- 1CNRS UMR 7197, Laboratoire de Réactivité de Surface, LRS, Campus Pierre et Marie Curie, Sorbonne Université, Paris, France
- 2CNRS UMR 8232, Institut Parisien de Chimie Moléculaire, IPCM, Campus Pierre et Marie Curie, Sorbonne Université, Paris, France
Addition of a soluble or a supported CrIII-salophen complex as a co-catalyst greatly enhances the catalytic activity of Bu4NBr for the formation of styrene carbonate from styrene epoxide and CO2. Their combination with a very low co-catalyst:Bu4NBr:styrene oxide molar ratio = 1:2:112 (corresponding to 0.9 mol% of CrIII co-catalyst) led to an almost complete conversion of styrene oxide after 7 h at 80°C under an initial pressure of CO2 of 11 bar and to a selectivity in styrene carbonate of 100%. The covalent heterogenization of the complex was achieved through the formation of an amide bond with a functionalized {NH2}-SBA-15 silica support. In both conditions, the use of these CrIII catalysts allowed excellent conversion of styrene already at 50°C (69 and 47% after 24 h, respectively, in homogeneous and heterogeneous conditions). Comparison with our previous work using other metal cations from the transition metals particularly highlights the preponderant effect of the nature of the metal cation as a co-catalyst in this reaction, that may be linked to its calculated binding energy to the epoxides. Both co-catalysts were successfully reused four times without any appreciable loss of performance.
Introduction
Global warming remains one of mankind’s greatest challenges and, today, it is imperative to find environmentally friendly and cost-effective solutions to limit the increasing concentration of greenhouse gases in the atmosphere. (IPCC. Climate Change, 2014) For this reason, chemistry offers innovative solutions that encourage us to consider CO2 not as a waste but also as a source of high valued compounds. (Aresta et al., 2013). However, its conversion is not an easy task and requires catalysts, thus allowing its utilization as a C1 precursor. Among the various targets considered in the literature, the formation of cyclic carbonates with a rather biodegradability through the reaction of CO2 with epoxides generates actually a growing interest. Indeed, cyclic carbonates have a wide range of applications such as monomers in plastics, solvents in paints, batteries, and even degreasers, or as organic intermediates for the synthesis of dimethylcarbonate (Kamphuis et al., 2019). Furthermore the so-called cyclocarbonatation reaction of epoxides represents a green alternative to one of the conventional synthesis of carbonates based on the phosgenation of diols used industrially since 1833 (Fukuoka et al., 2003).
It is noteworthy that this particular reaction requires the use of organocatalysts (Guo et al., 2021), generally quaternary ammonium salts, but the addition of metal complexes or salts as well as hydrogen bond donors acting as Lewis acids (co-catalysts) (Kim et al., 2013; Adhikari et al., 2014; Supasitmongkol and Styring, 2014; Wang et al., 2014; Sharma et al., 2018; Rehman et al., 2021; Zhou et al., 2021), allowing the activation of epoxides, is a strategy often implemented (Darensbourg and Holtcamp, 1996; Laugel et al., 2013; He et al. 2014; Comerford et al., 2015; Claver et al., 2020). Among the various co-catalysts used, transition metal complexes with Schiff-base ligands (salen or salophen) represent a particularly attractive class of compounds due to their ease of synthesis and the large variety of metal centres that can be incorporated within their N2O2 coordination sphere (Yoon and Jacobsen, 2003; Cozzi, 2004; Gupta and Sutar, 2008; Decortes et al., 2010). Their structure allows the introduction of a large range of substituents that can influence the Lewis acidity of the metal centre. Furthermore, it is also possible to use this chemical flexibility for the covalent immobilization of such catalysts onto a support. Excellent reviews have already been published on the subject (Baleizao and Garcia, 2006; Wezenberg and Kleij, 2008; Gupta et al, 2009).
While there are many examples of salen/salophen CO2 cycloaddition catalysts incorporating transition metal ions such as Mn3+ or Zn2+, those with Cr3+ are far fewer of them (Paddock and Nguyen, 2001; Alvaro et al., 2004; North et al., 2015; Castro-Osma et al., 2016).
Indeed, most studies in the literature reported their activity as efficient catalysts for the copolymerisation of oxiranes and CO2 to provide the corresponding polycarbonates (Darensbourg et al., 2006; Darensbourg et al., 2008; Li et al., 2007; Niu et al., 2009; Veronese et al., 2020), a process that was predicted to be more kinetically favorable than the formation of the corresponding cyclic carbonate in the case of chromium salen complexes (Darensbourg et al., 2003).
However, the selective formation of cyclic carbonates in the presence of chromium salophen complexes was first described by Paddock and Nguyen in 2001 (Paddock and Nguyen, 2001). Since, chromium-salophen co-catalysts combined with various Lewis bases such as DMAP (Adhikari et al., 2014), Bu4NBr (North et al., 2015) or ionic liquids (Alvaro et al., 2004) have allowed the cycloaddition of CO2 under increasingly mild conditions, some working at room temperature or atmospheric pressure. As such, North and co-workers (North et al., 2015) reported a 89% yield of the carbonate of the 1,2-epoxy-3-phenoxypropane at room temperature and under 1 bar of CO2 within 24 h using a homogeneous dual catalysts system based on Bu4NCl and CrIII Jacobsen-type salen complex in solvent-free conditions. However, in this case, the authors used 2.5% of CrIII co-catalyst, which is a particularly large amount for a metal catalyst, especially in the case of a metal such as chromium.
In order to have an easy recovery by filtration, supported versions of those complexes are highly desirable. In this respect, different supports allowing the heterogenization of salen/salophen complexes were studied in the literature, among these are zeolites or mesoporous silica (Srivastava et al., 2003; Ayala et al., 2004; Kureshy et al., 2006; Silva et al., 2006; Kleij, 2009; Melendez et al, 2011). However even though immobilisation of chromium salen/salophen was already achieved with coordinative and covalent bonding for several catalytic applications (Canali and Sherrington, 1999; Gigante et al., 2000; Baleizão et al., 2002; Wang et al., 2007), only a few studies mention their use for the cycloaddition of CO2 onto epoxides, highlighting in this particular case the relevance of covalent anchoring (including the use of MOF) for better recyclability (Ramin et al., 2005; Zalomaeva et al., 2013; Xie et al., 2014). The present work focuses on the co-catalytic performances in the CO2 cycloaddition reaction onto styrene oxide of a CrIII salophen complex bearing a carboxylic acid group, either in homogeneous conditions or after its covalent grafting onto propylamine-functionalized mesoporous silica of the SBA-15 type.
Materials and Methods
The synthesis and the characterization of the ligand and of the Salophen-tBu-Cr complex can be obtained in the Supplementary Material, as well as the protocols for the catalysis tests in homogeneous and heterogeneous conditions and all the details on the different characterization techniques for the complexes and materials are reported.
The SBA-15 functionalized by 2.3 mmol of {NH2}.g−1 (TGA, c.a. 77% incorporation yield) ({NH2}-SBA-15) was obtained by the reaction of SBA-15 with (3-aminopropyl) triethoxysilane (APTES). After anchoring the chromium (III) complex, the material recovered was characterized by XRD, TGA (metal concentration of 0.8 mmol g−1 from Supplementary Figure S6) and N2 physisorption. Overall, a significant decrease of the specific surface area was observed after grafting as expected (see Supplementary Table S1 and Supplementary Figure S7) (from 900 m2 g−1 for SBA-15 to 530 for {NH2}-SBA-15, then 310 m2 g−1 for {Salophen-tBu-Cr}-SBA-15). This was accompanied by a concomitant decrease in pores volume (from 0.69 for {NH2}-SBA-15 to 0.44 cm3 g−1 for {Salophen-tBu-Cr}-SBA-15) and mean pores size values (from 5.7 nm for {NH2}-SBA-15 to 5.2 for {Salophen-tBu-Cr}-SBA-15). These data are compatible with those observed in the case of manganese and nickel complexes grafted on silica SBA-15 described elsewhere (Balas et al., 2021). Furthermore, {Salophen-tBu-Cr}-SBA-15 was characterized by a high hexagonal structuration of its porosity (presence of the (100), (110), and (200) reticular planes characteristic of the SBA-15 structure, see Supplementary Figure S8). It is noteworthy that the successive grafting of the propylamine groups, then of the salophen complex onto SBA-15 did not significantly alter the support.
Results and Discussions
Synthesis and Characterization of the Co-Catalysts
In the continuity of our previous work (Balas et al., 2021), the preparation, the characterization, and the immobilization of a Cr3+-salophen complex bearing a –CO2H function (N,N’-bis(3,5-di-tert-butylsalicylidene)-1-carboxy-3,4-phenylene-diamine-chromium (III)chloride) onto a mesoporous SBA-15 silica through amide bonding was thus addressed in the present manuscript. This compound showed excellent co-catalytic performances in the cycloaddition reaction of CO2 onto styrene oxide, both in its soluble form or after heterogenization.
The ligand (Salophen-tBu, Scheme 1), i.e., N,N’-bis(3,5-di-tert-butylsalicylidene)-1-carboxy-3,4-phenylene-diamine was synthesized according to the experimental protocol developed by Hey-Hawkins and coworkers (Schley et al., 2010; see also Supplementary Figures S1, S3 for more details as well as operating protocols in the supplementary information section). Then, the chromium derivative, (Salophen-tBu-Cr), i.e., N,N’-bis(3,5-di-tert-butylsalicylidene)-1-carboxy-3,4-phenylene-diamine-chromium(III)chloride was easily prepared from the reaction of Salophen-tBu in distilled tetrahydrofuran (thf for short) with commercial [CrCl3(thf)3] under argon, leading to a 94% yield (see Supplementary Figures S4, S5 for the full characterization of the compound).
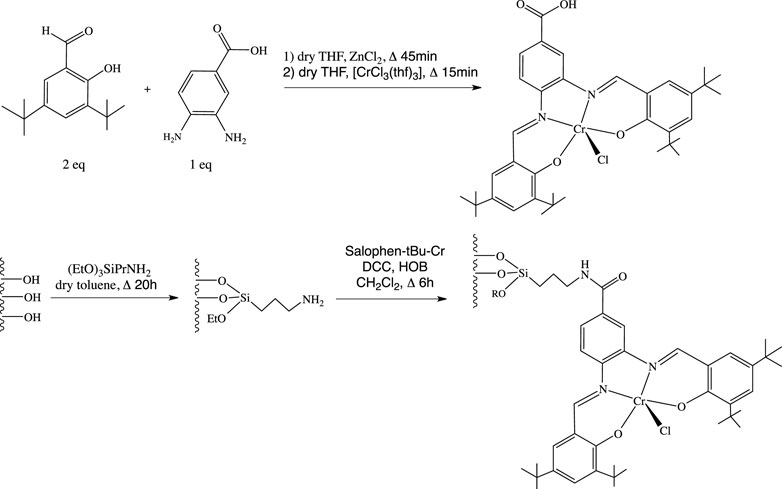
SCHEME 1. Synthesis steps of the co-catalyst and of its covalent grafting onto the surface of an amino functionalized silica support.
The presence of a –COOH function on the central phenyl group of the salophen ligand allowed to covalently graft the complex at the surface of an amino functionalized SBA-15 silica ({NH2}-SBA-15), according to our previous works on the anchoring of hybrid polyoxometalates (Villanneau et al.,2013; Bentaleb et al., 2015) and as shown also for the Mn and Ni derivatives (Balas et al., 2021). This occurred through the formation of a covalent amide bond using N,N’-dicyclohexylcarbodiimide (DCC) and 1-hydroxy-1H-benzotriazole (HOBT) coupling agents, in dichloromethane under refluxing conditions, affording Salophen-tBu-Cr@{NH2}-SBA-15. According to the comparison of the TGA profiles of {NH2}-SBA-15 and Salophen-tBu-Cr@{NH2}-SBA-15, the Cr loading could be estimated to 0.8 wt%.
Catalytic Performances of Salophen-tBu-Cr and Salophen-tBu-Cr@{NH2}-SBA-15 in the Cycloaddition Reaction of Styrene Oxide With CO2
The performances of the soluble and heterogenized forms of the chromium complex to act as co-catalysts were compared in the formation of styrene carbonate from styrene oxide and CO2 using tetra-butyl ammonium bromide as a catalyst (Scheme 2). The experiments were performed in an autoclave under 11 bar of CO2 (initial pressure) with temperatures as low as 50–80°C. The reaction products were quantified by Gas Chromatography and 1H NMR spectroscopy after 3, 7, and 23 h. In all experiments, styrene carbonate was found as the only reaction product and no polymeric materials could be detected. Consequently, the yield of styrene carbonate was equal to the styrene oxide conversion in this work.
The process was initially implemented using soluble tetrabutylammonium bromide (Bu4NBr) and Salophen-tBu-Cr, respectively, as the main catalyst and the Lewis acid co-catalyst, either in benzonitrile or acetonitrile and in the presence p-xylene (internal standard). Regarding our previous results and the corresponding literature, Bu4NBr was shown to have better catalytic performances for this particular reaction compared to other tetraalkylammonium salts bearing shorter chain lengths. Thus, at the highest reaction temperature tested (80°C), Bu4NBr alone (using a styrene oxide:Bu4NBr ratio = 112:2, corresponding to 1.8 mol%) led to a 12% yield of styrene carbonate after 3 h, the reaction being complete after 23 h (Table 1, entry 1). At the same time, Salophen-tBu-Cr (using a styrene oxide:co-catalyst ratio = 112:1, corresponding to 0.9 mol%) showed no activity for this specific reaction in the absence of NBu4Br at 80°C (Table 1, entry 2).
However, using Bu4NBr and Salophen-tBu-Cr together at once (styrene oxide:co-catalyst:Bu4NBr ratio = 112:1:2) led to a dramatic increase of the styrene oxide conversion at the early stages of the reaction with a 75% yield of styrene carbonate within 3 h at 80°C with 100% selectivity (Table 1, entry 3). At this temperature the reaction was almost complete (92%) after 7 h, while a conversion of 20% was observed with Bu4NBr alone.
Furthermore, the presence of the co-catalyst also allowed to obtain an important added value at lower temperature. An increase of the styrene oxide conversion at 60°C was thus observed after 23 h from 24% in the absence of Salophen-tBu-Cr to 96% in its presence (Table 1, entries 4 and 5). Finally, the styrene oxide conversion reached 69% after 23 h at 50°C (Table 1, entries 6 and 7) with both Salophen-tBu-Cr and Bu4NBr (11% in the absence of Salophen-tBu-Cr).
From this study, it appeared that the chromium (III) salophen complex behaved clearly as a better homogeneous co-catalyst compared to the corresponding manganese (III) and nickel (II) complexes (Balas et al., 2021). Indeed a higher yield of styrene carbonate was observed at 80°C for Salophen-tBu-Cr (92% after 7 h) compared to Salophen-tBu-Mn and Salophen-tBu-Ni (respectively, 48% for Mn and 26% for Ni) during the same reaction time (Table 1, entries 8 and 9). It is noteworthy that no styrene oxide conversion was observed with these two last co-catalysts at 50°C. This should be seen in conjunction with the DFT calculations performed by Deng and Lu’s team, which concern the interactions between different metal-salophen complex monomers and propylene oxide (Wu et al., 2017). Of all the metals tested, Cr(III) was indeed found to have the highest binding energy with propylene oxide (15.4 kcal mol−1), higher than those of Mn(III) and Ni(II), although not higher than the desorption energy of the products. It is also in accordance with recent studies from the group of North in which they showed that using CrIII salophen co-catalysts allowed the experimental conditions to be lowered to room temperature under specific conditions (use of cardice pellets, increase of the catalyst and co-catalyst mol% up to 2.5 mol% together) (Castro-Osma et al., 2016).
In parallel, the reactivity of the anchored co-catalyst Salophen-tBu-Cr@{NH2}-SBA-15 was also investigated at 50, 60, and 80°C (Table 2, entries 1, 2 and 3). For this, the set of parameters, and in particular the substrate:catalyst:co-catalyst molar ratio, was kept identical to that fixed in the study in homogeneous conditions. Furthermore, it is worth noting that Bu4NBr was also used as a homogeneous catalyst in that study.
The impact of the heterogenization of the Salophen-tBu-Cr catalyst resulted in a reasonable decrease (from 10 to 20%) in the catalytic activity from the early stage of the reaction. However, in spite of this, the reaction was complete after 23 h at 80°C and the immobilized catalyst was still active even at the lowest temperature (47% styrene oxide conversion after 23 h at 50°C). It should be remembered that such decrease in reactivity between homogeneous and supported catalysts was not observed in the case of the Salophen-tBu-Mn and Salophen-tBu-Ni complexes. On the contrary, reactions conducted in the presence of the Salophen-tBu-Mn@{NH2}-SBA-15 and Salophen-tBu-Ni@{NH2}-SBA-15 supported catalysts had shown better results compared to those obtained in a homogeneous phase. In the latter examples, the reason proposed for the increased reactivity was related to the non-innocent character of the free amine functions of the support. Indeed, the investigation of the potential co-catalytic activity of the {NH2}-SBA-15 support itself, keeping Bu4NBr as the main catalyst, had led to a styrene oxide conversion of 86% after 7 h, using the same set of experimental conditions as in the present work. However, the reaction was conducted at 120°C against 80°C in the present study. These results therefore suggest that lowering the reaction temperature from 120°C to 80°C did not allow the amine functions to participate. This is in accordance with the fact that the carbamate obtained by reaction of CO2 and amines are more stable at lower temperature (Gouedard et al., 2012). It is therefore expected that the release of CO2 will be slowed down at 80°C rather than at 120°C.
In general, the relatively small decrease in reactivity observed in heterogeneous catalytic systems is largely compensated by the ease of catalyst recycling compared to homogeneous systems. The stability of the co-catalyst was thus investigated at 80°C in homogeneous conditions with Salophen-tBu-Cr (4 runs of 7 h with styrene oxide and CO2 replenishment without any work-up) and in heterogeneous conditions with Salophen-tBu-Cr@{NH2}-SBA-15 (4 runs of 7 h separated by filtration and drying steps). The detailed operating protocols for both experiments are described in the supplementary information section. Yields of styrene carbonate in the corresponding experiments are presented in Figure 1.
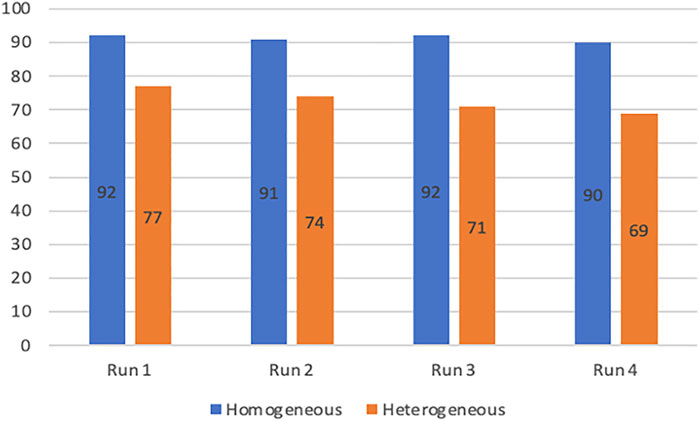
FIGURE 1. Yields of styrene carbonate obtained after four runs in the presence of Salophen-tBu-Cr (in blue) or Salophen-tBu-Cr@{NH2}-SBA-15 (in orange). Conditions: Styrene oxide (5.6 mmol), Bu4NBr (0.1 mmol), soluble or supported Salophen-tBu-Cr (0.05 mmol) in 13 ml of benzonitrile under 11 bar of CO2, 7h, 80°C.
The soluble Salophen-tBu-Cr co-catalyst could be successfully re-used without any appreciable loss of performance after respectively four runs, leading to an excellent styrene oxide conversion (from 92 to 90%) for all runs. A weak, but significant decrease, in the conversion (from 77 to 69%) was observed after each run for the supported Salophen-tBu-Cr@{NH2}-SBA-15. Regarding the results obtained in homogeneous conditions, this relative loss of reactivity may hardly be explained by a degradation of the catalyst, but was probably the consequence of the recurrent loss of 5 wt% of the supported co-catalyst during the work-up procedure leading to its recovery. Taken together, these results suggest an excellent stability in homogeneous and heterogeneous conditions for Salophen-tBu-Cr, and more generally for this family of complex even in the harsher conditions.
Reactions were also conducted for 7 h at 80°C in acetonitrile in homogeneous (Table 1, entry 10) and heterogeneous conditions (Table 2, entry 4), which is a more desirable solvent than benzonitrile (Byrne et al., 2016). It appears from these experiments, that, taking into account our experimental errors, the yields of styrene carbonate were very close in both solvents (92% with MeCN vs. 91% with PhCN in homogeneous conditions; 78% with MeCN vs. 77% with PhCN in heterogeneous conditions), which means that these solvents have no impact on the reactivity of the catalyst.
Conclusion
In conclusion, the present work addressed the successful heterogenization of a chromium (III)-salophen complex at the surface of a mesoporous SBA-15 silica as well as its co-catalytic activity in the cycloaddition of CO2 onto styrene oxide. The synthesis of the molecular catalyst as well as its strong covalent grafting onto the support were based on simple and efficient experimental protocols. The cycloaddition reaction was investigated in the presence of soluble Bu4NBr using the Cr(III) co-catalyst either in solution (homogeneous conditions) or after its immobilization onto the SBA-15 support. In both cases, the presence of the Cr(III)-salophen complex allowed to obtain a dramatic increase of the styrene oxide conversion with respect to the reaction using Bu4NBr as the only catalyst, whatever the temperature used. Remarkable yields of styrene carbonate were thus obtained with a nearly complete conversion of styrene oxide after 7 h at 80°C or 23 h at 60°C in solution. This selective catalyst (no other reaction products were detected) also proved to work even at 50°C. Despite a reasonable reactivity decrease after grafting at the surface of the SBA-15, the immobilized catalyst proved to be easily reused after simple filtration and drying steps, without any observable degradation after several runs. From a more general point of view, the use of a CrIII catalyst seems to make it possible to significantly reduce the metal content in this reaction while allowing the process to operate at the lowest temperatures.
Data Availability Statement
The original contributions presented in the study are included in the article/Supplementary Material, further inquiries can be directed to the corresponding authors.
Author Contributions
FL and RV contributed to conception and design of the study. MB and LK performed the experimental work. RV wrote the first draft of the article. RV, FL, and MB wrote sections of the article. All authors contributed to article revision, read, and approved the submitted version.
Fundings
This study benefited from the support of the project OxCyCat-CO2 ANR-17-CE06-0009 of the French Agence Nationale de la Recherche (ANR) for a PhD fellowship to MB, for financial reward for LK and more generally for funding this work.
Conflict of Interest
The authors declare that the research was conducted in the absence of any commercial or financial relationships that could be construed as a potential conflict of interest.
Publisher’s Note
All claims expressed in this article are solely those of the authors and do not necessarily represent those of their affiliated organizations, or those of the publisher, the editors and the reviewers. Any product that may be evaluated in this article, or claim that may be made by its manufacturer, is not guaranteed or endorsed by the publisher.
Acknowledgments
The authors want also to acknowledge the Centre National de la Recherche Scientifique (CNRS) and Sorbonne Université.
Dedication
In memory of our deceased colleague Alain Shum Cheong Sing.
Supplementary Material
The Supplementary Material for this article can be found online at: https://www.frontiersin.org/articles/10.3389/fchem.2021.765108/full#supplementary-material
References
Adhikari, D., Nguyen, S. T., and Baik, M.-H. (2014). A Computational Study of the Mechanism of the [(salen)Cr + DMAP]-catalyzed Formation of Cyclic Carbonates from CO2 and Epoxide. Chem. Commun. 50, 2676–2678. doi:10.1039/C3CC48769E
Alvaro, M., Baleizao, C., Das, D., Carbonell, E., and García, H. (2004). CO Fixation Using Recoverable Chromium Salen Catalysts: Use of Ionic Liquids as Cosolvent or High-Surface-Area Silicates as Supports. J. Catal. 228, 254–258. doi:10.1016/j.jcat.2004.08.022
Aresta, M., Dibenedetto, A., and Angelini, A. (2013). The Changing Paradigm in CO2 Utilization. J. CO2 Utilization 3-4, 65–73. doi:10.1016/j.jcou.2013.08.001
Ayala, V., Corma, A., Iglesias, M., Rincón, J. A., and Sánchez, F. (2004). Hybrid Organic-Inorganic Catalysts: a Cooperative Effect between Support, and Palladium and Nickel Salen Complexes on Catalytic Hydrogenation of Imines. J. Catal. 224, 170–177. doi:10.1016/j.jcat.2004.02.017
Balas, M., Beaudoin, S., Proust, A., Launay, F., and Villanneau, R. (2021). Advantages of Covalent Immobilization of Metal‐Salophen on Amino‐Functionalized Mesoporous Silica in Terms of Recycling and Catalytic Activity for CO 2 Cycloaddition onto Epoxides. Eur. J. Inorg. Chem. 2021, 1581–1591. doi:10.1002/ejic.202100150
Baleizão, C., and Garcia, H. (2006). Chiral Salen Complexes: An Overview to Recoverable and Reusable Homogeneous and Heterogeneous Catalysts. Chem. Rev. 106, 3987–4043. doi:10.1021/cr050973n
Baleizão, C., Gigante, B., Sabater, M. J., Garcia, H., and Corma, A. (2002). On the Activity of Chiral Chromium Salen Complexes Covalently Bound to Solid Silicates for the Enantioselective Epoxide Ring Opening. Appl. Catal. A: Gen. 228, 279–288. doi:10.1016/S0926-860X(01)00979-6
Bentaleb, F., Makrygenni, O., Brouri, D., Coelho Diogo, C., Mehdi, A., Proust, A., et al. (2015). Efficiency of Polyoxometalate-Based Mesoporous Hybrids as Covalently Anchored Catalysts. Inorg. Chem. 54, 7607–7616. doi:10.1021/acs.inorgchem.5b01216
Byrne, F. P., Jin, S., Paggiola, G., Petchey, T. H. M., Clark, J. H., Farmer, T. J., et al. (2016). Tools and Techniques for Solvent Selection: green Solvent Selection Guides. Sustain. Chem. Process. 4, 7. doi:10.1186/s40508-016-0051-z
Canali, L., and Sherrington, D. C. (1999). Utilisation of Homogeneous and Supported Chiral Metal(salen) Complexes in Asymmetric Catalysis. Chem. Soc. Rev. 28, 85–93. doi:10.1039/A806483K
Castro-Osma, J. A., Lamb, K. J., and North, M. (2016). Cr(salophen) Complex Catalyzed Cyclic Carbonate Synthesis at Ambient Temperature and Pressure. ACS Catal. 6, 5012–5025. doi:10.1021/acscatal.6b01386
Claver, C., Yeamin, M. B., Reguero, M., and Masdeu-Bultó, A. M. (2020). Recent Advances in the Use of Catalysts Based on Natural Products for the Conversion of CO2into Cyclic Carbonates. Green. Chem. 22, 7665–7706. doi:10.1039/D0GC01870H
Comerford, J. W., Ingram, I. D. V., North, M., and Wu, X. (2015). Sustainable Metal-Based Catalysts for the Synthesis of Cyclic Carbonates Containing Five-Membered Rings. Green. Chem. 17, 1966–1987. doi:10.1039/C4GC01719F
Cozzi, P. G. (2004). Metal-Salen Schiff Base Complexes in Catalysis: Practical Aspects. Chem. Soc. Rev. 33, 410–421. doi:10.1039/B307853C
Darensbourg, D., and Holtcamp, M. W. (1996). Catalysts for the Reactions of Epoxides and Carbon Dioxide. Coord. Chem. Rev. 153, 155–174. doi:10.1016/0010-8545(95)01232-X
Darensbourg, D. J., Ganguly, P., and Choi, W. (2006). Metal Salen Derivatives as Catalysts for the Alternating Copolymerization of Oxetanes and Carbon Dioxide to Afford Polycarbonates. Inorg. Chem. 45, 3831–3833. doi:10.1021/ic052109j
Darensbourg, D. J., Moncada, A. I., Choi, W., and Reibenspies, J. H. (2008). Mechanistic Studies of the Copolymerization Reaction of Oxetane and Carbon Dioxide to Provide Aliphatic Polycarbonates Catalyzed by (Salen)CrX Complexes. J. Am. Chem. Soc. 130, 6523–6533. doi:10.1021/ja800302c
Darensbourg, D. J., Yarbrough, J. C., Ortiz, C., and Fang, C. C. (2003). Comparative Kinetic Studies of the Copolymerization of Cyclohexene Oxide and Propylene Oxide with Carbon Dioxide in the Presence of Chromium Salen Derivatives. In Situ FTIR Measurements of Copolymer vs Cyclic Carbonate Production. J. Am. Chem. Soc. 125, 7586–7591. doi:10.1021/ja034863e
Decortes, A., Castilla, A. M., and Kleij, A. W. (2010). Salen-Complex-Mediated Formation of Cyclic Carbonates by Cycloaddition of CO2 to Epoxides. Angew. Chem. Int. Edition 49, 9822–9837. doi:10.1002/anie.201002087
Fukuoka, S., Kawamura, M., Komiya, K., Tojo, M., Hachiya, H., Hasegawa, K., et al. (2003). A Novel Non-phosgene Polycarbonate Production Process Using By-Product CO2as Starting Material. Green. Chem. 5, 497–507. doi:10.1039/B304963A
Gigante, B., Corma, A., García, H., and Sabater, M. J. (2000). Assessment of the Negative Factors Responsible for the Decrease in the Enantioselectivity for the Ring Opening of Epoxides Catalyzed by Chiral Supported Cr(III)-salen Complexes. Catal. Lett. 68, 113–119. doi:10.1023/A:1019046411998
Gouedard, C., Picq, D., Launay, F., and Carrette, P.-L. (2012). Amine Degradation in CO2 Capture. I. A Review. Int. J. Greenhouse Gas Control. 10, 244–270. doi:10.1016/j.ijggc.2012.06.015
Guo, L., Lamb, K. J., and North, M. (2021). Recent Developments in Organocatalysed Transformations of Epoxides and Carbon Dioxide into Cyclic Carbonates. Green. Chem. 23, 77–118. doi:10.1039/D0GC03465G
Gupta, K. C., Kumar Sutar, A., and Lin, C.-C. (2009). Polymer-supported Schiff Base Complexes in Oxidation Reactions. Coord. Chem. Rev. 253, 1926–1946. doi:10.1016/j.ccr.2009.03.019
Gupta, K. C., and Sutar, A. K. (2008). Catalytic Activities of Schiff Base Transition Metal Complexes. Coord. Chem. Rev. 252, 1420–1450. doi:10.1016/j.ccr.2007.09.005
He, Q., O'Brien, J. W., Kitselman, K. A., Tompkins, L. E., Curtis, G. C. T., and Kerton, F. M. (2014). Synthesis of Cyclic Carbonates from CO2 and Epoxides Using Ionic Liquids and Related Catalysts Including Choline Chloride-Metal Halide Mixtures. Catal. Sci. Technol. 4, 1513–1528. doi:10.1039/C3CY00998J
Intergovernmental Panel on Climate Change (2014). Climate Change 2013 - The Physical Science Basis: Working Group I Contribution to the Fifth Assessment Report of the Intergovernmental Panel on Climate Change. Cambridge, United Kingdom: Cambridge University Press. doi:10.1017/CBO9781107415324
Kamphuis, A. J., Picchioni, F., and Pescarmona, P. P. (2019). CO2-fixation into Cyclic and Polymeric Carbonates: Principles and Applications. Green. Chem. 21, 406–448. doi:10.1039/C8GC03086C
Kim, J., Kim, S.-N., Jang, H.-G., Seo, G., and Ahn, W.-S. (2013). CO2 Cycloaddition of Styrene Oxide over MOF Catalysts. Appl. Catal. A: Gen. 453, 175–180. doi:10.1016/j.apcata.2012.12.018
Kleij, A. W. (2009). Nonsymmetrical Salen Ligands and Their Complexes: Synthesis and Applications. Eur. J. Inorg. Chem. 2009, 193–205. doi:10.1002/ejic.200800936
Kureshy, R., Ahmad, I., Khan, N., Abdi, S., Pathak, K., and Jasra, R. (2006). Chiral Mn(III) Salen Complexes Covalently Bonded on Modified MCM-41 and SBA-15 as Efficient Catalysts for Enantioselective Epoxidation of Nonfunctionalized Alkenes. J. Catal. 238, 134–141. doi:10.1016/j.jcat.2005.11.042
Laugel, G., Rocha, C. C., Massiani, P., Onfroy, T., and Launay, F. (2013). Homogeneous and Heterogeneous Catalysis for the Synthesis of Cyclic and Polymeric Carbonates from CO2 and Epoxides: A Mechanistic Overview. Adv. Chem. Lett. 1, 195–214. doi:10.1166/acl.2013.1036
Li, B., Zhang, R., and Lu, X.-B. (2007). Stereochemistry Control of the Alternating Copolymerization of CO2 and Propylene Oxide Catalyzed by SalenCrX Complexes. Macromolecules 40, 2303–2307. doi:10.1021/ma062735f
Meléndez, J., North, M., Villuendas, P., and Young, C. (2011). One-component Bimetallic Aluminium(salen)-Based Catalysts for Cyclic Carbonate Synthesis and Their Immobilization. Dalton Trans. 40, 3885–3902. doi:10.1039/C0DT01196G
Niu, Y., Zhang, W., Li, H., Chen, X., Sun, J., Zhuang, X., et al. (2009). Carbon Dioxide/propylene Oxide Coupling Reaction Catalyzed by Chromium Salen Complexes. Polymer 50, 441–446. doi:10.1016/j.polymer.2008.11.008
North, M., Quek, S. C. Z., Pridmore, N. E., Whitwood, A. C., and Wu, X. (2015). Aluminum(salen) Complexes as Catalysts for the Kinetic Resolution of Terminal Epoxides via CO2 Coupling. ACS Catal. 5, 3398–3402. doi:10.1021/acscatal.5b00235
Paddock, R. L., and Nguyen, S. T. (2001). Chemical CO2 Fixation: Cr(III) Salen Complexes as Highly Efficient Catalysts for the Coupling of CO2 and Epoxides. J. Am. Chem. Soc. 123, 11498–11499. doi:10.1021/ja0164677
Ramin, M., Jutz, F., Grunwaldt, J.-D., and Baiker, A. (2005). Solventless Synthesis of Propylene Carbonate Catalysed by Chromium-Salen Complexes: Bridging Homogeneous and Heterogeneous Catalysis. J. Mol. Catal. A: Chem. 242, 32–39. doi:10.1016/j.molcata.2005.08.004
Rehman, A., Saleem, F., Javed, F., Qutab, H. G., Eze, V. C., and Harvey, A. (2021). Kinetic Study for Styrene Carbonate Synthesis via CO2 Cycloaddition to Styrene Oxide Using Silica-Supported Pyrrolidinopyridinium Iodide Catalyst. J. CO2 Utilization 43, 101379. doi:10.1016/j.jcou.2020.101379
Schley, M., Fritzsche, S., Lönnecke, P., and Hey-Hawkins, E. (2010). Soluble Monometallic Salen Complexes Derived from O-Functionalised Diamines as Metalloligands for the Synthesis of Heterobimetallic Complexes. Dalton Trans. 39, 4090–4106. doi:10.1039/B920706F
Sharma, R. K., Gaur, R., Yadav, M., Goswami, A., Zbořil, R., and Gawande, M. B. (2018). An Efficient Copper-Based Magnetic Nanocatalyst for the Fixation of Carbon Dioxide at Atmospheric Pressure. Sci. Rep. 8, 1901. doi:10.1038/s41598-018-19551-3
Silva, M., Freire, C., de Castro, B., and Figueiredo, J. L. (2006). Styrene Oxidation by Manganese Schiff Base Complexes in Zeolite Structures. J. Mol. Catal. A: Chem. 258, 327–333. doi:10.1016/j.molcata.2006.05.070
Srivastava, R., Srinivas, D., and Ratnasamy, P. (2003). Synthesis of Cyclic Carbonates from Olefins and CO2 over Zeolite-Based Catalysts. Catal. Lett. 89, 81–85. doi:10.1023/A:1024723627087
Supasitmongkol, S., and Styring, P. (2014). A Single centre Aluminium(III) Catalyst and TBAB as an Ionic Organo-Catalyst for the Homogeneous Catalytic Synthesis of Styrene Carbonate. Catal. Sci. Technol. 4, 1622–1630. doi:10.1039/C3CY01015E
Veronese, L., Brivio, M., Biagini, P., Po, R., Tritto, I., Losio, S., et al. (2020). Effect of Quaternary Phosphonium Salts as Cocatalysts on Epoxide/CO2 Copolymerization Catalyzed by Salen-type Cr(III) Complexes. Organometallics 39, 2653–2664. doi:10.1021/acs.organomet.0c00269
Villanneau, R., Marzouk, A., Wang, Y., Djamaa, A. B., Laugel, G., Proust, A., et al. (2013). Covalent Grafting of Organic-Inorganic Polyoxometalates Hybrids onto Mesoporous SBA-15: A Key Step for New Anchored Homogeneous Catalysts. Inorg. Chem. 52, 2958–2965. doi:10.1021/ic302374v
Wang, T.-T., Xie, Y., and Deng, W.-Q. (2014). Reaction Mechanism of Epoxide Cycloaddition to CO2 Catalyzed by Salen-M (M = Co, Al, Zn). J. Phys. Chem. A. 118, 9239–9243. doi:10.1021/jp506124h
Wang, X., Wu, G., Li, J., Zhao, N., Wei, W., and Sun, Y. (2007). Surface-modified Improvement in Catalytic Performance of Cr(salen) Complexes Immobilized on MCM-41 in Solvent-free Selective Oxidation of Benzyl Alcohol. Catal. Lett. 119, 87–94. doi:10.1007/s10562-007-9197-3
Wezenberg, S. J., and Kleij, A. W. (2008). Material Applications for Salen Frameworks. Angew. Chem. Int. Ed. 47, 2354–2364. doi:10.1002/anie.200702468
Wu, T., Wang, T., Sun, L., Deng, K., Deng, W., and Lu, R. (2017). A DFT Exploration of Efficient Catalysts Based on Metal-Salen Monomers for the Cycloaddition Reaction of CO2 to Propylene Oxide. ChemistrySelect 2, 4533–4537. doi:10.1002/slct.201700043
Xie, Y., Yang, R.-X., Huang, N.-Y., Luo, H.-J., and Deng, W.-Q. (2014). Efficient Fixation of CO2 at Mild Conditions by a Cr-Conjugated Microporous Polymer. J. Energ. Chem. 23, 22–28. doi:10.1016/S2095-4956(14)60113-3
Yoon, T. P., and Jacobsen, T. P. (2003). Privileged Chiral Catalysts. Science 299, 1691–1693. doi:10.1126/science.1083622
Zalomaeva, O. V., Chibiryaev, A. M., Kovalenko, K. A., Kholdeeva, O. A., Balzhinimaev, B. S., and Fedin, V. P. (2013). Cyclic Carbonates Synthesis from Epoxides and CO2 over Metal-Organic Framework Cr-MIL-101. J. Catal. 298, 179–185. doi:10.1016/j.jcat.2012.11.029
Keywords: heterogeneous catalysis, CO2 utilization, cycloaddition reaction, styrene oxide, chromium salophen complexes
Citation: Balas M, K/Bidi L, Launay F and Villanneau R (2021) Chromium-Salophen as a Soluble or Silica-Supported Co-Catalyst for the Fixation of CO2 Onto Styrene Oxide at Low Temperatures. Front. Chem. 9:765108. doi: 10.3389/fchem.2021.765108
Received: 26 August 2021; Accepted: 30 September 2021;
Published: 29 October 2021.
Edited by:
Florent Allais, AgroParisTech Institut des Sciences et Industries du Vivant et de L’environnement, FranceReviewed by:
Zhuohua Sun, Beijing Forestry University, ChinaEsmail Doustkhah, National Institute for Materials Science, Japan
AidaLuz Villa, University of Antioquia, Colambia
Copyright © 2021 Balas, K/Bidi, Launay and Villanneau. This is an open-access article distributed under the terms of the Creative Commons Attribution License (CC BY). The use, distribution or reproduction in other forums is permitted, provided the original author(s) and the copyright owner(s) are credited and that the original publication in this journal is cited, in accordance with accepted academic practice. No use, distribution or reproduction is permitted which does not comply with these terms.
*Correspondence: Richard Villanneau, cmljaGFyZC52aWxsYW5uZWF1QHNvcmJvbm5lLXVuaXZlcnNpdGUuZnI=; Franck Launay, ZnJhbmNrLmxhdW5heUBzb3Jib25uZS11bml2ZXJzaXRlLmZy