- 1State Key Laboratory of Natural and Biomimetic Drugs, School of Pharmaceutical Sciences, Peking University, Beijing, China
- 2State Key Laboratory of Pharmaceutical Biotechnology, School of Life Sciences, Nanjing University, Nanjing, China
Herein, the convenient one-step electrochemical bromination of glycals using Bu4NBr as the brominating source under metal-catalyst-free and oxidant-free reaction conditions was described. A series of 2-bromoglycals bearing different electron-withdrawing or electron-donating protective groups were successfully synthesized in moderate to excellent yields. The coupling of tri-O-benzyl-2-bromogalactal with phenylacetylene, potassium phenyltrifluoroborate, or a 6-OH acceptor was achieved to afford 2C-branched carbohydrates and disaccharides via Sonogashira coupling, Suzuki coupling, and Ferrier rearrangement reactions with high efficiency. The radical trapping and cyclic voltammetry experiments indicated that bromine radicals may be involved in the reaction process.
Introduction
Carbohydrates mainly exist in the form of glycoconjugates, polysaccharides, oligosaccharides, and monosaccharides and play a pivotal role in a broad range of important biological processes including cell proliferation, host–pathogen interactions, cell adhesion, hormone function, and the immune response (Kiessling and Kraft, 2013; Wang et al., 2020). Chemical synthesis can afford both naturally occurring important carbohydrates and biologically active carbohydrate mimetics in sufficient quantities, providing a powerful tool to understand the biological functions of carbohydrates (Muthana et al., 2009; Panza et al., 2018; Li and Ye, 2020).
Organic electrosynthesis is of current interest as one of the most promising methods for the efficient, sustainable, and green synthesis of medicinally significant compounds (Francke and Little, 2014; Horn et al., 2016; Liang and Zeng, 2020; Meyer et al., 2020; Yuan et al., 2021). In recent years, the electrochemical synthesis of oligosaccharides has been successfully demonstrated through the activation of different types of glycosyl donors, such as thio-, seleno-, and telluro-glycosides (Nokami et al., 2015; Manmode et al., 2018; Zhang et al., 2020). In addition, our group has been involved in the electrochemical transformation of glycals to obtain significant synthetic carbohydrate compounds (Liu et al., 2020; Liu et al., 2021). Along with the use of MnBr2 as the redox mediator, the electrochemical trifluoromethylation of glycals has been realized (Liu et al., 2021). In the exploration of this reaction, we found that 2-bromoglycals could also be isolated when the equivalent of Bu4NBr was added. Inspired by this surprising result, we turned our focus to the electrochemical bromination of glycals (Scheme 1).
Over the past few decades, 2-bromoglycals have been widely employed as important synthons in combination with metal-catalyzed cross-coupling reactions to access 2C-branched carbohydrates and their analogs (Leibeling et al., 2010a; Leibeling et al., 2010b; Leibeling and Werz, 2012; Dharuman and Vankar, 2014; Martin et al., 2015). Due to the importance of 2-bromoglycals, the development of a novel, practical, and environmentally friendly method for the synthesis of 2-bromoglycals is still of high interest. The most common way to obtain 2-bromoglycals consists of two steps using Br2, which is toxic and unstable, as the brominating source (Leibeling et al., 2010a). An alternative approach involves the one-step synthesis of 2-bromoglycals from glycals using N-bromosuccinimide and silver nitrate (Dharuman and Vankar, 2014). We herein report a one-step electrochemical bromination of glycals using commercially available, stable, and safe Bu4NBr as the bromine source in an undivided cell under metal-catalyst-free and oxidant-free reaction conditions.
Results and Discussion
Initially, we began our investigation with 6-O-benzyl-3,4-dideoxy-glycal 1a as the model substrate for electrochemical bromination using Pt as the anode and cathode in an undivided cell. Unfortunately, no desired 2-bromo-3,4-dideoxy-glycal 3a was detected using KBr (Ye and Shreeve, 2004; Zhao and Lu, 2018) or NaBr (Alberto et al., 2014) as the “Br” reagent (Table 1, entries 1 and 2). To our delight, the product 3a could be obtained in 10% isolated yield when 1a was treated with 1.5 equiv of Bu4NBr (Yoshimitsu et al., 2009; Kamon et al., 2012) in dry CH3CN at room temperature under a constant electric current of 2 mA (Table 1, entry 3). The yield was increased slightly when the reaction temperature was increased to 50°C (Table 1, entry 4). Further raising the temperature to 75°C was beneficial to this transformation, leading to the formation of 3a in 35% yield (Table 1, entry 5), and the yield could be increased to 40% when the amount of Bu4NBr was increased to 2.0 equiv (Table 1, entry 6). It was found that the addition of base, such as K2CO3 or Na2CO3, could further improve the reaction yield (Table 1, entries 7 and 8). Surprisingly, when the bromination reaction was conducted with NaSO2CF3 as the additive, the desired product 3a was isolated in 67% yield (Table 1, entry 9). Altering the amount of NaSO2CF3 to 2.0 equiv led to an increased yield of 82% (Table 1, entry 10). Comparatively, when other solvents such as CH3CN/H2O (3:1), 1,2-dimethoxyethane, or ClCH2CH2Cl, were used instead of dry CH3CN, lower yields were achieved (Table 1, entries 11–13). Extensive screening experiments revealed that either changing electrode materials or modifying the reaction current were not effective for improving the yield of 3a (Table 1, entries 14–18). Moreover, the yield of 3a was decreased drastically when the electrochemical bromination reaction was performed in an air atmosphere (Table 1, entry 19). Finally, the control experiment confirmed that the role of electricity was essential, as the reaction could not proceed in the absence of an electric current (Table 1, entry 20).
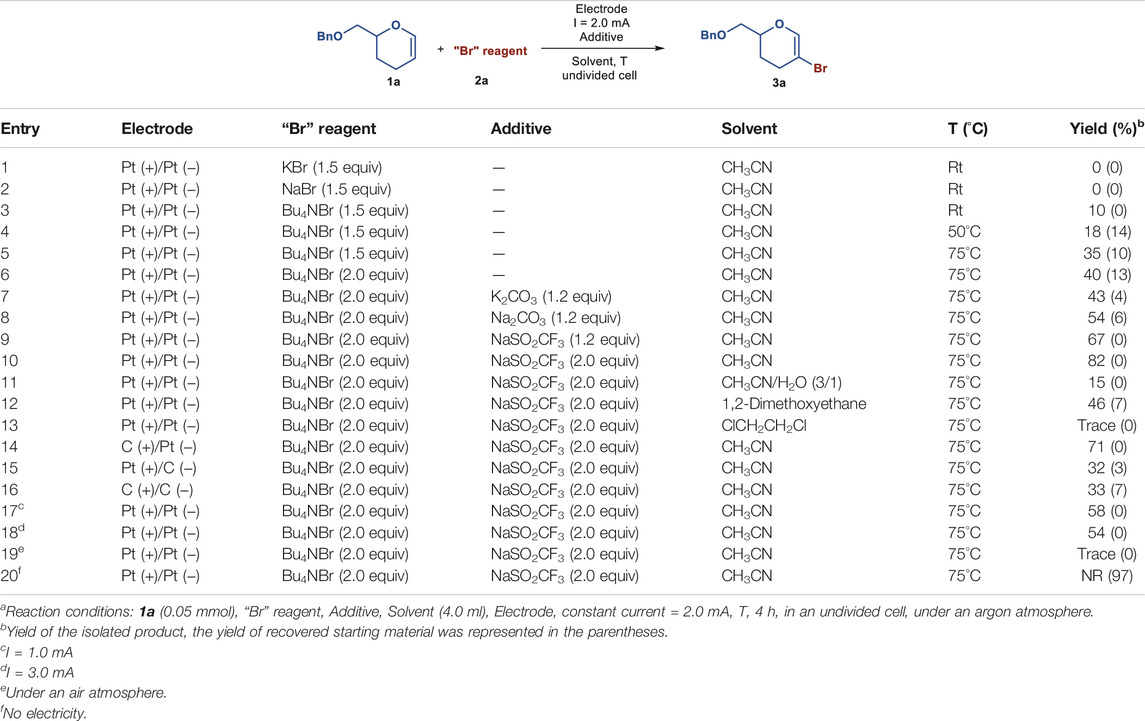
TABLE 1. Optimization of reaction conditionsa.
With the optimal reaction conditions in hand, we then evaluated the substrate scope of the electrochemical bromination of various types of glycals with Bu4NBr (Table 2). First, 3,4-dideoxy-glycals with electron-withdrawing groups were examined. Substrates with an acetyl or benzoyl group provided the respective brominated products 3b and 3c in good yields. In addition, benzyl (Bn), p-methoxybenzyl (PMB), tert-butyldimethylsilyl (TBS), and methyl (Me) substituted glucals could also be converted into the corresponding products 3d–g. Similarly, galactals bearing Bn, PMB, or TBS groups were found to be amenable to the electrochemical reaction, providing the desired products 3h–j in 56–73% yields. And the scalability of this electrochemical bromination was further showed by an efficient conversion of compound 1h on a 250 mg scale in 57% isolated yield. Peracetylated and perbenzoylated galactals could also undergo the electrochemical transformation to afford 2-bromo-galactals 3k–l, albeit in slightly low yields. Notably, bromination of peracetylated L-rhamnal proceeded smoothly to deliver compound 3m in 89% yield. Under the same conditions, the L-rhamnal, L-arabinal, and D-xylal equipped with Bn or PMB groups were also able to furnish the corresponding brominated products 3n–s in moderate to excellent yields. Furthermore, benzylated lactal 1t underwent this reaction to afford the desired product 3t in 72% yield.
To demonstrate the potential applicability of 2-bromoglycals, the reaction of 2-bromogalactal 3h with different substrates was carried out (Scheme 2). First, we explored the utility of 2-bromogalactal 3h in the synthesis of 2C-substituted carbohydrates, which exist in many natural products (Yin and Linker, 2012; Dubbu et al., 2018; Darbem et al., 2020). Compound 3h reacted with phenylacetylene 4a in the presence of Pd(PPh3)2Cl2, CuI and Et3N to afford the coupled product 5a in 80% yield (Koester and Werz, 2012). The reaction of 3h with potassium phenyltrifluoroborate 4b also proceeded smoothly to provide the corresponding product 5b in 79% yield (Molander and Fumagalli, 2006). Moreover, disaccharide 5c was successfully synthesized in the promotion of BF3·Et2O in 72% yield with excellent α-selectivity via the Ferrier rearrangement reaction (Dharuman et al., 2013; Wang et al., 2019).
To gain insight into the mechanism of this electrochemical bromination, radical trapping experiments were performed. As expected, the reaction was completely shut down when 3.0 equiv of the radical scavenger 2,2,6,6-tetramethylpiperidine-1-oxyl (TEMPO) was added under the standard reaction conditions, indicating that radical chemistry was likely involved in the reaction (Scheme 3A) (Makai et al., 2020; Wang et al., 2019). Furthermore, another experiment using 1,1-diphenylethylene (6) was also conducted under the standard reaction conditions, and (2-bromoethene-1,1-diyl)dibenzene (7) was successfully detected in the GC–MS, confirming the participation of the bromine radical in the reaction system (Scheme 3B) (Chen et al., 2020; Kale et al., 2021). We observed that the reaction mixture gradually turned brown during the reaction process, indicating that Br2 might be generated. The 2-bromoglycal 3h was resubmitted to the standard reaction conditions for 20 h with the recovery of 3h in a 78% yield. In addition, cyclic voltammetry experiments were carried out to investigate the redox behavior of the reaction. The cyclic voltammetry measurements of Bu4NBr indicated two obvious oxidative peaks at 1.07 and 1.37 V (vs. Ag/AgCl) (Figure 1, red curve), which likely corresponded to Br3–/Br– and Br3–/Br2 redox couples, respectively (Damljanovic et al., 2011; Bennett et al., 2016; Kang et al., 2016). The first oxidative peak was associated with the oxidation of Br– to the bromine radical. The bromine radical then integrated into Br2, which could combine with Br– to form Br3–. The second oxidative peak was attributed to the oxidation of Br3– to Br2. An increase in the oxidative peak current was observed when Bu4NBr and 1d were combined, which was attributed to a catalytic current, resulting from the chemical reaction of the electrochemically-generated Br2 and glucal 1d (Figure 1, blue curve).
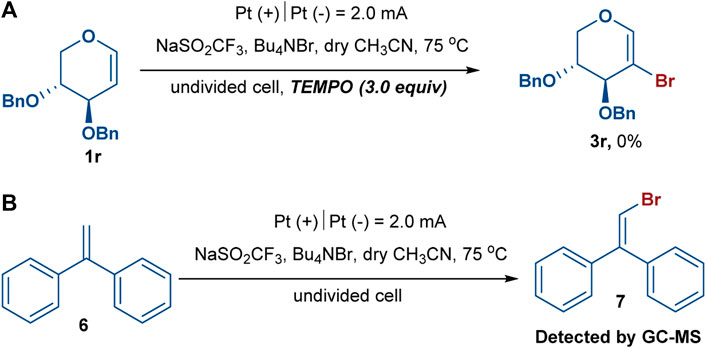
SCHEME 3. In the radical trapping experiments, experiment (A) was performed with glycal (0.05 mmol) and TEMPO (0.15 mmol, 3.0 equiv) under standard reaction condition: NaSO2CF3 (0.10 mmol, 2.0 equiv), Bu4NBr (0.10 mmol, 2.0 equiv), dry CH3CN (4.0 ml) in an undivided cell with Pt as the anode and cathode, constant current = 2.0 mA, 75 oC, under argon atmosphere, 4 h. Besides, experiment (B) was performed with 1,1-diphenylethylene (0.15 mmol) under standard reaction condition as well.
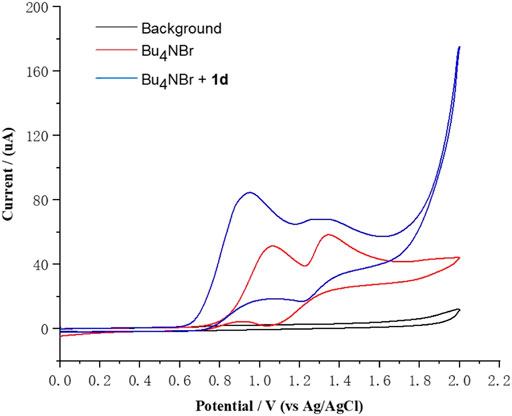
FIGURE 1. Cyclic voltammetry measurements of Bu4NBr and 1d. Conditions: glassy carbon disk electrode (diameter is 3.0 mm, PTFE shroud) as the working electrode, platinum wire as the counter electrode, Ag/AgCl electrode (3.5 M KCl solution) as the reference electrode, Bu4NOTf (0.10 M in MeCN), under an argon atmosphere, cyclic voltammogram at 0.05 V s−1 with Bu4NBr (5 mM) or Bu4NBr (5 mM) and 1d (5 mM).
Mechanism
Based on the above results and previous reports (Yuan et al., 2019; Gou et al., 2021; Wu et al., 2021), a plausible reaction mechanism for the electrochemical bromination of glycals was depicted in Scheme 4. A bromine anion was oxidized to the bromine radical on the anode and subsequently molecular Br2. This was then attacked by glycal to yield the intermediate I. Finally, the brominated product was obtained by the deprotonation of II, which would be stabilized by acetonitrile. NaSO2CF3 might be used as the electrolyte to increase the conductivity of the reaction solution and a proton scavenger to generate strong acid for cathode reduction; besides, it may be an anion to stabilize the glycosyl cation. At the same time, H+ was reduced to produce hydrogen on the cathode.
Conclusion
In summary, we performed the one-step electrochemical bromination of various glycals with electron-withdrawing and electron-donating protective groups using commercially available, nontoxic Bu4NBr as the brominating source under metal-catalyst-free and oxidant-free reaction conditions. The synthetic applicability of 2-bromoglycals has been demonstrated by providing the corresponding 2C-substituted carbohydrates and disaccharides via palladium-catalyzed cross-coupling reactions and the Ferrier rearrangement reaction. The readily available substrates and ease of handling make this methodology a practical tool to access diversified brominating synthons for the preparation of biologically relevant carbohydrates.
Data Availability Statement
The original contributions presented in the study are included in the article/Supplementary Material, further inquiries can be directed to the corresponding authors.
Author Contributions
Z-XL and ML contributed equally to this work. Z-XL, ML, and TL performed the experiments. Z-XL and ML carried out the mechanistic studies. Z-XL, ML, D-CX, and X-SY analyzed and discussed the research results. D-CX and X-SY designed the experiments. Z-XL, ML, and D-CX wrote the manuscript.
Funding
This research was funded by grants from the National Natural Science Foundation of China (Grant Nos. 21772006, 91853122), the Beijing Outstanding Young Scientist Program (BJJWZYJH01201910001001), the open fund of State Key Laboratory of Pharmaceutical Biotechnology, Nanjing University, China (Grant no. KF-GN-202106), and the Fundamental Research Funds for the Central Universities.
Conflict of Interest
The authors declare that the research was conducted in the absence of any commercial or financial relationships that could be construed as a potential conflict of interest.
Publisher’s Note
All claims expressed in this article are solely those of the authors and do not necessarily represent those of their affiliated organizations, or those of the publisher, the editors and the reviewers. Any product that may be evaluated in this article, or claim that may be made by its manufacturer, is not guaranteed or endorsed by the publisher.
Supplementary Material
The Supplementary Material for this article can be found online at: https://www.frontiersin.org/articles/10.3389/fchem.2021.796690/full#supplementary-material
References
Alberto, E. E., Muller, L. M., and Detty, M. R. (2014). Rate Accelerations of Bromination Reactions with NaBr and H2O2 via the Addition of Catalytic Quantities of Diaryl Ditellurides. Organometallics 33, 5571–5581. doi:10.1021/om500883f
Bennett, B., Chang, J., and Bard, A. J. (2016). Mechanism of the Br −/Br2 Redox Reaction on Platinum and Glassy Carbon Electrodes in Nitrobenzene by Cyclic Voltammetry. Electrochimica Acta 219, 1–9. doi:10.1016/j.electacta.2016.09.129
Chen, D., Li, J., Cui, P., Shan, Y., Zhao, Y., and Qiu, G. (2020). Tandem Oxidative Radical Halogenated Addition of Alkynyl Imines: Regioselective Synthesis of 3-Haloquinolines. Eur. J. Org. Chem. 2020, 169–175. doi:10.1002/ejoc.201901395
Damljanović, I., Stevanović, D., Vukićević, M., and Vukićević, R. D. (2011). Electrochemical Bromochlorination of Peracetylated Glycals. Carbohydr. Res. 346, 2683–2687. doi:10.1016/j.carres.2011.09.016
Darbem, M. P., Esteves, H. A., Oliveira, I. M., and Stefani, H. A. (2020). α,β‐Unsaturated 2‐Ketoglycosides via Pd‐Catalyzed Carbonylative Heck Reaction of 2‐Iodoglycals. Eur. J. Org. Chem. 2020, 5220–5226. doi:10.1002/ejoc.202000846
Dharuman, S., Gupta, P., Kancharla, P. K., and Vankar, Y. D. (2013). Synthesis of 2-Nitroglycals from Glycals Using the Tetrabutylammonium Nitrate-Trifluoroacetic Anhydride-Triethylamine Reagent System and Base-Catalyzed Ferrier Rearrangement of Acetylated 2-Nitroglycals. J. Org. Chem. 78, 8442–8450. doi:10.1021/jo401165y
Dharuman, S., and Vankar, Y. D. (2014). N-Halosuccinimide/AgNO3-Efficient Reagent Systems for One-step Synthesis of 2-Haloglycals from Glycals: Application in the Synthesis of 2C-Branched Sugars via Heck Coupling Reactions. Org. Lett. 16, 1172–1175. doi:10.1021/ol500039s
Dubbu, S., Verma, A. K., Parasuraman, K., and Vankar, Y. D. (2018). Stereoselective Synthesis of 1,2-Annulated-C-Aryl Glycosides from Carbohydrate-Derived Terminally Unsubstituted Dienes and Arynes: Application towards Synthesis of Sugar-Fused- or Branched- Naphthalenes, and C-Aryl Glycosides. Carbohydr. Res. 465, 29–34. doi:10.1016/j.carres.2018.06.001
Francke, R., and Little, R. D. (2014). Redox Catalysis in Organic Electrosynthesis: Basic Principles and Recent Developments. Chem. Soc. Rev. 43, 2492–2521. doi:10.1039/c3cs60464k
Gou, X.-Y., Li, Y., Luan, Y.-Y., Shi, W.-Y., Wang, C.-T., An, Y., et al. (2021). Ruthenium-Catalyzed Radical Cyclization/meta-Selective C-H Alkylation of Arenes via σ-Activation Strategy. ACS Catal. 11, 4263–4270. doi:10.1021/acscatal.1c00359
Horn, E. J., Rosen, B. R., Chen, Y., Tang, J., Chen, K., Eastgate, M. D., et al. (2016). Scalable and Sustainable Electrochemical Allylic C-H Oxidation. Nature 533, 77–81. doi:10.1038/nature17431
Kamon, T., Shigeoka, D., Tanaka, T., and Yoshimitsu, T. (2012). Intramolecular Iron(II)-catalyzed Aminobromination of Allyl N-Tosyloxycarbamates. Org. Biomol. Chem. 10, 2363–2365. doi:10.1039/c2ob07190h
Kang, L.-S., Luo, M.-H., Lam, C. M., Hu, L.-M., Little, R. D., and Zeng, C.-C. (2016). Electrochemical C-H Functionalization and Subsequent C-S and C-N Bond Formation: Paired Electrosynthesis of 3-Amino-2-Thiocyanato-α,β-Unsaturated Carbonyl Derivatives Mediated by Bromide Ions. Green. Chem. 18, 3767–3774. doi:10.1039/c6gc00666c
Kiessling, L. L., and Kraft, M. B. (2013). A Path to Complex Carbohydrates. Science 341, 357–358. doi:10.1126/science.1241788
Koester, D. C., and Werz, D. B. (2012). Sonogashira-Hagihara Reactions of Halogenated Glycals. Beilstein J. Org. Chem. 8, 675–682. doi:10.3762/bjoc.8.75
Leibeling, M., Koester, D. C., Pawliczek, M., Kratzert, D., Dittrich, B., and Werz, D. B. (2010a). Hybrids of Sugars and Aromatics: A Pd-Catalyzed Modular Approach to Chromans and Isochromans. Bioorg. Med. Chem. 18, 3656–3667. doi:10.1016/j.bmc.2010.03.004
Leibeling, M., Koester, D. C., Pawliczek, M., Schild, S. C., and Werz, D. B. (2010b). Domino Access to Highly Substituted Chromans and Isochromans from Carbohydrates. Nat. Chem. Biol. 6, 199–201. doi:10.1038/nchembio.302
Leibeling, M., and Werz, D. B. (2012). Winding up Alkynes: A Pd-Catalyzed Tandem-Domino Reaction to Chiral Biphenyls. Chem. Eur. J. 18, 6138–6141. doi:10.1002/chem.201200175
Li, B.-H., and Ye, X.-S. (2020). Recent Advances in Glycan Synthesis. Curr. Opin. Chem. Biol. 58, 20–27. doi:10.1016/j.cbpa.2020.04.009
Liang, S., and Zeng, C.-C. (2020). Organic Electrochemistry: Anodic Construction of Heterocyclic Structures. Curr. Opin. Electrochemistry 24, 31–43. doi:10.1016/j.coelec.2020.06.005
Liu, M., Liu, K. M., Xiong, D. C., Zhang, H., Li, T., Li, B., et al. (2020). Stereoselective Electro‐2‐deoxyglycosylation from Glycals. Angew. Chem. Int. Ed. 59, 15204–15208. doi:10.1002/anie.202006115
Liu, M., Luo, Z.-X., Li, T., Xiong, D.-C., and Ye, X.-S. (2021). Electrochemical Trifluoromethylation of Glycals. J. Org. Chem. 86, 16187–16194. doi:10.1021/acs.joc.1c01318
Makai, S., Falk, E., and Morandi, B. (2020). Direct Synthesis of Unprotected 2-Azidoamines from Alkenes via an Iron-Catalyzed Difunctionalization Reaction. J. Am. Chem. Soc. 142, 21548–21555. doi:10.1021/jacs.0c11025
Manmode, S., Matsumoto, K., Nokami, T., and Itoh, T. (2018). Electrochemical Methods as Enabling Tools for Glycosylation. Asian J. Org. Chem. 7, 1719–1729. doi:10.1002/ajoc.201800302
Martin, A., Arda, A., Désiré, J., Martin-Mingot, A., Probst, N., Sinaÿ, P., et al. (2015). Catching Elusive Glycosyl Cations in a Condensed Phase with HF/SbF5 Superacid. Nat. Chem 8, 186–191. doi:10.1038/nchem.2399
Meyer, T. H., Choi, I., Tian, C., and Ackermann, L. (2020). Powering the Future: How Can Electrochemistry Make a Difference in Organic Synthesis? Chem 6, 2484–2496. doi:10.1016/j.chempr.2020.08.025
Molander, G. A., and Fumagalli, T. (2006). Palladium(0)-Catalyzed Suzuki−Miyaura Cross-Coupling Reactions of Potassium Aryl- and Heteroaryltrifluoroborates with Alkenyl Bromides. J. Org. Chem. 71, 5743–5747. doi:10.1021/jo0608366
Muthana, S., Cao, H., and Chen, X. (2009). Recent Progress in Chemical and Chemoenzymatic Synthesis of Carbohydrates. Curr. Opin. Chem. Biol. 13, 573–581. doi:10.1016/j.cbpa.2009.09.013
Nokami, T., Isoda, Y., Sasaki, N., Takaiso, A., Hayase, S., Itoh, T., et al. (2015). Automated Electrochemical Assembly of the Protected Potential TMG-Chitotriomycin Precursor Based on Rational Optimization of the Carbohydrate Building Block. Org. Lett. 17, 1525–1528. doi:10.1021/acs.orglett.5b00406
Panza, M., Pistorio, S. G., Stine, K. J., and Demchenko, A. V. (2018). Automated Chemical Oligosaccharide Synthesis: Novel Approach to Traditional Challenges. Chem. Rev. 118, 8105–8150. doi:10.1021/acs.chemrev.8b00051
Prabhakar Kale, A., Nikolaienko, P., Smirnova, K., and Rueping, M. (2021). Intramolecular Electrochemical Oxybromination of Olefins for the Synthesis of Isoxazolines in Batch and Continuous Flow. Eur. J. Org. Chem. 2021, 3496–3500. doi:10.1002/ejoc.202100640
Wang, J., Deng, C., Zhang, Q., and Chai, Y. (2019). Tuning the Chemoselectivity of Silyl Protected Rhamnals by Temperature and Brønsted Acidity: Kinetically Controlled 1,2-Addition vs Thermodynamically Controlled Ferrier Rearrangement. Org. Lett. 21, 1103–1107. doi:10.1021/acs.orglett.9b00009
Wang, Y., Carder, H. M., and Wendlandt, A. E. (2020). Synthesis of Rare Sugar Isomers through Site-Selective Epimerization. Nature 578, 403–408. doi:10.1038/s41586-020-1937-1
Wu, Y., Xu, S., Wang, H., Shao, D., Qi, Q., Lu, Y., et al. (2021). Directing Group Enables Electrochemical Selectively Meta-Bromination of Pyridines under Mild Conditions. J. Org. Chem. 86, 16144–16150. doi:10.1021/acs.joc.1c00923
Ye, C., and Shreeve, J. n. M. (2004). Structure-Dependent Oxidative Bromination of Unsaturated C−C Bonds Mediated by Selectfluor. J. Org. Chem. 69, 8561–8563. doi:10.1021/jo048383x
Yin, J., and Linker, T. (2012). Recent Advances in the Stereoselective Synthesis of Carbohydrate 2-C-Analogs. Org. Biomol. Chem. 10, 2351–2362. doi:10.1039/c2ob06529k
Yoshimitsu, T., Ino, T., Futamura, N., Kamon, T., and Tanaka, T. (2009). Total Synthesis of the β-Catenin Inhibitor, (−)-Agelastatin A: A Second-Generation Approach Based on Radical Aminobromination. Org. Lett. 11, 3402–3405. doi:10.1021/ol9012684
Yuan, Y., Yang, J., and Lei, A. (2021). Recent Advances in Electrochemical Oxidative Cross-Coupling with Hydrogen Evolution Involving Radicals. Chem. Soc. Rev. 50, 10058–10086. doi:10.1039/d1cs00150g
Yuan, Y., Yao, A., Zheng, Y., Gao, M., Zhou, Z., Qiao, J., et al. (2019). Electrochemical Oxidative Clean Halogenation Using HX/NaX with Hydrogen Evolution. Iscience 12, 293–303. doi:10.1016/j.isci.2019.01.017
Zhang, H. Y., Liu, M., Wu, X., Liu, M., Xiong, D. C., and Ye, X. S. (2020). Photo-/Electro-Driven Carbohydrate-Based Reactions. Prog. Chem. 32, 1804–1823. doi:10.7536/pc200608
Keywords: electrochemistry, bromination, glycals, 2-bromoglycals, cross-coupling, ferrier rearrangement
Citation: Luo Z-X, Liu M, Li T, Xiong D-C and Ye X-S (2021) Electrochemical Bromination of Glycals. Front. Chem. 9:796690. doi: 10.3389/fchem.2021.796690
Received: 18 October 2021; Accepted: 01 December 2021;
Published: 23 December 2021.
Edited by:
Jian Yin, Jiangnan University, ChinaReviewed by:
Bruno Linclau, University of Southampton, United KingdomXiaodong Shi, University of South Florida, United States
Copyright © 2021 Luo, Liu, Li, Xiong and Ye. This is an open-access article distributed under the terms of the Creative Commons Attribution License (CC BY). The use, distribution or reproduction in other forums is permitted, provided the original author(s) and the copyright owner(s) are credited and that the original publication in this journal is cited, in accordance with accepted academic practice. No use, distribution or reproduction is permitted which does not comply with these terms.
*Correspondence: De-Cai Xiong, ZGVjYWlAYmptdS5lZHUuY24=; Xin-Shan Ye, eGluc2hhbkBiam11LmVkdS5jbg==
†These authors have contributed equally to this work