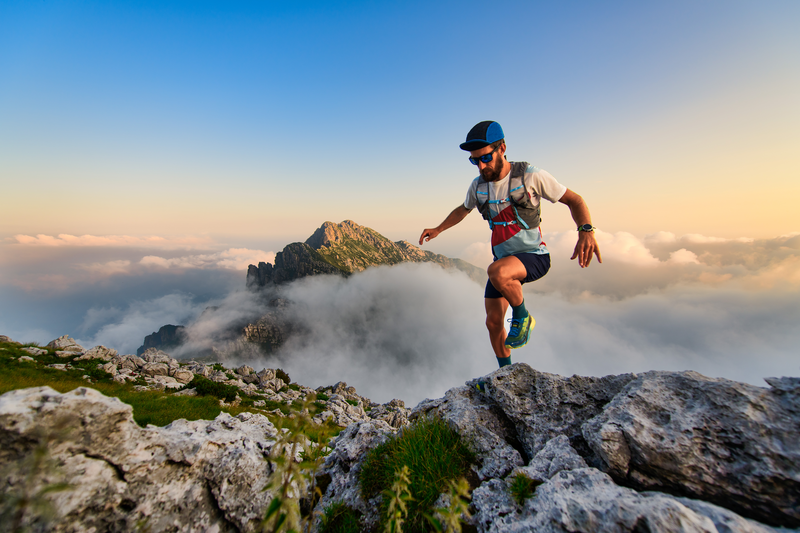
95% of researchers rate our articles as excellent or good
Learn more about the work of our research integrity team to safeguard the quality of each article we publish.
Find out more
MINI REVIEW article
Front. Chem. , 15 November 2021
Sec. Catalysis and Photocatalysis
Volume 9 - 2021 | https://doi.org/10.3389/fchem.2021.749839
This article is part of the Research Topic Photocatalysts for Air Purification: Design, Synthesis, and Mechanism Investigations View all 5 articles
With their outstanding diversities in both structures and performances, newly emerging metal-organic frameworks (MOFs) materials are considered to be the most promising artificial catalysts to meet multiple challenges in the fields of energy and environment. Especially in absorption and conversion of solar energy, a variety of MOFs can be readily designed to cover and harvest the sun irradiation of ultraviolet (UV), visible and near-infrared region through tuning both organic linkers and metal nodes to create optimal photocatalytic efficiency. Nowadays, a variety of MOFs were successfully synthesized as powerful photocatalysts for important redox reactions such as water-splitting, CO2 reduction and aqueous environmental pollutants detoxification. MOFs applications in indoor-air VOCs pollutants cleaning, however, are less concerned partially because of limited diffusion of both gaseous pollutant molecules and photo-induced active species in very porous MOFs structures. In this mini-review, we focus on the major breakthroughs of MOFs as photocatalysts for the effective removal of indoor-air VOCs such as aldehydes, aromatics and short-chain alcohols. According to their nature of photoactive centers, herein MOFs photocatalysts are divided into two categories to comment, that is, MOFs with variable valence metal nodes as direct photoactive centers and MOFs with non-variable valence metal nodes but after combining other photoactive variable valence metal centers as excellent concentrated and concerted electron-transfer materials. The mechanisms and current challenges of the photocatalytic degradation of indoor-air VOC pollutants by these MOFs will be discussed as deeply as possible.
With the increasing economic and industrial developments, more and more anthropogenic gaseous pollutants such as NOx, SO2, VOCs (volatile organic compounds), and PMs (particulate matters) are being emitted from exhaust gases of automobiles, power plants, steel mills, burning of straw and various other industrial sources (Poschl and Shiraiwa, 2015). These substances have been evidenced to lead to multiple threats to human health (Bari and Kindzierski, 2018), particularly in the confined space such as cabins, offices, hospitals and submarines. Among the various gaseous pollutants, VOCs are considered to be one of the most common and notorious pollutants of indoor air. VOCs are defined as those organic compounds with the normal boiling point between room temperature to 260°C. The common VOCs in indoor-air mainly include aromatics (benzene, toluene, xylene, mesitylene and styrene), short-chain alcohols (methanol, ethanol and isopropanol), short-chain carbonyl compounds (formaldehyde, acetaldehyde and acetones) and low molecular-weight aliphatic hydrocarbons (n-hexane, cyclohexane, and isoprene). (Li and Gaillard, 2009). Due to its high mutagenic and carcinogenic toxicity, VOCs in indoor-air are extremely hazardous to human health (Chen et al., 2009; Huang et al., 2014a). Now, more and more strict laws and regulations with lower limit of these indoor-air pollutants are issuing (Liotta, 2010).
To meet the demands of these pacts, various methods such as adsorption (Glomb et al., 2017), filtering (Koo et al., 2018), catalytic oxidation (Huang et al., 2015; Chen et al., 2020) and photocatalytic oxidation (Li et al., 2019) are emerging. Among these methods, photocatalysis is deemed to be one of the most promising technologies because it adopts the clean solar light (or indoor light) to drive the chemical degradation reactions. Since Fujishima and Honda reported that TiO2 can catalyze the water-splitting to generate H2 and O2 upon UV light irradiation (Fujishima and Honda, 1972), novel materials possessing photocatalytic activity are appearing at an exponential speed, especially in the past 2 decades (Ma et al., 2018; Ma et al., 2019; Li et al., 2020a). Especially, Yaghi’s group discovered the first metal-organic framework MOF-5, which is typically porous crystalline material and constructed from Zn2+ and H2BDC (1,4-benzenedicarboxylate) (Li et al., 1999). Garcia et al. thereafter evidenced its photocatalytic activity by the degradation of dye in water (Alvaro et al., 2007). From then on, a large number of MOFs-based photocatalysts have been prepared for the purpose of environmental pollutants degradation (Wang et al., 2014; Tian et al., 2018; Chen et al., 2019; Cheng and Zhang, 2020; Wang et al., 2020a; Han et al., 2021). However, compared with most researches focusing on the degradation of aqueous environment pollutants, less concentration has been paid to the gaseous pollutants degradation and elimination (Wen et al., 2019), especially indoor-air pollutants decontamination with MOFs photocatalysts. One of the main reasons is the restricted lighting-area and difficult diffusion of trace VOCs as well as their degradation products in the inconvenient packed gaseous-solid reactors, which makes few photocatalysts including most MOFs for such tough burdens. Nevertheless, some MOFs photocatalytic applications for indoor-air quality control have garnered considerable success albeit mostly in the lab scale. These achievements may shed light on the key molecular basis of MOFs photocatalysts for practicable degradation of indoor-air pollutants (Li et al., 2019).
In this mini-review, we will focus on two kinds of MOFs photocatalysts for typical indoor air VOCs treatments according to whether the metal nodes in MOFs are or not photoactive centers. For the former, since MOFs materials bear variable valence metal ion such as Ti4+/3+, Fe3+/2+, Cu2+/1+ as nodes, they act as photo-induced redox sites by themselves directly to initiate indoor VOCs degradation. For the latter, because MOFs are made of non-variable valence metal such as Zr4+, Zn2+ ion as nodes, they must combine other photoactive centers in which MOFs themselves act as excellent concentrated and concerted ET role to enhance photo-degradation. Some paragon examples of these two kinds of MOFs photocatalysts applied in decomposition of representative indoor air VOCs will be discussed in detail. The motivation of this mini-review is based on MOFs photocatalytic materials recently emerging of the outstanding diversities in both porous structures and performances that may just meet two challenges, namely, the restricted lighting-area and difficult diffusion of trace VOCs in the elimination of indoor air pollutants. To demonstrate and comment on photocatalytic removal of indoor air pollutants in terms of metal node redox properties of MOFs is the main innovation of this review.
Differing from the mature photocatalytic degradation of organic pollutants in water, the removal of indoor VOCs pollutants has its own characteristics. 1) Due to the gas-solid interface reaction traits, the highly effective oxidative degradation nearly for any organics by highly active •OH radicals (generated via valence band holes, hvb+ + H2O → •OH process) in traditional photocatalytic degradation of aqueous organic pollutants is invalid. Only the direct hvb+ oxidative degradation of VOCs is left behind (i.e., hvb+ + VOCs → VOCs+•). At this time, gaseous O2 will play pivotal roles: one is to rapidly capture, remove the conduction-band electron (ecb−) so as to efficiently generate more hvb+ to oxidize VOCs; another is directly adding to the VOCs• intermediates (VOCs• + O2 → VOCs-•OO), or the secondary products with ecb− including O2−•, •OOH, H2O2 by ET or the singlet 1O2 via energy transfer, which will diffuse in the pores and react with VOCs pollutants. The hvb+ cannot leave the surface to react with VOCs via indirect solvent-relay route, while O2 and its secondary active species can diffuse and migrate in MOFs channels. Thus, the design of MOFs structure will mainly focus on the O2 diffusion channels and favorable reaction sites (see Figure 1) (Li et al., 2019). 2) From the practical applications in indoor air cleaning, the as-used gas/solid photocatalysts hardly accommodate highly light-harvesting area/volume ratio whether photocatalysts are in membrane or powder forms. The optimum strategy to increase the efficiency of the surface photo-reaction is to utilize the convection and diffusion effect to concentrate and confine the VOCs in the outer-surface and internal pores of the whole catalyst. Compared with the previous common inorganic oxides and organic photocatalysts, MOFs possess large advantages on this occasion. Their abundant pores, large specific surfaces area (500–7,000 m2/g) can assist to pre-concentrate and store a large amount of VOCs. Once upon sunlight irradiation, the enriched VOCs can conveniently diffuse from the internal pores to the surface to be photo-degraded (see Figure 1A) (Saouma et al., 2018). 3) Combined the above-mentioned double properties of photo-degradation of indoor-air VOCs, the feasible MOFs photocatalysts are mainly divided into two categories. One is that the variable valence metal ions construct the MOF’s node, such as common Ti4+, Fe3+, Cu2+. They can convert to the low valence Ti4+/3+, Fe3+/2+, Cu2+/1+ upon irradiation by LMCT/MLCT mechanism, which creates the hvb+/ecb− active center to react with VOCs and O2, respectively. Another kind of MOFs photocatalysts is using non-redox-active metal such as Zr4+, Zn2+ as the nodes and hybridizing with other photoactive materials (Ma et al., 2019). For these composite MOFs, the photoactive centers can be inorganic metal-oxides such as TiO2, ZnO, noble-metal nanoparticles and organometallic complexes. On this basis, MOFs mainly contribute to the large specific surface areas and abundant pore structures, which can pre-concentrate the indoor-VOCs and utilize its highly-ordered stacking π electrons to boost the composites photoactive center charge-separation efficiency (see Figure 1B). The critical point of this kind MOFs is the high accordance of the hybridizing interfaces and channels to the corresponding indoor VOCs. In the following part, we will divide these two categories of MOFs materials further into the most common Ti-based MOFs materials and other MOFs photocatalyst and, introduce their applications in the indoor-air VOCs photo-degradation.
FIGURE 1. Schematic representation of MOFs as direct photoactive center such as Mil-125-Ti (A) or as base material to combine other photoactive component such as TiO2/UiO-66-Zr (B) for degradation of indoor-air VOCs.
Due to the TiO6 octahedral coordination and Ti4+/Ti3+ redox similar to the star heterogeneous photocatalyst TiO2, Ti-based MOFs are investigated in depth for the purpose of the semiconductor photocatalysts for VOCs removal. By a microwave heating procedure, NH2-MIL-125 (Ti) was hybridized with graphene oxide (GO) to form a heterojunction photocatalyst (Li et al., 2018b). As-prepared 10-GO/NH2-MIL-125(Ti) sample exhibits much enhanced photo-degradation activity of gaseous acetaldehyde under visible-light irradiation (λ > 420 nm) in comparison with mechanically mixed two components and unmodified NH2-MIL-125(Ti). The authors attributed the superior photocatalytic performance of the hybridized photocatalyst to the enhanced visible-light absorbance, more electron carrier density, more efficient charge carrier transfer and less electron-hole recombination. This example indicates that the formation of heterojunction can considerably improve the MOFs photocatalyst performance in VOCs remediation.
Zhao et al. developed a N/Zn co-decoration strategy to strengthen the photocatalytic activity of MIL-125(Ti) towards indoor-air acetaldehyde oxidative degradation (Gao et al., 2020). N and Zn dopants were introduced by a simple solvothermal process. N/Zn co-doped MIL-125(Ti) exhibits 10.5 and 4.4 times higher removal rate of gaseous acetaldehyde under visible-light irradiation in comparison with Zn-doped MIL-125(Ti) and pristine MIL-125(Ti), even surpasses the state-of-the-art metal-oxides and noble-metal-based photocatalysts. DFT theoretical simulations evidenced the N/Zn co-doping tactic evolves the inter-gap band, which plays dual role either as shallow trap for charge carrier to enhance separation efficiency or narrowing the gap to increase visible-light absorptivity. This report shows that via the co-doping strategy to modify the surface acidity, polarity and the electronic structure, MOFs photocatalyst can achieve higher activity and humid environment resistance for VOCs removal.
The same group discovered that by an ionic-liquid assisted synthetic procedure, defect-rich polyaniline (PANI)/NH2-MIL-125(Ti) nanohybrid photocatalyst can realize ultra-rapid degradation of acetaldehyde even under high humidity (Shah et al., 2021). [EMIM]BF4 ionic-liquid was exploited to dissolve the PANI to oligomers and graft the in-situ formed PANI oligomers to NH2-MIL-125(Ti). The smart-grafted IL-PANI/NH2-MIL-125(Ti) exhibits strong interfacial π-π conjugation and van der Waals interactions between NH2-MIL-125(Ti) and PANI oligomer. Besides, the IL-treated PANI/interfacial displays more defects such as ligand vacancies and Ti3+ sites thereby created much enhanced Lewis acidity, which is critically important for effective adsorption of acetaldehyde with Lewis basicity (O lone pair). Interestingly, water molecules can enhance the performance of this photocatalyst. From the electron paramagnetic resonance (EPR) trapping experiments, H2O participates in ROS generation by reacting with O2−• to yield more active hydroxyl radicals to oxidize acetaldehyde. The IL-treated PANI-Ti-MOFs exhibited much increased affinity to acetaldehyde and acetic acid in comparison with CO2, which improves the retention of the intermediate acetic acid in the photocatalyst reactive site and the desorption of the final innocuous CO2 product.
Apart from MIL-125(Ti)-based MOFs materials, their Zr-MOF counterparts exhibit much enhanced stability towards humidity and long-term light-irradiation. Thus, it is also highly desirable to apply Zr-MOFs for photocatalytic VOCs degradation especially in highly humid environment. To just take advantage of the hydrolytic stability, if proper semiconductor or metal nanoparticles as photoactive centers were hybridized with Zr-MOFs, their photocatalytic performances can be considerably improved. By an in-situ solvothermal synthesis of NH2-UiO-66 (Zr) in the presence of pre-formed TiO2 nanoparticles, a TiO2/NH2-UiO-66 (Zr) composite photocatalyst was successfully prepared. (Yao et al., 2018). Ultra-small TiO2 nanoparticles were encapsulated inside the interior of Zr-MOF. The composite photocatalyst demonstrates much superior performance of the photocatalytic oxidation of styrene in comparison with TiO2 and pristine NH2-UiO-66 (Zr). The authors proposed that due to the affinity of styrene with Zr-MOF’s linker 2-aminoterephthalate by strong π-π interaction, the VOC (styrene) could be concentrated in the NH2-UiO-66 interior pores. The encapsulated TiO2 photoactive sites can contact more conveniently and react with the neighboring styrene inside the porous structure in comparison with styrene in the outer surface. Besides the easier diffusion and reaction of VOCs substrate, the photo-generated electrons and holes could be transferred and separated between NH2-UiO-66 (Zr) and encapsulated TiO2. Namely, the elaborate conjugation of semiconductors with stable Zr-MOFs significantly improves its photocatalytic activity for indoor VOCs degradation through concerted strong interfacial effects.
Besides common static VOCs photocatalytic degradation, continuous flow reactor system provides more potential for practical indoor-air decontamination. Hu et al. utilized an evaporation method generating TiO2-UiO-66-NH2 composite photocatalyst for gaseous toluene and acetaldehyde removal. (Zhang et al., 2020a). The large specific surface area of Zr-MOF considerably boosted photocatalytic performance of TiO2 under UV irradiation by providing better dispersion media for TiO2 nanoparticles. As-synthesized composite photocatalyst demonstrates exciting performance for toluene and acetaldehyde degradation with satisfactory conversion rate and CO2 selectivity. This demonstrates that smooth removal of indoor air VOCs by Zr-MOF-based hybrid materials can be realized even under very fast continuous flow condition.
Moreover, the core/shell NH2-UiO-66@TiO2 was also applied for the more effective photocatalytic degradation of VOC pollutant toluene. By the in-situ preparation of amorphous TiO2 from the hydrolysis of Ti (t-BuO)4 in the presence of pre-formed UiO-66-NH2, more effective photocatalytic removal of toluene can be achieved comparing with TiO2 and unmodified UiO-66-NH2. (Zhou et al., 2021). Detailed EPR mechanistic investigations were conducted compared with An’s and Hu’s pioneering work using the same type MOFs composite material, demonstrating that O2−• superoxide anion plays a pivotal role during photocatalytic reaction.
To enhance the light-absorption and extend the absorption spectrum to the visible-light and near-infrared region, porphyrin-based organic linkers were introduced to the MOFs structure. After implantation of iron (III) ions into the center of porphyrin units of PCN-224 MOF photocatalyst bonded Zr-oxo cluster with tetra-kis (4-carboxyphenyl)-porphyrin, a novel Fe@PCN-224 photocatalyst was constructed (Shi et al., 2018). Its photocatalytic performance for oxidation of isopropanol (a common VOC pollutant of indoor air) into CO2 was significantly enhanced via Fe-implanted porphyrin-based Zr-MOF. Herein Fe not only improves the photo-generated charge-carrier separation efficiency, but also produces additional Fenton reaction activity to assist less active H2O2 transformation to much stronger •OH by Fe3+/2+ cycles. This report provides an innovative strategy to combine Fenton reactivity with stable Zr-MOFs photocatalyst towards indoor air VOC pollutants such as isopropanol.
Zn-based zeolite imidazole framework ZIF-8 is another kind of highly stable MOFs against hydrolytic and photo-irradiation conditions (Huang et al., 2014b). To exploit its application for ethylene removal under UV irradiation, a bimetallic PtPd alloy encapsulated-ZIF-8 photocatalyst was prepared and exhibited synergistic function in contrast with the pristine ZIF-8 and monometal encapsulated ZIF-8 materials.
Although ZIF-8 photocatalyst can provide excellent performance under UV irradiation, it rarely has visible-light activity. To create its visible-light activity, a post-modification strategy was applied to fine-tune the chemical structure of the ZIF-8 photocatalyst.(Wang et al., 2020b). After heating in the air atmosphere, ZIF-8 was partially oxidized to incorporate an isocyanate group N=C=O, with the concomitant cleavage of N-methyl group of the pristine imidazole unit. The introduced isocyanate moiety not only strongly extends ZIF-8 absorption band-edge from 325 nm (UV) to 715 nm (visible light region), but also introduces more adsorption activity towards formaldehyde and less adsorptivity to CO2 comparing with its original ZIF-8 parent. Both the augmentation of light absorption, transmittance and formaldehyde adsorption result in much enhanced photocatalytic removal efficiency of indoor HCHO. Interestingly, water in this system can accelerate the generation of strongly oxidative H2O2 species thereby enhance the degradation of formaldehyde in moist air, by which further boosts its activity and stability for this porous photocatalyst under humid environment.
In addition, Cu and Fe-based HKUST-1 and NH2-MIL-101(Fe) MOFs also act as efficient photocatalyst for VOCs degradation under irradiation conditions. Cu-based HKUST-1 MOF material merged with anatase TiO2 semiconductor to form a core/shell structure with Cu3(BTC)2 (BTC = 1,3,5-benzenetricarboxylate) as the core and ultra-thin anatase film as a shell (Wang et al., 2016). The as-formed core/shell MOFs improve the efficiency of photo-generated electron-hole separation due to the facilitated ET from CB of TiO2 to HKUST-1 Cu2+ center. Moreover, the Cu-HKUST-1 core structure enhances the adsorption of isopropanol and the toxic intermediate acetone, while the final product CO2 is less adsorbed.
As an earth-abundant and non-toxic element, iron-based materials are considered as promising catalysts for remediation of indoor air. NH2-MIL-101(Fe) photocatalyst was reported to effectively remove the toluene in hermetic space (Zhang et al., 2016). The microspindle Fe-MOF photocatalyst demonstrates excellent toluene degradation efficiency upon visible-light irradiation because of the much intense and broad visible-light absorption spectrum. The NH2-terephthalate organic ligand acts as antenna to harvest visible-light to initiate LCCT (ligand-to-metal-cluster charge-transfer) process. Moreover, the Fe3-μ3-oxo cluster can directly absorb the visible-light and initiate an electron-hole separation event. Photo-induced electron and hole migrate separately to the catalyst surface to react with the adsorbed O2 and H2O molecules, yielding strongly oxidative ROS species such as O2−• and •OH to degrade toluene into CO2 and H2O by consecutive radical addition, hydroxylation, ring-opening and chain-cleavage processes.
Great efforts have been devoted to developing MOF-based materials specifically towards photocatalytic elimination of indoor VOC pollutants in recent years. Every as-prepared MOF photocatalyst example commented in Ti-Based MOFs Photocatalysts for Degradation of Indoor Air VOCs and Other Metal-Based MOFs Photocatalysts for Degradation of Indoor Air VOCs section is now summarized in Table 1. More importantly, the latest preparation and modification strategies of MOFs-based photocatalysts are emerging, mainly for overcoming the inherent disadvantages in degradation of VOC pollutants (Hao et al., 2020; Li et al., 2020b; Zhang et al., 2020b). 1) The organic linkers with huge diversity in MOFs usually serve as antennas for light harvesting. Therefore, a preferred methodology has been attempted to improve the light absorption of MOFs by furnishing aromatic carboxylate ligands with −NH2 to improve its light harvesting (Fu et al., 2012). Indeed, most MOFs photocatalyst examples in Table 1 have -NH2 groups. 2) Location of the Pt and metalloporphyrin cocatalyst in MOF composites can significantly boost electron−hole separation (Xiao et al., 2016). In addition, noble metals loaded in MOFs commonly catalyzed VOCs molecules transformation even under mild conditions. 3) Combination of plasmonic metals and Schottky junction into MOFs can simultaneously improve light harvesting and charge separation (Xiao et al., 2018). Alternatively, integrating upconversion nanoparticles into MOFs photocatalyst is specially adapted to the use of indoor light (Li et al., 2018a). 4) Photothermal effect by both plasmonic metals and MOFs in MOF-based composites, such as PCN-224 (M) and Pt nanocrystals, is able to activate O2 to 1O2 under light irradiation, which ROS species driven by photothermal effect is very effective to selectively react with olefins VOC pollutants (Chen et al., 2017). All of these approaches to preparation and modification of MOFs photocatalysts show that the well-defined and tailorable MOF structures hold great advantages in eventually solving the problem of indoor VOC pollutants (Xiao and Jiang, 2019).
Despite some successful applications of MOFs photocatalysts for the removal of indoor-air VOCs pollutants (Table 1), there still remain various challenges that decisively limit the practical application beyond laboratory level. Firstly, the considerably low space-time yields still restrain the large-scale treatment of real indoor air. Secondly, the mineralization yields are still not satisfactory. The common MOFs-based photocatalyst system often possesses less oxidativity compared with traditional UV-excitable metal oxides photocatalysts such as TiO2. Such poor mineralization results in the accumulation of organic intermediates on the surface of photocatalysts, which usually leads to catalyst deactivation and blocks the catalytic cycle. Although there have been some MOFs-based photocatalysts with excellent stability for moisture under long-term illumination, the weak coordinative bonds still restrain the discoveries of more powerful MOFs-based photocatalysts for VOCs removal. Lastly, up till now, there still lacks MOFs photocatalysts to realize indoor VOCs removal under NIR irradiation due to the low-energy of NIR photon not enough cleaving the high-energy VOCs chemical bonds. This considerably decreases the solar-light-utilization efficiency as NIR possesses about half of the overall energy of sunlight. The doping, co-doping, dye-sensitization can be useful methods to extend absorption spectrum and enhance charge-carrier separation. The utilization of the organic ligands with electron donor-acceptor structure is another potential tactic to enhance the visible-light absorption or even to induce NIR absorptivity. The merging of two-photon absorbing organic moiety may initiate its NIR photocatalytic ability for indoor VOCs degradation (Li et al., 2018a). Although great challenges facing to us in this research area, we believe that the future of MOFs photocatalytic applications for indoor VOCs degradation will be much exciting and awarding.
YQ and DM performed the literature research, organized and wrote the manuscript. DM revised the manuscript. JZ and DM edited the paper.
This work was financially supported by the National Natural Science Foundation of China (Grant Numbers 22076007, 21703005) and Scientific Research Project of Beijing Educational Committee (Grant Number KM202010011005).
The authors declare that the research was conducted in the absence of any commercial or financial relationships that could be construed as a potential conflict of interest.
All claims expressed in this article are solely those of the authors and do not necessarily represent those of their affiliated organizations, or those of the publisher, the editors and the reviewers. Any product that may be evaluated in this article, or claim that may be made by its manufacturer, is not guaranteed or endorsed by the publisher.
Alvaro, M., Carbonell, E., Ferrer, B., Llabrés i Xamena, F. X., and Garcia, H. (2007). Semiconductor Behavior of a Metal-Organic Framework (MOF). Chem. Eur. J. 13, 5106–5112. doi:10.1002/chem.200601003
Bari, M. A., and Kindzierski, W. B. (2018). Ambient Volatile Organic Compounds (VOCs) in Communities of the Athabasca Oil Sands Region: Sources and Screening Health Risk Assessment. Environ. Pollut. 235, 602–614. doi:10.1016/j.envpol.2017.12.065
Chen, H. L., Lee, H. M., Chen, S. H., Chang, M. B., Yu, S. J., and Li, S. N. (2009). Removal of Volatile Organic Compounds by Single-Stage and Two-Stage Plasma Catalysis Systems: A Review of the Performance Enhancement Mechanisms, Current Status, and Suitable Applications. Environ. Sci. Technol. 43, 2216–2227. doi:10.1021/es802679b
Chen, S., Zhou, Y., Li, J., Hu, Z., Dong, F., Hu, Y., et al. (2020). Single-Atom Ru-Implanted Metal-Organic Framework/MnO2 for the Highly Selective Oxidation of NOx by Plasma Activation. ACS Catal. 10, 10185–10196. doi:10.1021/acscatal.0c02001
Chen, X.-H., Wei, Q., Hong, J.-D., Xu, R., and Zhou, T.-H. (2019). Bifunctional Metal-Organic Frameworks toward Photocatalytic CO2 Reduction by post-synthetic Ligand Exchange. Rare Met. 38, 413–419. doi:10.1007/s12598-019-01259-6
Chen, Y.-Z., Wang, Z. U., Wang, H., Lu, J., Yu, S.-H., and Jiang, H.-L. (2017). Singlet Oxygen-Engaged Selective Photo-Oxidation over Pt Nanocrystals/Porphyrinic MOF: The Roles of Photothermal Effect and Pt Electronic State. J. Am. Chem. Soc. 139, 2035–2044. doi:10.1021/jacs.6b12074
Cheng, Q., and Zhang, G.-K. (2020). Enhanced Photocatalytic Performance of Tungsten-Based Photocatalysts for Degradation of Volatile Organic Compounds: a Review. Tungsten 2, 240–250. doi:10.1007/s42864-020-00055-5
Fu, Y., Sun, D., Chen, Y., Huang, R., Ding, Z., Fu, X., et al. (2012). An Amine-Functionalized Titanium Metal-Organic Framework Photocatalyst with Visible-Light-Induced Activity for CO2 Reduction. Angew. Chem. Int. Ed. 51, 3364–3367. doi:10.1002/anie.201108357
Fujishima, A., and Honda, K. (1972). Electrochemical Photolysis of Water at a Semiconductor Electrode. Nature 238, 37–38. doi:10.1038/238037a0
Gao, Z., Wang, J. X., Muhammad, Y., Zhang, Y. B., Shah, S. J., Hu, Y., et al. (2020). Enhanced Moisture-Resistance and Excellent Photocatalytic Performance of Synchronous N/Zn-decorated MIL-125(Ti) for Vaporous Acetaldehyde Degradation. Chem. Eng. J. 388, 1. doi:10.1016/j.cej.2020.124389
Glomb, S., Woschko, D., Makhloufi, G., and Janiak, C. (2017). Metal-Organic Frameworks with Internal Urea-Functionalized Dicarboxylate Linkers for SO2 and NH3 Adsorption. ACS Appl. Mater. Inter. 9, 37419–37434. doi:10.1021/acsami.7b10884
Han, D. L., Yu, P. L., Liu, X. M., Xu, Y. D., and Wu, S. L. (2021). Polydopamine Modified CuS@HKUST for Rapid Sterilization through Enhanced Photothermal Property and Photocatalytic Ability. Rare Metals. doi:10.1007/s12598-021-01786-1
Hao, S., Zhao, X., Cheng, Q., Xing, Y., Ma, W., Wang, X., et al. (2020). A Mini Review of the Preparation and Photocatalytic Properties of Two-Dimensional Materials. Front. Chem. 8, 582146. doi:10.3389/fchem.2020.582146
Huang, H., Xu, Y., Feng, Q., and Leung, D. Y. C. (2015). Low Temperature Catalytic Oxidation of Volatile Organic Compounds: a Review. Catal. Sci. Technol. 5, 2649–2669. doi:10.1039/c4cy01733a
Huang, R.-J., Zhang, Y., Bozzetti, C., Ho, K.-F., Cao, J.-J., Han, Y., et al. (2014a). High Secondary Aerosol Contribution to Particulate Pollution during Haze Events in China. Nature 514, 218–222. doi:10.1038/nature13774
Huang, Y., Zhang, Y., Chen, X., Wu, D., Yi, Z., and Cao, R. (2014b). Bimetallic alloy Nanocrystals Encapsulated in ZIF-8 for Synergistic Catalysis of Ethylene Oxidative Degradation. Chem. Commun. 50, 10115–10117. doi:10.1039/c4cc04479g
Koo, W.-T., Jang, J.-S., Qiao, S., Hwang, W., Jha, G., Penner, R. M., et al. (2018). Hierarchical Metal-Organic Framework-Assembled Membrane Filter for Efficient Removal of Particulate Matter. ACS Appl. Mater. Inter. 10, 19957–19963. doi:10.1021/acsami.8b02986
Li, D., Yu, S. H., and Jiang, H. L. (2018a). From UV to Near-Infrared Light-Responsive Metal-Organic Framework Composites: Plasmon and Upconversion Enhanced Photocatalysis. Adv. Mater. 30, e1707377. doi:10.1002/adma.201707377
Li, H., Eddaoudi, M., O'keeffe, M., and Yaghi, O. M. (1999). Design and Synthesis of an Exceptionally Stable and Highly Porous Metal-Organic Framework. Nature 402, 276–279. doi:10.1038/46248
Li, J., Zhao, D., Liu, J., Liu, A., and Ma, D. (2020a). Covalent Organic Frameworks: A Promising Materials Platform for Photocatalytic CO2 Reductions. Molecules 25, 2425. doi:10.3390/molecules25102425
Li, N., and Gaillard, F. (2009). Catalytic Combustion of Toluene over Electrochemically Promoted Ag Catalyst. Appl. Catal. B: Environ. 88, 152–159. doi:10.1016/j.apcatb.2008.09.010
Li, P., Li, J., Feng, X., Li, J., Hao, Y., Zhang, J., et al. (2019). Metal-organic Frameworks with Photocatalytic Bactericidal Activity for Integrated Air Cleaning. Nat. Commun. 10, 2177. doi:10.1038/s41467-019-10218-9
Li, X., Le, Z., Chen, X., Li, Z., Wang, W., Liu, X., et al. (2018b). Graphene Oxide Enhanced Amine-Functionalized Titanium Metal Organic Framework for Visible-Light-Driven Photocatalytic Oxidation of Gaseous Pollutants. Appl. Catal. B: Environ. 236, 501–508. doi:10.1016/j.apcatb.2018.05.052
Li, Y., Li, X., Zhang, H., Fan, J., and Xiang, Q. (2020b). Design and Application of Active Sites in G-C3n4-Based Photocatalysts. J. Mater. Sci. Tech. 56, 69–88. doi:10.1016/j.jmst.2020.03.033
Liotta, L. F. (2010). Catalytic Oxidation of Volatile Organic Compounds on Supported noble Metals. Appl. Catal. B: Environ. 100, 403–412. doi:10.1016/j.apcatb.2010.08.023
Ma, D., Liu, A., Li, S., Lu, C., and Chen, C. (2018). TiO2 Photocatalysis for C-C Bond Formation. Catal. Sci. Technol. 8, 2030–2045. doi:10.1039/c7cy01458a
Ma, X., Chai, Y., Li, P., and Wang, B. (2019). Metal-Organic Framework Films and Their Potential Applications in Environmental Pollution Control. Acc. Chem. Res. 52, 1461–1470. doi:10.1021/acs.accounts.9b00113
Pöschl, U., and Shiraiwa, M. (2015). Multiphase Chemistry at the Atmosphere-Biosphere Interface Influencing Climate and Public Health in the Anthropocene. Chem. Rev. 115, 4440–4475. doi:10.1021/cr500487s
Saouma, C. T., Richard, S., Smolders, S., Delley, M. F., Ameloot, R., Vermoortele, F., et al. (2018). Bulk-to-Surface Proton-Coupled Electron Transfer Reactivity of the Metal-Organic Framework MIL-125. J. Am. Chem. Soc. 140, 16184–16189. doi:10.1021/jacs.8b09120
Shah, S. J., Wang, R., Gao, Z., Muhammad, Y., Zhang, H., Zhang, Z., et al. (2021). IL-assisted Synthesis of Defect-Rich polyaniline/NH2-MIL-125 Nanohybrids with Strengthened Interfacial Contact for Ultra-fast Photocatalytic Degradation of Acetaldehyde under High Humidity. Chem. Eng. J. 411, 128590. doi:10.1016/j.cej.2021.128590
Shi, L., Yang, L., Zhang, H., Chang, K., Zhao, G., Kako, T., et al. (2018). Implantation of Iron(III) in Porphyrinic Metal Organic Frameworks for Highly Improved Photocatalytic Performance. Appl. Catal. B: Environ. 224, 60–68. doi:10.1016/j.apcatb.2017.10.033
Tian, L., Yang, X., Liu, Q., Qu, F., and Tang, H. (2018). Anchoring Metal-Organic Framework Nanoparticles on Graphitic Carbon Nitrides for Solar-Driven Photocatalytic Hydrogen Evolution. Appl. Surf. Sci. 455, 403–409. doi:10.1016/j.apsusc.2018.06.014
Wang, C.-C., Li, J.-R., Lv, X.-L., Zhang, Y.-Q., and Guo, G. (2014). Photocatalytic Organic Pollutants Degradation in Metal-Organic Frameworks. Energy Environ. Sci. 7, 2831–2867. doi:10.1039/c4ee01299b
Wang, H., Yu, T., Tan, X., Zhang, H., Li, P., Liu, H., et al. (2016). Enhanced Photocatalytic Oxidation of Isopropanol by HKUST-1@TiO2 Core-Shell Structure with Ultrathin Anatase Porous Shell: Toxic Intermediate Control. Ind. Eng. Chem. Res. 55, 8096–8103. doi:10.1021/acs.iecr.6b01400
Wang, Q., Gao, Q., Al-Enizi, A. M., Nafady, A., and Ma, S. (2020a). Recent Advances in MOF-Based Photocatalysis: Environmental Remediation under Visible Light. Inorg. Chem. Front. 7, 300–339. doi:10.1039/c9qi01120j
Wang, T., Wang, Y., Sun, M., Hanif, A., Wu, H., Gu, Q., et al. (2020b). Thermally Treated Zeolitic Imidazolate Framework-8 (ZIF-8) for Visible Light Photocatalytic Degradation of Gaseous Formaldehyde. Chem. Sci. 11, 6670–6681. doi:10.1039/d0sc01397h
Wen, M., Li, G., Liu, H., Chen, J., An, T., and Yamashita, H. (2019). Metal-organic Framework-Based Nanomaterials for Adsorption and Photocatalytic Degradation of Gaseous Pollutants: Recent Progress and Challenges. Environ. Sci. Nano 6, 1006–1025. doi:10.1039/c8en01167b
Xiao, J.-D., Han, L., Luo, J., Yu, S.-H., and Jiang, H.-L. (2018). Integration of Plasmonic Effects and Schottky Junctions into Metal-Organic Framework Composites: Steering Charge Flow for Enhanced Visible-Light Photocatalysis. Angew. Chem. Int. Ed. 57, 1103–1107. doi:10.1002/anie.201711725
Xiao, J.-D., and Jiang, H.-L. (2019). Metal-Organic Frameworks for Photocatalysis and Photothermal Catalysis. Acc. Chem. Res. 52, 356–366. doi:10.1021/acs.accounts.8b00521
Xiao, J. D., Shang, Q., Xiong, Y., Zhang, Q., Luo, Y., Yu, S. H., et al. (2016). Boosting Photocatalytic Hydrogen Production of a Metal-Organic Framework Decorated with Platinum Nanoparticles: The Platinum Location Matters. Angew. Chem. Int. Ed. 55, 9389–9393. doi:10.1002/anie.201603990
Yao, P., Liu, H., Wang, D., Chen, J., Li, G., and An, T. (2018). Enhanced Visible-Light Photocatalytic Activity to Volatile Organic Compounds Degradation and Deactivation Resistance Mechanism of Titania Confined inside a Metal-Organic Framework. J. Colloid Interf. Sci. 522, 174–182. doi:10.1016/j.jcis.2018.03.075
Zhang, J., Hu, Y., Qin, J., Yang, Z., and Fu, M. (2020a). TiO2-UiO-66-NH2 Nanocomposites as Efficient Photocatalysts for the Oxidation of VOCs. Chem. Eng. J. 385, 123814. doi:10.1016/j.cej.2019.123814
Zhang, M.-L., Zhai, Z.-M., Yang, X.-G., Huang, Y.-D., Zheng, Y.-J., and Ma, L.-F. (2020b). Near-Infrared Phosphorescence Emission of Binuclear Mn(II) Based Metal-Organic Framework for Efficient Photoelectric Conversion. Front. Chem. 8, 593948. doi:10.3389/fchem.2020.593948
Zhang, Z., Li, X., Liu, B., Zhao, Q., and Chen, G. (2016). Hexagonal Microspindle of NH2-MIL-101(Fe) Metal-Organic Frameworks with Visible-Light-Induced Photocatalytic Activity for the Degradation of Toluene. RSC Adv. 6, 4289–4295. doi:10.1039/c5ra23154j
Keywords: MOFs, photocatalytic degradation, indoor-air pollutants, VOCs, photocatalysis mechanism
Citation: Qian Y, Ma D and Zhong J (2021) Metal-Organic Frameworks With Variable Valence Metal-Photoactive Components: Emerging Platform for Volatile Organic Compounds Photocatalytic Degradation. Front. Chem. 9:749839. doi: 10.3389/fchem.2021.749839
Received: 30 July 2021; Accepted: 22 October 2021;
Published: 15 November 2021.
Edited by:
Fan Dong, University of Electronic Science and Technology of China, ChinaReviewed by:
Xiaofei Yang, Nanjing Forestry University, ChinaCopyright © 2021 Qian, Ma and Zhong. This is an open-access article distributed under the terms of the Creative Commons Attribution License (CC BY). The use, distribution or reproduction in other forums is permitted, provided the original author(s) and the copyright owner(s) are credited and that the original publication in this journal is cited, in accordance with accepted academic practice. No use, distribution or reproduction is permitted which does not comply with these terms.
*Correspondence: Dongge Ma, bWFkb25nZ2VAYnRidS5lZHUuY24=
Disclaimer: All claims expressed in this article are solely those of the authors and do not necessarily represent those of their affiliated organizations, or those of the publisher, the editors and the reviewers. Any product that may be evaluated in this article or claim that may be made by its manufacturer is not guaranteed or endorsed by the publisher.
Research integrity at Frontiers
Learn more about the work of our research integrity team to safeguard the quality of each article we publish.