- 1Institute of Laser Engineering, Beijing University of Technology (BJUT), Beijing, China
- 2China Electronics Standardization Institute, Beijing, China
- 3Key Laboratory of Flexible Electronics (KLOFE), Institute of Advanced Materials (IAM), Nanjing Tech University (NanjingTech), Nanjing, China
Recently, there has been remarkable progress of the host-guest doped pure organic room-temperature phosphorescence (RTP) materials. However, it remains a great challenge to develop highly efficient host-guest doping systems. In this study, we have successfully developed a heavy atom free pure organic molecular doped system (benzophenone-thianthrene, respectively) with efficient RTP through a simple host-guest doping strategy. Furthermore, by optimizing the doping ratios, the host-guest material with a molar ratio of 100:1 presented an efficient RTP emission with 46% quantum efficiency and a long lifetime of up to 9.17 ms under ambient conditions. This work will provide an effective way to design new organic doping systems with RTP.
1 Introduction
Recently, purely organic room temperature phosphorescence (RTP) materials are gaining more and more attention because of their long luminescent lifetimes, larger Stokes shift, convenient syntheses, low cost, and so on (Ma et al., 2019; Xiong et al., 2019), showing potential applications in display (Wang et al., 2019), data storage (Xu et al., 2016), encryption (Hirata, 2017), and bioimaging (Qu et al., 2019), and so on. Due to the inherent spin-forbidden and the fast-non-radiative transition of triplet excitons, it is quite hard to obtain RTP based on metal-free organic compounds. So far, in order to increase intersystem crossing (ISC) rate and to suppress non-radiative transitions, many strategies have succeeded to achieve efficient organic RTP materials, such as host-guest doping, heavy-atom effects, intermolecular electronic coupling, supramolecular self-assembly, and so forth (Yang et al., 2016; Chai et al., 2017; Cai et al., 2018; Yang et al., 2018; Shi et al., 2019; Alam et al., 2020; Lee et al., 2020; Li and Li, 2020). Among them, host-guest doping materials containing heavy atoms are an effective way to obtain efficient RTP by constructing a relatively rigid environment to restrain the nonradiative decay of the triplet state and promoting the ISC through the heavy-atom effect (Bolton et al., 2011; Kabe and Adachi, 2017; Zhang et al., 2019a; Zhang et al., 2019b; Lei et al., 2020; Liu et al., 2021a; Wang et al., 2021a). For example, Bolton et al. (2011). have developed an efficient RTP system with efficiency and lifetime up to 55% and 8.3 ms, respectively, through diluting the aldehyde chromophore into a host crystal with a similar halogen-bonding motif. Liu et al. (2021b) have developed an RTP emission of organic host-guest doped system with fluorescence and phosphorescence efficiency up to ∼67.5% and ∼13.2%, respectively, in a solid state using a phenylamine derivative containing halogen atoms as a guest and benzophenone as a host. Halogen-bonding is a crucial way to enhance the heavy-atom effect and promote spin-orbit coupling (SOC) to accelerate phosphorescence with high quantum yields in organic materials (Qu et al., 2019). However, the presence or substitution of heavy halogen atoms seriously affects the chemical stability upon thermal and electrical treatments (Zhou et al., 2018).
To avoid or overcome these shortcomings, heavy atom free host-guest interactions are an important approach in this research field. For instance, Lei et al. (2019) have developed a series of heavy-atom-free pure organic host-guest doping systems by using 4-(2-(4-(diphenylamino) -phenyl)-2-oxoethyl)benzonitrile with a donor moiety (triphenylamine, TPA) and an acceptor moiety (benzonitrile) within the same molecule as a guest, and TPA and 4-(cyanomethyl) benzonitrile as a donor host and acceptor host respectively, which lead to an enhanced fluorescence (Φ = 63∼76%) and RTP (Φ = 7.6∼14.5%, τ = 119∼317 ms) under ambient conditions. In another instance, Wang et al. (2020) have successfully developed an efficient heat-responsive RTP material with three components by utilizing N,N-dimethylpyridin-4-amine as a host and a blue RTP molecule (N,N,N,N-tetramethylbenzidine as a guest, and energy acceptor (fluorescein) with phosphorescence efficiency and lifetime of up to 13.4% and 2.08 s, respectively, through the simple host-guest doping strategy. Furthermore, Wang et al. (2021b) have also developed a series of efficient pure organic RTP materials by using phenothiazine derivatives (CzS-CH3 and CzS-C2H5) as guests and their corresponding dioxide derivatives (CS-CH3 and CS-C2H5) as hosts, showing high phosphorescence efficiency (43%) as well as a long afterglow of up to 25 min in an aqueous environment. As can be seen from the above analysis, the phosphorescence efficiency of host-guest doping materials without heavy atoms is lower than that of RTP materials containing heavy atoms. Therefore, it remains a challenge to further improve the phosphorescent quantum efficiency of RTP materials without heavy atoms.
In the present study, we have developed a heavy atom free pure organic host-guest doped material, in which thianthrene (TTR) was selected as a guest molecule and benzophenone (BPO) as a host. TTR was selected as the guest because it contains dual-heteroatom of sulfur with abundant lone-pair electrons which can effectively facilitate the SOC and further increase the intersystem crossing (Lei et al., 2019; Zhao et al., 2020). BPO was chosen as the host due to the characteristics of low melting point, good crystallinity, and phosphorescent features. According to the literature (Chen et al., 2020; Liu et al., 2021b), the host matrix is believed to play a synergistic role in energy transfer during the phosphorescence emission of the guest molecule, so the abundant lone-pair electrons of TTR are obviously beneficial for energy transfer. The low melting point of BPO (48.5°C) promotes the preparation of the doped materials by the melt-casting method, and the good crystallinity is beneficial to provide a rigid environment for the guest molecule (Chen et al., 2020). As we expected, a series of host-guest doped crystals all showed excellent room-temperature phosphorescence (RTP). Interestingly, the multi-emission (phosphorescence) peaks of individual guest (BPO) molecules nearly became a single emission peak, especially at a 100:1 molar ratio of the host-guest doped system, which presented an efficient RTP emission with 46% quantum efficiency and a long lifetime of up to 9.17 ms under ambient conditions. This work will provide an effective way to further design new organic doping systems with RTP.
2 Materials and Methods
Reagents and materials: Unless otherwise noted, all reagents used in the experiments were purchased from commercial sources without further purification. For flash column chromatography, silica gel with 200–300 mesh was used.
Measurements: The Steady-state luminescence, delayed luminescence spectra, and lifetimes were recorded on an Edinburgh Instruments LTD FLS1000 photoluminescence spectrometer, which is equipped with a xenon arc lamp, xenon flash lamp (μF2), picosecond pulsed diode laser (EPL), and picosecond pulsed led (EPLED). The delayed luminescence spectra were collected with a delay time of 1 or 8 ms. Photoluminescence efficiency was collected on a Hamamatsu Absolute PL Quantum Yield Spectrometer C11347. Luminescent photos were taken by a Canon EOS 700D camera. Powder X-Ray diffraction (XRD) patterns at room temperature were measured on an X-ray diffractometer (RIGAKU, RINT-ULTIMA III) using Cu Kα radiation (λ = 1.54051 Å). All the measurements of the photophysical properties for emissive materials are made carried out in air.
3 Results and Discussions
The molecular structures of TTR (m. p. 151–155°C) and BPO (m. p. 48.5°C) are shown in Figure 1A. Their doped systems were prepared via a melt-casting method (within a temperature range of 453 K to 395 K) by taking different molar ratios of the BPO and TTR in a vacuum. The powder X-ray diffraction (XRD) patterns (Figure 1B) reveal that there are no significant changes in the spectra of BPO from 1000:1 to 5:1 molar ratio of BPO/TTR. However, the doped powder with 1:1 molar ratio has some new peaks but there are still all the major peaks (11.2°, 15.8°, 18.3°, 20.3°, 21.7°, 22.2°, 28.1°) of BPO in the spectra. These results suggested that the host and guest molecules are homogeneously cocrystallized in the host lattice at a low doping ratio, while at a high molar ratio of BPO (1:1), both molecules are crystallized individually.
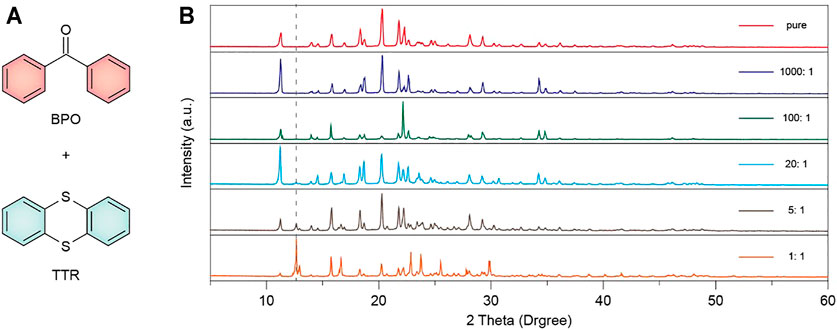
FIGURE 1. (A) Chemical structures of the host matrices BPO and guest emitter TTR. (B) Powder XRD patterns of pure BPO and BPO/TTR doped crystalline materials at different molar ratios (1000:1, 100:1, 20:1, 5:1 and 1:1 Mol %).
Next, their photophysical properties were studied through steady-state photoluminescence (PL) spectra, and time-resolved emission decay curves in solid state. Pure crystalline BPO powders showed almost identical multiple peaks for steady-state photoluminescence (PL) and phosphorescence with emission peaks at 416 nm (409.91 μs), 446 nm (409.49 μs), 479 nm (409.63 μs) and a shoulder band around 521 nm (409.79 μs) under ambient conditions (Figures 2A,B). After doping with TTR, their blend crystalline powders exhibit a new emission band at 540 nm in both steady-state PL and phosphorescence spectra with a slight red shift of pure BPO (Figure 3A). Moreover, the phosphorescence spectra of blend crystalline powders became almost a single emission band at 540 nm, indicating that the energy transfer between singlet-triplet states from BPO to TTR may occur. It can significantly improve the phosphorescence properties of the doping system. (Lei et al., 2019; Lei et al., 2020) Besides, the doped materials exhibit bright yellow emission under the UV lamp excitation at 365 nm. Moreover, from the ratio of phosphorescent intensity at 540 nm and steady-state PL intensity (416 nm and 446 nm) in the BPO/TTR systems, we can find that different doping concentrations have different influences on the photophysical properties of organic room temperature phosphorescence (Figure 3B). Notably, compared with the phosphorescence in a vacuum, the intensity of the phosphorescence emission band around 540 nm significantly decreased in an oxygen atmosphere, indicating the phosphorescence feature.
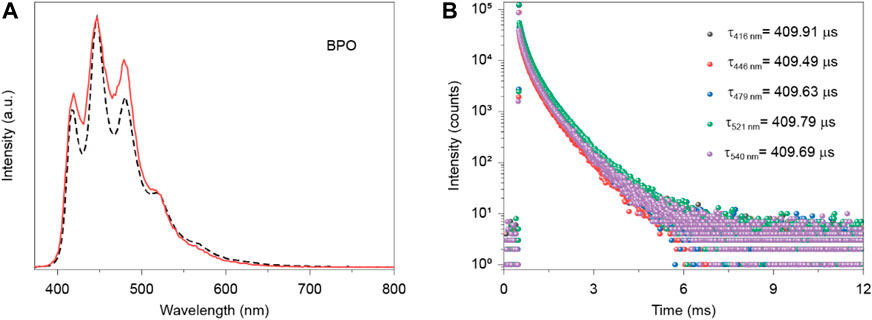
FIGURE 2. (A) Steady-state PL (dotted line) and decay emission spectra (full line) of the host BPO in crystal excited by 383 nm. Inset image show the photograph under 365 nm UV light. (B) Lifetime profiles of decay emission bands at 416, 446, 479, 521 and 540 nm for the host (BPO) excited by 396 nm.
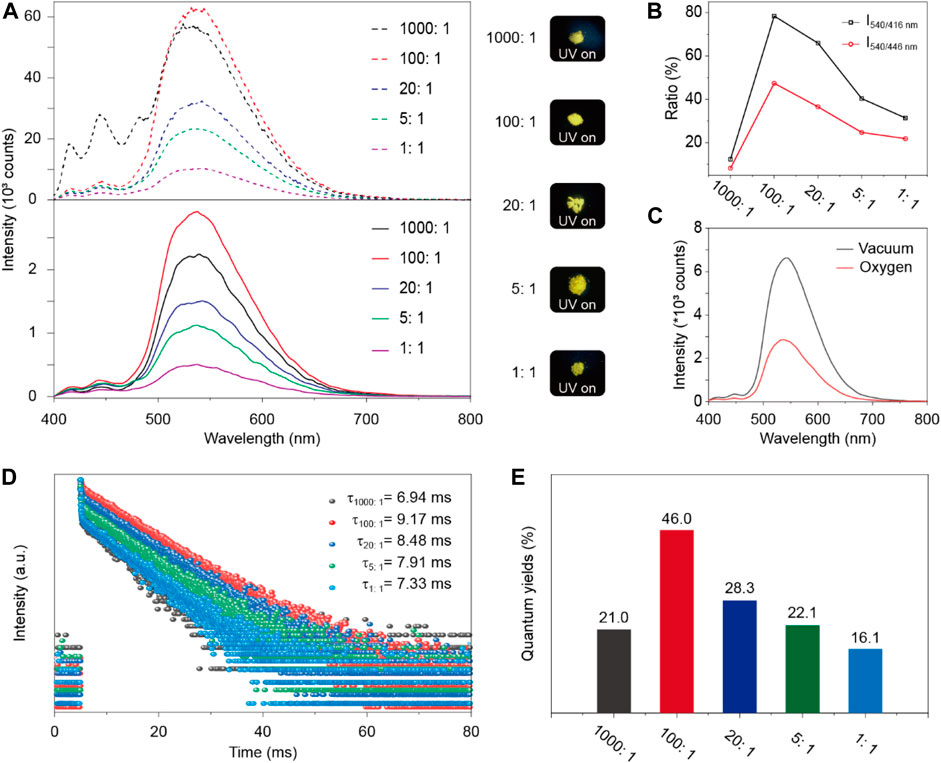
FIGURE 3. (A) Steady-state PL (dotted line) and decay emission spectra (full line) of BPO/TTR-doped crystalline powders at different molar ratios excited by 390 nm. Insets show photographs under 365 nm UV light. (B) The ratio of phosphorescent intensity at 540 nm and fluorescent intensity at 416 or 446 nm of the decay emission spectra in the BPO/TTR doping system with different doping ratios. (C) Phosphorescence spectra of BPO/TTR composite (100:1) under oxygen and vacuum atmosphere, respectively. (D) Lifetime profiles of decay emission band at 540 nm for the BPO/TTR composites excited by 390 nm. (E) Phosphorescence quantum yields of BPO/TTR-doped materials with different molar ratios of guest (1000:1, 100:1, 20:1, 5:1 and 1:1 Mol %).
It is noteworthy to mention that when guest molecules were doped into the host material with the molar ratio of 1:100, the characteristic steady-state PL and phosphorescence emission peaks (at 416, 446, 479, and 521 nm) of the host material (BPO) were reduced to their lowest level compared to other doped molar ratios (1:1000, 1:20, 1:5 and 1:1). Besides, the phosphorescence lifetime of all doped materials is higher than the individual host (BPO) at their maximum emission peak at 540 nm and the doped material with a 100:1 molar ratio showed the longest emission lifetime of 9.17 ms (Table 1 and Figure 3D). From the performance of 1:100 doped molar ratio, it is not only indicated that the doping system does not disturb the original structure of the host which provides a suitable rigid environment, but also it can form a suitable co-crystallized system that effectively facilitates electron SOC and ISC transitions of molecules, increase the rate of intersystem crossing (S1→T1) and accelerate the subsequent T1→S0 phosphorescence processes (Qu et al., 2019).
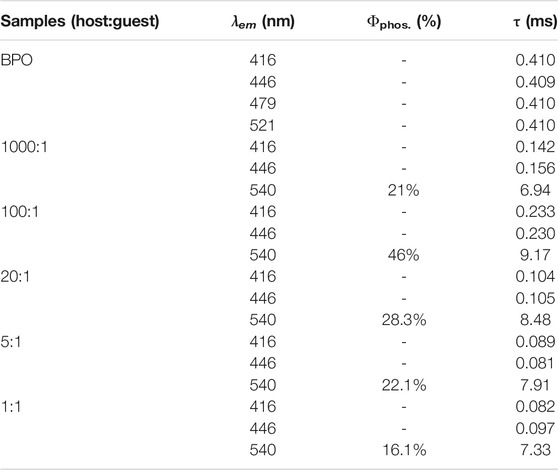
TABLE 1. Photophysical properties of the BPO/TTR-doped materials at different molar ratios of guest.
Moreover, it was surprising to find out that the characteristic peaks of host materials almost disappeared, and a new band at 540 nm appeared after doping the guest materials. This unusual phenomenon can be initially understood by the El-Sayed rule (El Sayed, 1964; Zhou et al., 2018) that the ISC process takes place more easily due to the distinct transition pattern between singlet and triplet states. The dual-heteroatom of sulfur in TTR and carboxylic oxygen in BPO are playing a similar role as that of heavy atoms, which provide the abundant lone pair electrons to strengthen SOC and to facilitate the ISC process between n→π* and π→π* transition states according to the El-Sayed rule.(El Sayed, 1964; Zhou et al., 2018)
The emission wavelength and quantum yield of phosphorescence can be tuned by optimizing the doping ratio of the guest (TTR) molecule. Among these experiments, the doped material with a molar ratio of 100:1 presented the highest phosphorescence quantum yields of up to 46% (Figure 3E), which is consistent with the declining trend of characteristic fluorescence and phosphorescence peaks of BPO, due to the spin-orbit coupling to accelerate both the S1→T1 and subsequent T1→S0 phosphorescence processes.
To gain insight into the mechanism of the highly efficient RTP for BPO/TTR composite, we conducted a series of control experiments including excitation and phosphorescence spectra of BPO, TTR, and composites, as well as luminescent lifetime change. From Figure 4A, it is easily found that the excitation spectra of the BPO and BPO/TTR doped systems were similar, which is different from that of TTR in solid state, indicating the phosphorescence emission band around 540 nm in BPO/TTR composite was related to the BPO host material. Taking the different lifetimes of the emission bands at 540 nm for BPO host and BPO/TTR composite (Figures 2B, 3D) and the phosphorescence spectrum for TTR in an isolated state (Figure 4B) together, we concluded that the phosphorescence emission around 540 nm stemmed from the isolated TTR molecules in BPO/TTR composite. It is worth noting that the lifetimes of emission bands at 446 nm gradually decrease with the ratio of TTR guests increasing (Figure 4C), suggesting that there existed energy transfer from BPO host to TTR guest molecules. To sum up, the RTP was ascribed to the phosphorescence emission of the isolated TTR molecules in crystalline BPO/TTR composite (Figure 4D). Both crystalline molecular environment and efficient energy transfer between host and guest molecules significantly boost the phosphorescence for high efficiency.
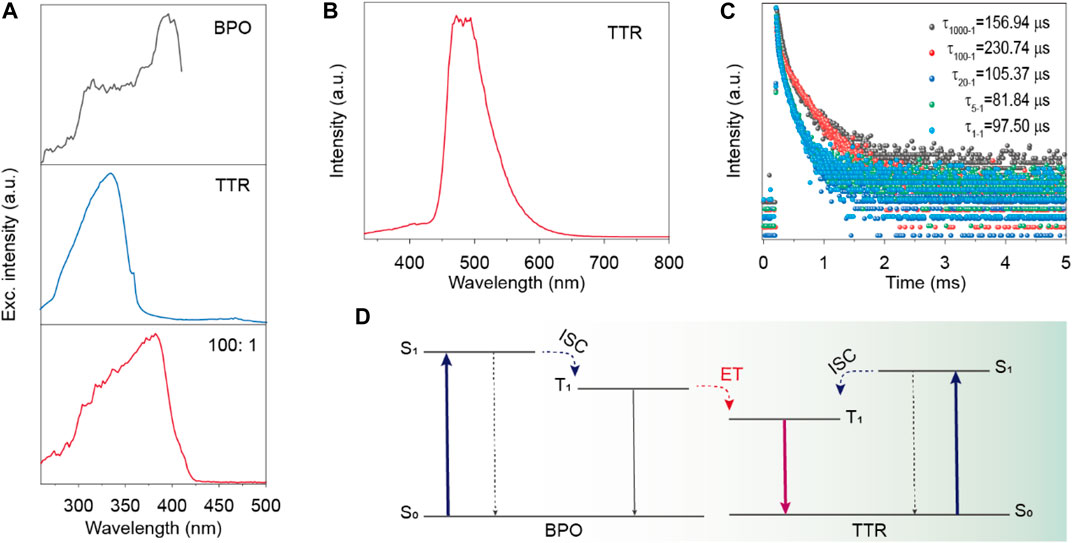
FIGURE 4. (A) Excitation spectra of the BPO, TTR and BPO/TTR composite (1000:1) in solid state under ambient conditions. (B) Phosphorescence spectrum of the TTR molecules in dilute m-THF solution at 77 K. (C) Lifetime profiles of decay emission band at 446 nm for the BPO/TTR composite excited by 390 nm. (D) A plausible mechanism for the RTP in BPO/TTR composite.
4 Conclusion
In conclusion, we have developed an efficient RTP doping material based on two heavy atom free organic small molecules, benzophenone and thianthrene. The doped materials have contained different heteroatoms (TTF contains dual S atoms and BPO contains O atom) with abundant lone pair electrons, which make them promising candidates to enhance SOC, leading to an efficient ISC in pure organic metal-free RTP materials, instead of commonly used heavy atoms. We achieved an efficient RTP emission with phosphorescence efficiency of up to 46% under ambient conditions by tuning the doping molar ratio of the host and guest molecules. Our work not only provides a new approach to develop heavy atom free pure organic host-guest doped RTP materials but also expands the methods to enhance their quantum efficiency.
Data Availability Statement
The original contributions presented in the study are included in the article/supplementary material, further inquiries can be directed to the corresponding author.
Author Contributions
JC and MZ conducted the experiments. HS and JC designed the project and wrote the manuscript, MS and ZA revised the manuscript. All the authors discussed the results.
Funding
This work has been financially supported by the National Natural Science Foundation of China under Grants 11674018 and 21975120.
Conflict of Interest
The authors declare that the research was conducted in the absence of any commercial or financial relationships that could be construed as a potential conflict of interest.
Publisher’s Note
All claims expressed in this article are solely those of the authors and do not necessarily represent those of their affiliated organizations, or those of the publisher, the editors and the reviewers. Any product that may be evaluated in this article, or claim that may be made by its manufacturer, is not guaranteed or endorsed by the publisher.
Acknowledgments
Special thanks to Beijing University of Technology and Nanjing Tech University for their technical help.
References
Alam, P., Leung, N. L. C., Liu, J., Cheung, T. S., Zhang, X., He, Z., et al. (2020). Two Are Better Than One: A Design Principle for Ultralong‐Persistent Luminescence of Pure Organics. Adv. Mater. 32, 2001026. doi:10.1002/adma.202001026
Bolton, O., Lee, K., Kim, H.-J., Lin, K. Y., and Kim, J. (2011). Activating Efficient Phosphorescence from Purely Organic Materials by crystal Design. Nat. Chem. 3, 205–210. doi:10.1038/nchem.984
Cai, S., Shi, H., Tian, D., Ma, H., Cheng, Z., Wu, Q., et al. (2018). Enhancing Ultralong Organic Phosphorescence by Effective π-Type Halogen Bonding. Adv. Funct. Mater. 28, 1705045. doi:10.1002/adfm.201705045
Chai, Z., Wang, C., Wang, J., Liu, F., Xie, Y., Zhang, Y.-Z., et al. (2017). Abnormal Room Temperature Phosphorescence of Purely Organic boron-containing Compounds: the Relationship between the Emissive Behaviorand the Molecular Packing, and the Potential Related Applications. Chem. Sci. 8, 8336–8344. doi:10.1039/c7sc04098a
Chen, Y., Xie, Y., Shen, H., Lei, Y., Zhou, Y., Dai, W., et al. (2020). Tunable Phosphorescence/Fluorescence Dual Emissions of Organic Isoquinoline‐Benzophenone Doped Systems by Alkoxy Engineering. Chem. Eur. J. 26, 17376–17380. doi:10.1002/chem.202004291
El Sayed, M. A. (1964). Vanishing First‐ and Second‐Order Intramolecular Heavy‐Atom Effects on the (π*→n) Phosphorescence in Carbonyls. J. Chem. Phys. 41, 2462–2467. doi:10.1063/1.1726288
Hirata, S. (2017). Recent Advances in Materials with Room-Temperature Phosphorescence: Photophysics for Triplet Exciton Stabilization. Adv. Opt. Mater. 5, 1700116. doi:10.1002/adom.201700116
Kabe, R., and Adachi, C. (2017). Organic Long Persistent Luminescence. Nature 550, 384–387. doi:10.1038/nature24010
Lee, D. R., Lee, K. H., Shao, W., Kim, C. L., Kim, J., and Lee, J. Y. (2020). Heavy Atom Effect of Selenium for Metal-free Phosphorescent Light-Emitting Diodes. Chem. Mater. 32, 2583–2592. doi:10.1021/acs.chemmater.0c00078
Lei, Y., Dai, W., Tian, Y., Yang, J., Li, P., Shi, J., et al. (2019). Revealing Insight into Long-Lived Room-Temperature Phosphorescence of Host-Guest Systems. J. Phys. Chem. Lett. 10, 6019–6025. doi:10.1021/acs.jpclett.9b02411
Lei, Y., Dai, W., Guan, J., Guo, S., Ren, F., Zhou, Y., et al. (2020). Wide‐Range Color‐Tunable Organic Phosphorescence Materials for Printable and Writable Security Inks. Angew. Chem. Int. Ed. 59, 16054–16060. doi:10.1002/anie.202003585
Li, Q., and Li, Z. (2020). Molecular Packing: Another Key point for the Performance of Organic and Polymeric Optoelectronic Materials. Acc. Chem. Res. 53, 962–973. doi:10.1021/acs.accounts.0c00060
Liu, X., Pan, Y., Lei, Y., Liu, N., Dai, W., Liu, M., et al. (2021). Influence of Guest/host Morphology on Room Temperature Phosphorescence Properties of Pure Organic Doped Systems. J. Phys. Chem. Lett. 12, 7357–7364. doi:10.1021/acs.jpclett.1c01893
Liu, X., Dai, W., Junjie, Q., Lei, Y., Liu, M. C., Cai, Z., et al. (2021). Pure Room Temperature Phosphorescence Emission of Organic Host-Guest Doped System with Quantum Efficiency of 64%. J. Mater. Chem. C. 9, 3391–3395. doi:10.1039/D1TC00403D
Ma, X., Wang, J., and Tian, H. (2019). Assembling-induced Emission: an Efficient Approach for Amorphous Metal-free Organic Emitting Materials with Room-Temperature Phosphorescence. Acc. Chem. Res. 52, 738–748. doi:10.1021/acs.accounts.8b00620
Qu, G., Zhang, Y., and Ma, X. (2019). Recent Progress on Pure Organic Room Temperature Phosphorescence Materials Based on Host-Guest Interactions. Chin. Chem. Lett. 30, 1809–1814. doi:10.1016/j.cclet.2019.07.042
Shi, H., Song, L., Ma, H., Sun, C., Huang, K., Lv, A., et al. (2019). Highly Efficient Ultralong Organic Phosphorescence through Intramolecular-Space Heavy-Atom Effect. J. Phys. Chem. Lett. 10, 595–600. doi:10.1021/acs.jpclett.8b03712
Wang, D., Wang, X., Xu, C., and Ma, X. (2019). A Novel Metal-free Amorphous Room-Temperature Phosphorescent Polymer without Conjugation. Sci. China Chem. 62, 430–433. doi:10.1007/s11426-018-9383-2
Wang, Y., Yang, J., Gong, Y., Fang, M., Li, Z., and Tang, B. Z. (2020). Host-guest Materials with Room Temperature Phosphorescence: Tunable Emission Color and thermal Printing Patterns. SmartMat 1006, 1–9. doi:10.1002/smm2.1006
Wang, D., Xie, Y., Wu, X., Lei, Y., Zhou, Y., Cai, Z., et al. (2021). Excitation-Dependent Triplet-Singlet Intensity from Organic Host-Guest Materials: Tunable Color, White-Light Emission, and Room-Temperature Phosphorescence. J. Phys. Chem. Lett. 12, 1814–1821. doi:10.1021/acs.jpclett.1c00188
Wang, Y., Gao., H., Yang, J., Fang, M., Ding, D., Tang, B. Z., et al. (2021). High Performance of Simple Organic Phosphorescence Host-Guest Materials and Their Application in Time‐Resolved Bioimaging. Adv. Mater. 33, 2007811. doi:10.1002/adma.202007811
Xiong, Q., Xu, C., Jiao, N., Ma, X., Zhang, Y., and Zhang, S. (2019). Pure Organic Room-Temperature Phosphorescent N-Allylquinolinium Salts as Anti-counterfeiting Materials. Chin. Chem. Lett. 30, 1387–1389. doi:10.1016/j.cclet.2019.04.010
Xu, S., Chen, R., Zheng, C., and Huang, W. (2016). Excited State Modulation for Organic Afterglow: Materials and Applications. Adv. Mater. 28, 9920–9940. doi:10.1002/adma.201602604
Yang, Z., Mao, Z., Zhang, X., Ou, D., Mu, Y., Zhang, Y., et al. (2016). Intermolecular Electronic Coupling of Organic Units for Efficient Persistent Room-Temperature Phosphorescence. Angew. Chem. 128, 2221–2225. doi:10.1002/ange.201509224
Yang, J., Zhen, X., Wang, B., Gao, X., Ren, Z., Wang, J., et al. (2018). The Influence of the Molecular Packing on the Room Temperature Phosphorescence of Purely Organic Luminogens. Nat. Commun. 9, 840. doi:10.1038/s41467-018-03236-6
Zhang, Z. Y., Chen, Y., and Liu, Y. (2019). Efficient Room‐Temperature Phosphorescence of a Solid‐State Supramolecule Enhanced by Cucurbit[6]uril. Angew. Chem. Int. Ed. 58, 6028–6032. doi:10.1002/anie.201901882
Zhang, X., Du, L., Zhao, W., Zhao, Z., Xiong, Y., He, X., et al. (2019). Ultralong UV/mechano-excited Room Temperature Phosphorescence from Purely Organic Cluster Excitons. Nat. Commun. 10, 5161. doi:10.1038/s41467-019-13048-x
Zhao, W., He, Z., and Tang, B. Z. (2020). Room-temperature Phosphorescence from Organic Aggregates. Nat. Rev. Mater. 5, 869–885. doi:10.1038/s41578-020-0223-z
Keywords: host-guest doping, heavy atom free, room temperature phosphorescence (RTP), triplet state, nonradiative transition
Citation: Cao J, Zhang M, Singh M, An Z, Ji L, Shi H and Jiang Y (2021) Highly Efficient Heavy Atom Free Room Temperature Phosphorescence by Host-Guest Doping. Front. Chem. 9:781294. doi: 10.3389/fchem.2021.781294
Received: 22 September 2021; Accepted: 01 November 2021;
Published: 23 November 2021.
Edited by:
Wang Zhang Yuan, Shanghai Jiao Tong University, ChinaCopyright © 2021 Cao, Zhang, Singh, An, Ji, Shi and Jiang. This is an open-access article distributed under the terms of the Creative Commons Attribution License (CC BY). The use, distribution or reproduction in other forums is permitted, provided the original author(s) and the copyright owner(s) are credited and that the original publication in this journal is cited, in accordance with accepted academic practice. No use, distribution or reproduction is permitted which does not comply with these terms.
*Correspondence: Huifang Shi, iamhfshi@njtech.edu.cn
†These authors have contributed equally to this work and share first authorship