- Higher Colleges of Technology-Dubai Women’s Campus-Health Sciences, Dhabi, United Arab Emirates
Extensive efforts have been made in the last decades to simplify the holistic sample preparation process. The idea of maximizing the extraction efficiency along with the reduction of extraction time, minimization/elimination of hazardous solvents, and miniaturization of the extraction device, eliminating sample pre- and posttreatment steps and reducing the sample volume requirement is always the goal for an analyst as it ensures the method’s congruency with the green analytical chemistry (GAC) principles and steps toward sustainability. In this context, the microextraction techniques such as solid-phase microextraction (SPME), stir bar sorptive extraction (SBSE), microextraction by packed sorbent (MEPS), fabric phase sorptive extraction (FPSE), in-tube extraction dynamic headspace (ITEX-DHS), and PAL SPME Arrow are being very active areas of research. To help transition into wider applications, the new solventless microextraction techniques have to be commercialized, automated, and validated, and their operating principles to be anchored to theory. In this work, the benefits and drawbacks of the advanced microextraction techniques will be discussed and compared, together with their applicability to the analysis of pharmaceuticals in different matrices.
Introduction
As green analysis is becoming more significant, reduction of solvent use and method miniaturization gain most relevance in pharmaceutical analysis (Mohamed, 2015). Sample preparation is considered the cornerstone step for the green analytical procedure. There are different ways for more environment-friendly sample preparation, which mainly includes excluding or at least minimizing the amounts of reagents and solvents in the analysis. Besides, a green solvent is used, rather than petrol-based ones. Integration of automation is a very valuable principle in greening your procedure, which can lead to miniaturization of the used method (Płotka-Wasylka et al., 2013). As a result, novel solid-phase microextraction techniques (SPME, SBSE, MEPS, FPSE, ITEX-DHS, and PAL SPME Arrow) have been lately explored (Spieteluna et al., 2013). All of these innovative solutions enhance and enable the direct injection of the analytes into the separation unit, and accordingly, they require fewer solvents, time, and labor work (Płotka-Wasylka et al., 2015).
The SPME technique has been widely used in various areas such as food analysis, environmental analysis, bioanalysis, drug monitoring, and toxicology (Moein et al., 2015). Pharmaceuticals and biological materials are complex and may contain acids, bases, salts, biomolecules, and other additives with comparable properties to the analytes of interest that areactive ingredients and metabolites acting as interfering compounds (Ebele et al., 2017). Generally, sampling and sample preparation steps make up more than 80% of the total time of analysis, and these are very crucial steps in determining the success of the analysis of the compounds of interest in complex matrices such as biological samples, for instance, plasma, urine, sputum, oral fluid, and whole blood (Abuzar et al., 2017) or water, soil, and food samples (Zambonin and Aresta, 2021). Thus, it is not amplification when saying that the choice of a proper sample preparation method significantly affects the reliability and accuracy of the analysis in addition to its greenness and sustainability profiles.
The aim of this review is to report and compare the advantages and drawbacks of the recent techniques and devices used for the extraction and procedures for pharmaceuticals analyses in complex matrices, with the main goal being the reduction of solvent consumption, analysis time, and sample manipulation, in accordance with green analytical chemistry (GAC) concepts.
Solid-Phase Microextraction
Solid-phase microextraction (SPME) presents a cornerstone for a new era of solventless extraction, miniaturization, and automation in pharmaceutical analysis. It assimilates sampling, extraction, and analyte pre-concentration into one single step, which results in low cost, reduction of labor, increased sensitivity, reduced carryover, and sample losses, and enhances the overall analytical process performance. Figure 1 shows the direct immersion (DI) and headspace (HS) SPME extraction and their direct coupling to the chromatographic system (Luo et al., 2011).
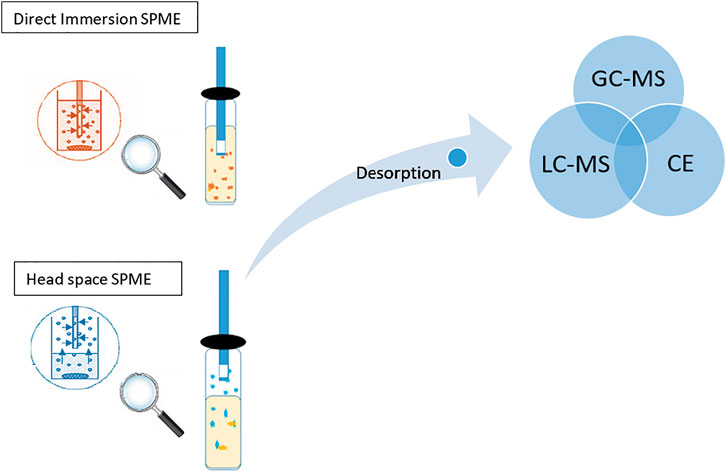
FIGURE 1. Process of extraction of analytes using direct immersion and headspace SPME that further coupled to chromatographic instrument (GC-MS or LC-MS or CE).
Nevertheless, one of the major downsides of SPME is the instability of the fibers: breaking and stripping of coatings that can significantly reduce their overall lifetime (Reyes-Garcés et al., 2018). Using HS extraction, rather than the DI, has improved the lifetime, yet it is still considered a drawback (Płotka-Wasylka et al., 2015). Another negative point of SPME is the reproducibility that mainly results from various factors related to batch-to-batch variation of fiber coatings, low thermal stability of the fibers, short expiry date, and small selectivity. These factors trigger the improvement of materials used for SPME. In general, the DI-SPME has better reproducibility, but it does not allow changes to the sample matrix, for example, change in pH (Płotka-Wasylka et al., 2015).
Fiber Solid-Phase Microextraction and Capillary Solid-Phase Microextraction
The commercially available fibers can be classified into non-bonded, bonded, partially cross-linked, and highly cross-linked phases, which all differ in their degree of stability with organic, polar, and non-polar solvents. The physicochemical properties and coating thickness strongly affect the distribution of analytes between the sample matrix and the extraction phase, which in turn influences the efficiency of extraction, selectivity, and reproducibility of the analysis. Factors to be considered while selecting of the fibers are molecular weight, polarity, and volatility of target analytes (Spietelun et al., 2010). To enhance the selectivity and applicability of solid-phase microextraction (SPME), different coating techniques have been explored and utilized.
Conventional SPME Fiber Coatings
Polydimethylsiloxane (PDMS), polydimethylsiloxane/divinylbenzene (PDMS/DVB), divinylbenzene/carboxen/polydimethylsiloxane (DVB/CAR/PDMS), carbowax/divinylbenzene (CW/DVB), and carbowax/templated resin are used for conventional SPME fibers in both HS and DI modes (Portillo-Castillo et al., 2018). The main problem for the conventional coating is that they are mostly restricted to non-polar or relatively non-polar analytes with relatively low commercial availability (Souza et al., 2013).
Ionic Liquid–Based SPME Fiber Coatings
Ionic liquids are common alternative fiber coating materials. IL-SPME fibers are mostly manufactured by immersion–agglutination techniques for both fused silica and metallic supports. Sometimes, a combination of ILs and adhesive or binder is used to obtain thicker and more resistant coatings. One of the superior advantages is their high viscosity that helps to acquire enhanced coatings and improve film homogeneity and integrity. Moreover, due to the high thermal stability of ionic liquids, the fibers are more resistant with higher half-lives than the conventional ones. In addition, the hydrophobicity of the ionic liquids has to be considered while preparing the coating material. Better extraction efficiency is due to the easier analyte diffusion from bulk matrix samples to SPME fibers with the aid of the ionic liquid. The most commonly used ionic liquids for SPME coating are the ones containing imidazolium cations combined with different anions (Portillo-Castillo et al., 2018).
Polymeric ionic liquids (PILs) are polymeric analogs of ILs; they have the same selectivity and solvation power. However, their viscosity and thermal stability are much higher. As a result, PIL-based coatings have been used for SPME to improve their thermal, mechanical, and chemical resistance. Additionally, it extended their applicability to various samples and different analytes. Afterward, the functionalized PIL-SPME was used to further expand their application. Figure 2A shows an immersion–agglutination coating procedure to obtain IL/PIL-SPME fibers.
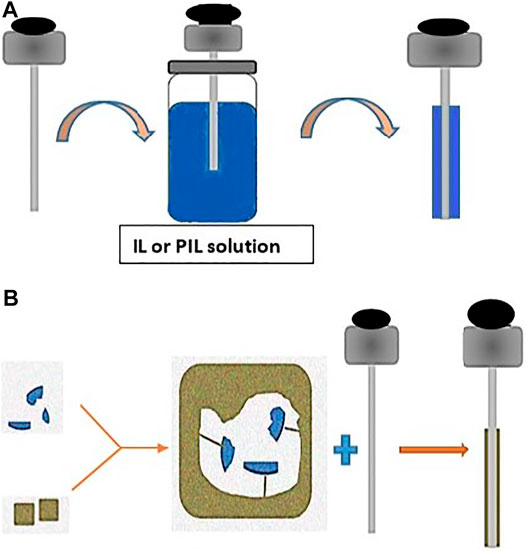
FIGURE 2. (A) Immersion–agglutination coating procedure to obtain IL- or PIL-coated SPME fibers. (B) MIP fabrication and coating of SPME fibers.
In further modification, monolithic SPME fibers are prepared with different materials such as polymers or graphene in a form of very thin rods. Their key advantages are simple preparation, flexibility, and solvent resistance, and they show higher mass transference rates than traditional fibers. Monolithic fibers can be used for direct immersion–solid-phase microextraction (DI-SPME) extractions due to their physical and chemical characteristics (Mei et al., 2014).
Another alternative approach is the multiple monolithic fiber SPME (MMF-SPME); it is based on using several monolithic fibers bound together in a device. The available spaces between the fibers allow sample convection and improve the mass transfer (Mei et al., 2014). Recently, PILs have been used as MMF-SPME fiber coating.
Molecularly Imprinted Polymer SPME Fiber Coatings
Molecularly imprinted polymers are obtained by monomer polymerization with a cross-linking agent using a selected template molecule, mainly through free radical polymerization. The role of the template molecules is to organize the monomer functional groups and outline the properties of binding sites (Zhang et al., 2013). Among the common templates that are used for the molecularly imprinted polymers are drugs, amino acids, proteins, carbohydrates, nucleotides, and hormones. Cross-linking agents mainly function to control the morphology of the matrix and to stabilize the binding site; examples of the common cross-linking agents are ethylene glycol dimethacrylate, trimethylolpropane trimethacrylate, and others. Chloroform, dichloromethane, and acetonitrile are the commonly used solvent in the manufacturing process to maintain all components in only one phase (Vasapollo et al., 2011).
Figure 2B presents a scheme of the fabrication basic principle of MIP and an example of an SPME fiber coating process. MPIs are mainly used for coating SPME-fused silica or metallic fibers. MIP-SPME fibers can be used for direct immersion due to their high solvent resistance and wide pH stability.
Carbon-Based SPME Fiber Coatings
Carbon nanotubes (CNTs), graphene, graphene oxide (GO), and fullerenes are very widely used in sample extraction techniques. Their high superficial area ensures a high extraction capacity (Souza, Risticevic, and Pawliszyn 2013). Graphene (mostly GO) is commonly used in SPME fiber coating for its high surface area and mechanical, chemical, and thermal stability. Being easily functionalized, it offers greater flexibility in extracting different compounds with different natures. It can be prepared by immersion of a stainless steel wire in a graphene adhesive suspension that results in a 6- to 8-μm-thick coating film or by sol-gel procedures. Graphene-based fibers yield 1.5 times higher extraction efficiencies than poly(dimethylsiloxane (PDMS) and poly(dimethylsiloxane/divinylbenzene (PDMS/DVB) commercial fibers; this can be related to their porous surface and their strong affinities to analytes (Portillo-Castillo et al., 2018). Graphene-based SPME fiber was used for extraction of some UV filters that are frequently used in cosmetics, sunscreens, and personal care products (Zhang and Lee 2012a). Multi-walled nanotubes (MWNTs) functionalized with polyethylene glycol were used for coating a fused silica fiber via a sol-gel process. CNTs and MWNTs were used to extract ibuprofen, naproxen, and diclofenac from water samples; it was stable at high temperatures up to 320°C and used for at least 150 extraction cycles (Sarafraz et al., 2012).
Other Materials for SPME Fiber Coatings
Some inorganic materials can be used in SPME fibers due to their good thermal, mechanical, and chemical stability. They also show good selectivity due to their electronic structures, hydrophobic interactions, electrostatic attractions, or covalent bond formation. Ti–TiO2–ZrO2 fiber was used for PPCP analysis, 2-hydroxy-4-methoxybenzophenone, 2-ethylhexyl-4-methoxycinnamate, 2-ethylhexyl-4-(N,N-dimethylamino) benzoate, and ethylhexyl salicylate were extracted by DI of the Ti–TiO2–ZrO2 fiber into the samples with good performance (Li et al., 2020). Gold nanoparticles (C8-S-AuNPs/SS) have also been used for SPME fiber coating and applied to UV filters and phthalate ester (Yang et al., 2017). Metal oxide nanosheets, such as, TiO2 nanosheets and Zn–ZnO nanosheets were used. Conducting polymers (CPs), namely, polypyrrole, polyaniline, and its derivatives have been used as SPME fiber coating. CPs are rigid molecules producing fibers with adequate hardness and sturdiness and show food adsorption capacity (Bagheri, Ayazi, and Naderi 2013). Metal–organic frameworks (MOFs) are also used as a coating material; they are porous materials formed by metal ion clusters connected by organic ligands (Tian et al., 2013).
In-Tube Solid-Phase Microextraction/In-Tube Extraction Dynamic Headspace ITEX-DHS
This technique uses an open tubular capillary as an SPME device and can be coupled with liquid chromatography (LC). It can also be coupled to GC using a GC capillary tube (Kataoka, 2005). In in-tube SPME, the extraction, desorption, and injection can be automated using a standard autosampler. The automation has advantages like shorter analysis time, more accurate, and precise results. The analyte extraction depends on the polarity of the capillary coating, the number and volume of draw/eject cycles, and pH (Kataoka, 2005). In addition, the internal diameter, length, and film thickness of the column have to be chosen carefully. For optimum extraction, the capillary column length should be around 50–60 cm; if the extraction is lower, efficiency is reduced, and above this level, peak broadening can be observed (Kataoka, 2005).
ITEX-DHS grants many advantages compared to the previously described techniques, especially having an external heater unit that allows desorption to be done independently of the injector temperature (Parodi et al., 2019). So far, most of the volatile organic compounds (VOCs) have been analyzed using ITEX-DHS, although many commercial sorbent materials are available. This could be related to the fact that ITEX-DHS is only suitable for HS extraction (Kataoka, 2005).
Solid-Phase Dynamic Extraction
It is an inside-needle technique for vapor and liquid sampling (Ke˛dziora-Koch and Wasiak, 2018). It is composed of stainless steel needles (8 cm) coated with a 50-mm film of PDMS and 10% activated carbon on which the analytes are concentrated. The robustness of the capillary is the main advantage of SPDE over SPME. SPDE has been successfully applied to the analysis of volatile compounds, pesticides, and some drugs. Nevertheless, one of SPDE’s disadvantages is the carryover, since the analytes tend to remain in the inside needle wall followed by heat desorption in the GC injection port (Luo et al., 2010).
Microextraction in a Packed Sorbent
MEPS is considered a potential extraction method that allows for little solvent consumption, small sample volume (10–250 µl) that can be directly injected without additional treatments into GC, LC, or MS and without any modification of the instrument. Another green key point is that it only requires a small amount of sorbent, so only relatively small amounts of solvents are needed for the elution process of the different analytes (Moein et al., 2015). The process for MEPS includes conditional step, sample loading, sample washing, sample elution, and a final MEPS cleaning step. The MEPS device has multiple uses and variable applications, such as, biologicals and environmental and food applications, yet in environmental applications, where the sample volume is high, MEPS shows some drawbacks (Silva et al., 2014).
Several types of packing materials are available; unmodified silica, C2, C8, C18, polystyrene–divinylbenzene (PS-DVB), porous graphitic carbon, MIPs, monoclonal antibodies (mAbs) for immunoaffinity sorbent production, and other commercial ones, as recently reviewed (Yang et al., 2017). In order to improve the reproducibility of the MEPS devices, they are coupled to syringes as they help in the reproducibility of the flow rates. Figure 3A shows the general procedure applied in MEPS extraction.
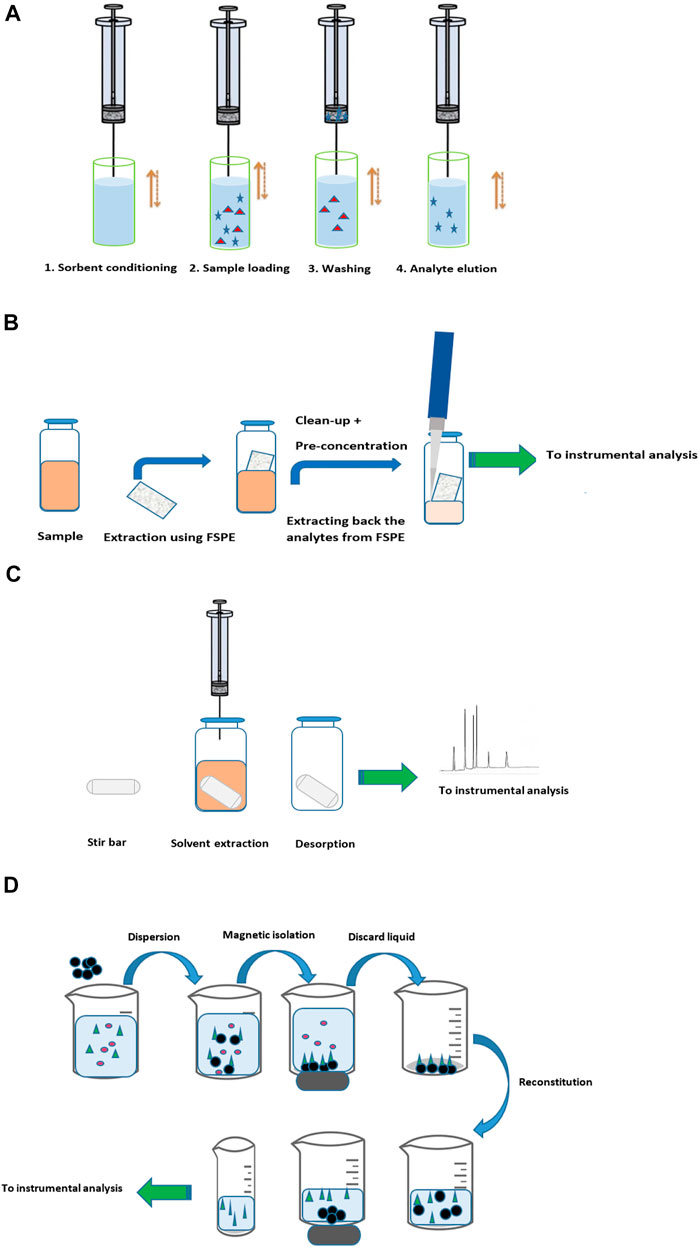
FIGURE 3. (A) General procedure applied in MEPS extraction. (B) General procedure applied in FPSE. (C) General procedure applied in SBSE. (D) General procedure applied in magnetic nanoparticle extraction.
Fabric Phase Sorptive Extraction Procedures
It is a relatively new method, developed by Kabir and Furton (2014), that overcomes the drawback of MEPS as it is effectively used for small- and large-volume samples and could be practically applied in all fields (environmental, biological, food, toxicological, pharmaceutical, and quality control). Recently, the inventors introduce adjustments for FPSE not to either require matrix modifications or clean up (Kabir et al., 2018). FPSE significantly simplifies the sample preparation workflow compared to other available techniques. Figure 3B shows the general procedure applied in FPSE.
Stir Bar Sorptive Extraction
A stir bar with a polymer coat is used to extract the analytes typically by direct immersion; the analytes are extracted and pre-concentrated when the SBSE spins inside the solution. Afterward, the analytes are desorbed using a thermal desorption unit coupled to gas chromatography or by solvent desorption busing a small volume of a suitable organic solvent. It was developed to increase the extraction sensitivity by incorporating substantially higher sorbent loading. As it eliminates the use of solvents and reduces the exhaustive and time-consuming sample preparation step, SBSE simply satisfies the GAC requirements. Only two phases are available, PDMS and poly(ethylene glycol) in PDMS, to be coated onto a glass-coated magnetic bar, and due to their high viscosity, they slow down analyte diffusion during extraction that negatively impacted the extraction sensitivity in SBSE (Płotka-Wasylka et al., 2015). The availability of only two phases is considered as the major drawback of the method. The SBSE is considered as a direct and independent sample preparation device, it can simply operate without the need of an external magnet by diffusing the sample matrix on a magnetic stirrer where both extraction and pre-concentration are directly done. Figure 3C shows the general procedure applied in SBSE.
Magnetic Nanoparticle Extraction
Recently, magnetic nanoparticles have been used for selective extraction of trace species from complex matrices. The dominant advantages of this innovative technique are it is possible to retain the adsorbed analytes directly in the tube, clean away the matrix and the interference compounds, and analyze the trace species in the extract directly. In addition, the use of strong magnet ensures no loss of analytes in the washing step and the possibility of having a great pre-concentration factor for trace analyses. This technique is reported to be used in food analysis (Hernández-Hernández et al., 2017), for drugs in biological matrices (Vasconcelos and Fernandes, 2017), or in other research fields (Ríos and Zougagh, 2017). Figure 3C shows the general procedure applied in magnetic nanoparticle extraction.
PAL SPME Arrow
PAL SPME Arrow was recently introduced to overcome the drawbacks of SPME and SBSE. It combines trace-level sensitivity with high mechanical robustness. Contrary to the classical SPME fibers, the Arrow design fully shields the sorptive material, reducing adverse effects and losing of analytes during transfer processes. Additionally, PAL SPME Arrow has a larger sorbent volume, providing a higher extraction capacity than SPME, as seen in Figure 4. In contrary to SBSE, it can be fully automated. It was used and compared to SPME in extracting polycyclic aromatic hydrocarbons (PAHs) from the freely dissolved fraction in laboratory water and groundwater using polydimethylsiloxane (PDMS) as common sorption phase material via direct immersion (Kremser et al., 2016). Table 1 compares the advantages and drawbacks of these microextraction techniques.
Extraction Efficiencies of SPME Techniques
Despite the practical applicability of SPME in sampling procedures, modifications to improve the extraction efficiencies are still a research aim. In general, the extraction efficiencies for SPME will depend on extraction conditions, that is, temperature, extraction time, and mixing method, ionic strength of solutions, sample pH, stationary phase volume, headspace phase volume, the volume of the sample, and fiber material (Rutkowska et al., 2014).
It could be improved by adding salt to the sample for example sodium chloride and sodium sulfate (salting out), pH or temperature changes (as it affects the partitioning of the targeted analytes, due to the different pKa values); agitating the sample by stirring, for example, it can enhance the extraction efficiency in non-equilibrium situations (Fiorini et al., 2015).
Other solutions related to the use of unique phases have been developed to improve extraction efficiency, for instance, using inner coating with polypyrrole polymers for the commercially fused silica capillary (Portillo-Castillo et al., 2018). In addition, optimum column length is important for the efficiency of extraction. Other techniques include wire-in-tube or fiber-in-tube SPME, which improves extraction efficiency while extending the method to microscale applications.
In SBSE, the sample volume and the speed of stirring impact the extraction efficiency, and the usual stirring times for equilibration are between 30 and 60 min (Pang et al., 2017). The introductions of SPME–trap and SPME–trap with multi-step enrichment (MSE) are improvements in sample extraction and lower detection limits (Emmons et al., 2019).
Since the extraction efficiency can be affected by a high number of factors, the role of each factor and its interactions have to be studied for better extraction outcomes. For this purpose, formal design of experiments (DoE) can provide a fast, efficient, and cost-effective approach that is superior to that achievable by the one-factor-at-a-time (OFAT) methodology. Two broad groups of experimental designs are used: simple linear models (screen from two to 6–8 factors) and the more complicated quadratic models to describe the response as a function of two to four factors by applying the response surface methodology (RSM) (Marrubini et al., 2020). There are many pieces of software used for DoE computations, namely, Chemometric Agile Tool (CAT), Design Expert, Minitab® Statistical Software, JMP, and MATLAB. Marrubini and coworkers reviewed articles published in the period from 2009 to 2019 that used microextraction techniques and applied DoE. They included some points that represent the least information needed to describe and report a DoE study: the experimental plan and experimental matrix, the model equation and model adequacy checking (R2, adjusted R2, residuals, analysis of variance (ANOVA), t-test of the coefficients, normal probability plot, inflation factors), the model validation and finally the response surfaces plots, interpretation, and discussion of the role of the factors (Marrubini et al., 2020).
Selection Criteria for the Microextraction Technique
The selection of the optimal microextraction technique can be an overwhelming task for the researchers, and it highly depends on the specific analytical problem. The characteristics of the analytes are very crucial to determine the most suitable extraction technique, and the analytes’ partitioning constants can help predict the suitability of the DI or HS extraction mode (Parodi et al., 2019). Another factor to be considered is the volatility of the analytes, if the analytes are sufficiently volatile, HS is always preferred over DI extraction. Using HS with volatile analytes allows exclusion of more interferences, faster equilibrium due to the diffusion boundary layer being thinner, and prolonged lifetime of the sorbent material (Płotka-Wasylka et al., 2015). Both DI and HS sampling modes of SPME are used extensively in SPME–GC application. HS extraction can be performed with all the techniques previously described; however, it is best fit with the dynamic extraction techniques like ITEX-DHS. While DI–SPME is more suitable for gaseous or simple liquid sample matrices, HS–SPME is preferentially used for extraction from complex liquid and solid samples. For very dirty samples, the HS mode is the most suitable, yet if the analysis becomes more complicated, pH adjustment or salt addition should be performed to facilitate the transfer of the target compounds to the headspace. HS also is more suitable to analyze highly volatile and low-polar analytes in contrary to DI extraction. Commonly, for SPME–LC applications, DI is used as the analytes of interest are not sufficiently volatile. PAL SPME Arrow, MEPS, and SBSE are the recommended techniques, especially when coupled to GC. Better sensitivities are achieved by SBSE due to the higher phase volume available. Nevertheless, it still requires a significantly longer time to reach equilibrium in addition to substantial matrix effects (Płotka-Wasylka et al., 2015). The limiting spectrum of analytes for SBSE is mainly due to the limited availability of the coating material. If the optimal phase is not available in the SBSE design, PAL SPME Arrow is the recommended alternative. Table 1 summarizes the advantages and limitations of these green microextraction techniques.
Applications
A wide application range for pharmaceutical extractions using solventless techniques is reported, which includes analysis of pharmaceuticals in environmental, water, food, and biological matrices. All applications would never be possible to summarize; yet in Table 2, some solventless extraction techniques used for pharmaceutical applications in different matrices are presented.
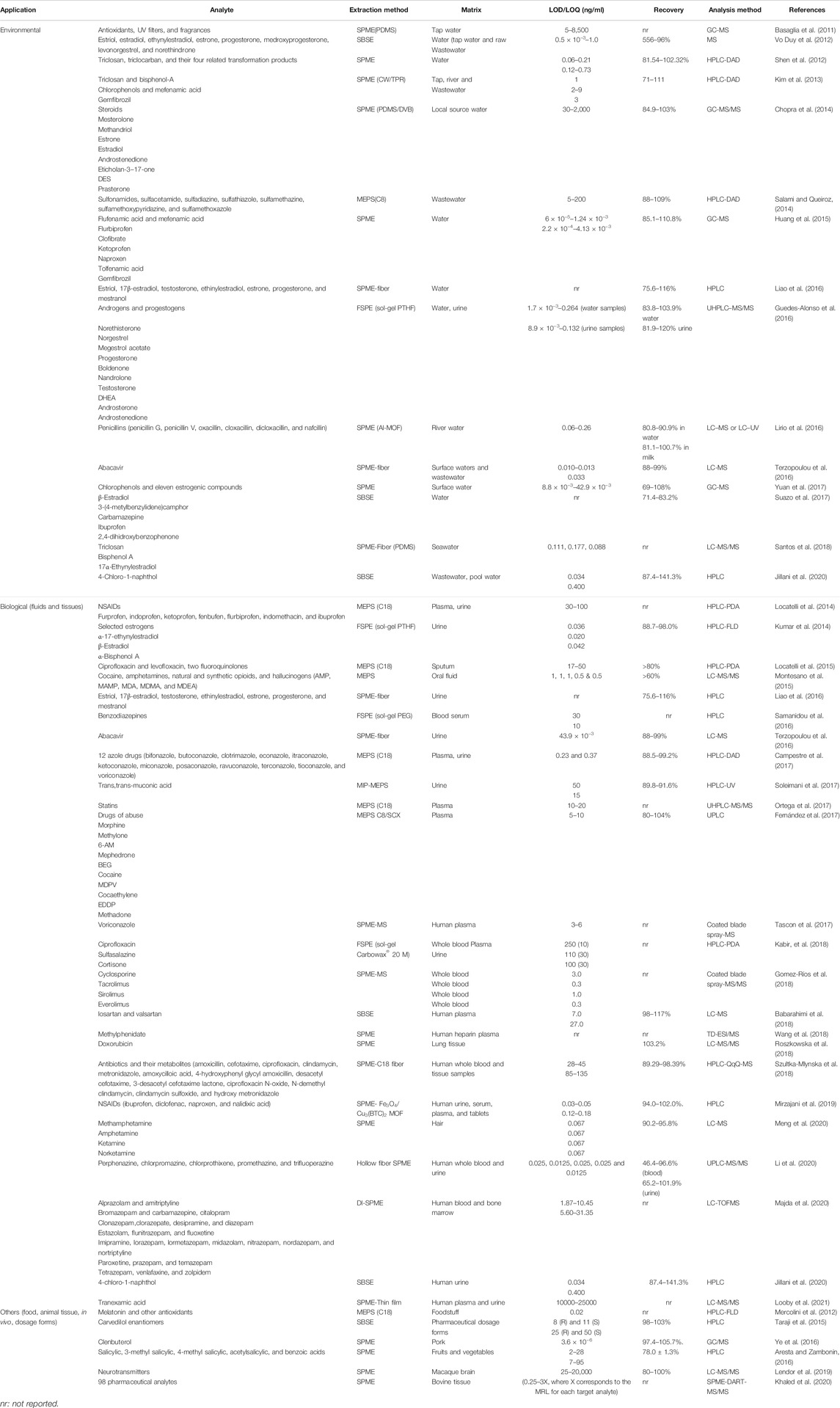
TABLE 2. Applications of the microextraction techniques discussed in this review for determination of pharmaceuticals in different matrices.
Environment Applications
Many novel microextraction techniques are now replacing liquid extraction for the analysis of pharmaceuticals in water samples. It enriches and enables the direct injection of the analytes into the separation unit, which requires less solvents, time, and labor (Płotka-Wasylka et al., 2015; Ke˛dziora-Koch and Wasiak, 2018). They are usually coupled to gas chromatography–mass spectrometry or liquid chromatography–mass spectrometry. The determination of chlorophenols in water is very important due to its high toxicity level (Gallego et al., 2018). Many studies had reported the extraction of chlorophenols and some estrogenic compounds using HS-SPME, followed by on-fiber derivatization coupled with GC-MS (Yuan et al., 2017).
VOCs, being volatile, can be analyzed using preferably HS-SPME–based methods, rather than DI (DIN EN ISO 17943:2016). SPDE and ITEX-DHS are very useful for the VOC analysis in water, and due to the greater sorption volume, their linear range is broader and LODs are much lower. SPME-GC was developed for monitoring selected personal care products, estrogens, and pharmaceutical compounds (clofibric acid and carbamazepine) in surface water (Huang et al., 2015).
In a study to determine phenolic compounds, bisphenol A, and acidic pharmaceuticals, the sensitivity was adjusted by calculating the log D values of the target analytes at different pH levels. To ensure the desorption of strong polar–polar interactions between analyte and solid phase, SPME on a carbowax/templated fiber was used, and the results showed better sensitivity and remarkable improvement in the level of detection, 1 ng/ml in tap and river water and 2–9 ng/ml for wastewater (Kim et al., 2013).
Monolithic fibers have been developed and used to improve the mechanical stability of MIP to extract diacetylmorphine and analogous compounds in aqueous solution (Yılmaz et al., 2017). In another study, using multiple monolithic fiber SPME, analysis of sex hormones in tap and lake water was done with good performance and recoveries between 77.7 and 115%. The use of poly(1-allyl-3-methylimidazolium) bis(trifluoromethylsulfonyl)imide copolymerized with ethylene dimethacrylate as the monolithic fiber coat has resulted in a lifetime that is higher than 200 cycles and the analysis time is shorter (Liao et al., 2016).
MEPS provides a significant advantage by allowing the use of small sample volume, particularly when the amount of sample is limited, especially in forensic toxicology. The method showed a good recovery range for all the studied drugs of abuse 80–104%. In addition, the sorbent can be reused up to 80 times in human plasma (Fernández et al., 2017), the use of limited volumes, and the reusability of the sorbent can add to the green credit of the method (Mohamed, 2015). In another study, Soleimani and coworkers combined the MIP and MEPS (MIMEPS) and used it for the extraction of trans,trans-muconic acid; the LOQ of the method was lower than that suggested by the American Conference of Governmental Industrial Hygienists (75 μg/ml) (Soleimani et al., 2017). The MIMEPS used much smaller volumes of solvents for conditioning, washing, and elution procedures, which makes it a greener alternative than the conventional SPE technique (Soleimani et al., 2017). To enhance the extraction recoveries of sulphonamides from wastewater (88–109%), Salami et al. (Salami and Queiroz, 2014), used salting out by adding 15% NaCl, where the water molecules form hydration spheres around the NaCl molecules. In consequence, they reduce the water concentration available for dissolution of the analyte molecules, and this will drive additional analytes into the extraction phase.
Biological Applications
The use of solventless microextraction techniques has a broad spectrum of applications in clinical control for diagnosis and treatment of diseases, doping, forensic analysis, and toxicology. All of the different techniques could be coupled to on-line HPLC, LC/MS, or GC/MS. Each technique as discussed earlier has its advantage and disadvantage. Table 2 represents some trials on extracting pharmaceuticals in the biological matrix with the extraction and analysis methods, recoveries, and LOD/LOQ.
Twenty illicit drugs and their metabolites were determined in oral fluid using MEPS; the method was successful in the determination of the selected drugs with fairly lower cutoff values than recommended by the Substance Abuse and Mental Health Services Administration (SAMHSA) (Montesano et al., 2015), using a miniaturized extraction procedure.
Analysis of amphetamine, methamphetamine, cannabinoids, cocaine, opioids, and hallucinogens was reported using various microextraction techniques. Determination was done in saliva samples for forensic purposes (Jain, 2017). The SPME coupled to mass spectroscopy and coated blade spray (CBS) was used to determine voriconazole in human plasma (Tascon et al., 2017) and immunosuppressant drugs in whole blood (Gomez-Ríos et al., 2018). Using the CBS has a challenge that the analytes largely bound to plasma proteins or red blood cells resulting in noticeably lower extraction recovery rates. Hence, it was treated by performing the analyte enrichment step through direct immersion in a solvent-modified matrix (Gomez-Ríos et al., 2018).
Abacavir, an anti-HIV drug, was determined using an SPME fiber coated with an acrylic acid–based MIP (Terzopoulou et al., 2016). An observed good recognition capability for abacavir in real sample analysis was reported, with extraction efficiencies 88–99%, and no matrix effects in either biological or environmental matrices—urine and wastewater.
Solid-phase microextraction (SPME) was also used for therapeutic drug monitoring of tranexamic acid in plasma and urine of patients with renal failure. The method was a greener alternative for the fast high-throughput monitoring of tranexamic with no biological matrix interference. The use of a biocompatible polyacrylonitrile-based hydrophilic–lipophilic–balance extraction phase help prevent the macromolecules from any interaction with the device which make the method convenient and easy to use as it allows direct exposure of the sample without major sample pretreatment steps which make it a greener alternative (Looby et al., 2021).
The use of FPSE for the determination of important biological molecules, 17-ethynylestradiol, β-estradiol, and bisphenol A, had shown lower detection limits of the analytes over previously reported methods. The analysis time was lower than the compared methods, and this contributes to the economic efficiency of the method and its greenness profile. Good recoveries were obtained for drinking water (96–98%), river water (92–94%), and groundwater (94–95%) (Kumar et al., 2014). In further study that determines some androgens and progestogens in water and urine samples, the analysis showed lower values for the matrix effect (between −10 and +7% for all the compounds), which ensures high selectivity from the interferences and the target analytes (Guedes-Alonso et al., 2016).
SPME coupled with thermal desorption–electrospray ionization/mass spectrometry (TD-ESI/MS) was used to study the pharmacokinetics of methylphenidate in plasma in a very small sample volume. High sensitivity and reproducibility of the SPME-TD-ESI/MS were reported due to the suitability of the PDMS for concentrating plasma methylphenidate, complete desorption in the thermal decomposition chamber, and the ionization efficiency of methylphenidate (Wang et al., 2018).
SBSE has been successfully applied to pharmaceutical analysis in different kinds of matrices, including environmental water, biological fluids, soils, and gaseous samples (Vo Duy et al., 2012; Hu et al., 2013). Several trials have been going on to improve the SBSE efficiency and its recent developments (Amlashi and Hadjmohammadi, 2016); nevertheless, the number of applications within this field is lower than that in food and environment analyses (Camino-Sánchez et al., 2014). This could be related to the complexity of the biological matrix where SBSE is not a highly selective or specific extraction technique. Moreover, many pharmaceutical analytes are polar compounds, and they have reduced extraction efficiency when PDMS is in the stationary phase (Camino-Sánchez et al., 2014; Taraji et al., 2015). Some approaches were carried out recently to increase the recovery of polar analytes, for instance, in situ derivatization and SBSE with solvent-swollen PDMS (Ochiai et al., 2018).
Other Applications
Moreover, solventless extraction techniques can be used for pharmaceutical extraction in pharmaceutical formulations, animal tissues, and food samples, as shown in Table 2. Mercolini and coworkers (Mercolini et al., 2012) had determined melatonin and other antioxidants in food and beverages derived from grapes. The sample pretreatment was carried out by fast and reliable microextraction by packed sorbent (MEPS) procedure. This was followed by an HPLC determination coupled with fluorescence detection. Similarly, sodium chloride addition from 0 to 0.3 g/ml was used to enhance the extraction efficiencies of salicylic, 3-methyl salicylic, 4-methyl salicylic, acetylsalicylic, and benzoic acids from fruits and vegetables (Aresta and Zambonin, 2016).
SPME was used to determine clenbuterol in pork using GC-MS, with 97.4–105.7% recovery (Ye et al., 2016), and neurotransmitters in bovine tissue using LC-MS/MS (Lendor et al., 2019). SPME was used for direct analysis in real time, coupled to tandem mass spectroscopy, for rapid and high-throughput screening of multi-residue pharmaceuticals in bovine tissue. Although it has limited analyte scope and lower detectability levels compared to electrospray ionization–based methods, yet the high-throughput, simplicity, and real-time use are advantages of the technique. In another work (Khaled et al., 2020), the multi-residue pharmaceutical drugs in bovine tissue were analyzed using solid-phase microextraction and direct analysis in real-time-tandem mass spectrometry (SPME-DART-MS/MS).
SBSE was used to separate carvedilol enantiomers (Taraji et al., 2015) in pharmaceutical dosage forms. The applicability of two sorptive phases, poly(methyl methacrylate/ethyleneglycol dimethacrylate) (PA–EG) and polydimethylsiloxane, was evaluated for extracting carvedilol enantiomers from aqueous samples. The homemade PA–EG sorptive phase has shown recovery yields much better than the conventional PDMS and the least limit of detections and shorter time of analysis (Taraji et al., 2015).
Conclusion
The extraction (cleanup) process is considered one of the major steps that hinder the greenness of any analysis procedure, especially complex matrices, due to high solvent consumption and use of toxic solvents; in addition, it prolongs the time of the analysis. Solid-phase extraction (SPE) techniques offer an interesting alternative to conventional liquid–liquid extraction. Having the advantage of being simple, economic in terms of time and solvents use, the SPE techniques have become more popular overtime. Advancement to improve them to be better solutions for sample preparation has been introduced, for instance, SPME, SBSE, MEPS, FPSE, and PAL SPME Arrow. These new techniques are considering the green analytical chemistry principles at many aspects (miniaturization, cost, solventless, etc.) that allow them to be a greener alternative.
Various factors contribute to select the optimum technique, for instance, the sample type, the analyte of interest, and the priority of the analysis. Moreover, the analytes’ characteristics, for instance, the partitioning constant, can affect the selection of headspace or direct immersion for the extraction mode.
Another advantage of these microextraction techniques is that they allow the analysis to take place using simpler analytical methods such as HPLC-UV/Vis while avoiding the use of more complex and expensive ones if needed, which again supports the GAC principles. Further investigation of more novel sorbents with advanced features that improve selectivity, loading capacity, or retention efficiency, and recoveries is still needed. In addition, exploring futuristic tools such as smartphones, microfluidics, and other small handheld detectors will be useful to ensure their sustainability and suitability.
Author Contributions
The author confirms being the sole contributor of this work and has approved it for publication.
Conflict of Interest
The author declares that the research was conducted in the absence of any commercial or financial relationships that could be construed as a potential conflict of interest.
Publisher’s Note
All claims expressed in this article are solely those of the authors and do not necessarily represent those of their affiliated organizations, or those of the publisher, the editors, and the reviewers. Any product that may be evaluated in this article, or claim that may be made by its manufacturer, is not guaranteed or endorsed by the publisher.
Abbreviations
CNTs, carbon nanotubes; DART-MS/MS, direct analysis in real-time tandem mass spectrometry; DI, direct immersion; DI-SPME, direct immersion–solid-phase microextraction; FPSE, fabric phase sorptive extraction; GAC, green analytical chemistry; GO, graphene oxide; HS, headspace; HS-SPME, headspace–solid-phase microextraction; IL-SPME, ionic liquid–solid-phase microextraction; ITEX-DHS, in-tube extraction–dynamic headspace; MEPS, microextraction in a packed sorbent; MMF-SPME, multiple monolithic fiber–solid-phase microextraction; MPI-SPME, molecularly imprinted polymer–solid-phase micro extraction; MWNTs, multi-walled nanotubes; PAL, prep and load solution; PILs, polymeric ionic liquids; SBSE, stir bar sorptive extraction; SPDE, solid-phase dynamic extraction; SPME, solid-phase microextraction; VOCs, volatile organic compounds.
References
Abuzar, K., Locatelli, M., and Ulusoy, H. (2017). Recent Trends in Microextraction Techniques Employed in Analytical and Bioanalytical Sample Preparation. Separations 4, 36. doi:10.3390/separations4040036
Amlashi, N. E., and Hadjmohammadi, M. R. (2016). Sol-gel Coating of Poly(ethylene Glycol)-Grafted Multiwalled Carbon Nanotubes for Stir Bar Sorptive Extraction and its Application to the Analysis of Polycyclic Aromatic Hydrocarbons in Water. J. Sep. Sci. 39, 3445–3456. doi:10.1002/jssc.201600416
Aresta, A., and Zambonin, C. (2016). Simultaneous Determination of Salicylic, 3-methyl Salicylic, 4-methyl Salicylic, Acetylsalicylic and Benzoic Acids in Fruit, Vegetables and Derived Beverages by SPME-LC-UV/DAD. J. Pharm. Biomed. Anal. 121, 63–68. doi:10.1016/j.jpba.2015.12.016
Babarahimi, V., Talebpour, Z., Haghighi, F., Adib, N., and Vahidi, H. (2018). Validated Determination of Losartan and Valsartan in Human Plasma by Stir Bar Sorptive Extraction Based on Acrylate Monolithic Polymer, Liquid Chromatographic Analysis and Experimental Design Methodology. J. Pharm. Biomed. Anal. 153, 204–213. doi:10.1016/j.jpba.2018.02.030
Bagheri, H., Ayazi, Z., and Naderi, M. (2013). Conductive Polymer-Based Microextraction Methods: a Review. Analytica Chim. Acta 767, 1–13. doi:10.1016/j.aca.2012.12.013
Basaglia, G., Pasti, L., and Pietrogrande, M. C. (2011). Multi-residual GC-MS Determination of Personal Care Products in Waters Using Solid-phase Microextraction. Anal. Bioanal. Chem. 399, 2257–2265. doi:10.1007/s00216-010-4609-4
Camino-Sánchez, F. J., Rodríguez-Gómez, R., Zafra-Gómez, A., Santos-Fandila, A., and Vílchez, J. L. (2014). Stir Bar Sorptive Extraction: Recent Applications, Limitations and Future Trends. Talanta 130, 388–399.
Campestre, C., Locatelli, M., Guglielmi, P., De Luca, E., Bellagamba, G., Menta, S., et al. (2017). Analysis of Imidazoles and Triazoles in Biological Samples after MicroExtraction by Packed Sorbent. J. Enzyme Inhib. Med. Chem. 32, 1053–1063. doi:10.1080/14756366.2017.1354858
Chopra, S., Gomes, P. C. F. L., Dhandapani, R., and Snow, N. H. (2014). Analysis of Steroids Using Solid Phase Microextraction-Gas Chromatography-Mass Spectrometry-Mass Spectrometry (SPME-GC-MS-MS). Scientia Chromatographica 6, 105–116. doi:10.4322/sc.2014.024
Ebele, A. J., Abou-Elwafa Abdallah, M., and Harrad, S. (2017). Pharmaceuticals and Personal Care Products (PPCPs) in the Freshwater Aquatic Environment. Emerging Contaminants 3 (1), 1–16. doi:10.1016/j.emcon.2016.12.004
Emmons, R. V., Tajali, R., and Gionfriddo, E. (2019). Development, Optimization and Applications of Thin Film Solid Phase Microextraction (TF-SPME) Devices for Thermal Desorption: A Comprehensive Review. Separations 6, 39. doi:10.3390/separations6030039
Fernández, P., González, M., Regenjo, M., Ares, A. M., Fernández, A. M., Lorenzo, R. A., et al. (2017). Analysis of Drugs of Abuse in Human Plasma Using Microextraction by Packed Sorbents and Ultra-high-performance Liquid Chromatography. J. Chromatogr. A. 1485, 8–19.
Fiorini, D., Pacetti, D., Gabbianelli, R., Gabrielli, S., and Ballini, R. (2015). A Salting Out System for Improving the Efficiency of the Headspace Solid-phase Microextraction of Short and Medium Chain Free Fatty Acids. J. Chromatogr. A 1409, 282–287. doi:10.1016/j.chroma.2015.07.051
Gallego, A., Laurino Soulé, J., Napolitano, H., Rossi, S. L., Vescina, C., and Korol, S. E. (2018). Biodegradability of Chlorophenols in Surface Waters from the Urban Area of Buenos Aires. Bull. Environ. Contam. Toxicol. 100, 541–547. doi:10.1007/s00128-018-2300-1
Gómez-Ríos, G. A., Tascon, M., Reyes-Garcés, N., Boyacı, E., Poole, J. J., and Pawliszyn, J. (2018). Rapid Determination of Immunosuppressive Drug Concentrations in Whole Blood by Coated Blade spray-tandem Mass Spectrometry (CBS-MS/MS). Anal. Chim. Acta 999, 69–75. doi:10.1016/j.aca.2017.10.016
Guedes-Alonso, R., Ciofi, L., Sosa-Ferrera, Z., Santana-Rodríguez, J. J., Bubba, M. d., Kabir, A., et al. (2016). Determination of Androgens and Progestogens in Environmental and Biological Samples Using Fabric Phase Sorptive Extraction Coupled to Ultra-high Performance Liquid Chromatography Tandem Mass Spectrometry. J. Chromatogr. A 1437, 116–126. doi:10.1016/j.chroma.2016.01.077
Hernández-Hernández, A. A., Álvarez-Romero, G. A., Contreras-López, E., Aguilar-Arteaga, K., and Castañeda-Ovando, A. (2017). Food Analysis by Microextraction Methods Based on the Use of Magnetic Nanoparticles as Supports: Recent Advances. Food Anal. Methods 10, 2974–2993.
Hu, C., He, M., Chen, B., and Hu, B. (2013). A Sol-Gel Polydimethylsiloxane/polythiophene Coated Stir Bar Sorptive Extraction Combined with Gas Chromatography-Flame Photometric Detection for the Determination of Organophosphorus Pesticides in Environmental Water Samples. J. Chromatogr. A 1275, 25–31. doi:10.1016/j.chroma.2012.12.036
Huang, S., Zhu, F., Jiang, R., Zhou, S., Zhu, D., Liu, H., et al. (2015). Determination of Eight Pharmaceuticals in an Aqueous Sample Using Automated Derivatization Solid-phase Microextraction Combined with Gas Chromatography-Mass Spectrometry. Talanta 136, 198–203. doi:10.1016/j.talanta.2014.11.071
International Organization for Standardization (2016). DIN EN ISO 17943:2016, Water quality e Determination of volatile organic compounds in water - Method using headspace solid-phase micro-extraction (HS-SPME) followed by gas chromatography-mass spectrometry (GCeMS). Geneva, Switzerland: International Organization for Standardization.
Jain, R. (2017). Microextraction Techniques for Forensic Drug Analysis in Saliva. Forensic Res. Criminol Int. J. 5 (4), 00164. doi:10.15406/frcij.2017.05.00164
Jillani, S. M. S., Ganiyu, S. A., and Alhooshani, K. (2020). Development of a SBSE-HPLC Method Using Sol-Gel Based Germania Coated Twister for the Analysis of 4-Chloro-1-Naphthol in Biological and Water Samples. Arabian J. Chem. 13, 3440–3447. doi:10.1016/j.arabjc.2018.11.016
Kabir, A., and Furton, K. G. (2014). Fabric Phase Sorptive Extractor (FPSE). U.S. Patent US 20140274660A1. 18 September 2014.
Kabir, A., Furton, K. G., Tinari, N., Grossi, L., Innosa, D., Macerola, D., et al. (2018). Fabric Phase Sorptive Extraction-High Performance Liquid Chromatography-Photo Diode Array Detection Method for Simultaneous Monitoring of Three Inflammatory Bowel Disease Treatment Drugs in Whole Blood, Plasma and Urine. J. Chromatogr. B 1084, 53–63. doi:10.1016/j.jchromb.2018.03.028
Kataoka, H. (2005). Recent Advances in Solid-Phase Microextraction and Related Techniques for Pharmaceutical and Biomedical Analysis. Curr. Pharm. Anal. 1, 65–84.
Kędziora-Koch, K., and Wasiak, W. (2018). Needle-based Extraction Techniques with Protected Sorbent as Powerful Sample Preparation Tools to Gas Chromatographic Analysis: Trends in Application. J. Chromatogr. A 1565, 1–18. doi:10.1016/j.chroma.2018.06.046
Khaled, A., Belinato, J. R., and Pawliszyn, J. (2020). Rapid and High‐throughput Screening of Multi-Residue Pharmaceutical Drugs in Bovine Tissue Using Solid Phase Microextraction and Direct Analysis in Real Time-Tandem Mass Spectrometry (SPME-DART-MS/MS). Talanta 217, 121095. doi:10.1016/j.talanta.2020.121095
Kim, D., Han, J., and Choi, Y. (2013). On-line Solid-phase Microextraction of Triclosan, Bisphenol A, Chlorophenols, and Selected Pharmaceuticals in Environmental Water Samples by High-Performance Liquid Chromatography-Ultraviolet Detection. Anal. Bioanal. Chem. 405, 377–387. doi:10.1007/s00216-012-6490-9
Kremser, A., Jochmann, M. A., and Schmidt, T. C. (2016). PAL SPME Arrow-Evaluation of a Novel Solid-phase Microextraction Device for Freely Dissolved PAHs in Water. Anal. Bioanal. Chem. 408 (3), 943–952. doi:10.1007/s00216-015-9187-z
Kumar, R., Gaurav, A. K., Heena, A., Malik, A. K., Kabir, A., and Furton, K. G. (2014). Efficient Analysis of Selected Estrogens Using Fabric Phase Sorptive Extraction and High Performance Liquid Chromatography-Fluorescence Detection. J. Chromatogr. A 1359, 16–25. doi:10.1016/j.chroma.2014.07.013
Lendor, S., Hassani, S.-A., Boyaci, E., Singh, V., Womelsdorf, T., and Pawliszyn, J. (2019). Solid Phase Microextraction-Based Miniaturized Probe and Protocol for Extraction of Neurotransmitters from Brains In Vivo. Anal. Chem. 91, 4896–4905. doi:10.1021/acs.analchem.9b00995
Li, J., An, J., and Jiang, y. (2020). Development of a Method of Hollow Fiber-Based Solid-phase Microextraction Followed by Ultra Performance Liquid Chromatography-Tandem Mass Spectrometry for Determination of Five Antipsychotics in Human Whole Blood and Urine. J. Chromatogr. A. 1620, 461000. doi:10.1016/j.chroma.2020.461000
Liao, K., Mei, M., Li, H., Huang, X., and Wu, C. (2016). Multiple Monolithic Fiber Solid-phase Microextraction Based on a Polymeric Ionic Liquid with High-Performance Liquid Chromatography for the Determination of Steroid Sex Hormones in Water and Urine. J. Sep. Sci. 39, 566–575. doi:10.1002/jssc.201501156
Lirio, S., Liu, W.-L., Lin, C.-L., Lin, C.-H., and Huang, H.-Y. (2016). Aluminum Based Metal-Organic Framework-Polymer Monolith in Solid-phase Microextraction of Penicillins in River Water and Milk Samples. J. Chromatogr. A 1428, 236–245. doi:10.1016/j.chroma.2015.05.043
Locatelli, M., Ciavarella, M. T., Paolino, D., Celia, C., Fiscarelli, E., Ricciotti, G., et al. (2015). Determination of Ciprofloxacin and Levofloxacin in Human Sputum Collected from Cystic Fibrosis Patients Using Microextraction by Packed Sorbent-High Performance Liquid Chromatography PhotoDiode Array Detector. J. Chromatogr. A 1419, 58–66. doi:10.1016/j.chroma.2015.09.075
Locatelli, M., Ferrone, V., Cifelli, R., Barbacane, R. C., and Carlucci, G. (2014). Microextraction by Packed Sorbent and High Performance Liquid Chromatography Determination of Seven Non-steroidal Anti-inflammatory Drugs in Human Plasma and Urine. J. Chromatogr. A 1367, 1–8. doi:10.1016/j.chroma.2014.09.034
Looby, N., Vasiljevic, T., Reyes-Garcés, N., Roszkowska, A., Bojko, B., Wąsowicz, M., et al. (2021). Therapeutic Drug Monitoring of Tranexamic Acid in Plasma and Urine of Renally Impaired Patients Using Solid Phase Microextraction. Talanta 225, 121945. doi:10.1016/j.talanta.2020.121945
Lorenzo-Parodi, N., Kaziur, W., Stojanović, N., Jochmann, M. A., and Schmidt, T. C. (2019). Solventless Microextraction Techniques for Water Analysis. Trac Trends Anal. Chem. 113, 321–331. doi:10.1016/j.trac.2018.11.013
Luo, Y.-B., Ma, Q., and Feng, Y.-Q. (2010). Stir Rod Sorptive Extraction with Monolithic Polymer as Coating and its Application to the Analysis of Fluoroquinolones in Honey Sample. J. Chromatogr. A 1217, 3583–3589. doi:10.1016/j.chroma.2010.03.036
Luo, Y.-B., Zheng, H.-B., Wang, J.-X., Gao, Q., YuFeng, Q.-W. Y. Q., and Feng, Y.-Q. (2011). An Anionic Exchange Stir Rod Sorptive Extraction Based on Monolithic Material for the Extraction of Non-steroidal Anti-inflammatory Drugs in Environmental Aqueous Samples. Talanta 86, 103–108. doi:10.1016/j.talanta.2011.08.020
Majda, A., Mrochem, K., Wietecha-Posłuszny, R., Zapotoczny, S., and Zawadzki, M. (2020). Fast and Efficient Analyses of the post-mortem Human Blood and Bone Marrow Using DI-SPME/LC-TOFMS Method for Forensic Medicine Purposes. Talanta 209, 120533. doi:10.1016/j.talanta.2019.120533
Marrubini, G., Dugheri, S., Cappelli, G., Arcangeli, G., Mucci, N., Appelblad, P., et al. (2020). Experimental Designs for Solid-phase Microextraction Method Development in Bioanalysis: A Review. Analytica Chim. Acta 1119, 77–100. doi:10.1016/j.aca.2020.04.012
Mei, M., Huang, X., and Yuan, D. (2014). Multiple Monolithic Fiber Solid-phase Microextraction: a New Extraction Approach for Aqueous Samples. J. Chromatogr. A 1345, 29–36. doi:10.1016/j.chroma.2014.04.029
Meng, L., Dai, Y., Chen, C., and Zhang, J. (2020). Determination of Amphetamines, Ketamine and Their Metabolites in Hair with High-Speed Grinding and Solid-phase Microextraction Followed by LC-MS. Forensic Sci. Res. 6, 273–280. doi:10.1080/20961790.2020.1838403
Mercolini, L., Mandrioli, R., and Raggi, M. A. (2012). Content of Melatonin and Other Antioxidants in Grape-Related Foodstuffs: Measurement Using a MEPS-HPLC-F Method. J. Pineal Res. 53, 21–28. doi:10.1111/j.1600-079x.2011.00967.x
Mirzajani, R., Kardani, F., and Ramezani, Z. (2019). Preparation and Characterization of Magnetic Metal-Organic Framework Nanocomposite as Solid-phase Microextraction Fibers Coupled with High-Performance Liquid Chromatography for Determination of Non-steroidal Anti-inflammatory Drugs in Biological Fluids and Tablet Formulation Samples. Microchemical J. 144, 270–284. doi:10.1016/j.microc.2018.09.014
Moein, M. M., Abdel-Rehim, A., and Abdel-Rehim, M. (2015). Microextraction by Packed Sorbent (MEPS). Trac Trends Anal. Chem. 67, 34–44. doi:10.1016/j.trac.2014.12.003
Mohamed, H. M. (2015). Green, Environment-Friendly, Analytical Tools Give Insights in Pharmaceuticals and Cosmetics Analysis. Trac Trends Anal. Chem. 66, 176–192. doi:10.1016/j.trac.2014.11.010
Montesano, C., Simeoni, M. C., Curini, R., Sergi, M., Lo Sterzo, C., and Compagnone, D. (2015). Determination of Illicit Drugs and Metabolites in Oral Fluid by Microextraction on Packed Sorbent Coupled with LC-MS/MS. Anal. Bioanal. Chem. 407 (13), 3647–3658. doi:10.1007/s00216-015-8583-8
Ochiai, N., Sasamoto, K., David, F., and Sandra, P. (2018). Recent Developments of Stir Bar Sorptive Extraction for Food Applications: Extension to Polar Solutes. J. Agric. Food Chem. 66 (28), 7249–7255. doi:10.1021/acs.jafc.8b02182
Ortega, S. N., Santos-Neto, A. J., and Lancas, F. M. (2017). Development and Optimization of a Fast Method for the Determination of Statins in Human Plasma Using Microextraction by Packed Sorbent (MEPS) Followed by Ultra High-Performance Liquid Chromatography-Tandem Mass Spectrometry (UHPLC-MS/MS). Anal. Methods 9, 3039–3048. doi:10.1039/c7ay00185a
Pang, X., Lewis, A. C., and Shaw, M. D. (2017). Analysis of Biogenic Carbonyl Compounds in Rainwater by Stir Bar Sorptive Extraction Technique with Chemical Derivatization and Gas Chromatography-Mass Spectrometry. J. Sep. Sci. 40 (3), 753–766. doi:10.1002/jssc.201600561
Płotka, J., Tobiszewski, M., Sulej, A. M., Kupska, M., Górecki, T., and Namieśnik, J. (2013). Green Chromatography. J. Chromatogr. A. 1307, 1–20. doi:10.1016/j.chroma.2013.07.099
Płotka-Wasylka, J., SzczepańskaDe la Guardia, N. M., de la Guardia, M., and Namieśnik, J. (2015). Miniaturized Solid-phase Extraction Techniques. Trac Trends Anal. Chem. 73, 19–38. doi:10.1016/j.trac.2015.04.026
Portillo-Castillo, O. J., Castro-Ríos, R., Chávez-Montes, A., González-Horta, A., Cavazos-Rocha, N., de Torres, N. H. W., et al. (2018). Developments of Solid-phase Microextraction Fiber Coatings for Environmental Pharmaceutical and Personal Care Products Analysis. Rev. Anal. Chem. 37, 20170018. doi:10.1515/revac-2017-0018
Reyes-Garcés, N., Gionfriddo, E., Gómez-Ríos, G. A., Alam, M. D., Boyacı, E., Bojko, B., et al. (2018). Advances in Solid Phase Microextraction and Perspective on Future Directions. Anal. Chem. 90 (1), 302–360. doi:10.1021/acs.analchem.7b0450
Ríos, Á., and Zougagh, M. (2016). Recent Advances in Magnetic Nanomaterials for Improving Analytical Processes. Trac Trends Anal. Chem. 84, 72–83. doi:10.1016/j.trac.2016.03.001
Roszkowska, A., Tascon, M., Bojko, B., Goryński, K., dos Santos, P. R., Cypel, M., et al. (2018). Equilibrium Ex Vivo Calibration of Homogenized Tissue for In Vivo SPME Quantitation of Doxorubicin in Lung Tissue. Talanta 183, 304–310. doi:10.1016/j.talanta.2018.02.049
Rutkowska, M., Dubalska, K., Konieczka, P., and Namieśnik, J. (2014). Microextraction Techniques Used in the Procedures for Determining Organomercury and Organotin Compounds in Environmental Samples. Molecules 19, 7581–7609. doi:10.3390/molecules19067581
Salami, F. H., and Queiroz, M. E. C. (2014). Microextraction in Packed Sorbent for Analysis of Sulfonamides in Poultry Litter Wastewater Samples by Liquid Chromatography and Spectrophotometric Detection. J. Liquid Chromatogr. Relat. Tech. 37, 2377–2388. doi:10.1080/10826076.2013.836710
Samanidou, V., Kaltzi, I., Kabir, A., and Furton, K. G. (2016). Simplifying Sample Preparation Using Fabric Phase Sorptive Extraction Technique for the Determination of Benzodiazepines in Blood Serum by High-Performance Liquid Chromatography. Biomed. Chromatogr. 30, 829–836. doi:10.1002/bmc.3615
Santos, D. M., William, M., Kookan, R., and Marchi, R. R. (2018). Solid Phase Microextraction (SPME) Fibers: In Situ Measurements of Endocrine Disrupting Chemicals in Seawater. J. Braz. Chem. Soc. 29 (4), 888–894.
Sarafraz-Yazdi, A., Amiri, A., Rounaghi, G., and Eshtiagh-Hosseini, H. (2012). Determination of Non-steroidal Anti-inflammatory Drugs in Water Samples by Solid-phase Microextraction Based Sol-Gel Technique Using Poly(ethylene Glycol) Grafted Multi-Walled Carbon Nanotubes Coated Fiber. Analytica Chim. Acta 720, 134–141. doi:10.1016/j.aca.2012.01.021
Shen, J. Y., Chang, M. S., Yang, S.-H., and Wu, G. J. (2012). Simultaneous and Rapid Determination of Triclosan, Triclocarban and Their Four Related Transformation Products in Water Samples Using SPME-HPLC-DAD. J. Liquid Chromatogr. Relat. Tech. 35 (16), 2280–2293. doi:10.1080/10826076.2011.631258
Silva, C., Cavaco, C., Perestrelo, R., Pereira, J., and Câmara, J. (2014). Microextraction by Packed Sorbent (MEPS) and Solid-phase Microextraction (SPME) as Sample Preparation Procedures for the Metabolomic Profiling of Urine. Metabolites 4, 71–97. doi:10.3390/metabo4010071
Soleimani, E., Bahrami, A., Afkhami, A., and Shahna, F. G. (2017). Determination of Urinary Trans,trans-Muconic Acid Using Molecularly Imprinted Polymer in Microextraction by Packed Sorbent Followed by Liquid Chromatography with Ultraviolet Detection. J. Chromatogr. B 1061-1062, 65–71. doi:10.1016/j.jchromb.2017.07.008
Souza, E. A. S., Risticevic, S., and Pawliszyn, J. (2013). Recent Trends in SPME Concerning Sorbent Materials, Configurations and In Vivo Applications. Trac Trends Anal. Chem. 43, 24–36. doi:10.1016/j.trac.2012.10.006
Spietelun, A., Marcinkowski, Ł., de la Guardia, M., and Namieśnik, J. (2013). Recent Developments and Future Trends in Solid Phase Microextraction Techniques towards green Analytical Chemistry. J. Chromatogr. A 1321, 1–13. doi:10.1016/j.chroma.2013.10.030
Spietelun, A., Pilarczyk, M., Kloskowski, A., and Namieśnik, J. (2010). Current Trends in Solid-phase Microextraction (SPME) Fibre Coatings. Chem. Soc. Rev. 39, 4524–4537. doi:10.1039/c003335a
Suazo, F., Vásquez, J., RetamaL, M., Ascar, L., and Giordano, A. (2017). Pharmaceutical Compounds Determination in Water Samples: Comparison between Solid Phase Extraction and Stir Bar Sorptive Extraction. J. Chil. Chem. Soc. 62 (3), 3597–3601. doi:10.4067/s0717-97072017000303597
Szultka-Mlynska, M., Pomastowski, P., and Buszewski, B. (2018). Application of Solid Phase Microextraction Followed by Liquid Chromatography-Mass Spectrometry in the Determination of Antibiotic Drugs and Their Metabolites in Human Whole Blood and Tissue Samples. J. Chromatogr. B 1086, 153–165. doi:10.1016/j.jchromb.2018.04.013
Taraji, M., Talebpour, Z., Adib, N., Karimi, S., Haghighi, F., and Haghighi, F. (2015). Determination of Carvedilol Enantiomers in Pharmaceutical Dosages by SBSE–HPLC Based on Diastereomer Formation. J. Chromatogr. Sci. 53 (8), 1316–1321. doi:10.1093/chromsci/bmv013
Tascon, M., Gomez-Rios, G. A., Reyes-Garcés, N., and Pawliszyn, J. (2017). Ultra-fast Quantitation of Voriconazole in Human Plasma by Coated Blade spray Mass Spectrometry. J. Pharm. Biomed. Anal. 144, 106–111. doi:10.1016/j.jpba.2017.03.009
Terzopoulou, Z., Papageorgiou, M., Kyzas, G. Z., Bikiaris, D. N., and Lambropoulou, D. A. (2016). Preparation of Molecularly Imprinted Solid-phase Microextraction- Fiber for the Selective Removal and Extraction of the Antiviral Drug Abacavir in Environmental and Biological Matrices. Anal. Chim. Acta 913, 63–75. doi:10.1016/j.aca.2016.01.059
Tian, J., Xu, J., Zhu, F., Lu, T., Su, C., and Ouyang, G. (2013). Application of Nanomaterials in Sample Preparation. J. Chromatogr. A. 1300, 2–16. doi:10.1016/j.chroma.2013.04.010
Vasapollo, G., Del Sole, R., Mergola, L., Lazzoi, M. R., Scardino, A., Scorrano, S., et al. (2011). Molecularly Imprinted Polymers: Present and Future Prospective. Int. J. Mol. Sci. 12, 5908–5945. doi:10.3390/ijms12095908
Vasconcelos, I., and Fernandes, C. (2017). Magnetic Solid Phase Extraction for Determination of Drugs in Biological Matrices. Trac Trends Anal. Chem. 89, 41–52. doi:10.1016/j.trac.2016.11.011
Vo Duy, S., Fayad, P. B., Barbeau, B., Prévost, M., and Sauvé, S. (2012). Using a Novel Sol-Gel Stir Bar Sorptive Extraction Method for the Analysis of Steroid Hormones in Water by Laser Diode thermal Desorption/atmospheric Chemical Ionization Tandem Mass Spectrometry. Talanta 101, 337–345. doi:10.1016/j.talanta.2012.09.036
Wang, C., Su, S., Chou, J., Huang, M., Lin, M., and Shiea, J. (2018). Solid Phase Microextraction Combined with thermal-desorption Electrospray Ionization Mass Spectrometry for High-Throughput Pharmacokinetics Assays. Analytica Chim. Acta 1021, 60–68. doi:10.1016/j.aca.2018.03.002
Yang, L., Said, R., and Abdel-Rehim, M. (2017). Sorbent, Device, Matrix and Application in Microextraction by Packed Sorbent (MEPS): A Review. J. Chromatogr. B. 1043, 33–43. doi:10.1016/j.jchromb.2016.10.044
Ye, D., Wu, S., Xu, J., Jiang, R., Zhu, F., and Ouyang, G. (2016). Rapid Determination of Clenbuterol in Pork by Direct Immersion Solid-phase Microextraction Coupled with Gas Chromatography–Mass Spectrometry. J. Chromatogr. Sci. 54 (2), 112–118. doi:10.1093/chromsci/bmv126
Yılmaz, E., Garipcan, B., Patra, H. K., and Uzun, L. (2017). Molecular Imprinting Applications in Forensic Science. Sensors 17 (4), 691. doi:10.3390/s17040691
Yuan, S. F., Liu, Z. H., Lian, H. X., Yang, C. T., Lin, Q., Yin, H., et al. (2017). Simultaneous Determination of Eleven Estrogenic and Odorous Chloro- and Bromo-Phenolic Compounds in Surface Water through an Automated Online Headspace SPME Followed by On-Fiber Derivatization Coupled with GC-MS. Anal. Methods 9, 4819e4827. doi:10.1039/c7ay00641a
Yuan, S., Liu, Z., Lian, H., Yang, C., Lin, Q., Yin, H., et al. (2017). Simultaneous Determination of Eleven Estrogenic and Odorous Chloro- and Bromo-Phenolic Compounds in Surface Water through an Automated Online Headspace SPME Followed by On-Fiber Derivatization Coupled with GC-MS. Anal. Methods 9, 4819–4827. doi:10.1039/c7ay00641a
Zambonin, C., and Aresta, A. (2021). Recent Applications of Solid Phase Microextraction Coupled to Liquid Chromatography. Separations 8, 34–48. doi:10.3390/separations8030034
Zhang, H., and Lee, H. K. (2012a). Simultaneous Determination of Ultraviolet Filters in Aqueous Samples by Plunger-In-Needle Solid-phase Microextraction with Graphene-Based Sol-Gel Coating as Sorbent Coupled with Gas Chromatography-Mass Spectrometry. Anal. Chim. Acta 742, 67–73. doi:10.1016/j.aca.2012.03.016
Keywords: solventless extraction, microextraction, SPME, green analysis, pharmaceuticals
Citation: Mohamed HM (2022) Solventless Microextration Techniques for Pharmaceutical Analysis: The Greener Solution. Front. Chem. 9:785830. doi: 10.3389/fchem.2021.785830
Received: 29 September 2021; Accepted: 13 December 2021;
Published: 13 January 2022.
Edited by:
Luca Rivoira, Department of Chemistry, School of Nature Sciences, University of Turin, ItalyReviewed by:
Maria Bruzzoniti, University of Turin, ItalyMichele Castiglioni, University of Turin, Italy, in collaboration with reviewer MB
Giulia Selvolini, University of Florence, Italy
Lapo Renai, University of Florence, Italy, in collaboration with reviewer GS
Copyright © 2022 Mohamed. This is an open-access article distributed under the terms of the Creative Commons Attribution License (CC BY). The use, distribution or reproduction in other forums is permitted, provided the original author(s) and the copyright owner(s) are credited and that the original publication in this journal is cited, in accordance with accepted academic practice. No use, distribution or reproduction is permitted which does not comply with these terms.
*Correspondence: Heba M. Mohamed, aG1vaGFtZWRAaGN0LmFjLmFl