Erratum: Meroterpenoids From Ganoderma lucidum Mushrooms and Their Biological Roles in Insulin Resistance and Triple-Negative Breast Cancer
- 1Institute for Inheritance-Based Innovation of Chinese Medicine, School of Pharmaceutical Sciences, Health Science Center, Shenzhen University, Shenzhen, China
- 2Institute of Microscale Optoelectronics, Shenzhen University, Shenzhen, China
- 3Guangdong Key Laboratory for Functional Substances in Medicinal Edible Resources and Healthcare Products, School of Life Sciences and Food Engineering, Hanshan Normal University, Chaozhou, China
Ganoderma fungi as popular raw materials of numerous functional foods have been extensively investigated. In this study, five pairs of meroterpenoid enantiomers beyond well-known triterpenoids and polysaccharides, dayaolingzhiols I−M (1–5), were characterized from Ganoderma lucidum. Their structures were identified using spectroscopic and computational methods. Structurally, compound 1 features a novel dioxabicyclo[2.2.2]octan-3-one motif in the side chain. Ethnoknowledge-derived biological evaluation found that (+)-5 could activate Akt and AMPK phosphorylation in insulin-stimulated C2C12 cells, and (+)-5 could activate glucose uptake dose dependently in C2C12 cells. Furthermore, we found that (+)-1 (+)-4, and (–)-4 could significantly inhibit cell migration of the MDA-MB-231 cell line, of which (+)-4 showed significant inhibitory effects against cell migration of the MDA-MB-231 cell line in a dose-dependent manner. These findings revealed the meroterpenoidal composition of G. lucidum and its roles in the prevention of chronic diseases such as diabetes mellitus and triple-negative breast cancer.
Introduction
Insulin resistance (IR) is defined as an impaired biological response to insulin stimulation of target tissues mainly including the liver, muscle, and adipose tissue. Insulin resistance syndrome, also known as metabolic syndrome, is associated with a broad spectrum of diseases such as obesity, hyperglycemia, hypertension, dyslipidemia, non-alcoholic fatty liver disease, cardiovascular disease, polycystic ovarian syndrome, and type 2 diabetes mellitus (Allahbadia and Merchant, 2008; Willard et al., 2016; Cortés-Rojo et al., 2020; García-Carretero et al., 2021). Insulin resistance is mainly related to excess body fat and also genetic causes, which affects as many as one in three Americans and becomes a tremendous burden for the healthcare system of the United States (Balasubramanyam, 2006; McCarthy, 2014; Sharma et al., 2019; Rajesh and Sarkar, 2021). Now, the incidence of insulin resistance is growing at an alarming speed, and it is actually becoming a non-ignorable public concern worldwide. Accumulating evidence revealed that the inactivation of Akt and the activation of Foxo1 via inhibiting insulin receptor substrate 1 (IRS1) and insulin receptor substrate 2 (IRS2) might act as the underlying mechanism of metabolic syndromes (Guo, 2014). Targeting the pivotal molecules such as IRS or Akt or Foxo1 in the signaling cascade will therefore be a precise strategy for the intervention of insulin resistance–related diseases (Guo, 2014).
Breast cancer is still the most common cancer among women (Chowdhury et al., 2021; Loibl et al., 2021). The incidence rate accounts for about 30%, and the mortality rate is as high as 15% (Loibl et al., 2021). Triple-negative breast cancer (TNBC) is one of the subgroups of breast cancer which has an incidence of 15–20% and many characteristics such as invasive, resistant, and rapid growth rate (Chen et al., 2021; Chowdhury et al., 2021). Due to its aggressive behavior, metastasis usually occurs in the liver, lungs, and brain (Chen et al., 2021; Chowdhury et al., 2021). Discovering some potential molecules which could inhibit cell migration of TNBC may provide some enlightenment for its treatment.
Ganoderma fungi, well-known mushrooms of numerous functional foods, have received long-term attention worldwide. For example, 28785 documents are related to Ganoderma when searched using SciFinder till August 29, 2021. The taste and functional components of the Ganoderma fungus lead to its applications as food additives, raw materials, dietary supplements and remedies, even in Western countries (Leskosek-Cukalovic et al., 2010; Veljović et al., 2019; Krobthonga and Yingchutrakul, 2020). Polysaccharides and triterpenoids have long been considered the major chemical compositional elements of Ganoderma, whereas the other chemical composition remains largely unknown. In recent years, a number of meroterpenoids in Ganoderma have been reported (Jiang et al., 2021), representing a new research trend for Ganoderma metabolites. Phenol and terpene moieties are considered biologically active, and Ganoderma meroterpenoids possess both groups (Lu et al., 2007; Tan et al., 2018). We have focused on the investigation of Ganoderma meroterpenoids since 2009. As a result, many structurally intriguing and biologically important meroterpenoids have been characterized by us (Yan et al., 2019; Dai et al., 2020; Qin et al., 2020; Zhang et al., 2020). Due to considerable economic values, Ganoderma species have been cultivated in several places of our country. The concept of “one strain many compounds (OSMAC)” has been widely accepted in the related field. Ganoderma belongs to a higher fungal family; hence, it is necessary to gain an insight into the chemical profiling of Ganoderma meroterpenoids of Ganoderma lucidum produced in different locations, despite that much work has been carried out on this fungal species. G. lucidum, cultivated at Dayao County of Yunnan Province, is locally dictated to have distinct effects on diabetes, which thus inspired our interest. G. lucidum was reported to have antitumor effects, and triterpenoids are generally regarded as its antitumor ingredients. Given the increasing number of Ganoderma meroterpenoids being discovered, it is worth being concerned whether they have a role in tumor treatment. As a consequence, this study afforded five pairs of meroterpenoid enantiomers, dayaolingzhiol I−M (1–5), with biological activities toward insulin resistance and metastasis of TNBC. Herein, we describe their isolation, structure characterization, and biological evaluation.
Results and Discussion
Dayaolingzhiol I (1) (Figure 1) was isolated as yellowish gums, and its molecular formula was assigned as C21H26O6 (nine degrees of unsaturation) by the analysis of its HRESIMS, 13C NMR, and DEPT spectra. The 1H NMR spectrum (Table 1) shows a typical ABX spin system [δH 7.27 (1H, d, J = 2.9 Hz, H-3), 7.00 (1H, dd, J = 8.9, 2.9 Hz, H-5), and 6.78 (1H, d, J = 8.9 Hz, H-6)], suggesting the presence of a 1,2,4-trisubstituted benzene ring. The 13C NMR and DEPT (Table 1) spectra show three methyl, five sp3 methylene, one oxygenated sp3 methine (δC 81.1), four sp2 methine, eight non-protonated carbons (one ketonic carbonyl at δC 203.8, one ester carbonyl at δC 173.9, and four oxygenated quaternary carbons). The planar structure of 1 was mainly constructed by the results of 2D NMR experiments. The 1H–1H COSY spectrum (Figure 2) shows correlations of H2-11/H2-12/H-13 and H2-16/H2-17/H-18. The HMBC correlations of H-3, H2-8/C-7 (δC 203.8) indicate that the ketonic carbonyl C-7 is connected with C-2; the HMBC correlations (Figure 2) of H2-8, Ha-11, H-13 (δH 4.55)/C-10 (δC 173.9), and H2-8/C-9 (δC 74.4), C-11 (δC 27.8) imply that C-9 is connected with C-8, C-10, and C-11 by carbon bonds, while C-10 and C-13 (δC 81.1) are connected via oxygen bridges. These data show that the six-membered lactone ring is formed in 1. In addition, the HMBC correlations of H3-15 (δH 1.24)/C-13, C-14 (δC 78.6), C-16, and H2-16 (δH 1.53)/C-13 imply that C-14 is connected with C-13, C-14, and C-15 through carbon bonds, H2-17/C-19 (δC 133.0), H3-20/C-18 (δC 124.8), C-19 (δC 133.0), C-21, and H3-21/C-18 indicate that C-18 and C-19 are connected via a double bond, and two terminal methyl are attached to C-19. The ROESY correlations (in DMSO-d6) (Figure 2) of 1-OH (δH 11.06)/H-6 (δH 6.79) and 4-OH (δH 9.14)/H-3 (δH 7.16), H-5 (δH 6.96) indicate the presence of 1-OH and 4-OH groups. One benzene ring, one six-membered lactone ring, one ketone group, and one double bond account for eight degrees of unsaturation, and the remaining one degree of unsaturation, in consideration of the diagnostic chemical shift for C-9 (δC 74.4) and C-14 (δC 78.6), allows to conclude the presence of an ether bond formed by C-9 and C-14. Therefore, the planar structure of 1 was deduced. The relative configuration of 1 was assigned by the ROESY experiments. The ROESY correlation (Figure 2) of Ha-12/H2-16 indicates the relative configuration of 1 as 9S*,13R*,14S*. To further confirm this structure, the density functional theory (DFT) at the B3LYP/6–311g(d,p) level was used to calculate chemical shifts of carbon atoms in the deduced structure with different relative configurations as 9S*,13R*,14S* (1–1) and 9R*,13S*,14S* (1–2), as well as the ether linkage broken structures of 1 with the relative configurations as 9R*,13S*,14R* (1–3); 9R*,13S*,14S* (1–4); 9R*,13R*,14R* (1–5); and 9R*,13R*,14S* (1–6) (Supplementary Figure S42). By the analysis of 13C NMR calculations, it is shown that the correlation coefficient (R2) for calculated vs experimental chemical shifts in 1–1 to 1–6 were 0.99928, 0.99927, 0.99570, 0.99694, 0.99771, and 0.99776, respectively (Supplementary Figure S49), and the corrected mean absolute error (CMAE) values were 1.2, 1.2, 2.8, 2.5, 2.2, and 2.1 ppm (Supplementary Table S7–12). These values suggest that structural and stereochemical assignments to these substances are highly dependable. Moreover, the results of DP4+ probability analysis (Supplementary Figure S50) indicated a 100% matching degree between the experimental values and the calculated values of 1–1, which further confirmed the correctness of the deduced structure and its stereochemistry. To clarify the absolute configuration of 1, which is an enantiomeric isomer, ECD calculations at the PBE1PBE/def2SVP level and B3LYP/6–31(d,p) level were carried out. It was found that the ECD spectrum of (9S,13R,14S)-1 matches well with the experimental one of (–)-1 (Figure 3 and Supplementary Figure S51). Similarly, the calculated specific optical rotation for (9S,13R,14S)-1 was –4.14 (Supplementary Table S13), which is closer to the experimental one of (–)-1 (
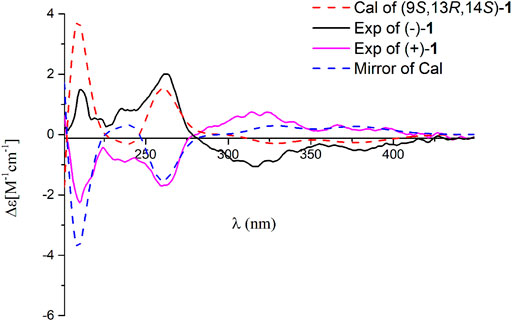
FIGURE 3. Comparison of the calculated ECD spectrum of 1 at the PBE1PBE/def2SVP level with the experimental one in MeOH. σ = 0.25 eV; shift = 13 nm.
Dayaolingzhiol J (2) was obtained as yellowish gums. It has a molecular formula C22H30O5 deduced by its HRESIMS, 13C NMR, and DEPT spectra. The 1H NMR spectrum of 2 (Table 1) shows a typical ABX spin system [(δH 6.92, d, J = 2.5 Hz, H-3; δH 6.72, dd, J = 8.8, 2.5 Hz, H-5; and δH 7.18, d, J = 8.8 Hz, H-6)]. The 13C NMR and DEPT spectra show four methyl (one oxygenated), four methylene, six methine (five sp2 and one sp3), and eight non-protonated carbons (one carbonyl and three oxygenated quaternary carbons). These data are similar to those of ganofuran B (Adams et al., 2010). The only difference is that a methoxy group appears at C-7 in 2 supported by the HMBC correlations (Figure 2) of -OCH3 (δH 3.90)/C-7 (δC 141.1). The significant ROESY correlation of H2-11 (δH 2.00)/H3-20 (δH 1.52) indicates that the Δ12(13) double bond is E form. Compound 2 was isolated as a racemic mixture, and chiral HPLC was used to afford (+)-2 and (–)-2. In general, it is a great challenge to assign the absolute configuration at C-9 in the side chain. In this case, computational methods such as ECD calculations at the APFD/6–311+g(2d,p) and B3LYP/6–31(d,p) level were carried out (Figure 4 and Supplementary Figure S52). The results reveal that the ECD spectrum of (9S)-2 matches well with the experimental one of (–)-2. Thus, the absolute configurations of the two enantiomers were assigned as 9R for (+)-2 and 9S for (–)-2, respectively. In this way, the structure of 2 was deduced and named dayaolingzhiol J.
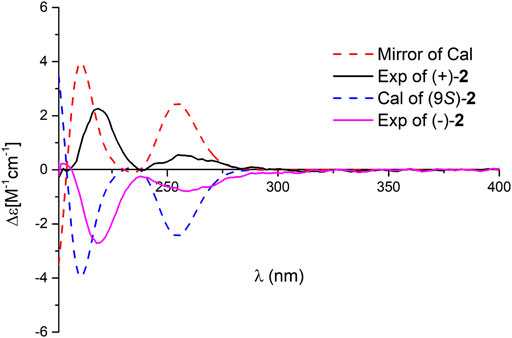
FIGURE 4. Comparison of the calculated ECD spectrum of 2 at the APFD/6–311+ g (2 d,p) level with the experimental one in MeOH. σ = 0.25 eV; shift = −25 nm.
Dayaolingzhiol K (3) has the molecular formula C21H28O5 (eight degrees of unsaturation) on the basis of its HRESIMS, 13C NMR, and DEPT spectra. The 1H NMR spectrum (Table 2) shows a typical ABX spin system [δH 6.46 (1H, d, J = 2.9 Hz, H-3), 6.61 (1H, dd, J = 8.7, 2.9 Hz, H-5), 6.68 (1H, d, J = 8.7 Hz, H-6)]. The 13C NMR and DEPT spectra show four methyl, five methylene, five sp2 methine, one oxygenated sp3 methine, and seven non-protonated carbons (one ester carbonyl and three oxygenated quaternary carbons). These data resemble those of ganomycin K (Niedermeyer et al., 2013), differing in that the OH-18 in ganomycin K is reduced in compound 3. This alteration is supported by the 1H–1H COSY correlation of H2-16/H2-17/H2-18 and the HMBC correlations of H2-16 (δH 1.96)/C-18 (δC 44.3) and H3-21 (δH 1.15)/C-18 (δC 44.3). Moreover, the significant ROESY correlations of H2-12 (δH 2.30)/H3-15 (δH 1.58) and H-13 (δH 5.14)/H2-16 (δH 1.96) indicate that the Δ13(14) double bond is E form. Compound 3 was isolated as a racemic mixture, and chiral HPLC was used to afford (+)-3 and (–)-3. By carefully comparing the CD spectra of (+)-3 and (–)-3 with those of (+)- and (–)-zizhine A (Cao et al., 2016), the absolute configurations of the two enantiomers were assigned as R for (+)-3 and S for (–)-3, respectively. Thus, the structure of 3 was deduced and named dayaolingzhiol K.
Dayaolingzhiol L (4) was obtained as yellowish gums, and its molecular formula was assigned as C22H30O5 by analysis of its HRESIMS, 13C NMR, and DEPT spectra. The 1H NMR spectrum of 4 (Table 2) shows a typical ABX spin system (δH 7.25, d, J = 2.9 Hz, H-3; δH 7.01, dd, J = 8.9, 2.9 Hz, H-5; δH 6.79, d, J = 8.9 Hz, H-6), suggesting the presence of a 1,2,4-trisubstituted benzene ring. The 13C NMR and DEPT spectra show four methyl (one oxygenated), five methylene, six methine (five sp2 and one sp3), and seven non-protonated carbons (one ketone and one carbonyl). These data are similar to those of fornicin C (Niu et al., 2006). The only difference is a methyl ester in 4, instead of the free carboxyl group in fornicin C, supported by the HMBC correlations (Figure 2) of -OCH3 (δH 3.68)/C-7 (δC 177.7). The ROESY correlation of H2-12 (δH 2.08)/H3-15 (δH 1.61) indicates that the Δ13(14) double bond is E form. Compound 4 is also a racemic mixture, and the absolute configurations of the two enantiomers after chiral separation by HPLC were assigned as R for (+)-4 and S for (–)-4, respectively, by comparison of their CD spectra with those of (+)− and (–)−applanatumol S (Luo et al., 2016). The structure of 4 was thus determined and named dayaolingzhiol L.
Dayaolingzhiol M (5) was isolated as yellow gums, and it bears the same carbon skeleton with that of 4 by careful analysis of their NMR spectra. The 1H NMR spectrum of 5 (Table 3) shows a typical ABX spin system (δH 7.22, d, J = 2.9 Hz, H-3; δH 7.01, dd, J = 8.9, 2.9 Hz, H-5; δH 6.79, d, J = 8.9 Hz, H-6). The 13C NMR and DEPT spectra show three methyl, five methylene, five sp2 methine, and eight non-protonated carbons (one ketone, one carbonyl, and three oxygenated quaternary carbons). These data also resemble those of fornicin C (Niu et al., 2006). Differently, a hydroxyl is attached to C-9 (δC 75.8) in 5, gaining support from the HMBC correlations of H2-8 and H2-11/C-9. The ROESY correlation of H2-12 (δH 2.23)/H3-15 (δH 1.62) indicates that the Δ13(14) double bond is E form. Compound 5 is also a racemate, which was further purified by chiral HPLC to afford (+)-5 and (–)-5. To clarify their absolute configurations, ECD calculation was used at the PBE1PBE/def2SVP level and B3LYP/6–31(d,p) level (Figure 5 and Supplementary Figure S53). It was found that the ECD spectrum of (9S)-5 matches well with the experimental one of (+)-5 (Figure 5); thus, the absolute configurations of the two enantiomers were assigned as 9S for (+)-5 and 9R for (–)-5, respectively. Therefore, the structure of 5, named dayaolingzhiol M, was deduced.
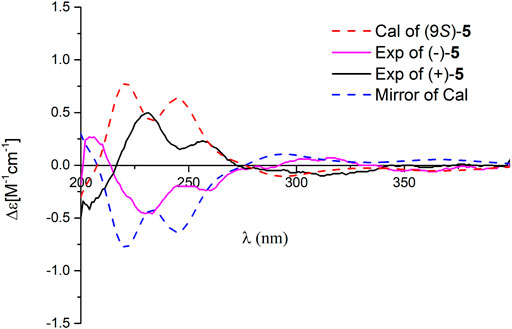
FIGURE 5. Comparison of the calculated ECD spectrum of 5 at the PBE1PBE/def2SVP level with the experimental one in MeOH. σ = 0.25 eV; shift = 0 nm.
In this experiment, we obtained five long-chain meroterpenoids dayaolingzhiols I−M (1–5). Among them, dayaolingzhiol I (1) is a relatively rare meroterpenoid with dioxabicyclo[2.2.2]octan-3-one motif of G. lucidum. At the same time, dayaolingzhiol J (2) belongs to the benzofuran type of meroterpenoid, and few benzofuran type of meroterpenoids have been reported from Ganoderma so far (Adams et al., 2010). These two pairs of meroterpenoids together with the remaining three pairs of new meroterpenoids reveal the structural diversity of Ganoderma meroterpenoids.
Ganoderma lucidum cultivated at Dayao County of Yunnan Province is locally used as a health-care product with a pronounced effect on diabetes. To explore the protective effect of Ganoderma meroterpenoids against IR, insulin exposed C2C12 myotubes were used. C2C12 cells were pretreated with different compounds for 24 h, and cell viability was not decreased at 20 μM. The CCK-8 assay showed no obvious cytotoxicity of compounds, except (+)-4 and (–)-4 (Supplementary Figure S54). When the cells differentiated into myotubes, they were pretreated with 100 nM insulin for 24 h to mimic the IR model and then exposed to 20 μM compounds for an additional 24 h. In the cellular model of IR, insulin-stimulated glucose uptake and the expression of phospho-adenosine monophosphate–activated protein kinase (p-AMPK) and phospho-AKT (p-AKT) were lower than those in control cells. The results showed that incubation with (+)-1, (–)-4, and (–)-5 caused an increase in the phosphorylation of AMPK in IR C2C12 cells after insulin stimulation. At the same time, compound (+)-5 treatment caused an increase in protein expression of p-AMPK and p-AKT in IR C2C12 cells (Figure 6A−C). Further glucose uptake experiments showed that compound (+)-5 improved the capacity of glucose uptake in IR cells in a concentration-dependent manner (Figure 6D). So far, some meroterpenoids were found to have beneficial effects to insulin sensitivity in PA-induced C2C12 cells, such as ganomycin C and ganodercin D (Qin et al., 2021). Ganomycin I exhibited potent insulin-sensitizing effects in KK-Ay mice, and its analog (R,E)-5-(4-(tert-butyl)phenyl)-3-(4,8-dimethylnona-3,7-dien-1-yl)furan-2(5H)-one ameliorates insulin resistance (Wang et al., 2017; Wang et al., 2018). These meroterpenoids with long side chains showed advantages in the amelioration of insulin resistance.
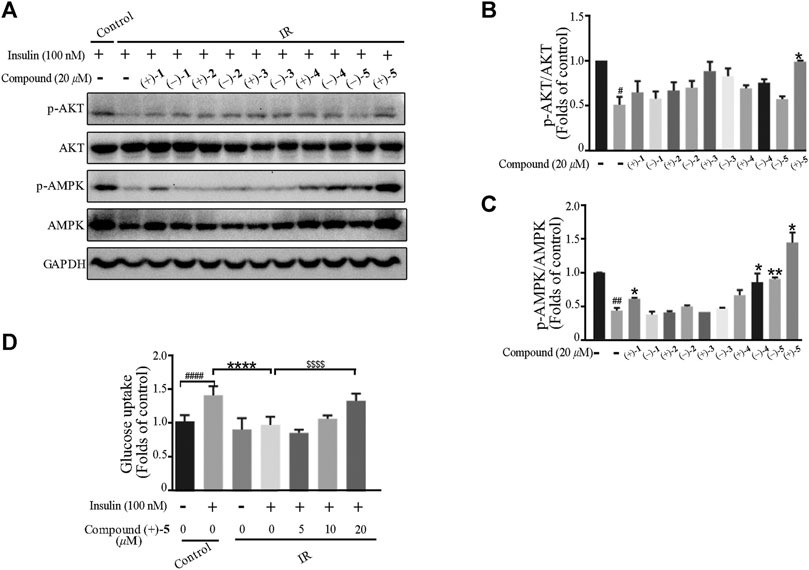
FIGURE 6. Effects of the compounds on insulin-induced insulin resistance in myotubes. (A–C) Compounds attenuated insulin signal pathway disruption. Bar graphs show the quantification of the indicated proteins. #p < 0.05 and ##p < 0.01 compared with without insulin treatment control; *p < 0.05 and **p < 0.01 compared with insulin treatment alone. (D) Compound (+)-5 improved insulin-stimulated glucose uptake in myotubes. ####p < 0.0001 compared with without insulin-stimulated control; ****p < 0.0001 compared with the insulin treatment group; $$$$p < 0.0001 compared with insulin treatment alone.
Our previous study showed that Ganoderma meroterpenoids with long side chains are active toward breast cancer cell migration (Cai et al., 2021). In this article, 10 optically active compounds, all possessing a long side chain, were tested for their suppressive activity in the triple-negative breast cancer cell line (MDA-MB-231) by using cell viability and cell migration assays. First, the cell viability assay was carried out in the MDA-MB-231 cell line. As shown in Figure 7A, compounds demonstrated weak effect on cell viability at the concentration of 20 μM. Wound healing assay was investigated in the MDA-MB-231 cell line at 20 μM. The results showed that compounds (+)-1, (+)-4, and (–)-4 exhibit a migration inhibitory effect compared to the DMSO group in the MDA-MB-231 cell line, of which (+)-4 showed stronger inhibitory effect (Figures 7B, D). Furthermore, the effect of compound (+)-4 was found to be dose-dependent at concentrations of 10 μM, 20 μM, and 30 μM (Figures 7C,E).
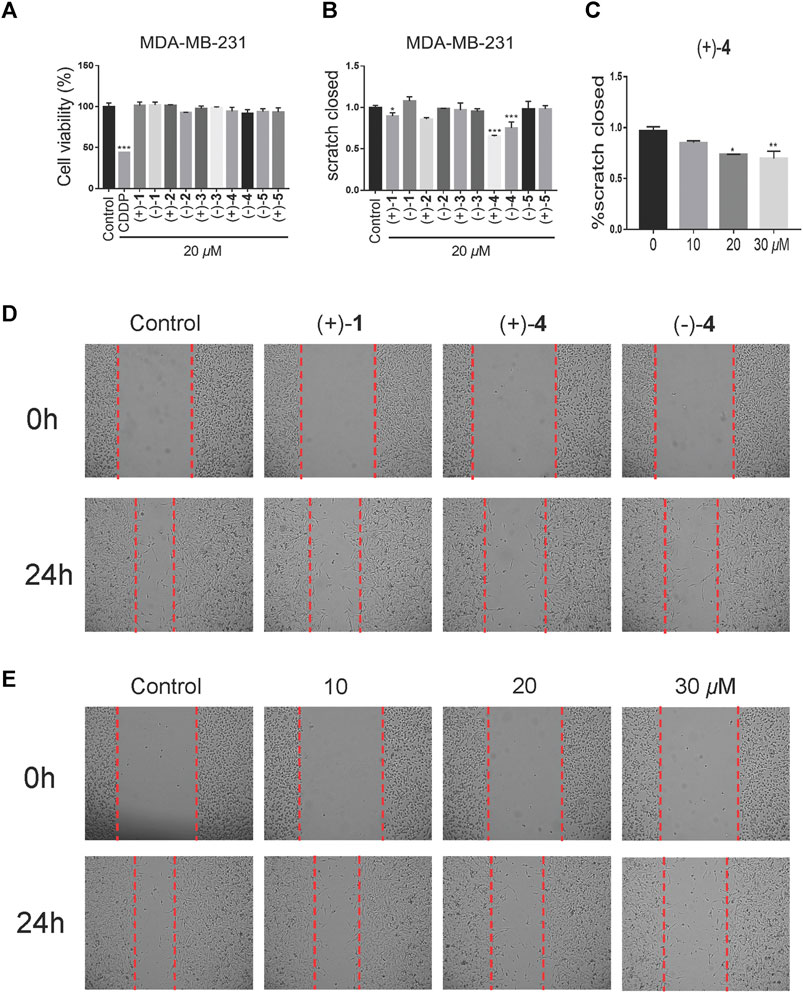
FIGURE 7. Effects of compounds on cell migration at low-toxicity doses. (A) Cell viability of MDA-MB-231 was treated with the vehicle or 20 μM compounds for 48 h (n = 3). (B) Wound healing assay of the MDA-MB-231 cell line, treated with the vehicle or 20 μM compounds for 24 h (n = 3). (C) The dose-dependent migratory inhibition effect of the compound (+)-4. (D) Representative pictures of compounds (+)-1 (+)-4 and (–)-4 at 20 μM for 24 h of MDA-MB-231. (E) Representative pictures of compound (+)-4 at different doses for 24 h in MDA-MB-231 cells. Data are of three replicates (n = 3) *p < 0.05, **p < 0.01, one-way ANOVA.
Experimental
General
Optical rotations of (+)-1, (–)-1, (+)-3, and (–)-3 were recorded on an Anton Paar MCP-100 digital polarimeter, and the optical rotations of (+)-2, (–)-2, (+)-4, (–)-4, (+)-5, and (–)-5 were tested on an Anton Paar MCP-500 digital polarimeter. UV and CD spectra were measured on a Chirascan instrument. NMR spectra were recorded on a Bruker 600 MHz or a Bruker 500 MHz spectrometer, with TMS as an internal standard. Positive HRESIMS data of 1 were collected by a Shimazu LC-20AD AB SCIEX triple TOF 6600+ MS spectrometer. The HRESIMS data of 2 and 4 were recorded on a Waters Xevo G2-XS Qtof MS spectrometer. An Agilent 6210 ESI/TOF MS spectrometer was used to collect HRESIMS data of 3 and 5. C-18 silica gel (40–60 μm; Daiso Co., Japan), MCI gel CHP 20 P (75–150 μm, Mitsubishi Chemical Industries, Tokyo, Japan), and Sephadex LH-20 (Amersham Pharmacia, Uppsala, Sweden) were used for column chromatography. Silica gel (Qingdao Marine Chemical Inc. Qingdao, People’s Republic of China) was used for preparative TLC. Semi-preparative HPLC was carried out on a Saipuruisi chromatograph with a YMC-Pack ODS-A column (250 mm × 10 mm, i.d. 5 μm), and preparative HPLC on a Chuangxin–Tongheng chromatograph equipped with a Thermo Hypersil GOLD-C18 column (250 mm × 21.2 mm, i.d. 5 μm). Chiral HPLC analysis of 1 and 4 were carried out on an Agilent 1260 chromatograph with a Cellulose-3 column (LC, 250 mm × 4.6 mm, i.d. 5 μm), chiral HPLC analysis of 2 and 5 were carried out on an Agilent 1260 chromatograph with an i-Amylose-1 column (LC, 250 mm × 10 mm, i.d. 5 μm), and chiral HPLC analysis of 3 was carried out on a Saipuruisi chromatograph with a Daicel Chiralpak column (IC, 250 mm × 4.6 mm,i.d. 5 µm).
Fungal Material
The fruiting bodies of G. lucidum were collected from Dayao County, Yunnan Province, People’s Republic of China, in April 2018. The authentication of this material was finished by Prof. Zhu-Liang Yang at the Kunming Institute of Botany, Chinese Academy of Sciences, Kunming, People’s Republic of China, and the voucher specimen (CHYX-0615) of it is deposited at the Institute for Inheritance-Based Innovation of Chinese Medicine, School of Pharmaceutical Sciences, Shenzhen University Health Science Center, People’s Republic of China.
Extraction and Isolation
The powdered fruiting bodies of G. lucidum (30.0 kg) were extracted with 95% EtOH under percolation (240 L) at room temperature, and a crude extract (2.1 kg) was provided and then was suspended in H2O and partitioned with EtOAc three times to obtain an EtOAc part (1.1 kg). This extract was separated by an MCI gel CHP 20P column (MeOH/H2O, 40–100%) to afford thirteen fractions (Fr.1–Fr.13).
Fr.10 (30.3 g) was gel-filtrated over Sephadex LH-20 (MeOH) and further subjected to a silica gel column with increasing acetyl acetate in petroleum ether (15:1–1:1) to afford three portions (Fr.10.1−Fr.10.3). The second portion (2.1 g) was cut into five parts (Fr.10.2.1−Fr.10.2.5) using preparative HPLC (MeOH/H2O containing 0.05% TFA in water, 55–100%). Fr.10.2.1 (197.0 mg) was separated by preparative thin-layer chromatography (PTLC) (CH2Cl2/acetone = 10:1) to obtain Fr.10.2.1.1−Fr.10.2.1.4. Among them, Fr.10.2.1.3 (13.0 mg) was purified by semi-preparative HPLC (MeOH/H2O containing 0.05% TFA in water, 58%, flow rate: 3 mL/min) to afford compound 3 (3.0 mg, tR = 36.9 min).
Fr.11 (123.0 g) was subjected to a silica gel column with increasing acetone in petroleum ether (10:1–5:1) to give four parts (Fr.11.1−Fr.11.4). Among them, the first part (2.6 g) was purified by Sephadex LH-20 (MeOH) to obtain a fraction (129.0 mg), which was subjected to semi-preparative HPLC (MeOH/H2O, 65%, 100%, flow rate: 3 mL/min) to give two fractions (Fr.11.1.1 and Fr.11.1.2). Fr.11.1.2 (70.0 mg) was then fractionated by semi-preparative HPLC (aqueous AcCN, 75%, flow rate: 3 mL/min) to produce compound 4 (0.5 mg, tR = 19.4 min). Fr.11.2 (3.4 g) was subjected to Sephadex LH-20 (MeOH) to acquire three parts (Fr.11.2.1−Fr.11.2.3). Then, Fr.11.2.3 (147.0 mg) was segregated by semi-preparative HPLC (MeOH/H2O, 73%, flow rate: 3 mL/min) to afford compound 1 (0.9 mg, tR = 13.6 min). Fr.11.4 (99.0 g) was gel-filtrated over Sephadex LH-20 (MeOH) to afford Fr.11.4.1−Fr.11.4.3. Then, the last part (1.7 g) was cut into five parts (Fr.11.4.3.1−Fr.11.4.3.5) by vacuum liquid chromatography (VLC) by increasing acetone in petroleum ether (15:1–5:1). Fr.11.4.3.5 (1.3 g) was subjected to PTLC (petroleum ether/acetone = 3:2) to obtain three parts (Fr.11.4.3.5.1−Fr.11.4.3.5.3), of which, Fr.11.4.3.5.1 (307.0 mg) (Rf = 0.8) was subjected to preparative HPLC (MeOH/H2O, 66–95%) to obtain six parts (Fr.11.4.3.5.1.1−Fr.11.4.3.5.1.6). Fr.11.4.3.5.1.1 (16.0 mg) was purified by semi-preparative HPLC (MeOH/H2O, 73%, flow rate: 3 mL/min) to afford compound 2 (2.8 mg, tR = 40.7 min). Fr.11.4.3.5.3 (374.0 mg) (Rf = 0.2) was cut by semi-preparative HPLC (aqueous AcCN with 0.05% TFA in water, 62%, flow rate: 3 mL/min) to afford eleven parts (Fr.11.4.3.5.3.1−Fr.11.4.3.5.3.11). Among them, Fr.11.4.3.5.3.1 (23.0 mg) was purified by semi-preparative HPLC (MeOH/H2O containing 0.05% TFA in water, 74%, flow rate: 3 mL/min) to produce compound 5 (1.3 mg, tR = 25.8 min).
In addition, racemic 1, 4, 5 and an aliquot of 2, 3 were further purified by chiral HPLC to provide their enantiomers (flow rate: 1 mL/min): (+)-1 (0.4 mg, tR = 29.0 min) and (–)-1 (0.5 mg, tR = 31.9 min) (n-hexane/ethanol, 93:7); (+)-2 (0.5 mg, tR = 14.5 min) and (–)-2 (0.5 mg, tR = 15.4 min) (n-hexane/ethanol containing 0.05% TFA, 95:5); (+)-3 (0.9 mg, tR = 11.5 min) and (–)-3 (0.6 mg, tR = 14.0 min) (n-hexane/ethanol, 90:10); (+)-4 (0.2 mg, tR = 17.1 min) and (–)-4 (0.2 mg, tR = 21.3 min) (n-hexane/ethanol containing 0.05% TFA, 95:5); (–)-5 (0.6 mg, tR = 25.4 min) and (+)-5 (0.7 mg, tR = 33.0 min) (n-hexane/ethanol containing 0.05% TFA, 92:8). Due to the less quantity of compounds 4 and 5, these two compounds were enriched from fungal material of Ganoderma cochlear (CHYX-0589 for G. cochlear, and the detail isolation was not described here).
Compound Characterization Data
(±)-Dayaolingzhiol I (1), yellowish gum; UV (MeOH) λmax (log ε) 366 (3.53), 258 (3.82), 229 (4.11) nm; {[α]
(±)-Dayaolingzhiol J (2), yellowish gum; UV (MeOH) λmax (log ε) 370 (2.66), 297 (3.02), 260 (3.47) nm; {[α]
(±)-Dayaolingzhiol K (3), yellowish gum; UV (MeOH) λmax (log ε) 299 (3.24) nm; {[α]
(±)-Dayaolingzhiol L (4), yellowish gum; UV (MeOH) λmax (log ε) 366 (3.08), 257 (3.35), 226 (3.69) nm; {[α]
(±)-Dayaolingzhiol M (5), Yellow Gum; UV (MeOH) λMax (log ε) 367 (2.89), 258 (3.18), 228 (3.48) nm; {[α]
Insulin Resistance Assay
Cell Culture
C2C12 cells, a mouse skeletal muscle myoblast line (Procell Life Science and Technology Co., Wuhan, China), were cultured by using the same method as previously described (Qin et al., 2021). When the cells differentiated into elongated, multinucleated myotubes, they were exposed to 100 nM insulin for an additional 24 h to mimic insulin resistance.
Cell Viability Assay
C2C12 (5× 105 cells/mL) were seeded into 96-well plates with complete DMEM. After being cultured overnight, C2C12 cells were treated with five pairs of meroterpenoids or DMSO for 24 h. Then Cell Count Kit-8 (CCK-8, Beyotime, Shanghai, China) was added into each well for 1 h at 37 °C. The absorbance of each well was recorded at 450 nm using a microplate reader (BioTek, United States).
Glucose Uptake Assay
C2C12 cells were incubated in DMEM (low glucose) for 6 h and placed in high-glucose DMEM before adding compounds, and 30 min after compound treatment, insulin (100 nmol/L) was added and cultured for 4 h. Glucose content of the culture supernatant was measured using the Glucose Colorimetric/Fluorometric Assay Kit (BioVison, K606-100).
Western Blot
After different compound treatment for 24 h in the IR model, total protein was extracted from the cell lines using radioimmunoprecipitation assay (RIPA) buffer (Beyotime, China) containing protease cocktail (Roche, Germany) and quantified the protein samples using the BCA assay (Thermo Scientific, United States). Equal amounts of protein extracts were separated by 8% SDS-PAGE and transferred to PVDF membranes. The membranes were blocked with 5% BSA, then with the indicated antibodies overnight at 4 °C, and followed the incubation with horseradish peroxidase (HRP)–conjugated secondary antibody at room temperature. The bands were visualized and measured using the ECL kit (Pierce, United States). The densitometry analysis of the immunoblot results was performed using ImageJ software (NIH, United States).
Statistical Analysis
All experimental data in this study were performed in triplicate. The results were represented as mean ± SD. Statistical analyses were performed using Graphpad Prism 6 (GraphPad Software, San Diego, CA, United States) with one-way ANOVA. Differences were considered significant when *p ≤ 0.05, **p ≤ 0.01, ***p ≤ 0.001, and ****p ≤ 0.0001.
Wound Healing Assay in MDA-MB-231 Cells
The biological evaluation for wound healing in MDA-MB-231 cells was conducted as the previously reported protocols (Cai et al., 2021).
Conclusion
To conclude, triterpenoids and polysaccharides as chemical compositions of Ganoderma mushrooms have been traditional gnosia for decades. Our present study resulted in the isolation of five pairs of meroterpenoidal enantiomers, representing new chemical composition of G. lucidum and aiding an in-depth insight into Ganoderma metabolites. The activation of (+)-5 on Akt, AMPK, and glucose uptake in C2C12 cells indicates its role in diabetes prevention. In addition, the inhibitory effects of compounds (+)-1, (+)-4, and (–)-4 on cell mobility in MDA-MB-231 cells indicate their role in TNBC. These results shed light on the traditional uses of G. lucidum, implying G. lucidum–derived compounds’ potential in insulin-resistant diseases and cancer, and might inspire a consideration of Ganoderma meroterpenoids as dietary supplements.
Data Availability Statement
The original contributions presented in the study are included in the article/Supplementary Material; further inquiries can be directed to the corresponding author.
Author Contributions
JZ conducted chemical experiments and drafted the manuscript. DW and DC conducted biological experiments. QL revised the manuscript. YC designed the work and revised the manuscript. All authors have approved the final version of the manuscript.
Funding
We are indebted to financial supports from the NSFC-Joint Foundation of Yunnan Province (U1702287), the National Natural Science Foundation of China (82030115), Shenzhen Fundamental Research Program (JCYJ20200109113803838), the National Science Fund for Distinguished Young Scholars (81525026), Guangdong Key Laboratory for Functional Substances in Medicinal Edible Resources and Healthcare Products (2021B1212040015), the National Natural Science Foundation of China (82104036), and the National Natural Science Foundation of China (81903506).
Conflict of Interest
The authors declare that the research was conducted in the absence of any commercial or financial relationships that could be construed as a potential conflict of interest.
Publisher’s Note
All claims expressed in this article are solely those of the authors and do not necessarily represent those of their affiliated organizations, or those of the publisher, the editors, and the reviewers. Any product that may be evaluated in this article, or claim that may be made by its manufacturer, is not guaranteed or endorsed by the publisher.
Supplementary Material
The Supplementary Material for this article can be found online at: https://www.frontiersin.org/articles/10.3389/fchem.2021.772740/full#supplementary-material
References
Adams, M., Christen, M., Plitzko, I., Zimmermann, S., Brun, R., Kaiser, M., et al. (2010). Antiplasmodial lanostanes from the Ganoderma lucidum mushroom. J. Nat. Prod. 73, 897–900. doi:10.1021/np100031c
Allahbadia, G., and Merchant, R. (2008). Polycystic ovary syndrome in the Indian subcontinent. Semin. Reprod. Med. 26, 022–034. doi:10.1055/s-2007-992921
Balasubramanyam, A. (2006). Health outcomes beyond glucose control. Am. J. Manag. Care 12, S382–S391.
Cai, D., Zhang, J. J., Wu, Z. H., Qin, F. Y., Yan, Y. M., Zhang, M., et al. (2021). Lucidumones B-H, racemic meroterpenoids that inhibit tumor cell migration from Ganoderma lucidum. Bioorg. Chem. 110, 104774. doi:10.1016/j.bioorg.2021.104774
Cao, W. W., Luo, Q., Cheng, Y. X., and Wang, S. M. (2016). Meroterpenoid enantiomers from Ganoderma sinensis. Fitoterapia 110, 110–115. doi:10.1016/j.fitote.2016.03.003
Chen, H., Yang, J., Yang, Y., Zhang, J., Xu, Y., and Lu, X. (2021). The natural products and extracts: anti‐triple‐negative breast cancer in vitro. Chem. Biodiver. 18, 2001047. doi:10.1002/cbdv.202001047
Chowdhury, P., Ghosh, U., Samanta, K., Jaggi, M., Chauhan, S. C., and Yallapu, M. M. (2021). Bioactive nanotherapeutic trends to combat triple negative breast cancer. Bioactive Mater. 6, 3269–3287. doi:10.1016/j.bioactmat.2021.02.037
Cortés-Rojo, C., Vargas-Vargas, M. A., Olmos-Orizaba, B. E., Rodríguez-Orozco, A. R., and Calderón-Cortés, E. (2020). Interplay between NADH oxidation by complex I, glutathione redox state and sirtuin-3, and its role in the development of insulin resistance. Biochim. Biophys. Acta (Bba) - Mol. Basis Dis. 1866, 165801. doi:10.1016/j.bbadis.2020.165801
Dai, W. F., Zhu, Y. X., Qin, F. Y., Chang, J. L., Zeng, Y., Wang, J. G., et al. (2020). Skeletal meroterpenoids from Ganoderma petchii mushrooms that potentially stimulate umbilical cord mesenchymal stem cells. Bioorg. Chem. 97, 103675. doi:10.1016/j.bioorg.2020.103675
García-Carretero, R., Holgado-Cuadrado, R., and Barquero-Pérez, Ó. (2021). Assessment of classification models and relevant features on nonalcoholic steatohepatitis using random forest. Entropy 23, 763. doi:10.3390/e23060763
Guo, S. (2014). Insulin signaling, resistance, and metabolic syndrome: insights from mouse models into disease mechanisms. J. Endocrinol. 220, T1–T23. doi:10.1530/joe-13-0327
Jiang, M., Wu, Z., Liu, L., and Chen, S. (2021). The chemistry and biology of fungal meroterpenoids (2009-2019). Org. Biomol. Chem. 19, 1644–1704. doi:10.1039/d0ob02162h
Krobthong, S., and Yingchutrakul, Y. (2020). Identification and enhancement of antioxidant P1-peptide isolated from Ganoderma lucidum hydrolysate. Food Biotechnol. 34, 338–351. doi:10.1080/08905436.2020.1844228
Leskosek-Cukalovic, I., Despotovic, S., Lakic, N., Niksic, M., Nedovic, V., and Tesevic, V. (2010). Ganoderma lucidum - medical mushroom as a raw material for beer with enhanced functional properties. Food Res. Int. 43, 2262–2269. doi:10.1016/j.foodres.2010.07.014
Loibl, S., Poortmans, P., Morrow, M., Denkert, C., and Curigliano, G. (2021). Breast cancer. The Lancet 397, 1750–1769. doi:10.1016/s0140-6736(20)32381-3
Lu, J., Zhao, H., Chen, J., Fan, W., Dong, J., Kong, W., et al. (2007). Evolution of phenolic compounds and antioxidant activity during malting. J. Agric. Food Chem. 55, 10994–11001. doi:10.1021/jf0722710
Luo, Q., Yang, X. H., Yang, Z. L., Tu, Z. C., and Cheng, Y. X. (2016). Miscellaneous meroterpenoids from Ganoderma applanatum. Tetrahedron 72, 4564–4574. doi:10.1016/j.tet.2016.06.019
McCarthy, M. (2014). Nearly one in 10 US residents has diabetes, CDC reports. BMJ 348, g3962. doi:10.1136/bmj.g3962
Niedermeyer, T. H. J., Jira, T., Lalk, M., and Lindequist, U. (2013). Isolation of farnesylhydroquinones from the basidiomycete Ganoderma pfeifferi. Nat. Prod. Bioprospect. 3, 137–140. doi:10.1007/s13659-013-0036-5
Niu, X. M., Li, S. H., Sun, H. D., and Che, C. T. (2006). Prenylated phenolics from Ganoderma fornicatum. J. Nat. Prod. 69, 1364–1365. doi:10.1021/np060218k
Qin, F. Y., Zhang, H. X., Di, Q. Q., Wang, Y., Yan, Y. M., Chen, W. L., et al. (2020). Ganoderma cochlear metabolites as probes to identify a COX-2 active site and as in vitro and in vivo anti-inflammatory agents. Org. Lett. 22, 2574–2578. doi:10.1021/acs.orglett.0c00452
Qin, F. Y., Zhang, J. J., Wang, D. W., Xu, T., Cai, D., and Cheng, Y. X. (2021). Direct determination of E and Z configurations for double bond in bioactive meroterpenoids from Ganoderma mushrooms by diagnostic 1H NMR chemical shifts and structure revisions of previous analogues. J. Funct. Foods 87, 104758. doi:10.1016/j.jff.2021.104758
Rajesh, Y., and Sarkar, D. (2021). Association of adipose tissue and adipokines with development of obesity-induced liver cancer. Ijms 22, 2163. doi:10.3390/ijms22042163
Sharma, N. K., Chuang Key, C. C., Civelek, M., Wabitsch, M., Comeau, M. E., Langefeld, C. D., et al. (2019). Genetic regulation of Enoyl-CoA hydratase domain-containing 3 in adipose tissue determines insulin sensitivity in African Americans and Europeans. Diabetes 68, 1508–1522. doi:10.2337/db18-1229
Tan, J. W., Israf, D. A., and Tham, C. L. (2018). Major bioactive compounds in essential oils extracted from the rhizomes of Zingiber zerumbet (L) Smith: a mini-review on the anti-allergic and immunomodulatory properties. Front. Pharmacol. 9, 652. doi:10.3389/fphar.2018.00652
Veljović, S. P., Belović, M. M., Tešević, V. V., Nikšić, M. P., Vukosavljević, P. V., Nikićević, N. J., et al. (2019). Volatile composition, colour, and sensory quality of spirit-based beverages enriched with medicinal fungus Ganoderma lucidum and herbal extract. Food Technol. Biotechnol. 57, 408–417. doi:10.17113/ftb.57.03.19.6106
Wang, K., Bao, L., Ma, K., Zhang, J., Chen, B., Han, J., et al. (2017). A novel class of α-glucosidase and HMG-CoA reductase inhibitors from Ganoderma leucocontextum and the anti-diabetic properties of ganomycin I in KK-Ay mice. Eur. J. Med. Chem. 127, 1035–1046. doi:10.1016/j.ejmech.2016.11.015
Wang, K., Bao, L., Zhou, N., Zhang, J., Liao, M., Zheng, Z., et al. (2018). Structural modification of natural product ganomycin I leading to discovery of α α-glucosidase and HMG-CoA reductase dual inhibitor improving obesity and metabolic dysfunction in vivo. J. Med. Chem. 61, 3609–3625. doi:10.1021/acs.jmedchem.8b00107
Willard, D. L., Stevenson, M., and Steenkamp, D. (2016). Type B insulin resistance syndrome. Curr. Opin. Endocrinol. Diabetes Obes. 23, 318–323. doi:10.1097/MED.0000000000000263
Yan, Y. M., Zhang, H. X., Liu, H., Wang, Y., Wu, J. B., Li, Y. P., et al. (2019). (+/−)-Lucidumone, a COX-2 inhibitory caged fungal meroterpenoid from Ganoderma lucidum. Org. Lett. 21, 8523–8527. doi:10.1021/acs.orglett.9b02840
Keywords: Ganoderma lucidum, meroterpenoids, Akt phosphorylation, AMPK phosphorylation, glucose uptake, cell migration
Citation: Zhang J-J, Wang D-W, Cai D, Lu Q and Cheng Y-X (2021) Meroterpenoids From Ganoderma lucidum Mushrooms and Their Biological Roles in Insulin Resistance and Triple-Negative Breast Cancer. Front. Chem. 9:772740. doi: 10.3389/fchem.2021.772740
Received: 08 September 2021; Accepted: 28 September 2021;
Published: 03 November 2021.
Edited by:
Guigen Li, Texas Tech University, United StatesReviewed by:
Liang Zhang, Jiangnan University, ChinaGeorge A O'Doherty, Northeastern University, United States
Copyright © 2021 Zhang, Wang, Cai, Lu and Cheng. This is an open-access article distributed under the terms of the Creative Commons Attribution License (CC BY). The use, distribution or reproduction in other forums is permitted, provided the original author(s) and the copyright owner(s) are credited and that the original publication in this journal is cited, in accordance with accepted academic practice. No use, distribution or reproduction is permitted which does not comply with these terms.
*Correspondence: Yong-Xian Cheng, eXhjaGVuZ0BzenUuZWR1LmNu