- Key Laboratory of Resource Biology and Biotechnology in Western China, Ministry of Education, College of Life Sciences, Northwest University, Xi’an, China
O-linked β-N-acetylglucosamine modification (O-GlcNAcylation) at proteins with low-abundance expression level and species diversity, shows important roles in plenty of biological processes. O-GlcNAcylations with abnormal expression levels are associated with many diseases. Systematically profiling of O-GlcNAcylation at qualitative or quantitative level is vital for their function understanding. Recently, the combination of affinity enrichment, metabolic labeling or chemical tagging with mass spectrometry (MS) have made significant contributions to structure-function mechanism elucidating of O-GlcNAcylations in organisms. Herein, this review provides a comprehensive update of MS-based methodologies for quali-quantitative characterization of O-GlcNAcylation.
Introduction
O-GlcNAcylation, a ubiquitous post-translational modification (PTM) on nuclear, and cytoplasmic proteins (Hart et al., 2007), takes charge of numerous cardinal biological processes, such as signal transduction, transcriptional regulation, stress response, etc. Abnormal expression of O-GlcNAcylation is associated with some diseases, such as alzheimer (Yuzwa and Vocadlo, 2014), diabetes mellitus (Yang et al., 2008) and cancer (Nie et al., 2020). Therefore, the qualitative and quantitative study of glycosylation pattern of O-GlcNAcylated proteins is significant to understand the biological roles of O-GlcNAcylation during a pathological process.
Due to biological importance of O-GlcNAcylation, systematical characterization of O-GlcNAcylation has received increasing attention. However, O-GlcNAcylated proteins with multifarious types are often expressed at low level in organism, such as transcription factor CREB (Rexach et al., 2012) and protein kinase (Dias et al., 2009). Thus, systematically profiling of overall O-GlcNAcylation still faces challenges.
MS with advantages of high sensitivity and traces sample consumption has been widely used in the structural profiling of O-GlcNAcylation (Ma and Hart, 2017). Due to the low abundance and structural diversity of glycosylation, direct MS analysis of O-GlcNAcylation faces challenges. Usually, an enrichment step is necessary for MS-based profiling of O-GlcNAcylation. With the development of stable isotope tagging, quali-quantitative profiling of O-GlcNAcylation has made remarkable progress, accelerating the structure-function mechanism elucidation of O-GlcNAcylated proteins. We summarize the recent research progress in MS-based quali-quantitative analysis of O-GlcNAcylated proteins.
Qualitative Characterization of O-GlcNAcylation by MS
Direct MS
Earlier, collision-induced dissociation (CID), quadrupole time-of-flight (Q-TOF), electron-capture dissociation (ECD) and electron-transfer dissociation (ETD) MS have been used in O-GlcNAcylation analysis. O-GlcNAc shows easier dissociation character over other glycosylation at proteins during ionization procedure, enabling direct MS profiling of O-GlcNAc (Chalkley and Burlingame, 2001). However, the obtained GlcNAc fragment, oxonium ion, often afforded at low yield, leading to signal loss of the O-GlcNAcylation, which might be not suitable for detecting of O-GlcNAcylated proteins expressed at low levels in organism.
Lectin Enrichment for MS
Due to the low expression level of O-GlcNAcylation, an enrichment procedure is usually needed before MS identification of the O-GlcNAcylated proteins. Lectins with feature of bonding GlcNAc have been used in enrichment of the O-GlcNAcylated proteins.
After enriching O-GlcNAcylated proteins by Ricinus comminis agglutinin I (RCAI) and Wheat germ agglutinin (WGA) affinity chromatography, O-GlcNAcylated proteins have been well determined by LC-ES/MS (Hayes et al., 1995; Cieniewski-Bernard et al., 2004). Succinylated wheat germ agglutinin (sWGA) and Agrocybe aegerita lectin 2 (AAL2), which show better binding specificity over WGA, have been used for O-GlcNAcylated proteins enrichment for subsequent MS profiling (Kupferschmid et al., 2017; Liu et al., 2018).
However, the non-specific binding of lectin to other glycan (N-glycosylated GlcNAc terminal) might decrease the detection accuracy of glycosylation. Thus, a PNGase F digestion is needed before lectin enrichment.
Antibody Enrichment for MS
Pan-specific antibody, CTD110.6 that could bind to O-GlcNAc has been employed to enrich the O-GlcNAcylated proteins to improve MS characterization (Wells et al., 2002). To improve the enrichment of proteins, the combined utilization of three O-GlcNAc-specific IgG monoclonal antibodies [18B10.C7(3), 9D1.E4(10) and 1F5.D6(14)] to immunoprecipitate the O-GlcNAcylated proteins for subsequent O-GlcNAc-omics analysis by MS (Teo et al., 2010).
Given the importance of antibodies enrichment, the low bonding efficiency of antibodies to O-GlcNAcylated proteins and certain peptide dependence might reduce the detection accuracy.
Metabolic Engineering and Solid Phase Enrichment for MS
With the development of metabolic oligosaccharides engineering (MOE), the O-GlcNAcylated proteins could be labeled with the reactive groups (such as alkynyl, azide, etc.) for subsequent enrichment, as shown in Figure 1. Generally, cells were cultured with metabolic chemical reporters (MCRs) such as Ac4GlcNAz (Sprung et al., 2005), Ac4GlcNAlk (Zaro et al., 2011), Ac36AzGlcNAc (Chuh et al., 2014), Ac34dGlcNAz (Li et al., 2016), Ac36AlkGlcNAc (Chuh et al., 2017), Ac46AzGlc (Darabedian et al., 2018), Ac36AzGalNAc (Guo et al., 2019) and 1,3-Pr2GalNAz (Hao et al., 2019), etc., to synthesize O-GlcNAcylated proteins with active reactive groups. Then, the biotin probes with corresponding reactive groups (Figure 1B) were introduced to tag the labeled O-GlcNAcylations through staudinger linkage, copper-catalyzed azido-alkyne cycloaddition (CuAAC) or strain-promoted azide-alkyne cycloaddition (SPAAC). Finally, the characterization of O-GlcNAcylated proteins could be achieved by MS profiling after the biotin-avidin enrichment.
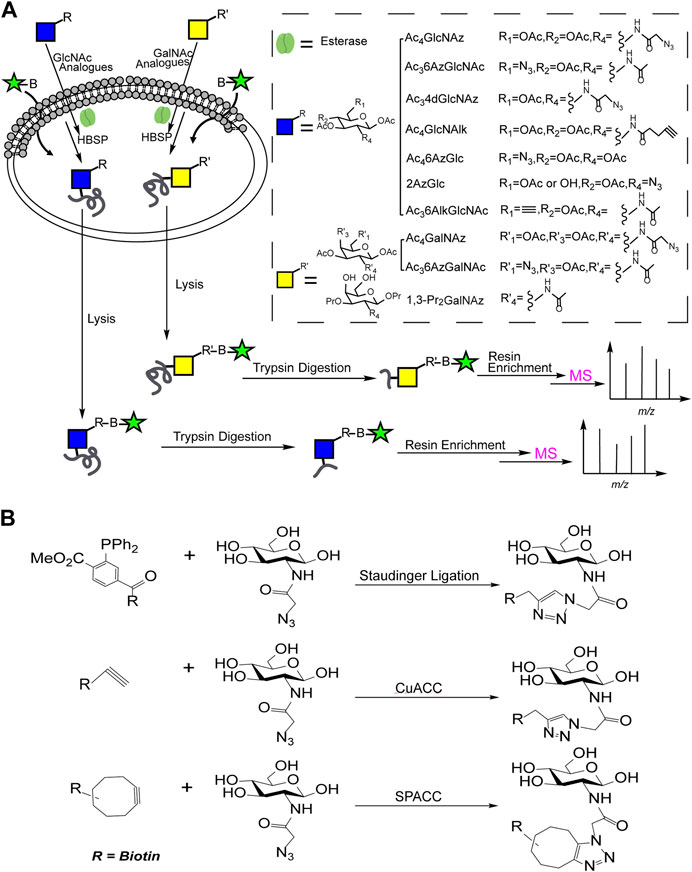
FIGURE 1. (A) The combination of MOE and solid phase enrichment for MS profiling of O-GlcNAcylated proteins; (B) Reactions involved in solid phase enrichment of O-GlcNAcylation.
The combination of MOE and solid phase enrichment for MS profiling has made great contribution in charactering of O-GlcNAcylation. Nevertheless, some unspecific labeling to other glycosylation such as S-glycoylation was observed (Qin et al., 2020).
Chemoenzymatic Labeling and Solid Phase Enrichment for MS
As shown in Supplementary Figure S1, GalT Y289L could transfer UDP-galactose analogues with reactive groups (ketone, alkynyl or azide) to C4-position of the O-GlcNAc at proteins. Then the labeled O-GlcNAcylated proteins could be captured by biotins with reactive groups through the orthogonal reactions such as ammoxidatin reaction (Tai et al., 2004) or click chemical reaction (Ma et al., 2019) for MS profiling. Since O-GlcNAc transferase (OGT) can recognize GlcNAc at other glycan terminals, the N-glycosylation interference should be eliminated by a PNGase F digestion before chemoenzymatic labeling.
Nevertheless, the enriched O-GlcNAcylated proteins might be difficult to elute for subsequent MS profiling. The developments of cleavable biotin linkers such as disulfide linker (Tsai et al., 2010), photocleavable linker (Li et al., 2019), acid cleavable linker (Szychowski et al., 2010), and diazobenzene linker (Yang et al., 2010), or affinity column with hydrazide cleavable linker (Nishikaze et al., 2013) to improve the dissociation efficiency of the enriched molecules have enabled more effectively profiling of O-GlcNAcylation by MS.
Quali-Quantitative Characterization of O-GlcNAcylation by MS
MS-Based Quali-Quantitative Characterization of O-GlcNAcylation Using 0D/6D-BEMAD Strategy
The glycosylation site of O-GlcNAcylation could be labeled with a nucleophile tag [dithiotreitol (DTT)] by β-elimination followed by Michael addition (BEMAD). As a result, the unstable O-GlcNAc glycosidic bond has been converted to be a stable derivative, enabling characterization of the O-GlcNAcylation by MS (Hédou et al., 2009). However, BEMAD strategy may not be suitable for distinguishing phosphorylation from O-GlcNAcylation.
When involving 0D/6D-DTT in BEMAD strategy, MS-based quali-quantitative characterization of O-GlcNAcylation could be achieved. Two samples, respectively, digested by PNGase F and trypsin digestion were subjected to 0D/6D-BEMAD, as shown in Supplementary Figure S2. Then the labeled glycopeptides captured through a mercaptans affinity chromatography and equally mixed were subjected to MS-based quali-quantitative characterization (Vosseller et al., 2005).
To improve detection efficiency and accuracy of O-GlcNAcylation by MS, an enrichment step for O-GlcNAcylated proteins has been involved (such as lectin, chemoenzyme labeling, etc.) before BEMAD.
MS-Based Quali-Quantitative Characterization of O-GlcNAcylation by Metabolic Labeling of Stable Isotope Labels
By feeding cells with 12C and 13C glucose successively, the O-GlcNAcylated proteins could be labeled through the hexosamine biosynthetic pathway. Then the dynamic changes of O-GlcNAcylated proteins during biological procedure were determined by MS, as shown in Supplementary Figure S3A (Wang et al., 2016).
As shown in Supplementary Figure S3B, feeding cells in the presence of normal (light) or isotopically enriched (heavy) amino acid could produce normally or isotopically labeled proteins by SILAC (stable isotope labeling with amino acids). After 1:1 mixing, the mixture subjected to trypsin digestion and enriched by affinity chromatography (antibodies, lectin, etc.) to capture O-GlcNAc modified peptides, were later assigned by MS-based quali-quantitative characterization (Wang et al., 2007).
MS-Based Quali-Quantitative Characterization of O-GlcNAcylation by Chemoenzymatic and Stable Isotope Labeling
As shown in Figure 2A, GalT Y289L transfers UDP-galactose analogues with reactive groups (acetylene or azide) to the C4-position of the O-GlcNAc. The “light” (0D, 12C, or 14N probe) and “heavy” (isotope-labeled, 7D, 13C, or 15N probe) biotin linker, respectively, were used to label each O-GlcNAcylation via biological orthogonal reaction. Then MS-based quali-quantitative characterization of O-GlcNAcylation between two samples was achieved after equally mixing (Qin et al., 2018; Li et al., 2019).
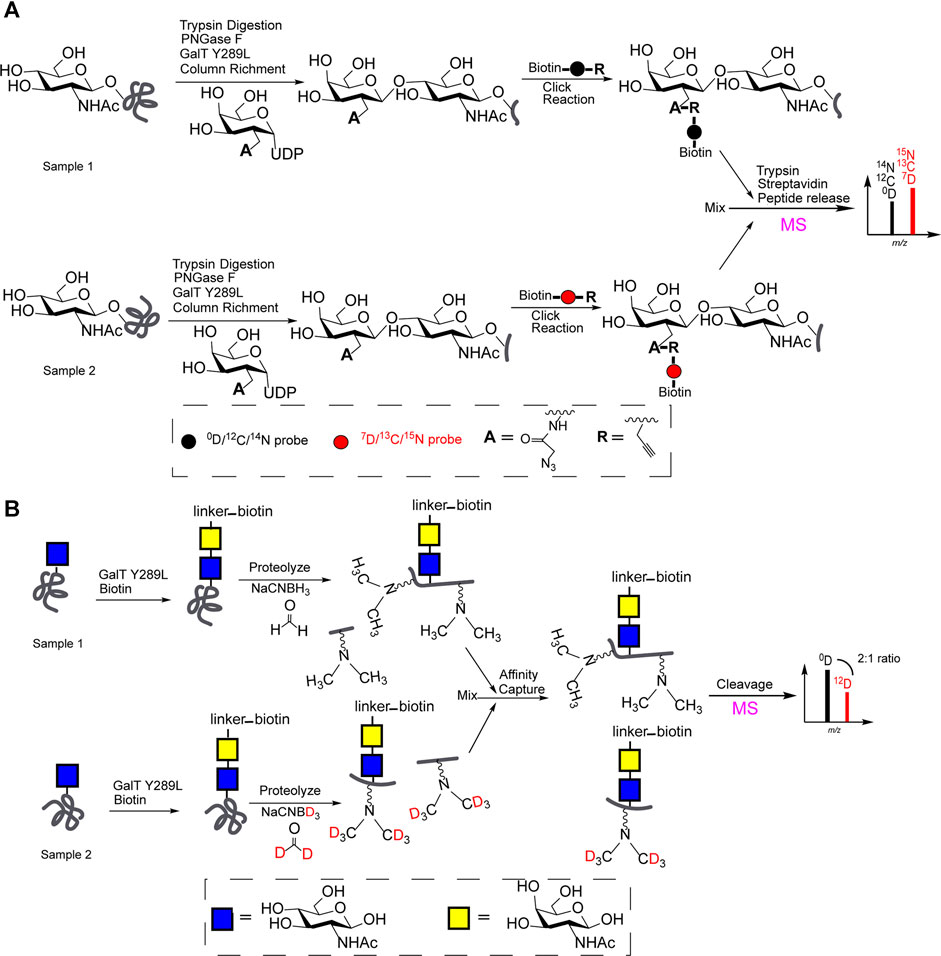
FIGURE 2. (A) Chemoenzymatic and stable isotope labeling (“light” and “heavy” biotin linker) for MS-based quali-quantitative characterization of O-GlcNAcylation; (B) QUIC-Tag for MS-based quali-quantitative profiling of O-GlcNAcylation.
Meanwhile, quantitative isotopic and chemoenzymatic tagging (QUIC-Tag) for MS-based quali-quantitative profiling of O-GlcNAcylation, was illustrated in Figure 2B. Generally, samples were enriched by avidin-biotin affinity chromatography after a chemoenzymatic labeling of O-GlcNAc. After a trypsin digestion, two samples (peptides) respectively were treated with formaldehyde/NaCNBH3 or deuterated formaldehyde/NaCNBD3 via reductive amination reaction for subsequent MS profiling. The expression levels of O-GlcNAcylation at proteins involved in the regulation of transcription has been quantitatively characterized (Khidekel et al., 2007).
Conclusion
O-GlcNAcylation plays an important role in plenty of biological activities, abnormal changes of O-GlcNAcylation are closely associated with the development of kinds of diseases. MS with advantages of quali-quantitatively profiling structural details of glycan compositions, glycosidic linkages and glycosylation sites, has accelerated understanding the O-GlcNAcylations.
Due to the low abundance and structural diversity of O-GlcNAc modified proteins, the combination of MOE or chemoenzymatic labeling, isotopic tagging or affinity chromatography enrichment with MS-based quali-quantitative profiling, have played important roles in understanding the biological roles of O-GlcNAcylation. However, some non-specific bonding (lectin), low bonding capacity (antibody) and unspecific labeling (S-glycosylation) occur, as summarized in Supplementary Tables S1, S2. Still, attentions should be paid to the development of specific enrichment strategy for selectively capturing the O-GlcNAcylated proteins.
Even enriching the O-GlcNAcylated proteins by specific affinity chromatography, the phosphorylation at peptide would produce the false positive signal, bringing inevitable interference in signal assignation. Efforts should be focused on developing MS-based technique combined with chemical releasing strategy to distinguish O-GlcNAcylations from O-phosphorylation in future.
Author Contributions
All authors listed have made a substantial, direct, and intellectual contribution to the work and approved it for publication.
Funding
This work was financially supported by the scientific research program funded by the Department of Education of Shaanxi Province (20JC035), the National Natural Science Foundation (Nos. 31300678 and 81803002), and the Basic Research Program of Natural Science of Shaanxi Province (2016JQ3018).
Conflict of Interest
The authors declare that the research was conducted in the absence of any commercial or financial relationships that could be construed as a potential conflict of interest.
Publisher’s Note
All claims expressed in this article are solely those of the authors and do not necessarily represent those of their affiliated organizations, or those of the publisher, the editors and the reviewers. Any product that may be evaluated in this article, or claim that may be made by its manufacturer, is not guaranteed or endorsed by the publisher.
Supplementary Material
The Supplementary Material for this article can be found online at: https://www.frontiersin.org/articles/10.3389/fchem.2021.737093/full#supplementary-material
References
Chalkley, R. J., and Burlingame, A. L. (2001). Identification of GlcNAcylation Sites of Peptides and α-crystallin Using Q-TOF Mass Spectrometry. J. Am. Soc. Mass. Spectrom. 12, 1106–1113. doi:10.1016/s1044-0305(01)00295-1
Chuh, K. N., Batt, A. R., Zaro, B. W., Darabedian, N., Marotta, N. P., Brennan, C. K., et al. (2017). The New Chemical Reporter 6-Alkynyl-6-Deoxy-GlcNAc Reveals O-GlcNAc Modification of the Apoptotic Caspases that Can Block the Cleavage/activation of Caspase-8. J. Am. Chem. Soc. 139, 7872–7885. doi:10.1021/jacs.7b02213
Chuh, K. N., Zaro, B. W., Piller, F., Piller, V., and Pratt, M. R. (2014). Changes in Metabolic Chemical Reporter Structure Yield a Selective Probe of O-GlcNAc Modification. J. Am. Chem. Soc. 136, 12283–12295. doi:10.1021/ja504063c
Cieniewski-Bernard, C., Bastide, B., Lefebvre, T., Lemoine, J., Mounier, Y., and Michalski, J.-C. (2004). Identification of O-Linked N-Acetylglucosamine Proteins in Rat Skeletal Muscle Using Two-Dimensional Gel Electrophoresis and Mass Spectrometry. Mol. Cell Proteomics 3, 577–585. doi:10.1074/mcp.M400024-MCP200
Darabedian, N., Gao, J., Chuh, K. N., Woo, C. M., and Pratt, M. R. (2018). The Metabolic Chemical Reporter 6-Azido-6-Deoxy-Glucose Further Reveals the Substrate Promiscuity of O-GlcNAc Transferase and Catalyzes the Discovery of Intracellular Protein Modification by O-Glucose. J. Am. Chem. Soc. 140, 7092–7100. doi:10.1021/jacs.7b13488
Dias, W. B., Cheung, W. D., Wang, Z., and Hart, G. W. (2009). Regulation of Calcium/calmodulin-dependent Kinase IV by O-GlcNAc Modification. J. Biol. Chem. 284, 21327–21337. doi:10.1074/jbc.M109.007310
Guo, J., Zhang, G., Ma, J., Zhao, C., Xue, Q., Wang, J., et al. (2019). Detection and Identification of O-GlcNAc-Modified Proteins Using 6-Azido-6-Deoxy-N-Acetyl-Galactosamine. Org. Biomol. Chem. 17, 4326–4334. doi:10.1039/c9ob00516a
Hao, Y., Fan, X., Shi, Y., Zhang, C., Sun, D.-e., Qin, K., et al. (2019). Next-generation Unnatural Monosaccharides Reveal that ESRRB O-GlcNAcylation Regulates Pluripotency of Mouse Embryonic Stem Cells. Nat. Commun. 10, 4065. doi:10.1038/s41467-019-11942-y
Hart, G. W., Housley, M. P., and Slawson, C. (2007). Cycling of O-Linked β-N-acetylglucosamine on Nucleocytoplasmic Proteins. Nature 446, 1017–1022. doi:10.1038/nature05815
Hayes, B. K., Greis, K. D., and Hart, G. W. (1995). Specific Isolation of O-Linked N-Acetylglucosamine Glycopeptides from Complex Mixtures. Anal. Biochem. 228, 115–122. doi:10.1006/abio.1995.1322
Hédou, J., Bastide, B., Page, A., Michalski, J.-C., and Morelle, W. (2009). Mapping of O-Linkedβ-N-Acetylglucosamine Modification Sites in Key Contractile Proteins of Rat Skeletal Muscle. Proteomics 9, 2139–2148. doi:10.1002/pmic.200800617
Khidekel, N., Ficarro, S. B., Clark, P. M., Bryan, M. C., Swaney, D. L., Rexach, J. E., et al. (2007). Probing the Dynamics of O-GlcNAc Glycosylation in the Brain Using Quantitative Proteomics. Nat. Chem. Biol. 3, 339–348. doi:10.1038/nchembio881
Kupferschmid, M., Aquino-Gil, M. O., Shams-Eldin, H., Schmidt, J., Yamakawa, N., Krzewinski, F., et al. (2017). Identification of O-GlcNAcylated Proteins in Plasmodium Falciparum. Malar. J. 16, 485. doi:10.1186/s12936-017-2131-2
Li, J., Li, Z., Duan, X., Qin, K., Dang, L., Sun, S., et al. (2019). An Isotope-Coded Photocleavable Probe for Quantitative Profiling of Protein O-GlcNAcylation. ACS Chem. Biol. 14, 4–10. doi:10.1021/acschembio.8b01052
Li, J., Wang, J., Wen, L., Zhu, H., Li, S., Huang, K., et al. (2016). An OGA-Resistant Probe Allows Specific Visualization and Accurate Identification of O-GlcNAc-Modified Proteins in Cells. ACS Chem. Biol. 11, 3002–3006. doi:10.1021/acschembio.6b00678
Liu, W., Han, G., Yin, Y., Jiang, S., Yu, G., Yang, Q., et al. (2018). AANL (Agrocybe Aegerita Lectin 2) Is a New Facile Tool to Probe for O-GlcNAcylation. Glycobiology 28, 363–373. doi:10.1093/glycob/cwy029
Ma, J., and Hart, G. W. (2017). Analysis of Protein O‐GlcNAcylation by Mass Spectrometry. Curr. Protoc. Protein Sci. 87, 24. doi:10.110.1610.1002/cpps.2410.1002/cpps.24
Ma, J., Wang, W.-H., Li, Z., Shabanowitz, J., Hunt, D. F., and Hart, G. W. (2019). O-GlcNAc Site Mapping by Using a Combination of Chemoenzymatic Labeling, Copper-free Click Chemistry, Reductive Cleavage, and Electron-Transfer Dissociation Mass Spectrometry. Anal. Chem. 91, 2620–2625. doi:10.1021/acs.analchem.8b05688
Nie, H., Ju, H., Fan, J., Shi, X., Cheng, Y., Cang, X., et al. (2020). O-GlcNAcylation of PGK1 Coordinates Glycolysis and TCA Cycle to Promote Tumor Growth. Nat. Commun. 11, 1–14. doi:10.1038/s41467-019-13601-8
Nishikaze, T., Kawabata, S.-i., Iwamoto, S., and Tanaka, K. (2013). Reversible Hydrazide Chemistry-Based Enrichment for O-GlcNAc-Modified Peptides and Glycopeptides Having Non-reducing GlcNAc Residues. Analyst 138, 7224–7232. doi:10.1039/c3an00880k
Qin, K., Zhang, H., Zhao, Z., and Chen, X. (2020). Protein S-Glyco-Modification through an Elimination-Addition Mechanism. J. Am. Chem. Soc. 142, 9382–9388. doi:10.1021/jacs.0c02110
Qin, K., Zhu, Y., Qin, W., Gao, J., Shao, X., Wang, Y.-l., et al. (2018). Quantitative Profiling of Protein O-GlcNAcylation Sites by an Isotope-Tagged Cleavable Linker. ACS Chem. Biol. 13, 1983–1989. doi:10.1021/acschembio.8b00414
Rexach, J. E., Clark, P. M., Mason, D. E., Neve, R. L., Peters, E. C., and Hsieh-Wilson, L. C. (2012). Dynamic O-GlcNAc Modification Regulates CREB-Mediated Gene Expression and Memory Formation. Nat. Chem. Biol. 8, 253–261. doi:10.1038/nchembio.770
Sprung, R., Nandi, A., Chen, Y., Kim, S. C., Barma, D., Falck, J. R., et al. (2005). Tagging-via-substrate Strategy for Probing O-GlcNAc Modified Proteins. J. Proteome Res. 4, 950–957. doi:10.1021/pr050033j
Szychowski, J., Mahdavi, A., Hodas, J. J. L., Bagert, J. D., Ngo, J. T., Landgraf, P., et al. (2010). Cleavable Biotin Probes for Labeling of Biomolecules via Azide−Alkyne Cycloaddition. J. Am. Chem. Soc. 132, 18351–18360. doi:10.1021/ja1083909
Tai, H.-C., Khidekel, N., Ficarro, S. B., Peters, E. C., and Hsieh-Wilson, L. C. (2004). Parallel Identification of O-GlcNAc-Modified Proteins from Cell Lysates. J. Am. Chem. Soc. 126, 10500–10501. doi:10.1021/ja047872b
Teo, C. F., Ingale, S., Wolfert, M. A., Elsayed, G. A., Nöt, L. G., Chatham, J. C., et al. (2010). Glycopeptide-specific Monoclonal Antibodies Suggest New Roles for O-GlcNAc. Nat. Chem. Biol. 6, 338–343. doi:10.1038/nchembio.338
Tsai, C.-S., Liu, P.-Y., Yen, H.-Y., Hsu, T.-L., and Wong, C.-H. (2010). Development of Trifunctional Probes for Glycoproteomic Analysis. Chem. Commun. 46, 5575–5577. doi:10.1039/c0cc00345j
Vosseller, K., Hansen, K. C., Chalkley, R. J., Trinidad, J. C., Wells, L., Hart, G. W., et al. (2005). Quantitative Analysis of Both Protein Expression and Serine?/?threonine post-translational Modifications through Stable Isotope Labeling with Dithiothreitol. Proteomics 5, 388–398. doi:10.1002/pmic.200401066
Wang, X., Yuan, Z.-F., Fan, J., Karch, K. R., Ball, L. E., Denu, J. M., et al. (2016). A Novel Quantitative Mass Spectrometry Platform for Determining Protein O-GlcNAcylation Dynamics. Mol. Cell Proteomics 15, 2462–2475. doi:10.1074/mcp.O115.049627
Wang, Z., Pandey, A., and Hart, G. W. (2007). Dynamic Interplay between O-Linked N-Acetylglucosaminylation and Glycogen Synthase Kinase-3-dependent Phosphorylation. Mol. Cell Proteomics 6, 1365–1379. doi:10.1074/mcp.M600453-MCP200
Wells, L., Vosseller, K., Cole, R. N., Cronshaw, J. M., Matunis, M. J., and Hart, G. W. (2002). Mapping Sites of O-GlcNAc Modification Using Affinity Tags for Serine and Threonine post-translational Modifications. Mol. Cell Proteomics 1, 791–804. doi:10.1074/mcp.M200048-MCP200
Yang, X., Ongusaha, P. P., Miles, P. D., Havstad, J. C., Zhang, F., So, W. V., et al. (2008). Phosphoinositide Signalling Links O-GlcNAc Transferase to Insulin Resistance. Nature 451, 964–969. doi:10.1038/nature06668
Yang, Y.-Y., Grammel, M., Raghavan, A. S., Charron, G., and Hang, H. C. (2010). Comparative Analysis of Cleavable Azobenzene-Based Affinity Tags for Bioorthogonal Chemical Proteomics. Chem. Biol. 17, 1212–1222. doi:10.1016/j.chembiol.2010.09.012
Yuzwa, S. A., and Vocadlo, D. J. (2014). O-GlcNAc and Neurodegeneration: Biochemical Mechanisms and Potential Roles in Alzheimer's Disease and beyond. Chem. Soc. Rev. 43, 6839–6858. doi:10.1039/C4CS00038B
Keywords: mass spectrometry, O-GlcNAcylation, O-GlcNAc, O-GlcNAcylated proteins, quali-quantitative charactering, isotope labeling
Citation: Yin R, Wang X, Li C, Gou Y, Ma X, Liu Y, Peng J, Wang C and Zhang Y (2021) Mass Spectrometry for O-GlcNAcylation. Front. Chem. 9:737093. doi: 10.3389/fchem.2021.737093
Received: 06 July 2021; Accepted: 21 October 2021;
Published: 06 December 2021.
Edited by:
Ganglong Yang, Jiangnan University, ChinaReviewed by:
Zhenli Zhu, China University of Geosciences Wuhan, ChinaGuanghui Han, BGI Americas, United States
Copyright © 2021 Yin, Wang, Li, Gou, Ma, Liu, Peng, Wang and Zhang. This is an open-access article distributed under the terms of the Creative Commons Attribution License (CC BY). The use, distribution or reproduction in other forums is permitted, provided the original author(s) and the copyright owner(s) are credited and that the original publication in this journal is cited, in accordance with accepted academic practice. No use, distribution or reproduction is permitted which does not comply with these terms.
*Correspondence: Ying Zhang, zhangying@nwu.edu.cn