- Key Laboratory of Advanced Energy Materials Chemistry (Ministry of Energy), College of Chemistry, Nankai University, Tianjin, China
The synthesis and characterization of a novel florescent chemosensor 1 with two different types of cationic binding sites have been reported in this work, which is a calix[4]crown derivative in 1,3-alternate conformation bearing two 2-phenyl-5-(4-dimethylaminopyenyl)-1,3,4-oxadiazole units. The recognition behaviors of 1 in dichloromethane/acetonitrile solution to alkali metal ions (Na+ and K+), alkaline earth metal ions (Mg2+ and Ca2+), and transition metal ions (Co2+, Ni2+, Zn2+, Cd2+, Cu2+, Mn2+, and Ag+) have been investigated by UV-Vis and fluorescence spectra. The fluorescence of 1 might be quenched selectively by Cu2+ due to the photo-induced electron transfer mechanism, and the quenched emission from 1 could be partly revived by the addition of Ca2+ or Mg2+; thus, the receptor 1 might be worked as an on–off switchable fluorescent chemosensor triggered by metal ion exchange.
Introduction
As the third most abundant transition metal ion after zinc and iron in the human body, copper is required by many living organisms for normal physiological processes (Turski and Thiele, 2009; Cotruvo Jr et al., 2015). Maintaining optimal concentration of Cu2+ ion for living cells is an essential factor to keep the normal functioning of enzymes and intracellular metabolic balance. Thus, the development of new fluorescent chemosensors for Cu2+ ion has drawn continuous interest during the past decades. The main progress in this area has been well reviewed (Cao et al., 2019; Sivaraman et al., 2018; Udhayakumari et al., 2017; Liu et al., 2017), and many fluorescent chemosensors for Cu2+ ion based on various fluorophores such as coumarin (Zhang et al., 2019), Bodipy (Ömeroğlu et al., 2021), rhodamine (Fernandes and Raimundo, 2021), Schiff base (Singh et al., 2020), pyrene (Kowser et al., 2021), and 1,3,4-oxadiazole (Wang, et al., 2018) have been reported by different research groups. Among these fluorescent chemosensors, the 1,3,4-oxadiazoles have drawn special interest due to their electron-deficient nature, high photoluminescence quantum yield, and excellent chemical stability, and have found practical applications in the fields of organic light-emitting diodes (Meng et al., 2020) and liquid crystals (Han et al., 2013; Han et al., 2015; Han et al., 2018). In addition, the nitrogen and oxygen atoms of the 1,3,4-oxadiazole unit can provide potential coordination sites with metal ions, which makes it usable as a signaling component in fluorescent chemosensors.
Calixarenes, as one kind of the most important super-molecules, have been widely used in design of fluorescent chemosensors for ions and neutral molecules due to their outstanding features such as preorganized binding sites, easy derivatization, and flexible three-dimensional structures (Kim et al., 2012; An et al., 2019; Miranda et al., 2019; Noruzi et al., 2019; Chen et al., 2020). Many calixarene-based fluorescent chemosensors for transition metal ions have been reported in recent years (Ma et al., 2015). However, the fluorescent switchable chemosensors triggered by different ions are quite few (Chung et al., 2007), which remains a challenge in the field of supramolecular chemistry. Herein, as part of our continuous research in the design and synthesis of new fluorescent chemosensors (Liu et al., 2021; Xie et al., 2016; Han et al., 2012), we utilize the 1,3-alternate calix[4]crown scaffold to construct an on–off switchable fluorescent chemosensor 1 in this work. The synthetic route for 1 is shown in Scheme 1. There are quite a number of chemosensors based on various macrocycles for copper detection reported in literatures (Lvova, et al., 2018; Doumani, et al., Kamei, et al., 2021), in which the macrocyles often only worked as receptors for Cu2+ ions. In contrast, the chemosensor 1 in this work is special in that it has two kinds of macrocycles: one is from the 1,3-alternate calixarene, which provides a three-dimensional scaffold with two appending 1,3,4-oxadiazole units as both signaling component and fluorophore; the other is from the calix[4]crown, which can bind the Mg2+ or Ca2+ ions and has an allosteric effect on the 1,3,4-oxadiazole units on opposite rings. The selective binding of 1,3,4-oxadiazole with Cu2+ ions results in the fluorescence quenching, while the binding of calix[4]crown with Mg2+ or Ca2+ ions can partly revive the fluorescence consequently. Thus, the compound 1 might work as a new type of switchable off–on fluorescent chemosensor.
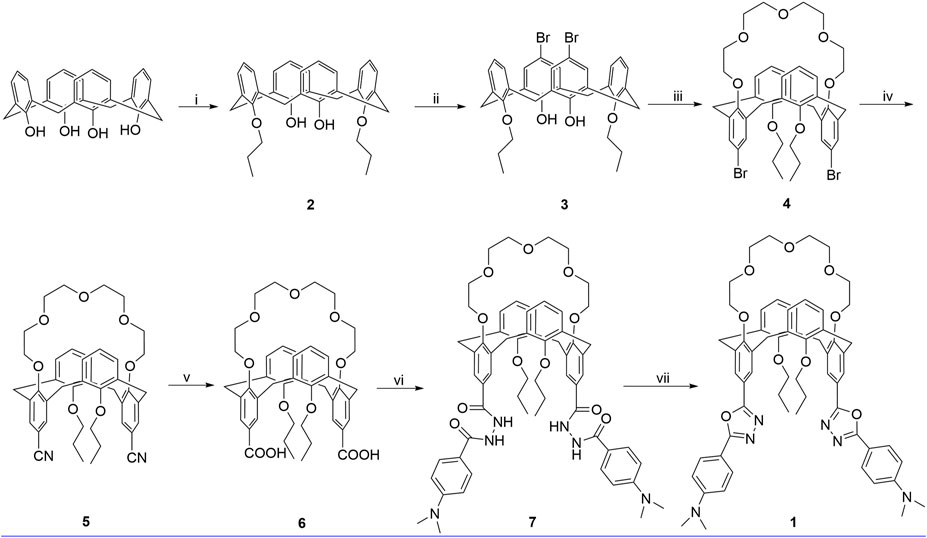
SCHEME 1. Synthetic route for 1, reagents, and conditions: (i) 1-iodopropane, K2CO3, CH3CN, reflux, 24 h; (ii) Br2, 0°C, 3 h; (iii) tetraethylene glycol ditosylate, Cs2CO3, CH3CN, reflux, 72 h; (iv) (1) CuCN, NMP, 180°C, 5 h; (2) FeCl3, 2 M HCl, 100°C, 1 h; (v) KOH, ethanol, reflux, 24 h; (vi) (1) SOCl2, toluene, reflux, 5 h; (2) 4-(dimethylamino)benzohydrazide, pyridine, r. t., 12 h; (vii) POCl3, reflux, 12 h.
Materials and Methods
25,27-Dihydroxy-26,28-dipropoxycalix[4]arene 2 and 5,17-dibromo-25,27-dihydroxy-26,28-dipropoxycalix[4]arene 3 were synthesized according to the literature procedures (Hobzova, 2010). Dichloromethane and acetonitrile used for photophysical studies were of spectrometric grade. All the other chemicals and solvents were of analytical grade and used as received from commercial sources. The solutions of metal ions were all prepared from their perchlorate salts. Column chromatography was performed on silica gel (200–300 mesh).
Solution 1H NMR (Proton Nuclear Magnetic Resonance) and 13C NMR (Carbon-13 Nuclear Magnetic Resonance) spectra were recorded on a Bruker AV400 spectrometer and the chemical shifts are quoted in parts per million (ppm) relative to tetramethylsilane (TMS) as an internal standard. ESI-HRMS (Electrospray Ionization-High Resolution Mass Spectrometry) data were obtained with a FTICR-MS mass spectrometer. Melting points were determined with an X-4 melting point apparatus, and the thermometer was uncorrected. Data for single x-ray structure were collected on a SMART1000 CCD-X diffractometer with graphite-monochromatized MoKα x-ray radiation (λ = 0.71073 Å) and Saturn CCD area detector. The x-ray crystal structure of 4 was solved by direct method and expanded using Fourier synthesis technique. No absorption correction was done. The non-hydrogen atoms were refined anisotropically. Hydrogen atoms were refined using riding model. Structural refinement based on full-matrix least-squares refinement on |F|2 was performed by using Crystal Structure or SHELXL97 suite program (Sheldrick, 1997).
Synthesis of 4
A mixture of 3 (9.23 g, 13.9 mmol) and Cs2CO3 (11.30 g, 34.7 mmol) in MeCN (700 ml) under nitrogen was stirred at reflux for 30 min and then a solution of the tetraethylene glycol ditosylate (7.85 g, 15.6 mmol) in MeCN (40 ml) was added during an hour. The mixture was refluxed for 72 h and allowed to cool to room temperature. After evaporation of the solvent in vacuo, the residue was taken up in CH2Cl2 (30 ml × 3) and the resultant solution was washed with 1 mol/L HCl (30 ml) and brine (30 ml × 2). The organic layer was dried over MgSO4 and evaporated in vacuo. Recrystallization of the residue from CH2Cl2/MeOH gave 4 a pale-yellow solid. Yield, 75%. Mp: 230–232 °C. 1H NMR (400 MHz, CDCl3) δ 7.17 (s, 4H), 7.08 (d, J = 8.0 Hz, 4H), 6.87 (t, J = 8.0 Hz, 2H), 3.78 (d, J = 4.0 Hz, 8H), 3.54 (s,8H), 3.45 (t, J = 8.0 Hz, 4H), 3.25–3.20 (m, 4H), 3.15 (m, 4H), 1.31 (m, 4H), 0.78 (t, J = 7.5 Hz, 6H). 13C NMR (101 MHz, CDCl3) δ 156.97, 155.14, 136.29, 133.81, 132.17, 129.70, 122.77, 115.15, 72.59, 72.09, 70.50, 70.13, 69.03, 37.89, 22.53, 10.23. HRMS-MALDI calculated for C42H48Br2O7 [M + Na] + 847.1639, found 847.1652.
Synthesis of 5
Under nitrogen, a mixture of 4 (10.17 g, 12.3 mmol) and cuprous cyanide (7.68 g, 86.4 mmol) in 20 ml of 1-methyl-2-pyrrolidinone was stirred at 180°C for 4 h. Then, the reaction mixture was cooled slowly to 100°C, and a solution of 23.23 g (86.4 mmol) of FeCl3·6H2O in 5 ml of concentrated hydrochloride and 25 ml of water was added to the reaction mixture. The reaction mixture was further stirred at 100°C for 1 h and cooled to room temperature. The solid was filtered off and recrystallized from chloroform/hexane yielding 5.5 g of compound 5 as yellow solid. Yield 62%. 1H NMR (400 MHz, CDCl3) δ 7.36 (s, 4H), 7.10 (d, J = 7.4 Hz, 4H), 6.90 (s, 2H), 3.82 (d, J = 5.5 Hz, 8H), 3.55 (s, 8H), 3.46 (t, J = 7.4 Hz, 4H), 3.30 (t, J = 6.1 Hz, 4H), 3.17 (t, J = 6.1 Hz, 4H), 1.24 (m, 4H), 0.74 (t, J = 7.5 Hz, 6H).13C NMR (101 MHz, CDCl3) δ 159.98, 156.80, 135.72, 133.54, 133.05, 129.94, 123.05, 119.17, 106.08, 77.36, 72.52, 72.17, 70.56, 69.85, 69.13, 37.75, 22.81, 10.05; HRMS: calcd for C44H48N2O7 [M+NH4]+ 734.3800, found 734.3796.
Synthesis of 6
A solution of 5.18 g (9.2 mmol) of KOH in 100 ml of water was added to the suspension of 1.32 g (1.80 mmol) of 5 in 20 ml of ethanol. The reaction mixture was heated under reflux for 24 h. After cooling, the aqueous solution hydrogen chloride (10% w/w) was added dropwise until the solution became slightly acidic. The precipitate was filtered off, washed with water, and dried to yield a yellow solid product 6 (1.33 g, 96%). Mp: 296–298°C. 1H NMR (400 MHz, CDCl3) δ 12.52 (s, 2H), 7.81–7.77 (m, 8H), 6.98–6.94 (m, 2H), 3.93–3.80 (m, 12H), 3.62 (s, 16H), 1.42–1.31 (m, 4H), 0.69 (t, J = 7.5 Hz, 6H).13C NMR (101 MHz, DMSO-d6) δ 167.03, 158.48, 156.72, 135.62, 133.71, 132.13, 130.41, 125.24, 122.05, 71.17, 70.07, 69.72, 40.15, 39.94, 39.73, 39.52, 39.31, 39.10, 38.89, 35.98, 21.74, 9.52. HRMS: calcd for C44H50O11 [M-H]+ 753.3280, found 753.3285.
Synthesis of 1
To a round-bottomed flask was added 6 (80 mg, 0.1 mmol), 10 ml of toluene, and 1 ml of thionyl chloride, and the mixture was refluxed for 5 h. After cooling, the solvent and the excess of thionyl chloride were removed at reduced pressure to give the benzoyl chloride, which was added to a solution of 4-(dimethylamino)benzohydrazide (39 mg, 0.22 mmol) in 10 ml of dichloromethane and 0.1 ml of pyridine. The reaction mixture was stirred for 12 h at ambient temperature and filtered. The precipitate was washed with ethanol to give the bishydrazide 7 as white solid, which was used to the next step reaction without further purification. The intermediate compound 7 was added to POCl3 (5 ml), and the resultant solution was refluxed overnight under a nitrogen atmosphere. After the reaction mixture cooled to room temperature, it was poured into ice water and extracted with dichloromethane (3 × 10 ml). The combined organic layer was washed with water and brine, respectively. Then, the solvent was removed under reduced pressure, and the crude solid was purified by silica gel column chromatography using petroleum ether/ethyl acetate (1:1) as eluent affording the product 1 as white solids. Yield, 35%. Mp: 281–283°C. 1H NMR (400 MHz, CDCl3) δ 7.91 (d, J = 8.0 Hz, 4H), 7.81 (s, 4H), 7.15 (d, J = 8.0 Hz, 4H), 6.93 (s, 2H), 6.72 (d, J = 8.0 Hz, 4H), 3.93 (m, 8H), 3.59–3.53 (m, 12H), 3.31 (d, J = 5.2 Hz, 4H), 3.26 (d, J = 5.2 Hz, 4H), 3.05 (m, 12H), 1.25–1.19 (m, 4H), 0.60 (t, J = 7.4 Hz, 6H). 13C NMR (101 MHz, CDCl3) δ 164.80, 163.55, 158.76, 157.10, 152.20, 135.07, 133.83, 129.79, 128.32, 128.13, 122.75, 121.14, 118.35, 111.59, 111.27, 72.43, 72.24, 70.42, 70.14, 69.22, 40.11, 38.05, 22.43, 9.95. HRMS-ESI calculated for C62H69N6O9 [M + H] + 1,041.5120, found 1,041.5126. (Supplementary Figure S5, ESI).
General Procedures for the UV/Vis and Fluorescence Experiments
UV-vis spectra were recorded on a Cary 3,010 spectrophotometer, and the resolution was set at 1 nm. Steady-state emission spectra were recorded on a Varian Cary Eclipse spectrometer. For all measurements of fluorescence spectra, excitation was set at 334 nm for complexation, and the excitation and emission slit width was set to be 2.5 nm. Fluorescence titration experiments were performed with CH2Cl2 solutions of compound 1 and varying concentrations of metal perchlorate in CH3CN solution. During all measurements, the temperature of the quartz sample cell and chamber was kept at 25°C.
Results and Discussion
Synthesis and Structural Analysis
As shown in Scheme 1, calix[4]arene 3 was reacted with tetraethylene glycol ditosylate in the presence of Cs2CO3 to successfully afford the calix[4]crown 3 in 75% yield. The substitution reaction of 4 with CuCN gave 5 in 62% yield, which was refluxed with KOH in ethanol and treated with hydrochloric acid solution, readily providing the carboxylic acid 6 in good yield. Then, the carboxylic acid 6 was reacted with thionyl chloride, and treated with benzyol hydrazine or 4-N,N′-dimethylaminobenzyol hydrazine to generate the intermediate bishydrazide 7, which was used in the next step without purification and refluxed with phosphorus oxychloride to afford the target products 1. Except for the calix[4]arene 3, all of the intermediate calix[4]crowns 3–6 and the chemosensor 1 are in 1,3-alternate conformation, which were well established by 1H NMR and 13C NMR data (Supplementary Figures S1–S4, ESI). The 1,3-alternate conformation of 5 was further confirmed unambiguously by x-ray single crystal diffraction as shown in Figure 1. The x-ray crystallographic data are collected in Supplementary Table S1.
UV-Vis Absorption and Fluorescence Spectra Analysis
The selectivity of the receptor 1 toward different perchlorate salts, including Na+, K+, Mg2+, Ca2+, Co2+, Ni2+, Zn2+, Cd2+, Mn2+, Ag+, and Cu2+, was first investigated by UV-Vis spectroscopy. The UV-Vis absorption spectra for free 1 in CH2Cl2 solution showed an intense and structureless absorption band (ε = 4.94 × 105 L/mol·cm) peaking at 340 nm (Figure 2), which might have resulted from the spin-allowed π-π* transitions involving the phenyloxadiazole moiety (Han et al., 2006). The addition of Cu2+ ions in the solution of 1 resulted in a significant decrease in the absorbance with an appreciable hypochromic shift of 20 nm. In contrast, only a slight decrease was observed upon addition of other metal ions mentioned above, which suggested that the selectivity of 1 toward Cu2+ is much higher than the other metal ions.
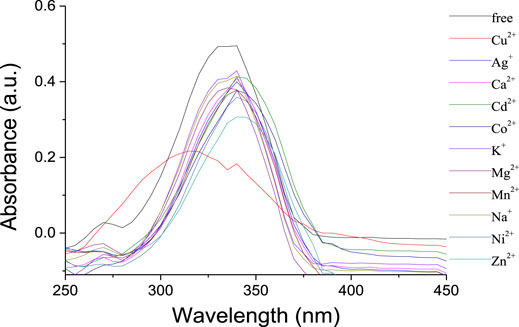
FIGURE 2. UV-vis spectra of 1 (1 × 10−6 mol/L) upon addition of metal ions (10 equiv) in CH2Cl2/CH3CN (1,000:1, v/v).
Ion recognition ability of 1 was further studied by the fluorescence spectra. As shown in Figure 3, the receptor 1 exhibited a strong emission with λmax at 405 nm in solution of CH2Cl2. Upon addition of Na+, K+, and Mg2+, respectively, almost no changes were observed in the intensity and shape of the emission spectra of 1. It is noted that the addition of Ca2+ might slightly increase the intensity with a bathochromic shift of ca. 15 nm, perhaps because the complexation between the Ca2+ and the crown ether moiety changed the space distance of the two phenyloxadiazole units and the fluorescence changed consequently. Apparently, the fluorescence response of 1 toward transition metal ions was found to be more pronounced, and the addition of Co2+, Ni2+, Zn2+, and Ag+ could quench the emission of 1 in a different extent, accompanied by a concomitant red shift of ca. 14–17 nm. In contrast, the addition of Cu2+ significantly quenched the fluorescence of 1 under the same conditions as the aforementioned metal ions, suggesting that there is a strong interaction between 1,3,4-oxadiazole moieties of 1 and Cu2+ ion over the other metal ions.
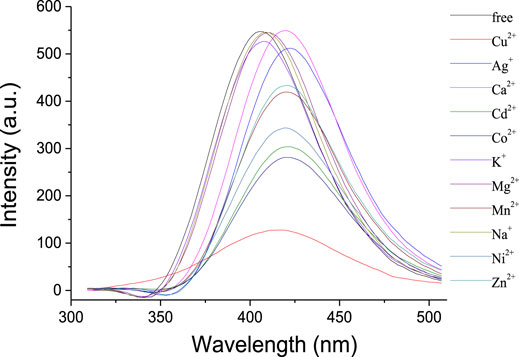
FIGURE 3. Fluorescence spectra (λexc = 334 nm, Slit = 2.5) of 1 (1 × 10−6 mol/L) upon addition of metal ions (10 equiv) in CH2Cl2/CH3CN (1,000:1, v/v).
The fluorescence emission properties of 1 in the presence of Cu2+ and a competitive metal ion were measured to investigate the selective recognition for Cu2+. As shown in Figure 4, no apparent changes were observed in fluorescence intensity when 10 equivalent amounts of transition metal ions (Co2+, Ni2+, Zn2+, Cd2+, Mn2+, and Ag+) were added to the solution of 1 and Cu2+ (10 equiv). This suggested that the recognition for Cu2+ was not interrupted by the competitive transition metal ions; thus, the receptor 1 might act as a selective fluorescent chemosensor for Cu2+. The addition of alkali metal ions (Na+ and K+) to the solution of 1 and Cu2+ could increase the fluorescence intensity slightly, while the alkaline earth metal ions (Mg2+ and Ca2+) could revive the emission significantly.
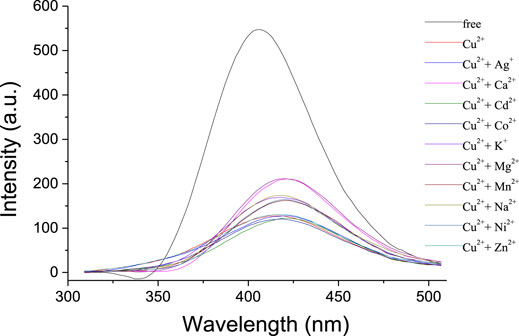
FIGURE 4. Fluorescence spectra (λexc = 334 nm, Slit = 2.5) of 1 (1 × 10−6 mol/L) and Cu2+ (10 equiv) upon addition of other metal ions (10 equiv) in CH2Cl2/CH3CN (1,000:1, v/v).
In order to elicit the binding property of the chemosensor 1 toward Cu2+ ion, fluorescence titration of 1 (1.0 × 10–5 mol/L) with Cu2+ ion (0–2 equiv) was carried out (Supplementary Figure S6). According to the fluorescence titration curves of 1 with Cu2+ ion at room temperatures, the association constant Ka was calculated as 1.6 × 10–4 L·mol−1 (R = 0.97526) for the 1–Cu2+ complex by the Benesi–Hildebrand plot (Thordarson, 2011) (Figure 5). Moreover, the emission intensity of 1 is linearly proportional to the Cu2+ concentration in the range of 0–20 μM.
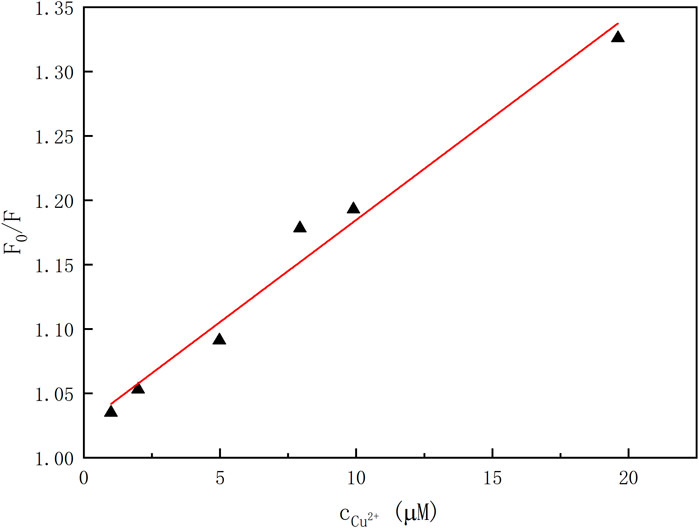
FIGURE 5. Plot of emission intensity versus the concentrations of Cu2+ ion (λem = 405 nm, λex = 334 nm).
The fluorescence changes of 1 upon addition of Cu2+ and Mg2+ ions are displayed in Figure 6. The nitrogen atoms of the 1,3,4-oxadiazle units can bind with Cu2+ to form the complex 1·Cu2+, and the paramagnetic nature of Cu2+ ion could strongly quench the fluorescence of the 1,3,4-oxadiazole units through the electron transfer mechanism, which is consistent to the results reported in literature (Han et al., 2012). In contrast, the polyether ring (crown-5 moiety) and the oxygens from the two propoxyl groups could provide coordination sites with the alkaline earth metal ions to form the complex 1·Mg2+, which will change the molecular conformation as well as the space distance of the two 1,3,4-oxadiazole units. Consequently, the decomplexations between the 1,3,4-oxadiazoles and Cu2+ ions took place and resulted in the increase of the fluorescence. Thus, the receptor 1 might be acted as an on–off–on switchable fluorescent chemosensor triggered by the exchange of Cu2+ and Mg2+.
To gain a better understanding about the switchable fluorescence of the chemosensor 1, DFT calculations with the GAUSSIAN 09 series of programs (Frisch et al., 2013) were carried out to analyze the molecular structures of 1 and 1·Mg2+, and DFT method B3-LYP with 6-31G(d) basis set was used for geometry optimizations (A. D. Becke, 1993). As shown in Figure 7, the distance between N1 and N2 in the free receptor 1 is 9.97 Ǻ, while the corresponding distance is 11.74 Ǻ in the complex 1·Mg2+, indicating that the molecular conformation changed simultaneously due to the allosteric effect (Kumar et al., 2012; Ni et al., 2013). The conformational change as well as the increase in distance makes it difficult for the chemosensor 1 to coordinate with Cu2+ ion to form the stable complex, which reasonably explains the fact that the addition of Mg2+ ions to the solution of 1 and Cu2+ can trigger the revival of fluorescence.
Conclusion
In summary, we have designed a new type of fluorescent chemosensor based on a 1,3-alternate calix[4]crown with two different cationic binding sites. The 1,3,4-oxadiazole units could bind selectively with Cu2+ to form the complexation and resulted in the fluorescence quenching of the chemosensor. The presence of various transition metal ions does not interfere with the quenching process, while the alkaline earth metal ions Mg2+ might be entrapped by the crown-5 moiety and revive the fluorescence significantly due to the allosteric effect. As the chemosensor in this work is not soluble in water, it is difficult to investigate the Cu2+ ions’ detection under physiological conditions. Devising a water-soluble chemosensor for Cu2+ ions is in progress in our lab.
Data Availability Statement
The original contributions presented in the study are included in the article/Supplementary Material, further inquiries can be directed to the corresponding author.
Author Contributions
JH designed the work and wrote the manuscript. CS synthesized and characterized the compounds. CS and SD carried out the UV-Vis absorption and fluorescence studies. TZ carried out the DFT calculations. JH revised and edited the manuscript. All authors contributed to discussion on the results for the manuscript.
Funding
This work was financially supported by the National Natural Science Foundation of China (No. 21272130) and the 111 Project (grant no. B12015).
Conflict of Interest
The authors declare that the research was conducted in the absence of any commercial or financial relationships that could be construed as a potential conflict of interest.
Publisher’s Note
All claims expressed in this article are solely those of the authors and do not necessarily represent those of their affiliated organizations, or those of the publisher, the editors and the reviewers. Any product that may be evaluated in this article, or claim that may be made by its manufacturer, is not guaranteed or endorsed by the publisher.
Supplementary Material
The Supplementary Material for this article can be found online at: https://www.frontiersin.org/articles/10.3389/fchem.2021.766442/full#supplementary-material
References
An, L., Wang, C., Han, L., Liu, J., Huang, T., Zheng, Y., et al. (2019). Structural Design, Synthesis, and Preliminary Biological Evaluation of Novel Dihomooxacalix[4]arene-Based Anti-tumor Agent. Front. Chem. 7, 856. doi:10.3389/fchem.2019.00856
Becke, A. D. (1993). Density-functional Thermochemistry. III. The Role of Exact Exchange. J. Chem. Phys. 98, 5648. doi:10.1063/1.464913
Cao, X., Li, Y., Gao, A., Yu, Y., Zhou, Q., and Chang, X. (2019). Multifunctional Fluorescent Naphthalimide Self-Assembly System for the Detection of Cu2+ and K+ and Continuous Sensing of Organic Amines and Gaseous Acids. J. Mater. Chem. C 7, 10589–10597. doi:10.1039/c9tc03243f
Chang, K.-C., Su, I.-H., Senthilvelan, A., and Chung, W.-S. (2007). Triazole-modified Calix[4]crown as Novel Fluorescent On-Off Switchable Chemosensor. Org. Lett. 9, 3363–3366. doi:10.1021/ol071337+
Chen, Y.-J., Chen, M.-Y., Lee, K.-T., Shen, L.-C., Hung, H.-C., Niu, H.-C., et al. (2020). 1,3-Alternate Calix[4]arene Functionalized with Pyrazole and Triazole Ligands as a Highly Selective Fluorescent Sensor for Hg2+ and Ag+ Ions. Front. Chem. 6, 593261. doi:10.3389/fchem.2019.59326110.3389/fchem.2020.593261
Cotruvo, Jr., J. A., Aron, A. T., Ramos-Torres, K. M., and Chang, C. J. (2015). Synthetic Fluorescent Probes for Studying Copper in Biological Systems. Chem. Soc. Rev. 44, 4400–4414. doi:10.1039/c4cs00346b
Doumani, N., Bou-Maroun, E., Maalouly, J., Tueni, M., Dubois, A., Bernhard, C., et al. (2019). A New pH-dependent Macrocyclic Rhodamine B-Based Fluorescent Probe for Copper Detection in white Wine. Sensors 19, 4514. doi:10.3390/s19204514
Fernandes, R. da. S., and Raimundo, I. M. (2021). Development of a Reusable Fluorescent Nanosensor Based on Rhodamine B Immobilized in Stober Silica for Copper Ion Detection. Anal. Methods 13, 1970–1975. doi:10.1039/d1ay00168j
Frisch, M. J., Trucks, G. W., Schlegel, H. B., Scuseria, G. E., Robb, M. A., Cheeseman, J. R., et al. (2013). Gaussian 09, Revision D.01. Wallingford, CT: Gaussian, Inc.
Han, J. (2013). 1,3,4-Oxadiazole Based Liquid Crystals. J. Mater. Chem. C. 1, 7779–7797. doi:10.1039/c3tc31458h
Han, J., Chui, S. S. Y., and Che, C. M. (2006). Thermotropic Liquid Crystals Based on Extended 2,5-Disubstituted-1,3,4-Oxadiazoles: Structure-Property Relationships, Variable-Temperature Powder X-ray Diffraction, and Small-Angel X-ray Scattering Studies. Chem. Asian J. 1, 814–825. doi:10.1002/asia.200600252
Han, J., Wang, F.-L., Liu, Y.-X., Zhang, F.-Y., Meng, J.-B., and He, Z.-J. (2012). Calix[4]arene-based 1,3,4-oxadiazole: Novel Fluorescent Chemosensors for Specific Recognition of Cu2+. ChemPlusChem 77, 196–200. doi:10.1002/cplu.201200004
Han, J., Wang, Z.-Z., Wu, J., and Zhu, L.-R. (2015). A Room-Temperature Liquid Crystalline Polymer Based on Discotic 1,3,4-oxadiazole. RSC Adv. 5, 47579–47583. doi:10.1039/c5ra05983f
Han, J., Xi, Z., Wang, F., Bu, L., and Wang, Y. (2018). Synthesis, Liquid Crystalline and Photoluminscent Properties of 1,3,4-oxadiazole Derivatives: from Calamitic Monomers, H-Shaped Dimers to Calix[4]arene-Based Tetramers. Dyes Pigm. 154, 234–241. doi:10.1016/j.dyepig.2018.03.008
Hobzova, R., Sysel, P., and Duskova-Smrckova, M. (2010). Synthesis and Characterization of Calix[4]arene-Containing Polyimides. Polym. Int. 60, 405–413. doi:10.1002/pi.2962
Kamel, A. H., Amr, A. E.-G. E., Almehizia, A. A., Elsayed, E. A., and Moustafa, G. O. (2021). Low-cost Potentiometric Paper-Based Analytical Device Based on Newly Synthesized Macrocyclic Pyrido-Pentapeptide Derivatives as Novel Ionophores for point-of-care Copper(II) Determination. RSC Adv. 11, 27174–27182. doi:10.1039/d1ra04712d
Kim, H. J., Min Hee Lee, M. H., Mutihac, L., Vicens, J., and Kim, J. S. (2012). Host–guest Sensing by Calixarenes on the Surfaces. Chem. Soc. Rev. 41, 1173–1190. doi:10.1039/c1cs15169j
Kowser, Z., Rayhan, U., Akther, T., Redshaw, C., and Yamato, T. (2021). A Brief Review on Novel Pyrene Based Fluorometric and Colorimetric Chemosensors for the Detection of Cu2+. Mater. Chem. Front. 5, 2173–2200. doi:10.1039/D0QM01008A
Kumar, M., Kumar, N., and Bhalla, V. (2012). Ratiometric Nanomolar Detection of Cu2+ Ions in Mixed Aqueous media: a Cu2+/Li+ Ions Switchable Allosteric System Based on Thiacalix[4]crown. Dalton Trans. 41, 10189–10193. doi:10.1039/c2dt31081c
Liu, S., Wang, Y.-M., and Han, J. (2017). Fluorescent Chemosensors for Copper(II) Ion: Structure, Mechanism and Application. J. Photochem. Photobio. C. 32, 78–103. doi:10.1016/j.jphotochemrev.2017.06.002
Liu, S., Wu, Q., Zhang, T., Zhang, H., and Han, J. (2021). Supramolecular brush Polymers Prepared from 1,3,4-oxadiazole and Cyanobutoxy Functionalized Pillar[5]arene for Detecting Cu2+. Org. Biomol. Chem. 19, 1287–1291. doi:10.1039/d0ob02587a
Lvova, L., Caroleo, F., Garau, A., Lippolis, V., Giorgi, L., Fusi, V., et al. (2018). A Fluorescent Sensor Array Based on Heteroatomic Macrocyclic Fluorophores for the Detection of Polluting Species in Natural Water Samples. Front. Chem. 6, 258. doi:10.3389/fchem.2018.00258
Ma, J., Song, M., Boussouar, I., Tian, D., and Li, H. (2015). Recent Progress of Calixarene-Based Fluorescent Chemosensors towards Mercury Ions. Supramole. Chem. 27, 444–452. doi:10.1080/10610278.2014.988627
Meng, X., Wang, P., Bai, R., and He, L. (2020). Blue-green-emitting Cationic Iridium Complexes with Oxadiazole-type Counter-anions and Their Use for Highly Efficient Solution-Processed Organic Light-Emitting Diodes. J. Mater. Chem. C 8, 6236–6244. doi:10.1039/d0tc01054e
Miranda, A. S., Serbetci, D., Marcos, P. M., Ascenso, J. R., Berberan-Santos, M. N., Hickey, N., et al. (2019). Ditopic Receptors Based on Dihomooxacalix[4]arenes Bearing Phenylurea Moieties with Electron-Withdrawing Groups for Anions and Organic Ion Pairs. Front. Chem. 7, 758. doi:10.3389/fchem.2019.00758
Ni, X.-L., Cong, H., Yoshizawa, A., Rahman, S., Tomiyasu, H., Rayhan, U., et al. (2013). Heteroditopic Thiacalix[4]arene Receptor Having Ester and Bipyridyl Moieties for Ions Binding with Positive/negative Allosteric Effect. J. Mol. Struc. 1046, 110–115. doi:10.1016/j.molstruc.2013.04.040
Noruzi, E. B., Kheirkhahi, M., Shaabani, B., Geremia, S., Hickey, N., Asaro, F., et al. (2019). Design of a Thiosemicarbazide-Functionalized Calix[4]arene Ligand and Related Transition Metal Complexes: Synthesis, Characterization, and Biological Studies. Front. Chem. 7, 663. doi:10.3389/fchem.2019.00663
Ömeroğlu, İ., Tümay, S. O., Makhseed, S., Husain, A., and Durmuş, M. (2021). A Highly Sensitive “ON–OFF–ON” Dual Optical Sensor for the Detection of Cu(II) Ion and Triazole Pesticides Based on Novel BODIPY-Substituted Cavitand. Dalton Trans. 50, 6437–6443. doi:10.1039/d1dt00792k
Singh, G., Shilpy, , Singh, A., Diksha, , and Pawan, (2020). Synthesis of Organosilocane Allied N-Heteroaryl Schiff Base Chemosensor for the Detection of Cu2+ Metal Ions and Their Biological Applications. New J. Chem. 44, 13542–13552. doi:10.1039/d0nj01774d
Sivaraman, G., Iniy, M., Anand, T., Kotla, N. G., Sunnapu, O., Singaravadivel, S., et al. (2018). Chemically Diverse Small Molecule Fluorescent Chemosensors for Copper Ion. Coord. Chem. Rev. 357, 50–104. doi:10.1016/j.ccr.2017.11.020
Thordarson, P. (2011). Determining Association Constants from Titration Experiments in Supramolecular Chemistry. Chem. Soc. Rev. 40, 1305–1323. doi:10.1039/c0cs00062k
Turski, M. L., and Thiele, D. J. (2009). New Roles for Copper Metabolism in Cell Proliferation, Signaling, and Disease. J. Biol. Chem. 284, 717–721. doi:10.1074/jbc.R800055200
Udhayakumari, D., Naha, S., and Velmathi, S. (2017). Colorimetric and Fluorescent Chemosensors for Cu2+. A Comprehensive Review from the Years 2013-15. Anal. Methods 9, 552–578. doi:10.1039/c6ay02416e
Wang, L., Gong, X., Bing, Q., and Wang, G. (2018). A New Oxadiazole-Based Dual-Mode Chemosensor: Colorimetric Detection of Co2+ and Fluorometric Detection of Cu2+ with High Selectivity and Sensitivity. Microchem. J. 142, 279–287. doi:10.1016/j.microc.2018.07.008
Xie, D.-H., Wang, X.-J., Sun, C., and Han, J. (2016). Calix[4]arene Based 1,3,4-oxadiazole as a Fluorescent Chemosensor for Copper(II) Ion Detection. Tetrahedron Lett. 57, 5834–5836. doi:10.1016/j.tetlet.2016.11.051
Keywords: calix[4]crown, 1,3-alternate conformation, 1,3,4-oxadiazole, copper (II) detection, fluorescent chemosensor
Citation: Sun C, Du S, Zhang T and Han J (2021) A Novel Calix[4]Crown-Based 1,3,4-Oxadiazole as a Fluorescent Chemosensor for Copper(II) Ion Detection. Front. Chem. 9:766442. doi: 10.3389/fchem.2021.766442
Received: 29 August 2021; Accepted: 18 October 2021;
Published: 12 November 2021.
Edited by:
Tony D. James, University of Bath, United KingdomReviewed by:
Xinhua Cao, Xinyang Normal University, ChinaPeter Cragg, University of Brighton, United Kingdom
Copyright © 2021 Sun, Du, Zhang and Han. This is an open-access article distributed under the terms of the Creative Commons Attribution License (CC BY). The use, distribution or reproduction in other forums is permitted, provided the original author(s) and the copyright owner(s) are credited and that the original publication in this journal is cited, in accordance with accepted academic practice. No use, distribution or reproduction is permitted which does not comply with these terms.
*Correspondence: Jie Han, aGFuamllQG5hbmthaS5lZHUuY24=