relationships that could be construed as a potential conflict of interest.
Publisher’s Note
All claims expressed in this article are solely those of the authors and do not necessarily represent those of their affiliated organizations, or those of the publisher, the editors and the reviewers. Any product that may be evaluated in this article, or claim that may be made by its manufacturer, is not guaranteed or endorsed by the publisher.
References
Abdel-Hamid, R., and Newair, E. F. (2013). Adsorptive Stripping Voltammetric Determination of Gallic Acid Using an Electrochemical Sensor Based on Polyepinephrine/Glassy Carbon Electrode and its Determination in Black Tea Sample. J. Electroanalytical Chem. 704, 32–37. doi:10.1016/j.jelechem.2013.06.006
Ahsan, M. A., Imam, M. A., Santiago, A. R. P., Rodriguez, A., Alvarado-Tenorio, B., Bernal, R., et al. (2020). Spent Tea Leaves Templated Synthesis of Highly Active and Durable Cobalt-Based Trifunctional Versatile Electrocatalysts for Hydrogen and Oxygen Evolution and Oxygen Reduction Reactions. Green. Chem. 22, 6967–6980. doi:10.1039/d0gc02155e
Alqarni, S. A., Hussein, M. A., Ganash, A. A., and Khan, A. (2020). Composite Material–Based Conducting Polymers for Electrochemical Sensor Applications: A Mini Review. BioNanoScience 10, 351–364. doi:10.1007/s12668-019-00708-x
Amare, M., and Admassie, S. (2012). Polymer Modified Glassy Carbon Electrode for the Electrochemical Determination of Caffeine in Coffee. Talanta 93, 122–128. doi:10.1016/j.talanta.2012.01.058
Araújo, D. A. G., Camargo, J. R., Pradela-Filho, L. A., Lima, A. P., Muñoz, R. A. A., Takeuchi, R. M., et al. (2020). A Lab-Made Screen-Printed Electrode as a Platform to Study the Effect of the Size and Functionalization of Carbon Nanotubes on the Voltammetric Determination of Caffeic Acid. Microchemical J. 158, 105297. doi:10.1016/j.microc.2020.105297
Araújo, D. A. G., Camargo, J. R., Pradela-Filho, L. A., Lima, A. P., Muñoz, R. A. A., Takeuchi, R. M., et al. (2020). A Lab-Made Screen-Printed Electrode as a Platform to Study the Effect of the Size and Functionalization of Carbon Nanotubes on the Voltammetric Determination of Caffeic Acid. Microchemical J. 158, 105297. doi:10.1016/j.microc.2020.105297
Azab, S. M., Shehata, M., and Fekry, A. M. (2019). A Novel Electrochemical Analysis of the Legal Psychoactive Drug Caffeine Using a Zeolite/MWCNT Modified Carbon Paste Sensor. New J. Chem. 43, 15359–15367. doi:10.1039/c9nj04070f
Baghayeri, M., Amiri, A., Hasheminejad, E., and Mahdavi, B. (2018). Poly(Aminohippuric Acid)–Sodium Dodecyl Sulfate/Functionalized Graphene Oxide Nanocomposite for Amplified Electrochemical Sensing of Gallic Acid. J. Iranian Chem. Soc. 15, 1931–1938. doi:10.1007/s13738-018-1390-3
Bard, A. J., and Faulkner, L. R. (2002). Student Solutions Manual to Accompany Electrochemical Methods: Fundamentals and Applicaitons. 2e. John Wiley & Sons. 0-471-40521-3.
Beringer, T., Kulak, M., Müller, C., Schaphoff, S., and Jans, Y. (2020). First Process-Based Simulations of Climate Change Impacts on Global Tea Production Indicate Large Effects in the World’s Major Producer Countries. Environ. Res. Lett. 15, 034023. doi:10.1088/1748-9326/ab649b
Boopathy, G., Keerthi, M., Chen, S.-M., Meenakshi, S., and Umapathy, M. J. (2021). Molybdenum Trioxide Embedded Graphitic Carbon Nitride Sheets Modified Electrode for Caffeine Sensing in Green Tea and Coffee Powder. Mater. Chem. Phys. 269, 124735. doi:10.1016/j.matchemphys.2021.124735
Broli, N., Vallja, L., Shehu, A., and Vasjari, M. (2019). Determination of Catechol in Extract of Tea Using Carbon Paste Electrode Modified with Banana Tissue. J. Food Process. Preservation 43, e13838. doi:10.1111/jfpp.13838
Chapagain, A. K., and Hoekstra, A. Y. (2007). The Water Footprint of Coffee and Tea Consumption in the Netherlands. Ecol. Econ. 64, 109–118. doi:10.1016/j.ecolecon.2007.02.022
Chatterjee, T. N., Das, D., Roy, R. B., Tudu, B., Sabhapondit, S., Tamuly, P., et al. (2018). Molecular Imprinted Polymer Based Electrode for Sensing Catechin (+ C) in Green Tea. IEEE Sensors J. 18, 2236–2244. doi:10.1109/jsen.2018.2791661
Chattopadhyay, S., and Sarkar, P. (2014). Estimation of Tea Polyphenols by Electrochemical Sensors via Ferric Chloride Modified Electrodes. JOURNAL INDIAN CHEMICAL SOCIETY 91, 2291–2298. doi:10.5281/zenodo.5746521
Chen, C. (2006). CiteSpace II: Detecting and Visualizing Emerging Trends and Transient Patterns in Scientific Literature. J. Am. Soc. Inf. Sci. Tech. 57, 359–377. doi:10.1002/asi.20317
Chen, C. (2004). Searching for Intellectual Turning Points: Progressive Knowledge Domain Visualization. Proc. Natl. Acad. Sci. 101, 5303–5310. doi:10.1073/pnas.0307513100
Chen, C., and Song, M. (2019). Visualizing a Field of Research: A Methodology of Systematic Scientometric Reviews. PloS one 14, e0223994. doi:10.1371/journal.pone.0223994
Chen, G., Zhang, H., and Ye, J. (2000). Determination of Rutin and Quercetin in Plants by Capillary Electrophoresis with Electrochemical Detection. Analytica Chim. Acta 423, 69–76. doi:10.1016/S0003-2670(00)01099-0
Chen, M., Lv, H., Li, X., Tian, Z., and Ma, X. (2019). Determination of Gallic Acid in Tea by a Graphene Modified Glassy Carbon Electrode. Int. J. Electrochem. Sci. 14, 4852–4860. doi:10.20964/2019.05.23
Chen, X., Li, Q., Yu, S., Lin, B., and Wu, K. (2012). Activated Silica Gel Based Carbon Paste Electrodes Exhibit Signal Enhancement for Quercetin. Electrochimica Acta 81, 106–111. doi:10.1016/j.electacta.2012.07.063
Choi, C., Seo, S.-D., Kim, B.-K., and Kim, D.-W. (2016). Enhanced Lithium Storage in Hierarchically Porous Carbon Derived from Waste Tea Leaves. Scientific Rep. 6, 39099. doi:10.1038/srep39099
Chu, K. O., Wang, C. C., Rogers, M. S., Choy, K. W., and Pang, C. P. (2004). Determination of Catechins and Catechin Gallates in Biological Fluids by HPLC with Coulometric Array Detection and Solid Phase Extraction. Analytica Chim. Acta 510, 69–76. doi:10.1016/j.aca.2003.12.060
Cortina-Puig, M., Muñoz-Berbel, X., Calas-Blanchard, C., and Marty, J.-L. (2010). Diazonium-Functionalized Tyrosinase-Based Biosensor for the Detection of Tea Polyphenols. Microchimica Acta 171, 187–193. doi:10.1007/s00604-010-0425-y
Cui, F., and Zhang, X. (2013). A Method Based on Electrodeposition of Reduced Graphene Oxide on Glassy Carbon Electrode for Sensitive Detection of Theophylline. J. Solid State. Electrochemistry 17, 167–173. doi:10.1007/s10008-012-1867-4
da Silva, W., Ghica, M. E., and Brett, C. M. A. (2018). Gold Nanoparticle Decorated Multiwalled Carbon Nanotube Modified Electrodes for the Electrochemical Determination of Theophylline. Anal. Methods 10, 5634–5642. doi:10.1039/C8AY02150C
Dan, X., Ruiyi, L., Zaijun, L., Haiyan, Z., Zhiguo, G., and Guangli, W. (2020). Facile Strategy for Synthesis of Silver-Graphene Hybrid with Controllable Size and Excellent Dispersion for Ultrasensitive Electrochemical Detection of Acetamiprid. Appl. Surf. Sci. 512, 145628. doi:10.1016/j.apsusc.2020.145628
Das, D., Chatterjee, T. N., Roy, R. B., Tudu, B., Hazarika, A. K., Sabhapondit, S., et al. (2020). Titanium Oxide Nanocubes Embedded Molecularly Imprinted Polymer-Based Electrode for Selective Detection of Caffeine in Green Tea. IEEE Sensors J. 20, 6240–6247. doi:10.1109/jsen.2020.2972773
de Carvalho Couto, C., dos Santos, D. G., Oliveira, E. M. M., and Freitas-Silva, O. (2021). Global Situation of Reference Materials to Assure Coffee, Cocoa, and Tea Quality and Safety. Trac Trends Anal. Chem., 116381. doi:10.1016/j.trac.2021.116381
Deng, X., Chan, C. K., and Tüysüz, H. (2016). Spent Tea Leaf Templating of Cobalt-Based Mixed Oxide Nanocrystals for Water Oxidation. ACS Appl. Mater. Inter. 8, 32488–32495. doi:10.1021/acsami.6b12005
Devadas, B., and Chen, S.-M. (2015). Controlled Electrochemical Synthesis of Yttrium (III) Hexacyanoferrate Micro Flowers and Their Composite with Multiwalled Carbon Nanotubes, and its Application for Sensing Catechin in Tea Samples. J. Solid State. Electrochemistry 19, 1103–1112. doi:10.1007/s10008-014-2715-5
Djitieu Deutchoua, A. D., Ngueumaleu, Y., Kenne Dedzo, G., Kenfack Tonle, I., and Ngameni, E. (2019). Electrochemical Study of DPPH Incorporated in Carbon Paste Electrode as Potential Tool for Antioxidant Properties Determination. Electroanalysis 31, 335–342.
Dongmo, L. M., Jiokeng, S. L., Pecheu, C. N., Walcarius, A., and Tonle, I. K. (2020). Amino-Grafting of Montmorillonite Improved by Acid Activation and Application to the Electroanalysis of Catechol. Appl. Clay Sci. 191, 105602. doi:10.1016/j.clay.2020.105602
Duan, Y., Luo, X., Qin, Y., Zhang, H., Sun, G., Sun, X., et al. (2013). Determination of Epigallocatechin-3-Gallate with a High-Efficiency Electrochemical Sensor Based on a Molecularly Imprinted Poly(o-Phenylenediamine) Film. J. Appl. Polym. Sci. 129, 2882–2890. doi:10.1002/app.39002
Eremia, S. A. V., Vasilescu, I., Radoi, A., Litescu, S.-C., and Radu, G.-L. (2013). Disposable Biosensor Based on Platinum Nanoparticles-Reduced Graphene Oxide-Laccase Biocomposite for the Determination of Total Polyphenolic Content. Talanta 110, 164–170. doi:10.1016/j.talanta.2013.02.029
Ezhil Vilian, A. T., Madhu, R., Chen, S.-M., Veeramani, V., Sivakumar, M., Huh, Y. S., et al. (2015). Facile Synthesis of MnO2/Carbon Nanotubes Decorated with a Nanocomposite of Pt Nanoparticles as a New Platform for the Electrochemical Detection of Catechin in Red Wine and Green Tea Samples. J. Mater. Chem. B 3, 6285–6292. doi:10.1039/C5TB00508F
Feng, L., Gwee, X., Kua, E.-H., and Ng, T.-P. (2010). Cognitive Function an Tea Consumption in Community Dwelling Older Chinese in Singapore. J. Nutr. Health Aging 14, 433–438. doi:10.1007/s12603-010-0095-9
Feng, S., Zhou, X., Chen, X., Zhang, G., Liu, G., Wu, N., et al. (2020). Au@ Ag Core-Shell Nanomaterials Embedded in N-Doped Graphene: A Novel Electrochemical Sensor for Determination of Gallic Acid. Int. J. Electrochem. Sci. 15, 6908–6919. doi:10.20964/2020.07.28
Gao, L., Yue, R., Xu, J., Liu, Z., and Chai, J. (2018). Pt-PEDOT/RGO Nanocomposites: One-Pot Preparation and Superior Electrochemical Sensing Performance for Caffeic Acid in Tea. J. Electroanalytical Chem. 816, 14–20. doi:10.1016/j.jelechem.2018.03.024
Gao, Y., and Guo, L. (2013). A Sensitive Theophylline Sensor Based on a Single Walled Carbon Nanotube–Large Mesoporous Carbon/Nafion/Glassy Carbon Electrode. Anal. Methods 5, 5785–5791. doi:10.1039/C3AY41236A
Gao, Y., Ru, Q., Zheng, M., Pan, Z., Lei, T., Zhang, J., et al. (2021). Recovery of Kitchen Bio-Waste from Spent Black Tea as Hierarchical Biomorphic Carbon Electrodes for Ultra-long Lifespan Potassium-Ion Storage. Appl. Surf. Sci. 555, 149675. doi:10.1016/j.apsusc.2021.149675
Guo, D., Zheng, D., Mo, G., and Ye, J. (2009). Adsorptive Stripping Voltammetric Detection of Tea Polyphenols at Multiwalled Carbon Nanotubes‐chitosan Composite Electrode. Electroanalysis: Int. J. Devoted Fundam. Pract. Aspects Electroanalysis 21, 762–766. doi:10.1002/elan.200804475
Guo, S., Zhu, Q., Yang, B., Wang, J., and Ye, B. (2011). Determination of Caffeine Content in Tea Based on Poly(Safranine T) Electroactive Film Modified Electrode. Food Chem. 129, 1311–1314. doi:10.1016/j.foodchem.2011.05.095
Guth, U., Vonau, W., and Zosel, J. (2009). Recent Developments in Electrochemical Sensor Application and Technology—A Review. Meas. Sci. Tech. 20, 042002. doi:10.1088/0957-0233/20/4/042002
Hagerman, A. E., Dean, R. T., and Davies, M. J. (2003). Radical Chemistry of Epigallocatechin Gallate and its Relevance to Protein Damage. Arch. Biochem. Biophys. 414, 115–120. doi:10.1016/s0003-9861(03)00158-9
Hallaj, R., Soltani, E., Mafakheri, S., and Ghadermazi, M. (2021). A Surface-Modified Silicon Carbide Nanoparticles Based Electrochemical Sensor for Free Interferences Determination of Caffeine in Tea and Coffee. Mater. Sci. Eng. B 274, 115473. doi:10.1016/j.mseb.2021.115473
Hegde, R. N., Hosamani, R. R., and Nandibewoor, S. T. (2009). Electrochemical Oxidation and Determination of Theophylline at a Carbon Paste Electrode Using Cetyltrimethyl Ammonium Bromide as Enhancing Agent. null 42, 2665–2682. doi:10.1080/00032710903243620
Hocker, N., Wang, C., Prochotsky, J., Eppurath, A., Rudd, L., and Perera, M. (2017). Quantification of Antioxidant Properties in Popular Leaf and Bottled Tea by High-Performance Liquid Chromatography (HPLC), Spectrophotometry, and Voltammetry. Anal. Lett. 50, 1640–1656. doi:10.1080/00032719.2016.1242008
Hong, L., Yongxin, Z., and Kissinger, P. T. (2003). Liquid Chromatography with Multi-Channel Electrochemical Detection for the Determination of Natural Phenolic Compounds. Chin. J. Anal. Chem. 31, 631–634. doi:10.1016/S1570-0232(02)00087-9
Hu, X., Xi, J., Xia, Y., Zhao, F., and Zeng, B. (2019). Space-Confined Synthesis of Ordered Mesoporous Carbon Doped with Single-Layer MoS2–Boron for the Voltammetric Determination of Theophylline. Microchimica Acta 186, 694. doi:10.1007/s00604-019-3824-8
Huang, D., Ou, B., and Prior, R. L. (2005). The Chemistry behind Antioxidant Capacity Assays. J. Agric. Food Chem. 53, 1841–1856. doi:10.1021/jf030723c
Incebay, H., Aktepe, L., and Leblebici, Z. (2020). An Electrochemical Sensor Based on Green Tea Extract for Detection of Cd(II) Ions by Differential Pulse Anodic Stripping Voltammetry. Surf. Inter. 21, 100726. doi:10.1016/j.surfin.2020.100726
Jiang, M., Ma, M.-J., Lin, C.-H., Yang, M., Fang, L., Liu, J.-H., et al. (2020). Uniform Manganese-Loaded Titanium Dioxide Nanotube Arrays for Accurate Detection of Trace Cd2+ in Water, Soil and Tea: Enhanced Stability and Sensitivity. Chem. Eng. J. 400, 125972. doi:10.1016/j.cej.2020.125972
Jiaojiao, X., Pengyun, W., Bin, Z., and Onyinye, A. I. (2021). Enhancing Electrochemical Sensing for Catechol by Biomimetic Oxidase Covalently Functionalized Graphene Oxide. Bioproc. Biosyst. Eng. 44, 343–353. doi:10.1007/s00449-020-02446-x
Kan, X., Liu, T., Li, C., Zhou, H., Xing, Z., and Zhu, A. (2012). A Novel Electrochemical Sensor Based on Molecularly Imprinted Polymers for Caffeine Recognition and Detection. J. Solid State. Electrochemistry 16, 3207–3213. doi:10.1007/s10008-012-1760-1
Karaboduk, K., and Hasdemi̇r, E. (2020). Simultaneous Determination of Quercetin and Luteolin in Mate and White Tea Samples by Voltammetry. REVUE ROUMAINE DE CHIMIE 65, 375–385. doi:10.33224/rrch/2020.65.4.07
Karimi-Maleh, H., Alizadeh, M., Orooji, Y., Karimi, F., Baghayeri, M., Rouhi, J., et al. (2021). Guanine-Based DNA Biosensor Amplified with Pt/SWCNTs Nanocomposite as Analytical Tool for Nanomolar Determination of Daunorubicin as an Anticancer Drug: A Docking/Experimental Investigation. Ind. Eng. Chem. Res. 60, 816–823. doi:10.1021/acs.iecr.0c04698
Karimi-Maleh, H., Ayati, A., Davoodi, R., Tanhaei, B., Karimi, F., Malekmohammadi, S., et al. (2021). Recent Advances in Using of Chitosan-Based Adsorbents for Removal of Pharmaceutical Contaminants: A Review. J. Clean. Prod. 291, 125880. doi:10.1016/j.jclepro.2021.125880
Karimi-Maleh, H., Karimi, F., Fu, L., Sanati, A. L., Alizadeh, M., Karaman, C., et al. (2022). Cyanazine Herbicide Monitoring as a Hazardous Substance by a DNA Nanostructure Biosensor. J. Hazard. Mater. 423, 127058. doi:10.1016/j.jhazmat.2021.127058
Karimi-Maleh, H., Khataee, A., Karimi, F., Baghayeri, M., Fu, L., Rouhi, J., et al. A Green and Sensitive Guanine-Based DNA Biosensor for Idarubicin Anticancer Monitoring in Biological Samples: A Simple and Fast Strategy for Control of Health Quality in Chemotherapy Procedure Confirmed by Docking Investigation. Chemosphere 2021, 132928. doi:10.1016/j.chemosphere.2021.132928
Karimi-Maleh, H., Orooji, Y., Karimi, F., Alizadeh, M., Baghayeri, M., Rouhi, J., et al. (2021). A Critical Review on the Use of Potentiometric Based Biosensors for Biomarkers Detection. Biosens. Bioelectron., 113252. doi:10.1016/j.bios.2021.113252
Karthika, A., Sudhakar, C., Suganthi, A., and Rajarajan, M. (2019). Eco-Friendly Synthesis of Aloe Vera Plant Extract Decorated Iron Tungstate Nanorods Immobilized Nafion for Selective and Sensitive Determination of Theophylline in Blood Serum, Black Tea and Urine Samples. J. Sci. Adv. Mater. Devices 4, 554–560. doi:10.1016/j.jsamd.2019.09.004
Karthika, A., Suganthi, A., and Rajarajan, M. (2020). An In-Situ Synthesis of Novel V2O5/G-C3n4/PVA Nanocomposite for Enhanced Electrocatalytic Activity toward Sensitive and Selective Sensing of Folic Acid in Natural Samples. Arabian J. Chem. 13, 3639–3652. doi:10.1016/j.arabjc.2019.12.009
Kartsova, L. A., and Ganzha, O. V. (2006). Electrophoretic Separation of Tea Flavanoids in the Modes of Capillary (Zone) Electrophoresis and Micellar Electrokinetic Chromatography. Russ. J. Appl. Chem. 79, 1110–1114. doi:10.1134/S1070427206070135
Kartsova, L., and Alekseeva, A. (2008). Chromatographic and Electrophoretic Methods for Determining Polyphenol Compounds. J. Anal. Chem. 63, 1024–1033. doi:10.1134/s1061934808110026
Khand, N. H., Solangi, A. R., Ameen, S., Fatima, A., Buledi, J. A., Mallah, A., et al. (2021). A New Electrochemical Method for the Detection of Quercetin in Onion, Honey and Green Tea Using Co3O4 Modified GCE. J. Food Meas. Characterization 15, 3720–3730. doi:10.1007/s11694-021-00956-0
Kilmartin, P. A., Zou, H., and Waterhouse, A. L. (2001). A Cyclic Voltammetry Method Suitable for Characterizing Antioxidant Properties of Wine and Wine Phenolics. J. Agric. Food Chem. 49, 1957–1965. doi:10.1021/jf001044u
Koch, W., Kukula-Koch, W., Komsta, Ł., Marzec, Z., Szwerc, W., and Głowniak, K. (2018). Green Tea Quality Evaluation Based on its Catechins and Metals Composition in Combination with Chemometric Analysis. Molecules 23, 1689. doi:10.3390/molecules23071689
Kochman, J., Jakubczyk, K., Antoniewicz, J., Mruk, H., and Janda, K. H. (2021). Benefits and Chemical Composition of Matcha Green Tea: A Review. Molecules 26, 85.
Kotani, A., Miyashita, N., and Kusu, F. (2003). Determination of Catechins in Human Plasma after Commercial Canned Green Tea Ingestion by High-Performance Liquid Chromatography with Electrochemical Detection Using a Microbore Column. J. Chromatogr. B 788, 269–275. doi:10.1016/s1570-0232(02)01036-x
Kotani, A., Takahashi, K., Hakamata, H., Kojima, S., and Kusu, F. (2007). Attomole Catechins Determination by Capillary Liquid Chromatography with Electrochemical Detection. Anal. Sci. 23, 157–163. doi:10.2116/analsci.23.157
Kottawa-Arachchi, J. D., Gunasekare, M. K., and Ranatunga, M. A. (2019). Biochemical Diversity of Global Tea [Camellia Sinensis (L.) O. Kuntze] Germplasm and its Exploitation: A Review. Genet. Resour. Crop Evol. 66, 259–273. doi:10.1007/s10722-018-0698-2
Koçak, Ç. C., Karabiberoğlu, Ş. U., and Dursun, Z. (2019). Highly Sensitive Determination of Gallic Acid on Poly (L-Methionine)-Carbon Nanotube Composite Electrode. J. Electroanalytical Chem. 853, 113552. doi:10.1016/j.jelechem.2019.113552
Kumar, R., Qadir, G., Rajar, K., Balouch, A., Ibupoto, Z. H., and Parkash, A. (2021). Voltammetric Detection of Caffeine Content in Different Tea Stuffs by Using Co3O4/GCE-Nafion Electrode. J. Iranian Chem. Soc. 18, 701–708. doi:10.1007/s13738-020-02059-x
Kuyumcu Savan, E. (2020). Square Wave Voltammetric (SWV) Determination of Quercetin in Tea Samples at a Single-Walled Carbon Nanotube (SWCNT) Modified Glassy Carbon Electrode (GCE). Anal. Lett. 53, 858–872. doi:10.1080/00032719.2019.1684514
Kuzin, Y., Ivanov, A., Evtugyn, G., and Hianik, T. (2016). Voltammetric Detection of Oxidative DNA Damage Based on Interactions between Polymeric Dyes and DNA. Electroanalysis 28, 2956–2964. doi:10.1002/elan.201600297
Kuzin, Y., Porfireva, A., Stepanova, V., Evtugyn, V., Stoikov, I., Evtugyn, G., et al. (2015). Impedimetric Detection of DNA Damage with the Sensor Based on Silver Nanoparticles and Neutral Red. Electroanalysis 27, 2800–2808. doi:10.1002/elan.201500312
Lambert, J. D., and Yang, C. S. (2003). Cancer Chemopreventive Activity and Bioavailability of Tea and Tea Polyphenols. Mutat. Research/Fundamental Mol. Mech. Mutagenesis 523–524, 201–208. doi:10.1016/S0027-5107(02)00336-6
Lee, M.-J., Maliakal, P., Chen, L., Meng, X., Bondoc, F. Y., Prabhu, S., et al. (2002). Pharmacokinetics of Tea Catechins after Ingestion of Green Tea and (−)-Epigallocatechin-3-Gallate by Humans: Formation of Different Metabolites and Individual Variability. Cancer Epidemiol. Prev. Biomarkers 11, 1025–1032.
Li, Y., and Huang, W. (2015). Electrode Modified with Porous Alumina Microfibers as a Highly Sensitive Electrochemical Sensor for Quercetin. Anal. Methods 7, 2537–2541. doi:10.1039/C5AY00206K
Li, Y., Luo, Z., Li, G., Belwal, T., Li, L., Xu, Y., et al. (2021). Interference-Free Detection of Caffeine in Complex Matrices Using a Nanochannel Electrode Modified with Binary Hydrophilic–Hydrophobic PDMS. ACS sensors 6, 1604–1612. doi:10.1021/acssensors.1c00004
Li, Y., Qi, H., Fan, M., Zhu, Z., Zhan, S., Li, L., et al. (2020). Quantifying the Efficiency of O-Benzoquinones Reaction with Amino Acids and Related Nucleophiles by Cyclic Voltammetry. Food Chem. 317, 126454. doi:10.1016/j.foodchem.2020.126454
Lima, A. B., dos Santos, W. T., and Compton, R. G. (2019). Simple and Sensitive Determination of Sibutramine in Slimming Tea Beverages Using a Carbon Screen‐printed Electrode with Adsorptive Stripping Voltammetry. Electroanalysis 31, 975–980. doi:10.1002/elan.201800888
Lima, A. P., dos Santos, W. T. P., Nossol, E., Richter, E. M., and Munoz, R. A. A. (2020). Critical Evaluation of Voltammetric Techniques for Antioxidant Capacity and Activity: Presence of Alumina on Glassy-Carbon Electrodes Alters the Results. Electrochimica Acta 358, 136925. doi:10.1016/j.electacta.2020.136925
Lin, H., Gan, T., and Wu, K. (2009). Sensitive and Rapid Determination of Catechol in Tea Samples Using Mesoporous Al-Doped Silica Modified Electrode. Food Chem. 113, 701–704. doi:10.1016/j.foodchem.2008.07.073
Liu, R., Long, L., Lei, C., Zhaoyang, W., Liu, Y., and Sijia, L. (2016). Fabrication and Application of a Molecularly Imprinted Sensor for Morin Detection. Chin. J. Anal. Chem., 385–390.
Liu, Y., Wang, D.-Z., Zhang, S.-Z., and Zhao, H.-M. (2015). Global Expansion Strategy of Chinese Herbal Tea Beverage. Adv. J. Food Sci. Tech. 7, 739–745. doi:10.19026/ajfst.7.1731
Liu, Y., Wang, M., Zhao, F., Xu, Z., and Dong, S. (2005). The Direct Electron Transfer of Glucose Oxidase and Glucose Biosensor Based on Carbon Nanotubes/Chitosan Matrix. Biosens. Bioelectron. 21, 984–988. doi:10.1016/j.bios.2005.03.003
Liu, Y., Zhu, L., Hu, Y., Peng, X., and Du, J. (2017). A Novel Electrochemical Sensor Based on a Molecularly Imprinted Polymer for the Determination of Epigallocatechin Gallate. Food Chem. 221, 1128–1134. doi:10.1016/j.foodchem.2016.11.047
Lu, L., Wu, L., Wang, W., Long, X., Xu, J., and He, H. (2018). Electrochemical Sensor Based on Poly (3, 4-Ethylenedioxy-Thiophene) Doped with Transition Metals for Detecting Rutin in Buck Wheat Tea. Int. J. Electrochem. Sci. 13, 2126–2135. doi:10.20964/2018.02.66
Lü, S. (2004). Electrochemical Determination of Tannins Using Multiwall Carbon Nanotubes Modified Glassy Carbon Electrode. Russ. J. Electrochemistry 40, 750–754. doi:10.1023/B:RUEL.0000035260.35980.f7
Luo, J. H., Li, B. L., Li, N. B., and Luo, H. Q. (2013). Sensitive Detection of Gallic Acid Based on Polyethyleneimine-Functionalized Graphene Modified Glassy Carbon Electrode. Sensors Actuators B: Chem. 186, 84–89. doi:10.1016/j.snb.2013.05.074
Luo, W., Ang, C. Y., Gehring, T. A., Heinze, T. M., Lin, L. J., and Mattia, A. (2003). Determination of Phenolic Compounds in Dietary Supplements and Tea Blends Containing Echinacea by Liquid Chromatography with Coulometric Electrochemical Detection. J. AOAC Int. 86, 202–208. doi:10.1093/jaoac/86.2.202
Malakootian, M., Abolghasemi, H., and Mahmoudi-Moghaddam, H. (2020). A Novel Electrochemical Sensor Based on the Modified Carbon Paste Using Eu3+− Doped NiO for Simultaneous Determination of Pb (II) and Cd (II) in Food Samples. J. Electroanalytical Chem. 876, 114474. doi:10.1016/j.jelechem.2020.114474
Manavalan, S., Govindasamy, M., Chen, S.-M., Rajaji, U., Chen, T.-W., Ajmal Ali, M., et al. (2018). Reduced Graphene Oxide Supported Raspberry-like SrWO4 for Sensitive Detection of Catechol in Green Tea and Drinking Water Samples. J. Taiwan Inst. Chem. Eng. 89, 215–223. doi:10.1016/j.jtice.2018.05.001
Mani, V., Beduk, T., Khushaim, W., Ceylan, A. E., Timur, S., Wolfbeis, O. S., et al. (2021). Electrochemical Sensors Targeting Salivary Biomarkers: A Comprehensive Review. Trac Trends Anal. Chem. 135, 116164. doi:10.1016/j.trac.2020.116164
Marx, W., Haunschild, R., and Bornmann, L. (2017). Global Warming and Tea Production—The Bibliometric View on a Newly Emerging Research Topic. Climate 5, 46. doi:10.3390/cli5030046
Masoum, S., Behpour, M., Azimi, F., and Motaghedifard, M. H. (2014). Potentiality of Chemometric Approaches for the Determination of (+)-Catechin in Green Tea Leaves at the Surface of Multiwalled Carbon Nanotube Paste Electrode. Sensors Actuators B: Chem. 193, 582–591. doi:10.1016/j.snb.2013.12.022
Maximino, M. D., Martin, C. S., Paulovich, F. V., and Alessio, P. (2016). Layer‐by‐layer Thin Film of Iron Phthalocyanine as a Simple and Fast Sensor for Polyphenol Determination in Tea Samples. J. Food Sci. 81, C2344–C2351. doi:10.1111/1750-3841.13394
McCants, A. E. (2008). Poor Consumers as Global Consumers: The Diffusion of Tea and Coffee Drinking in the Eighteenth Century 1. Econ. Hist. Rev. 61, 172–200. doi:10.1111/j.1468-0289.2008.00429.x
Meng, R., Li, Q., Zhang, S., Tang, J., Ma, C., and Jin, R. (2019). GQDs/PEDOT Bilayer Films Modified Electrode as a Novel Electrochemical Sensing Platform for Rutin Detection. Int. J. Electrochem. Sc 14, 11000–11011. doi:10.20964/2019.12.40
Moccelini, S. K., Fernandes, S. C., de Camargo, T. P., Neves, A., and Vieira, I. C. (2009). Self-Assembled Monolayer of Nickel (II) Complex and Thiol on Gold Electrode for the Determination of Catechin. Talanta 78, 1063–1068. doi:10.1016/j.talanta.2009.01.038
Muthukumaran, P., Ramya, R., Thivya, P., Wilson, J., and Ravi, G. (2019). Nanocomposite Based on Restacked Crystallites of β-NiS and Ppy for the Determination of Theophylline and Uric Acid on Screen-Printed Electrodes. New J. Chem. 43, 19397–19407. doi:10.1039/c9nj04246f
Mzembe, A. N., Lindgreen, A., Maon, F., and Vanhamme, J. (2016). Investigating the Drivers of Corporate Social Responsibility in the Global Tea Supply Chain: A Case Study of Eastern Produce Limited in Malawi. Corporate Soc. Responsibility Environ. Manag. 23, 165–178. doi:10.1002/csr.1370
Nagles, E., and García-Beltrán, O. (2016). Determination of Rutin in Black Tea by Adsorption Voltammetry (AdV) in the Presence of Morin and Quercetin. Food Anal. Methods 9, 3420–3427. doi:10.1007/s12161-016-0538-y
Nandy Chatterjee, T., Banerjee Roy, R., Tudu, B., Pramanik, P., Deka, H., Tamuly, P., et al. (2017). Detection of Theaflavins in Black Tea Using a Molecular Imprinted Polyacrylamide-Graphite Nanocomposite Electrode. Sensors Actuators B: Chem. 246, 840–847. doi:10.1016/j.snb.2017.02.139
Nandy Chatterjee, T., Das, D., Banerjee Roy, R., Tudu, B., Hazarika, A. K., Sabhapondit, S., et al. (2019). Development of a Nickel Hydroxide Nanopetal Decorated Molecular Imprinted Polymer Based Electrode for Sensitive Detection of Epigallocatechin-3-Gallate in Green Tea. Sensors Actuators B: Chem. 283, 69–78. doi:10.1016/j.snb.2018.11.159
Narumi, K., Sonoda, J.-I., Shiotani, K., Shigeru, M., Shibata, M., Kawachi, A., et al. (2014). Simultaneous Detection of Green Tea Catechins and Gallic Acid in Human Serum after Ingestion of Green Tea Tablets Using Ion-Pair High-Performance Liquid Chromatography with Electrochemical Detection. J. Chromatogr. B 945–946, 147–153. doi:10.1016/j.jchromb.2013.11.007
Naveen, M. H., Gurudatt, N. G., and Shim, Y.-B. (2017). Applications of Conducting Polymer Composites to Electrochemical Sensors: A Review. Appl. Mater. Today 9, 419–433. doi:10.1016/j.apmt.2017.09.001
Ng, K.-W., Cao, Z.-J., Chen, H.-B., Zhao, Z.-Z., Zhu, L., and Yi, T. (2018). Oolong Tea: A Critical Review of Processing Methods, Chemical Composition, Health Effects, and Risk. Crit. Rev. Food Sci. Nutr. 58, 2957–2980. doi:10.1080/10408398.2017.1347556
Novak, I., Šeruga, M., and Komorsky-Lovrić, Š. (2010). Characterisation of Catechins in Green and Black Teas Using Square-Wave Voltammetry and RP-HPLC-ECD. Food Chem. 122, 1283–1289. doi:10.1016/j.foodchem.2010.03.084
Novak, I., Šeruga, M., and Komorsky-Lovrić, Š. (2010). Characterisation of Catechins in Green and Black Teas Using Square-Wave Voltammetry and RP-HPLC-ECD. Food Chem. 122, 1283–1289. doi:10.1016/j.foodchem.2010.03.084
Novak, I., Šeruga, M., and Komorsky-Lovrić, Š. (2009). Square-Wave and Cyclic Voltammetry of Epicatechin Gallate on Glassy Carbon Electrode. J. Electroanalytical Chem. 631, 71–75. doi:10.1016/j.jelechem.2009.03.005
Nunes, E. W., Silva, M. K. L., and Cesarino, I. (2020). Evaluation of a Reduced Graphene Oxide-Sb Nanoparticles Electrochemical Sensor for the Detection of Cadmium and Lead in Chamomile Tea. Chemosensors 8. doi:10.3390/chemosensors8030053
Pal, A., and Das, C. (2020). A Novel Use of Solid Waste Extract from Tea Factory as Corrosion Inhibitor in Acidic Media on Boiler Quality Steel. Ind. Crops Prod. 151, 112468. doi:10.1016/j.indcrop.2020.112468
Pal, A., and Das, C. (2020). A Novel Use of Solid Waste Extract from Tea Factory as Corrosion Inhibitor in Acidic Media on Boiler Quality Steel. Ind. Crops Prod. 151, 112468. doi:10.1016/j.indcrop.2020.112468
Pang, J., Wu, X., Li, A., Liu, X., and Li, M. (2017). Detection of Catechin in Chinese Green Teas at N-Doped Carbon-Modified Electrode. Ionics 23, 1889–1895. doi:10.1007/s11581-017-2006-0
Power, A. C., Gorey, B., Chandra, S., and Chapman, J. (2018). Carbon Nanomaterials and Their Application to Electrochemical Sensors: A Review. Nanotechnology Rev. 7, 19–41. doi:10.1515/ntrev-2017-0160
Raluca-Ioana Stefan (2004). Semere Ghebru Bairu Diamond Paste Based Electrodes for the Determination of Pb(II) at Trace Concentration Levels. Talanta 63, 605–608. doi:10.1016/j.talanta.2003.12.023
Ranga, R., Kumar, A., Kumari, P., Singh, P., Madaan, V., and Kumar, K. (2021). Ferrite Application as an Electrochemical Sensor: A Review. Mater. Characterization, 111269. doi:10.1016/j.matchar.2021.111269
Rauf, A., and Mahdi, E. (2012). Evaluating Corrosion Inhibitors with the Help of Electrochemical Measurements Including Electrochemical Frequency Modulation. Int. J. Electrochem. Sci. 7, 4673–4685.
Ravishankar, T. N., Suresh Kumar, K., Teixeira, S. R., Fernandez, C., and Ramakrishnappa, T. (2016). Ag Doped Titanium Dioxide Nanocomposite-Modified Glassy Carbon Electrode as Electrochemical Interface for Catechol Sensing. Electroanalysis 28, 452–461. doi:10.1002/elan.201500238
Sakthinathan, S., Kubendhiran, S., and Chen, S.-M. (2017). Hydrothermal Synthesis of Three Dimensional Graphene-Multiwalled Carbon Nanotube Nanocomposite for Enhanced Electro Catalytic Oxidation of Caffeic Acid. Electroanalysis 29, 1103–1112. doi:10.1002/elan.201600687
Sandeep, S., Santhosh, A. S., Swamy, N. K., Suresh, G. S., Melo, J. S., and Chamaraja, N. A. (2018). A Biosensor Based on a Graphene Nanoribbon/Silver Nanoparticle/Polyphenol Oxidase Composite Matrix on a Graphite Electrode: Application in the Analysis of Catechol in Green Tea Samples. New J. Chem. 42, 16620–16629. doi:10.1039/C8NJ02325E
Sanjay, B. P., Kumara Swamy, N., Yashas, S. R., and Sandeep, S. (2021). Design of an Electrochemical Sensor Using 2D Sheet-like Cu@g-C3n4 Transducer Matrix for Electroanalysis of Catechol. J. Electrochem. Soc. 168, 076511. doi:10.1149/1945-7111/ac1495
Saritha, D., Gupta, V. K., Reddy, A. V. B., Agarwal, S., Moniruzzaman, M., Anitha, K., et al. (2019). Development of a Simple, Selective, Stable and Ultrasensitive Poly (Safranine/Nano NiO) Modified Carbon Paste Electrode for Selective Detection of Rutin in Buckwheat and Green Tea Samples. Int. J. Electrochem. Sci. 14, 10093–10110. doi:10.20964/2019.11.48
Sen, S., Chattopadhyay, S., and Sarkar, P. (2015). Electrochemical Sensing of Tea Polyphenols by Chloramine-T Modified Electrodes: A New Approach. J. Electrochem. Soc. 163, B49–B55. doi:10.1149/2.0491603jes
Şenocak, A., Basova, T., Demirbas, E., and Durmuş, M. (2019). Direct and Fast Electrochemical Determination of Catechin in Tea Extracts Using SWCNT-Subphthalocyanine Hybrid Material. Electroanalysis 31, 1697–1707. doi:10.1002/elan.201900214
Šeruga, M., Novak, I., and Jakobek, L. (2011). Determination of Polyphenols Content and Antioxidant Activity of Some Red Wines by Differential Pulse Voltammetry, HPLC and Spectrophotometric Methods. Food Chem. 124, 1208–1216. doi:10.1016/j.foodchem.2010.07.047
Seth, R., Bhandawat, A., Parmar, R., Singh, P., Kumar, S., and Sharma, R. K. (2019). Global Transcriptional Insights of Pollen-Pistil Interactions Commencing Self-Incompatibility and Fertilization in Tea [Camellia Sinensis (L.) O. Kuntze]. Int. J. Mol. Sci. 20, 539. doi:10.3390/ijms20030539
Shao, X., Lv, L., Parks, T., Wu, H., Ho, C.-T., and Sang, S. (2010). Quantitative Analysis of Ginger Components in Commercial Products Using Liquid Chromatography with Electrochemical Array Detection. J. Agric. Food Chem. 58, 12608–12614. doi:10.1021/jf1029256
Shi, H., Chen, F., Zhao, S., Ye, C., Lin, C.-T., Zhu, J., et al. (2021). Preparation of Cassava Fiber-Iron Nanoparticles Composite for Electrochemical Determination of Tea Polyphenol. J. Food Meas. Characterization 15, 4711–4717. doi:10.1007/s11694-021-01030-5
Šimková, D., and Labuda, J. “Electrochemical Flow-Through System with DNA Biosensor for Biomedical and Food Technology Applications,” in Proceedings of the 2009 2nd International Symposium on Applied Sciences in Biomedical and Communication Technologies, November 24 2009, 181–186. doi:10.1109/isabel.2009.5373651
Soussou, A., Gammoudi, I., Moroté, F., Kalboussi, A., Cohen-Bouhacina, T., Grauby-Heywang, C., et al. (2017). Efficient Immobilization of Tyrosinase Enzyme on Layered Double Hydroxide Hybrid Nanomaterials for Electrochemical Detection of Polyphenols. IEEE Sensors J. 17, 4340–4348. doi:10.1109/jsen.2017.2709342
Su, Y.-L., and Cheng, S.-H. (2015). Sensitive and Selective Determination of Gallic Acid in Green Tea Samples Based on an Electrochemical Platform of Poly(Melamine) Film. Analytica Chim. Acta 901, 41–50. doi:10.1016/j.aca.2015.10.026
Tang, J., Wang, H., Jiang, X., Zhu, Z., Xie, J., Tang, J., et al. (2018). Electrochemical Behavior of Jasmine Tea Extract as Corrosion Inhibitor for Carbon Steel in Hydrochloric Acid Solution. Int. J. Electrochem. Sci. 13, 3625–3642. doi:10.20964/2018.04.41
Tanui, J. K., Fang, W., Feng, W., Zhuang, P., and Li, X. (2012). World Black Tea Markets: Relationships and Implications for the Global Tea Industry. J. Int. Food Agribusiness Marketing 24, 148–168. doi:10.1080/08974438.2012.665791
Tashkhourian, J., and Ghaderizadeh, S. M. (2014). SiO2-Modified Carbon Paste Electrode for Electrochemical Determination of Pyrogallol. Russ. J. Electrochemistry 50, 959–966. doi:10.1134/S1023193514100139
Tian, L., Wang, B., Chen, R., Gao, Y., Chen, Y., and Li, T. (2015). Determination of Quercetin Using a Photo-Electrochemical Sensor Modified with Titanium Dioxide and a Platinum(II)-Porphyrin Complex. Microchimica Acta 182, 687–693. doi:10.1007/s00604-014-1374-7
Uliana, C. V., Garbellini, G. S., and Yamanaka, H. (2014). Electrochemical Investigations on the Capacity of Flavonoids to Protect DNA against Damage Caused by Textile Disperse Dyes. Sensors Actuators B: Chem. 192, 188–195. doi:10.1016/j.snb.2013.10.091
Vellaichamy, B., Ponniah, S. K., and Prakash, P. (2017). An In-Situ Synthesis of Novel Au@NG-PPy Nanocomposite for Enhanced Electrocatalytic Activity toward Selective and Sensitive Sensing of Catechol in Natural Samples. Sensors Actuators B: Chem. 253, 392–399. doi:10.1016/j.snb.2017.06.147
Venkadesh, A., Mathiyarasu, J., and Radhakrishnan, S. (2021). Voltammetric Sensing of Caffeine in Food Sample Using Cu‐MOF and Graphene. Electroanalysis 33, 1007–1013. doi:10.1002/elan.202060488
Wambu, E. W., Fu, H., and Ho, Y. (2017). Characteristics and Trends in Global Tea Research: A Science Citation Index Expanded‐based Analysis. Int. J. Food Sci. Tech. 52, 644–651. doi:10.1111/ijfs.13317
Wang, G., He, X., Zhou, F., Li, Z., Fang, B., Zhang, X., et al. (2012). Application of Gold Nanoparticles/TiO2 Modified Electrode for the Electrooxidative Determination of Catechol in Tea Samples. Food Chem. 135, 446–451. doi:10.1016/j.foodchem.2012.04.139
Wang, H.-B., Zhang, H.-D., Zhang, Y.-H., Chen, H., Xu, L.-L., Huang, K.-J., et al. (2015). Tungsten Disulfide Nano-Flowers/Silver Nanoparticles Composites Based Electrochemical Sensor for Theophylline Determination. J. Electrochem. Soc. 162, B173–B179. doi:10.1149/2.0941507jes
Wang, H., Yuan, X., Zeng, G., Wu, Y., Liu, Y., Jiang, Q., et al. (2015). Three Dimensional Graphene Based Materials: Synthesis and Applications from Energy Storage and Conversion to Electrochemical Sensor and Environmental Remediation. Adv. Colloid Interf. Sci. 221, 41–59. doi:10.1016/j.cis.2015.04.005
Wang, J., Mu, J., Ma, J., Yang, Y., Wang, M., Zhu, L., et al. (2012). Determination of Rutin and Puerarin in Teas and Pharmaceutical Preparations Using Poly (Evans Blue) Film-Modified Electrodes. J. Food Drug Anal. 20, 611–616.
Wang, J., Zareef, M., He, P., Sun, H., Chen, Q., Li, H., et al. (2019). Evaluation of Matcha Tea Quality Index Using Portable NIR Spectroscopy Coupled with Chemometric Algorithms. J. Sci. Food Agric. 99, 5019–5027. doi:10.1002/jsfa.9743
Wang, X.-G., Li, J., and Fan, Y.-J. (2010). Fast Detection of Catechin in Tea Beverage Using a Poly-Aspartic Acid Film Based Sensor. Microchimica Acta 169, 173–179. doi:10.1007/s00604-010-0335-z
Wang, Y., Wei, X., Wang, F., and Li, M. (2014). Sensitive Voltammetric Detection of Caffeine in Tea and Other Beverages Based on a DNA-Functionalized Single-Walled Carbon Nanotube Modified Glassy Carbon Electrode. Anal. Methods 6, 7525–7531. doi:10.1039/c4ay00837e
Wang, Y., Yao, Z., Pan, Z., Wang, R., Yan, G., Liu, C., et al. (2020). Tea-planted Soils as Global Hotspots for N2O Emissions from Croplands. Environ. Res. Lett. 15, 104018. doi:10.1088/1748-9326/aba5b2
Wang, Y., Zhong, J., Ding, F., Zhao, Q., Zhang, Z., Liu, X., et al. (2018). A Bifunctional NiCo2S4/Reduced Graphene Oxide@polyaniline Nanocomposite as a Highly-Efficient Electrode for Glucose and Rutin Detection. New J. Chem. 42, 9398–9409. doi:10.1039/C8NJ00663F
Wei, C., Yang, H., Wang, S., Zhao, J., Liu, C., Gao, L., et al. (2018). Draft Genome Sequence of Camellia Sinensis Var. Sinensis Provides Insights into the Evolution of the Tea Genome and Tea Quality. Proc. Natl. Acad. Sci. 115, E4151–E4158. doi:10.1073/pnas.1719622115
Wu, Y., Kou, J., Wang, L., Cheng, L., and Lu, K. (2019). Langmuir-Blodgett Films of Nafion-Nitrogen Doped Carbon Nanotubes as New Sensing Materials for the Determination of Caffeine in Tea. Int. J. Electrochem. Sci. 14, 11166–11177. doi:10.20964/2019.12.50
Xie, Y., Zhang, T., Chen, Y., Wang, Y., and Wang, L. (2020). Fabrication of Core-Shell Magnetic Covalent Organic Frameworks Composites and Their Application for Highly Sensitive Detection of Luteolin. Talanta 213, 120843. doi:10.1016/j.talanta.2020.120843
Xu, M., Wang, J., and Gu, S. (2019). Rapid Identification of Tea Quality by E-Nose and Computer Vision Combining with a Synergetic Data Fusion Strategy. J. Food Eng. 241, 10–17. doi:10.1016/j.jfoodeng.2018.07.020
Xu, M., Wang, J., and Zhu, L. (2019). The Qualitative and Quantitative Assessment of Tea Quality Based on E-Nose, E-Tongue and E-Eye Combined with Chemometrics. Food Chem. 289, 482–489. doi:10.1016/j.foodchem.2019.03.080
Yang, B., Kotani, A., Arai, K., and Kusu, F. (2001). Relationship of Electrochemical Oxidation of Catechins on Their Antioxidant Activity in Microsomal Lipid Peroxidation. Chem. Pharm. Bull. 49, 747–751. doi:10.1248/cpb.49.747
Yang, J., Deng, S., Lei, J., Ju, H., and Gunasekaran, S. (2011). Electrochemical Synthesis of Reduced Graphene Sheet–AuPd Alloy Nanoparticle Composites for Enzymatic Biosensing. Biosens. Bioelectron. 29, 159–166. doi:10.1016/j.bios.2011.08.011
Yang, L., Yang, J., Xu, B., Zhao, F., and Zeng, B. (2016). Facile Preparation of Molecularly Imprinted Polypyrrole-Graphene-Multiwalled Carbon Nanotubes Composite Film Modified Electrode for Rutin Sensing. Talanta 161, 413–418. doi:10.1016/j.talanta.2016.08.080
Yang, Y. J., and Li, W. (2015). High Sensitive Determination of Theophylline Based on Manganese Oxide Nanoparticles/Multiwalled Carbon Nanotube Nanocomposite Modified Electrode. Ionics 21, 1121–1128. doi:10.1007/s11581-014-1264-3
Yao, Y., Zhang, L., Wen, Y., Wang, Z., Zhang, H., Hu, D., et al. (2015). Voltammetric Determination of Catechin Using Single-Walled Carbon Nanotubes/Poly(Hydroxymethylated-3,4-Ethylenedioxythiophene) Composite Modified Electrode. Ionics 21, 2927–2936. doi:10.1007/s11581-015-1494-z
Yi, J., Liu, Z., Liu, J., Liu, H., Xia, F., Tian, D., et al. (2020). A Label-free Electrochemical Aptasensor Based on 3D Porous CS/RGO/GCE for Acetamiprid Residue Detection. Biosens. Bioelectron. 148, 111827. doi:10.1016/j.bios.2019.111827
Yin, C., Zhuang, Q., Xiao, Q., Wang, Y., and Xie, J. (2021). Electropolymerization of Poly(Methylene Blue) on Flower-like Nickel-Based MOFs Used for Ratiometric Electrochemical Sensing of Total Polyphenolic Content in Chrysanthemum Tea. Anal. Methods 13, 1154–1163. doi:10.1039/D1AY00028D
Yin, H., Meng, X., Su, H., Xu, M., and Ai, S. (2012). Electrochemical Determination of Theophylline in Foodstuff, Tea and Soft Drinks Based on Urchin-like CdSe Microparticles Modified Glassy Carbon Electrode. Food Chem. 134, 1225–1230. doi:10.1016/j.foodchem.2012.02.197
Ying, J., Zheng, Y., Zhang, H., and Fu, L. (2020). Room Temperature Biosynthesis of Gold Nanoparticles with Lycoris Aurea Leaf Extract for the Electrochemical Determination of Aspirin. Revista Mexicana de Ingeniería Química 19, 585–592. doi:10.24275/rmiq/mat741
Yue, X., Pang, S., Han, P., Zhang, C., Wang, J., and Zhang, L. (2013). Carbon Nanotubes/Carbon Paper Composite Electrode for Sensitive Detection of Catechol in the Presence of Hydroquinone. Electrochemistry Commun. 34, 356–359. doi:10.1016/j.elecom.2013.07.016
Zeng, Q., Chen, J., Gao, F., Tu, X., Qian, Y., Yu, Y., et al. (2021). Development of a New Electrochemical Sensing Platform Based on MoO3-Polypyrrole Nanowires/MWCNTs Composite and its Application to Luteolin Detection. Synth. Met. 271, 116620. doi:10.1016/j.synthmet.2020.116620
Zhang, G., Fu, H., Zou, D., Xiao, R., Liu, J., and Li, S. (2017). Electrochemical Determination of Caffeine in Tea Using a Polydopamine-Gold Nanocomposite. Int. J. Electrochem. Sci. 12, 11465–11472. doi:10.20964/2017.12.76
Zhang, H., Wu, S., Xing, Z., Wang, H.-B., and Liu, Y.-M. (2021). A Highly Sensitive Electrochemical Sensor for Theophylline Based on Dopamine-Melanin Nanosphere (DMN)–Gold Nanoparticles (AuNPs)-Modified Electrode. Appl. Phys. A 127, 844. doi:10.1007/s00339-021-04968-x
Zhang, M., Pan, B., Wang, Y., Du, X., Fu, L., Zheng, Y., et al. (2020). Recording the Electrochemical Profile of Pueraria Leaves for Polyphyly Analysis. ChemistrySelect 5, 5035–5040. doi:10.1002/slct.202001100
Zhang, Y., Shang, J., Jiang, B., Zhou, X., and Wang, J. (2017). Electrochemical Determination of Caffeine in Oolong Tea Based on Polyelectrolyte Functionalized Multi-Walled Carbon Nanotube. Int. J. Electrochem. Sci. 12, 2552–2562. doi:10.20964/2017.03.02
Zhao, H., Ran, Q., Li, Y., Li, B., Liu, B., Ma, H., et al. (2020). Highly Sensitive Detection of Gallic Acid Based on 3D Interconnected Porous Carbon Nanotubes/Carbon Nanosheets Modified Glassy Carbon Electrode. J. Mater. Res. Tech. 9, 9422–9433. doi:10.1016/j.jmrt.2020.05.102
Zheng, C., Wang, Y., Ding, Z., and Zhao, L. (2016). Global Transcriptional Analysis Reveals the Complex Relationship between Tea Quality, Leaf Senescence and the Responses to Cold-Drought Combined Stress in Camellia Sinensis. Front. Plant Sci. 7, 1858. doi:10.3389/fpls.2016.01858
Zhi, R., Zhao, L., and Zhang, D. (2017). A Framework for the Multi-Level Fusion of Electronic Nose and Electronic Tongue for Tea Quality Assessment. Sensors 17, 1007. doi:10.3390/s17051007
Zhong, T., Guo, Q., Yin, Z., Zhu, X., Liu, R., Liu, A., et al. (2019). Polyphenol Oxidase/Gold Nanoparticles/Mesoporous Carbon-Modified Electrode as an Electrochemical Sensing Platform for Rutin in Dark Teas. RSC Adv. 9, 2152–2155. doi:10.1039/C8RA08199A
Zhou, J., Zheng, Y., Zhang, J., Karimi-Maleh, H., Xu, Y., Zhou, Q., et al. (2020). Characterization of the Electrochemical Profiles of Lycoris Seeds for Species Identification and Infrageneric Relationships. Anal. Lett. 53, 2517–2528. doi:10.1080/00032719.2020.1746327
Zhu, X., Liu, P., Ge, Y., Wu, R., Xue, T., Sheng, Y., et al. (2020). MoS2/MWCNTs Porous Nanohybrid Network with Oxidase-like Characteristic as Electrochemical Nanozyme Sensor Coupled with Machine Learning for Intelligent Analysis of Carbendazim. J. Electroanalytical Chem. 862, 113940. doi:10.1016/j.jelechem.2020.113940
Keywords: electrochemical sensor, tea, antioxidant, caffeic acid, gallic acid, tea polyphenols, analytical chemistry
Citation: Shao J, Wang C, Shen Y, Shi J and Ding D (2022) Electrochemical Sensors and Biosensors for the Analysis of Tea Components: A Bibliometric Review. Front. Chem. 9:818461. doi: 10.3389/fchem.2021.818461
Received: 19 November 2021; Accepted: 28 December 2021;
Published: 14 January 2022.
Edited by:
Federico Marini, Sapienza University of Rome, ItalyReviewed by:
Masoumeh Ghalkhani, Shahid Rajaee Teacher Training University, IranMani Govindasamy, National Taipei University of Technology, Taiwan
Copyright © 2022 Shao, Wang, Shen, Shi and Ding. This is an open-access article distributed under the terms of the Creative Commons Attribution License (CC BY). The use, distribution or reproduction in other forums is permitted, provided the original author(s) and the copyright owner(s) are credited and that the original publication in this journal is cited, in accordance with accepted academic practice. No use, distribution or reproduction is permitted which does not comply with these terms.
*Correspondence: Jinhua Shao, aG5ranh5c2poQGh1c2UuZWR1LmNu