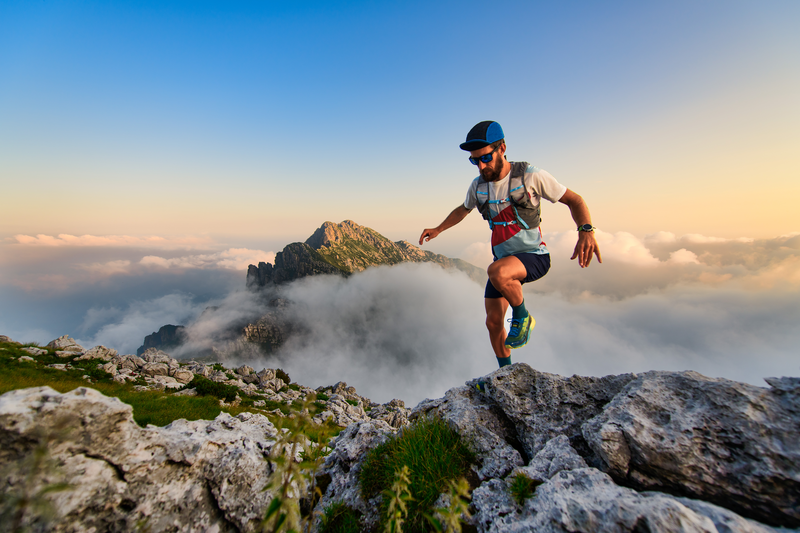
94% of researchers rate our articles as excellent or good
Learn more about the work of our research integrity team to safeguard the quality of each article we publish.
Find out more
ORIGINAL RESEARCH article
Front. Chem. , 19 November 2021
Sec. Nanoscience
Volume 9 - 2021 | https://doi.org/10.3389/fchem.2021.769648
This article is part of the Research Topic Bio-Inspired Nanomaterials in Surface Engineering and Bioapplications View all 12 articles
Escherichia coli (E. coli) O157:H7 can cause many food safety incidents, which seriously affect human health and economic development. Therefore, the sensitive, accurate, and rapid determination of E. coli O157:H7 is of great significance for preventing the outbreak and spread of foodborne diseases. In this study, a carbon dots-Fe3O4 nanomaterial (CDs-Fe3O4)-based sensitive electrochemical biosensor for E. coli O157:H7 detection was developed. The CDs have good electrical conductivity, and the surface of carbon dots contains abundant carboxyl groups, which can be used to immobilize probe DNA. Meanwhile, the CDs can be used as a reducing agent to prepare CDs-Fe3O4 nanomaterial. The Fe3O4 nanomaterial can improve the performance of the electrochemical biosensor; it also can realize the recovery of CDs-Fe3O4 due to its magnetism. As expected, the electrochemical biosensor has excellent specificity of E. coli O157:H7 among other bacteria. The electrochemical biosensor also exhibited good performance for detecting E. coli O157:H7 with the detection range of 10–108 CFU/ml, and the detection limit of this electrochemical biosensor was 6.88 CFU/ml (3S/N). Furthermore, this electrochemical biosensor was successfully used for monitoring E. coli O157:H7 in milk and water samples, indicating that this electrochemical biosensor has good application prospect. More importantly, this research can provide a new idea for the detection of other bacteria and viruses.
In recent years, food poisoning caused by foodborne pathogens has frequently occurred worldwide, which is the most prominent public health problem in the world (Hou et al., 2020; Duan et al., 2021). As one of the most hazardous foodborne pathogens, Escherichia coli (E. coli) O157:H7 can cause some diseases such as hemorrhagic colitis and hemolytic uremic syndrome. What is more, low-dose E. coli O157:H7 can make humans sick, and in severe cases, it can even cause kidney failure and death. Currently, more than two million acute foodborne diseases worldwide are attributed to E. coli O157:H7 each year (Li et al., 2015a; Zhou et al., 2020). Therefore, it is very important to monitor the E. coli O157:H7 with high sensitivity. At present, some bioanalytical methods have been applied for E. coli O157:H7 detection, including enzyme-linked immunosorbent assay (ELISA) (Guo et al., 2016; Zhao et al., 2020), lateral flow immunochromatographic detection technology (Xie et al., 2014; Ye et al., 2020), polymerase chain reaction (PCR) (Dong et al., 2015), and so on. These alternative assays are the most rapidly used tests because of their good specificity and stability. Nevertheless, they are still faced with some limitations in detecting foodborne pathogens, including low sensitivity, complicated pretreatment, and high environmental impact. Therefore, there is an urgent need to establish a sensitive, simple, and highly specific method for detecting E. coli O157:H7. At present, many different electrochemical sensors have been used in food safety due to their some advantages, such as high sensitivity, economy, and accuracy (Li et al., 2015b; Li et al., 2016; Liu Y. et al., 2020; Dong et al., 2020). Therefore, electrochemical biosensors for the detection of E. coli O157:H7 have received extensive attention.
As is known, due to the excellent chemical, physical and biological properties of nanomaterials, they have been widely used in biomedical (Liu H. et al., 2020; Kuang et al., 2020; Thakur et al., 2020; Wu et al., 2020; Yi et al., 2021), analytical science (Chen et al., 2018; Broza et al., 2019; Jia et al., 2020; Lee et al., 2020; Mao et al., 2020; He S. et al., 2021; Mao et al., 2021), energy catalytic (Zhuang et al., 2019a; Zhuang et al., 2019b; Enesca, 2020; Zhuang et al., 2021), and other fields. Till now, some nanomaterials have been successfully used to construct electrochemical sensors for E. coli O157:H7 detection, such as graphene/AuNPs (You et al., 2020), reduced graphene oxide/polyaniline/Au@Pt/neutral red (rGO/PANI/Au@Pt/Nr) (Mo et al., 2019), rGO/Au@Pt (Zhu et al., 2018), rGO-poly (vinyl alcohol)/gold nanoparticles nanocomposite (AuNPs/rGO-PVA) (Qaanei et al., 2021), and so on. Although the abovementioned materials have made certain contributions to electrochemical sensors for detecting E. coli O157:H7, the abovementioned materials still have disadvantages, including complicated preparation, insufficient economy, and environmental protection, or low sensitivity of sensors. Therefore, it is important to prepare an easy-to-obtain, economical, and environmentally friendly functional material for the construction of electrochemical sensors for detecting E. coli O157:H7. As a member of “zero-dimensional” carbon nanomaterials, carbon dots (CDs) have some advantages of good biocompatibility, simple synthesis, and excellent conductivity (Zhang et al., 2019; Huang et al., 2020; Zhang and Du, 2020; He H. et al., 2021; Lin et al., 2021; Xu and Liu, 2021), and they have been successfully used to construct new electrochemical sensors, such as Pd-Au@CDs nanocomposite that we prepared for the preparation of the novel electrochemical DNA biosensor (Huang et al., 2017). Liu et al. designed an electrochemical sensor for measuring catechol based on F, N-doped CDs (Liu et al., 2019). Giang et al. constructed a wireless electrochemical biosensor based on CDs-hyaluronic acid/TiO2/Cu2+ for cancer cell detection (Giang et al., 2021). Sri et al. developed a CD/polymethyl methacrylate-based electrochemical biosensor for monitoring TNF-α targeting (Sri et al., 2021).
In this study, a CDs-Fe3O4 nanomaterial-based electrochemical biosensor is constructed for detecting E. coli O157:H7, which is highly sensitive and reliable (Figure 1). The CDs have good electrical conductivity and large specific surface areas to improve the sensitivity of the sensor. The CDs also can be used as the reducing agent to synthesize the CDs-Fe3O4 nanomaterial. The Fe3O4 can improve the catalytic performance of the electrochemical biosensor, and it also can realize the recovery of CDs-Fe3O4 due to its magnetism. This electrochemical biosensor can achieve a good linear and detection limit for monitoring E. coli O157:H7. In addition, the biosensor has been also successfully applied to measure the E. coli O157:H7 in milk, indicating that the electrochemical biosensor has a broad application prospect. What is more, this study can provide a new idea for the preparation of electrochemical biosensors for other bacteria and virus detection, such as Staphylococcus aureus (S. aureus), Listeria, SARS-CoV-2, Hepatitis B virus, etc.
FIGURE 1. A sensitive electrochemical DNA biosensor based on CDs/Fe3O4 nanocomposite is constructed for E. coli O157:H7 detection.
The preparation of CDs-Fe3O4 nanocomposite was based on the method of instrument preparation of our group (Kuang et al., 2020): First, 3 g of citric acid and 1 g of glucose were added in 5 ml H2O and reacted under microwave with a radiation power of 900 W for 6 min to synthesize CDs. Second, the above solution was cooled and added 30 ml H2O, centrifuged them at 12,000 rpm for 15 min and took the supernatant, then dialyzed the supernatant for 24 h and then freeze dried. Third, the abovementioned CD solid was dissolved in 25 ml of the aqueous solution, then 1 g of sodium acetate and 1 g of ferric chloride was added into 15 ml of CD solution, the mixed solution was sonicated for 8 h, then placed in an autoclave, and reacted at 210°C for 24 h; after cooling, they were centrifuged at 12,000 rpm for 10 min to acquire CDs-Fe3O4 nanomaterial.
First, 5.0 μl of CDs-Fe3O4 nanomaterial was slowly dropped on the glassy surface of the carbon electrode (GCE) to prepare a CDs-Fe3O4/GCE working electrode. Second, the CDs-Fe3O4/GCE electrode was immersed in 1 ml of 0.1 mM PBS, 8.00 mM EDC, and 8.00 mM NHS mixed solution for 3 h to activate the electrode, then it was rinsed with TE buffer solution. Third, the above electrode was immersed in 1 ml of 0.1 μM probe DNA to react for 24 h, and it was rinsed with TE buffer solution to prepare the DNA/CDs-Fe3O4/GCE probe electrode. Finally, the prepared electrode was stored at 4°C.
The prepared DNA/CDs-Fe3O4/GCE was immersed into different concentrations (C) of E. coli O157:H7 solutions and reacted for 40 min at 42°C in a water bath. After the reaction, TE buffer solution was used to wash off non-specifically adsorbed E. coli on the surface to obtain the E. coli O157:H7-DNA/CDs-Fe3O4/GCE. Cyclic voltammetry (CV) and differential pulse voltammetry (DPV) have characterized the layer-by-layer assembly of each modified electrode: the [Fe (CN)6]3-/4- has been applied as the electrolyte. For the test parameters of CV, the potential scanning range was −0.2–0.8 V, and the scan rate(5) was 0.1 V/s. DPV was used to record the electrochemical signal changes before and after the identification of the electrode and different substances, and the potential interval was −0.2–0.8 V, the amplitude was 0.05 V, the pulse width was 0.05 s.
Transmission electron microscopy (TEM) has characterized the morphologies of CDs and CDs-Fe3O4 nanomaterial. As shown in Figure 2A, the particle size distribution of the prepared CDs is relatively uniform, mainly at 3–4 nm. Figure 2B shows the high-resolution TEM (HRTEM) image of CDs. It shows that CDs have a good crystal lattice, and the lattice spacing is 0.210 nm, which is a typical (002) carbon crystal plane. As shown in Figure 2C, the particle size of the CDs-Fe3O4 nanomaterial was in the range of 15–40 nm. The HRTEM characterization of CDs-Fe3O4 (Figure 2D) shows that the nanomaterial has two lattice spacings, 0.345 and 0.210 nm, respectively. The corresponding crystal planes are (220) of the Fe3O4 and (002) of the carbon. The above morphology characterization can indicate that CDs-Fe3O4 nanomaterial has been successfully prepared.
It is well known that [Fe(CN)6]3−/4− is often used as a supporting electrolyte for electrochemical biosensors (Fan et al., 2018; Li et al., 2021; Nano et al., 2021). The working electrodes were electrochemically characterized by CVs in 1.0 mM [Fe(CN)6]3−/4− and 0.1 M KCl solution (Figure 3A). On the surface of GCE, the redox peak current (I) has good reversibility, and the oxidation peak potential (Ipa) and reduction peak potential (Ipc) are −6.616 and 5.958 A, respectively. When the CDs are modified on the surface of GCE, the Ipa and Ipc are promoted to −12.29 and 12.06 A, respectively, indicating that the prepared CDs have good conductivity. When the electrode is CDs-Fe3O4/GCE, the Ipa and Ipc are further enhanced, indicating that the introduction of Fe3O4 can improve the conductivity of the electrode. However, when the probe DNA is self-assembled on the surface of CDs-Fe3O4/GCE through carboxyammonia coupling reaction, the I decreases; the main reason is that the DNA molecules will hinder the diffusion of [Fe(CN)6]3−/4− on the surface of CDs-Fe3O4/GCE. At the same time, the results indicate that the probe DNA was successfully modified on CDs-Fe3O4/GCE’s surface.
The effect of different v on the electrochemical response of DNA/CDs-Fe3O4/GCE in [Fe(CN)6]3−/4− has also been studied. Figure 4A shows that in the process of increasing v, the I also keeps increasing. As shown in Figure 4B, the Ipa and Ipc show an excellent linear relationship with ν1/2, and the linear regression equations are Ipa = 3.598 + 80.265 v1/2 and Ipa = −2.551–85.187 v1/2, respectively. The results show that the electrochemical behavior of [Fe(CN)6]3−/4− on the surface of DNA/CDs-Fe3O4/GCE is mainly affected by the diffusion-controlled process (Alam and Deen, 2020).
FIGURE 4. (A) CVs of DNA/CDs-Fe3O4/GCE in1.0 mM [Fe(CN)6]3-/4- and 0.1 M KCl solution with different v (a–m: 0.01, 0.025, 0.05, 0.075, 0.1, 0.125, 0.15, 0.175, 0.20, 0.225, 0.25, 0,275, 0.3 Vs−1). (B) The relationship of redox peak current (I) with v1/2.
The interaction time between the probe electrode and the target substance is one of the key factors affecting the performance of the biosensors. Therefore, the interaction time of E. coli O157:H7 with DNA/CDs-Fe3O4/GCE was studied. When the C of E. coli O157:H7 was 10 CFU/Ml, the optimum hybridization time was determined to be 40 min (Supplementary Figure S1). However, when the C of E. coli O157:H7 was 108 CFU/Ml, the optimum hybridization time was determined to be 35 min (Supplementary Figure S2). Therefore, in this electrochemical biosensor, the best reaction time between E. coli O157:H7 and DNA/CDs-Fe3O4/GCE is 40 min.
The specificity of the electrochemical biosensor is a necessary factor to ensure the accuracy of the biosensor. Therefore, the interferences of different bacteria with 105 CFU/ml on the DNA/CDs-Fe3O4/GCE through DPV have been investigated, such as S. aureus, Salmonella, Staphylococcus lactis (S. lactis), and Listeria. Figure 5A shows that DNA/CDs-Fe3O4/GCE has almost no effect on other bacteria. However, the DNA/CDs-Fe3O4/GCE has a very strong response to E. coli O157:H7, and the Ipa drops by 53.42%. As shown in Figure 5B, other interfering substances have almost no effect on the determination of E. coli O157:H7. The above results show that the electrochemical biosensor based on DNA/CDs-Fe3O4/GCE for detecting E. coli O157:H7 has very excellent specificity and is expected to realize the determination of E. coli O157:H7 in real samples.
FIGURE 5. (A) Ipa of 105 CFU/ml different bacteria on the DNA/CDs-Fe3O4/GCE, (B) Ipa of 105 CFU/ml E. coli O157:H7 with different bacteria on the DNA/CDs-Fe3O4/GCE (a: none, b: E. coli O157:H7, c: S. aureus, d: Salmonella, e: S. lactis, and f: Listeria).
As is known, DPV has been extensively applied in the construction of electrochemical sensors because it has good detection sensitivity. This study explores the sensitivity and detection range of the electrochemical biosensors via DPV. Figure 6A shows that when E. coli O157:H7 interacts with DNA/CDs-Fe3O4/GCE, Ipa decreases as the C of E. coli O157:H7 increases. The main possible reason is E. coli O157:H7 has poor electrical conductivity, which affects the electrical conductivity of the working electrode’s interface, leading to a weakening of the electrochemical signal. When the C of E. coli O157:H7 is in the range of 10–107 CFU/ml, the lgC and Ipa show a good linear relationship (Figure 6B): Ipa = 1.5422 lgC—14.387. At the same time, the detection limit (LOD) can reach 6.88 CFU/ml. Compared with other electrochemical biosensors for monitoring E. coli O157:H7 (Table 1), this electrochemical biosensor based on DNA/CDs-Fe3O4/GCE has very good sensitivity.
FIGURE 6. (A) Differential pulse voltammetry (DPV) of E. coli O157:H7 with different concentrations (from a to i: 0, 10, 102, 103, 104, 105, 106, 107, 108 CFU/ml). (B) The relationship of Ipa with the lgC.
To investigate the reproducibility of this biosensor, six DNA/CDs-Fe3O4/GCE working electrodes were prepared, and they have been applied to detect 105 CFU/ml of E. coli O157:H7 respectively. Supplementary Figure S3 shows that the relative standard deviation (RSD) was 2.6%, which indicates the electrochemical biosensor based on DNA/CDs-Fe3O4/GCE for E. coli O157:H7 detection has good reproducibility. The stability of the electrochemical biosensor has been also studied; the DNA/CDs-Fe3O4/GCE was placed in a dryer at 4°C, and the above working electrode was applied to measure 105 CFU/ml of E. coli O157:H7 every 3 days. As shown in Supplementary Figure S4 after 30 days, the Ipa was only dropped by 2.7%, which shows that the electrochemical biosensor based on DNA/CDs- Fe3O4/GCE has good stability.
Since milk and water are often contaminated by E. coli O157:H7, it is very necessary to efficiently determine E. coli O157:H7 in milk and water. In this study, to verify the practical applicability of the biosensor, the electrochemical biosensor is used to determine the E. coli O157:H7 in milk and water. Table 2 shows that 103, 104, and 105 CFU/ml of E. coli O157:H7 were added to the samples, and the recovery rates are between 95.2% and 102.0%, At the same time, the results were consistent with ELISA results, indicating that the method is feasible for monitoring E. coli O157:H7 in milk and water samples.
In conclusion, a sensitive electrochemical DNA biosensor has been prepared for monitoring E. coli O157:H7 by CDs-Fe3O4 nanomaterial. Under the best conditions, when the C of E. coli O157:H7 is in the range of 10–108 CFU/ml, the lgC has a good linear relationship with the Ipa, and the LOD reaches 6.88 CFU/ml. At the same time, the electrochemical DNA biosensor has been successfully used to determine E. coli O157:H7 in milk and water samples. Therefore, this research provides an effective biosensor manufacturing strategy, which is expected to provide a reference for the preparation of electrochemical DNA biosensors for other bacteria or viruses detection.
The original contributions presented in the study are included in the article/Supplementary Material. Further inquiries can be directed to the corresponding authors.
XL and YM handled the data curation, and preparation and writing of the original draft. CH and XM were also involved in the writing of the original draft. YL, MY, and YK edited the manuscript. HZ participated in the reviewing of the draft. QH contributed to the reviewing, editing, and writing of the manuscript.
This work has been supported by the National Natural Science Foundation of China (No. 82060599), the Natural Science Foundation of Jiangxi (No. 20202BABL213018), the Science and Technology Project of the Education Department of Jiangxi Province (Nos. GJJ190795, GJJ190775 and GJJ190827), the Science and Technology Project of Jiangxi Health Committee (No. 202131033), the Research Fund of Gannan Medical University (Nos. ZD201901, YQ202003, YB201905, YB201931, YB201911), and the Science and Technology Plan Post-subsidy Project of Ganzhou (No. 2019-60-174).
The authors declare that the research was conducted in the absence of any commercial or financial relationships that could be construed as a potential conflict of interest.
All claims expressed in this article are solely those of the authors and do not necessarily represent those of their affiliated organizations, or those of the publisher, the editors, and the reviewers. Any product that may be evaluated in this article, or claim that may be made by its manufacturer, is not guaranteed or endorsed by the publisher.
The Supplementary Material for this article can be found online at: https://www.frontiersin.org/articles/10.3389/fchem.2021.769648/full#supplementary-material
Alam, A. U., and Deen, M. J. (2020). Bisphenol A Electrochemical Sensor Using Graphene Oxide and β-Cyclodextrin-Functionalized Multi-Walled Carbon Nanotubes. Anal. Chem. 92, 5532–5539. doi:10.1021/acs.analchem.0c00402
Bai, H., Bu, S., Liu, W., Wang, C., Li, Z., Hao, Z., et al. (2020). An Electrochemical Aptasensor Based on Cocoon-Like DNA Nanostructure Signal Amplification for the Detection of Escherichia C O157:H7. Analyst 145, 7340–7348. doi:10.1039/d0an01258k
Broza, Y. Y., Zhou, X., Yuan, M., Qu, D., Zheng, Y., Vishinkin, R., et al. (2019). Disease Detection with Molecular Biomarkers: From Chemistry of Body Fluids to Nature-Inspired Chemical Sensors. Chem. Rev. 119, 11761–11817. doi:10.1021/acs.chemrev.9b00437
Bu, S., Wang, K., Li, Z., Wang, C., Hao, Z., Liu, W., et al. (2020). An Electrochemical Biosensor Based on Methylene Blue-Loaded Nanocomposites as Signal-Amplifying Tags to Detect Pathogenic Bacteria. Analyst 145, 4328–4334. doi:10.1039/d0an00470g
Chen, B., Chen, D., Li, F., Lin, X., and Huang, Q. (2018). Graphitic Porous Carbon: Efficient Synthesis by a Combustion Method and Application as a Highly Selective Biosensor. J. Mater. Chem. B 6, 7684–7691. doi:10.1039/c8tb02139b
Dong, L. H., Zhang, L., Jing, J., Wang, J. N., Wang, J., and Chen, W. J. (2015). Development of Droplet Digital Polymerase Chain Reaction for Quantifying Escherichia Coli O157: H7. Chin. J. Anal. Chem. 43, 319–324. doi:10.11895/j.issn.0253-3820.140569
Dong, Y., Li, F., and Wang, Y. (2020). Low-Dimension Nanomaterial-Based Sensing Matrices for Antibiotics Detection: A Mini Review. Front. Chem. 8, 551. doi:10.3389/fchem.2020.00551
Duan, R., Fang, X., and Wang, D. (2021). A Methylene Blue Assisted Electrochemical Sensor for Determination of Drug Resistance of Escherichia C. Front. Chem. 9, 689735. doi:10.3389/fchem.2021.689735
Enesca, A. (2020). Enhancing the Photocatalytic Activity of SnO2-TiO2 and ZnO-TiO2 Tandem Structures toward Indoor Air Decontamination. Front. Chem. 8, 583270. doi:10.3389/fchem.2020.583270
Fan, T., Du, Y., Yao, Y., Wu, J., Meng, S., Luo, J., et al. (2018). Rolling Circle Amplification Triggered Poly Adenine-Gold Nanoparticles Production for Label-Free Electrochemical Detection of Thrombin. Sensors Actuators B: Chem. 266, 9–18. doi:10.1016/j.snb.2018.03.112
Giang, N. N., Won, H. J., Lee, G., and Park, S. Y. (2021). Cancer Cells Targeted Visible Light and Alkaline Phosphatase-Responsive TiO2/Cu2+ Carbon Dots-Coated Wireless Electrochemical Biosensor. Chem. Eng. J. 417, 129196. doi:10.1016/j.cej.2021.129196
Guo, Q., Han, J.-J., Shan, S., Liu, D.-F., Wu, S.-S., Xiong, Y.-H., et al. (2016). DNA-Based Hybridization Chain Reaction and Biotin-Streptavidin Signal Amplification for Sensitive Detection of Escherichia C O157:H7 through ELISA. Biosens. Bioelectron. 86, 990–995. doi:10.1016/j.bios.2016.07.049
He, H., Meng, S., Li, H., Yang, Q., Xu, Z., Chen, X., et al. (2021a). Nanoplatform Based on GSH-Responsive Mesoporous Silica Nanoparticles for Cancer Therapy and Mitochondrial Targeted Imaging. Microchim Acta 188, 154. doi:10.1007/s00604-021-04810-4
He, S., Huang, Q., Zhang, Y., Zhang, H., Xu, H., Li, X., et al. (2021b). Magnetic Beads-Based Multicolor Colorimetric Immunoassay for Ultrasensitive Detection of Aflatoxin B1. Chin. Chem. Lett. 32, 1462–1465. doi:10.1016/j.cclet.2020.09.047
Hou, K., Zhao, P., Chen, Y., Li, G., Lin, Y., Chen, D., et al. (2020). Rapid Detection of Bifidobacterium Bifidum in Feces Sample by Highly Sensitive Quartz Crystal Microbalance Immunosensor. Front. Chem. 8, 548. doi:10.3389/fchem.2020.00548
Huang, Q., Lin, X., Zhu, J.-J., and Tong, Q.-X. (2017). Pd-Au@carbon Dots Nanocomposite: Facile Synthesis and Application as an Ultrasensitive Electrochemical Biosensor for Determination of Colitoxin DNA in Human Serum. Biosens. Bioelectron. 94, 507–512. doi:10.1016/j.bios.2017.03.048
Huang, Q., Lin, X., Tong, L., and Tong, Q.-X. (2020). Graphene Quantum Dots/Multiwalled Carbon Nanotubes Composite-Based Electrochemical Sensor for Detecting Dopamine Release from Living Cells. ACS Sustain. Chem. Eng. 8, 1644–1650. doi:10.1021/acssuschemeng.9b06623
Jia, A., Liu, B., Liu, H., Li, Q., and Yun, Y. (2020). Interface Design of SnO2@PANI Nanotube with Enhanced Sensing Performance for Ammonia Detection at Room Temperature. Front. Chem. 8, 383. doi:10.3389/fchem.2020.00383
Kuang, Y., Zhang, J., Xiong, M., Zeng, W., Lin, X., Yi, X., et al. (2020). A Novel Nanosystem Realizing Curcumin Delivery Based on Fe3O4@Carbon Dots Nanocomposite for Alzheimer's Disease Therapy. Front. Bioeng. Biotechnol. 8, 614906. doi:10.3389/fbioe.2020.614906
Lee, H. C., Hsieh, E. Y., Yong, K., and Nam, S. (2020). Multiaxially-Stretchable Kirigami-Patterned Mesh Design for Graphene Sensor Devices. Nano Res. 13, 1406–1412. doi:10.1007/s12274-020-2662-7
Li, Z., Fu, Y., Fang, W., and Li, Y. (2015a). Electrochemical Impedance Immunosensor Based on Self-Assembled Monolayers for Rapid Detection of Escherichia C O157:H7 with Signal Amplification Using Lectin. Sensors 15, 19212–19224. doi:10.3390/s150819212
Li, Z., Yu, Y., Li, Z., Wu, T., and Yin, J. (2015b). The Art of Signal Transforming: Electrodes and Their Smart Applications in Electrochemical Sensing. Anal. Methods 7, 9732–9743. doi:10.1039/c5ay02373d
Li, Z., Ye, Z., Fu, Y., Xiong, Y., and Li, Y. (2016). A Portable Electrochemical Immunosensor for Rapid Detection of Trace Aflatoxin B1 in rice. Anal. Methods 8, 548–553. doi:10.1039/c5ay02643a
Li, Y., Liu, H., Huang, H., Deng, J., Fang, L., Luo, J., et al. (2020). A Sensitive Electrochemical Strategy via Multiple Amplification Reactions for the Detection of E. C O157: H7. Biosens. Bioelectron. 147, 111752. doi:10.1016/j.bios.2019.111752
Li, C., Cui, Y., Ren, J., Zou, J., Kuang, W., Sun, X., et al. (2021). Novel Cells-Based Electrochemical Sensor for Investigating the Interactions of Cancer Cells with Molecules and Screening Multitarget Anticancer Drugs. Anal. Chem. 93, 1480–1488. doi:10.1021/acs.analchem.0c03566
Lin, X., Xiong, M., Zhang, J., He, C., Ma, X., Zhang, H., et al. (2021). Carbon Dots Based on Natural Resources: Synthesis and Applications in Sensors. Microchemical J. 160, 105604. doi:10.1016/j.microc.2020.105604
Liu, L., Anwar, S., Ding, H., Xu, M., Yin, Q., Xiao, Y., et al. (2019). Electrochemical Sensor Based on F,N-Doped Carbon Dots Decorated Laccase for Detection of Catechol. J. Electroanalytical Chem. 840, 84–92. doi:10.1016/j.jelechem.2019.03.071
Liu, H., Yao, J., Guo, H., Cai, X., Jiang, Y., Lin, M., et al. (2020a). Tumor Microenvironment-Responsive Nanomaterials as Targeted Delivery Carriers for Photodynamic Anticancer Therapy. Front. Chem. 8, 758. doi:10.3389/fchem.2020.00758
Liu, Y., Zhou, M., Jin, C., Zeng, J., Huang, C., Song, Q., et al. (2020b). Preparation of a Sensor Based on Biomass Porous Carbon/Covalent-Organic Frame Composites for Pesticide Residues Detection. Front. Chem. 8, 643. doi:10.3389/fchem.2020.00643
Mao, X., Wan, Y., Li, Z., Chen, L., Lew, H., and Yang, H. (2020). Analysis of Organophosphorus and Pyrethroid Pesticides in Organic and Conventional Vegetables Using QuEChERS Combined with Dispersive Liquid-Liquid Microextraction Based on the Solidification of Floating Organic Droplet. Food Chem. 309, 125755. doi:10.1016/j.foodchem.2019.125755
Mao, X., Xiao, W., Wan, Y., Li, Z., Luo, D., and Yang, H. (2021). Dispersive Solid-Phase Extraction Using Microporous Metal-Organic Framework UiO-66: Improving the Matrix Compounds Removal for Assaying Pesticide Residues in Organic and Conventional Vegetables. Food Chem. 345, 128807. doi:10.1016/j.foodchem.2020.128807
Mo, X., Wu, Z., Huang, J., Zhao, G., and Dou, W. (2019). A Sensitive and Regenerative Electrochemical Immunosensor for Quantitative Detection of Escherichia C O157:H7 Based on Stable Polyaniline Coated Screen-Printed Carbon Electrode and rGO-NR-Au@Pt. Anal. Methods 11, 1475–1482. doi:10.1039/c8ay02594k
Nano, A., Furst, A. L., Hill, M. G., and Barton, J. K. (2021). DNA Electrochemistry: Charge-Transport Pathways through DNA Films on Gold. J. Am. Chem. Soc. 143, 11631–11640. doi:10.1021/jacs.1c04713
Qaanei, M., Taheri, R. A., and Eskandari, K. (2021). Electrochemical Aptasensor for Escherichia coli O157:H7 Bacteria Detection Using a Nanocomposite of Reduced Graphene Oxide, Gold Nanoparticles and Polyvinyl Alcohol. Anal. Methods 13, 3101–3109. doi:10.1039/d1ay00563d
Sri, S., Lakshmi, G. B. V. S., Gulati, P., Chauhan, D., Thakkar, A., and Solanki, P. R. (2021). Simple and Facile Carbon Dots Based Electrochemical Biosensor for TNF-α Targeting in Cancer Patient's Sample. Analytica Chim. Acta 1182, 338909. doi:10.1016/j.aca.2021.338909
Thakur, N., Thakur, S., Chatterjee, S., Das, J., and Sil, P. C. (2020). Nanoparticles as Smart Carriers for Enhanced Cancer Immunotherapy. Front. Chem. 8, 597806. doi:10.3389/fchem.2020.597806
Wu, X., Jiang, X., Fan, T., Zheng, Z., Liu, Z., Chen, Y., et al. (2020). Recent Advances in Photodynamic Therapy Based on Emerging Two-Dimensional Layered Nanomaterials. Nano Res. 13, 1485–1508. doi:10.1007/s12274-020-2750-8
Xie, Q.-Y., Wu, Y.-H., Xiong, Q.-R., Xu, H.-Y., Xiong, Y.-H., Liu, K., et al. (2014). Advantages of Fluorescent Microspheres Compared with Colloidal Gold as a Label in Immunochromatographic Lateral Flow Assays. Biosens. Bioelectron. 54, 262–265. doi:10.1016/j.bios.2013.11.002
Xu, Z., and Liu, Y. (2021). The Behavior of Carbonized Polymer Dots at the Nano‐Bio Interface and Their Luminescent Mechanism: A Physical Chemistry Perspective. Chin. J. Chem. 39, 265–273. doi:10.1002/cjoc.202000334
Ye, Y., Su, W., Zhang, J., Huang, Y., Chen, W., and Huang, Y. (2020). Development of a Combined Immunochromatographic Lateral Flow Assay for Accurate and Rapid Escherichia C O157:H7 Detection. Lett. Appl. Microbiol. 71, 311–319. doi:10.1111/lam.13297
Yi, X., Zeng, W., Wang, C., Chen, Y., Zheng, L., Zhu, X., et al. (2021). A Step-by-Step Multiple Stimuli-Responsive Metal-Phenolic Network Prodrug Nanoparticles for Chemotherapy. Nano Res. doi:10.1007/s12274-021-3626-2
You, Z., Qiu, Q., Chen, H., Feng, Y., Wang, X., Wang, Y., et al. (2020). Laser-Induced Noble Metal Nanoparticle-Graphene Composites Enabled Flexible Biosensor for Pathogen Detection. Biosens. Bioelectron. 150, 111896. doi:10.1016/j.bios.2019.111896
Zhang, C., and Du, X. (2020). Electrochemical Sensors Based on Carbon Nanomaterial Used in Diagnosing Metabolic Disease. Front. Chem. 8, 651. doi:10.3389/fchem.2020.00651
Zhang, M., Su, R., Zhong, J., Fei, L., Cai, W., Guan, Q., et al. (2019). Red/Orange Dual-Emissive Carbon Dots for pH Sensing and Cell Imaging. Nano Res. 12, 815–821. doi:10.1007/s12274-019-2293-z
Zhao, Y., Zeng, D., Yan, C., Chen, W., Ren, J., Jiang, Y., et al. (2020). Rapid and Accurate Detection of Escherichia C O157:H7 in Beef Using Microfluidic Wax-Printed Paper-Based ELISA. Analyst 145, 3106–3115. doi:10.1039/d0an00224k
Zhou, S., Lu, C., Li, Y., Xue, L., Zhao, C., Tian, G., et al. (2020). Gold Nanobones Enhanced Ultrasensitive Surface-Enhanced Raman Scattering Aptasensor for Detecting Escherichia C O157:H7. ACS Sens. 5, 588–596. doi:10.1021/acssensors.9b02600
Zhu, F., Zhao, G., and Dou, W. (2018). A Non-Enzymatic Electrochemical Immunoassay for Quantitative Detection of Escherichia coli O157:H7 Using Au@Pt and Graphene. Anal. Biochem. 559, 34–43. doi:10.1016/j.ab.2018.08.016
Zhuang, Z., Huang, J., Li, Y., Zhou, L., and Mai, L. (2019a). The Holy Grail in Platinum‐Free Electrocatalytic Hydrogen Evolution: Molybdenum‐Based Catalysts and Recent Advances. ChemElectroChem 6, 3570–3589. doi:10.1002/celc.201900143
Zhuang, Z., Li, Y., Huang, J., Li, Z., Zhao, K., Zhao, Y., et al. (2019b). Sisyphus Effects in Hydrogen Electrochemistry on Metal Silicides Enabled by Silicene Subunit Edge. Sci. Bull. 64, 617–624. doi:10.1016/j.scib.2019.04.005
Keywords: Escherichia coli O157:H7, electrochemical biosensing interface, carbon dots, Fe3O4 nanomaterial, DNA biosensor
Citation: Lin X, Mei Y, He C, Luo Y, Yang M, Kuang Y, Ma X, Zhang H and Huang Q (2021) Electrochemical Biosensing Interface Based on Carbon Dots-Fe3O4 Nanomaterial for the Determination of Escherichia coli O157:H7. Front. Chem. 9:769648. doi: 10.3389/fchem.2021.769648
Received: 02 September 2021; Accepted: 05 October 2021;
Published: 19 November 2021.
Edited by:
Zhong Feng Gao, Linyi University, ChinaReviewed by:
Miaomiao Yuan, Sun Yat-sen University, ChinaCopyright © 2021 Lin, Mei, He, Luo, Yang, Kuang, Ma, Zhang and Huang. This is an open-access article distributed under the terms of the Creative Commons Attribution License (CC BY). The use, distribution or reproduction in other forums is permitted, provided the original author(s) and the copyright owner(s) are credited and that the original publication in this journal is cited, in accordance with accepted academic practice. No use, distribution or reproduction is permitted which does not comply with these terms.
*Correspondence: Huifang Zhang, emhhbmdodWlmYW5nOTNAMTYzLmNvbQ==; Qitong Huang, aHF0QGdtdS5lZHUuY24=, aHF0Ymx1ZUAxNjMuY29t
Disclaimer: All claims expressed in this article are solely those of the authors and do not necessarily represent those of their affiliated organizations, or those of the publisher, the editors and the reviewers. Any product that may be evaluated in this article or claim that may be made by its manufacturer is not guaranteed or endorsed by the publisher.
Research integrity at Frontiers
Learn more about the work of our research integrity team to safeguard the quality of each article we publish.