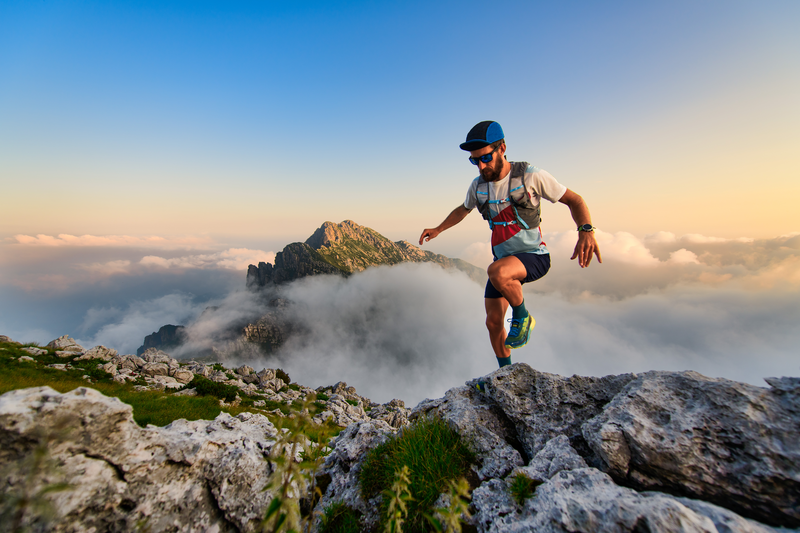
94% of researchers rate our articles as excellent or good
Learn more about the work of our research integrity team to safeguard the quality of each article we publish.
Find out more
ORIGINAL RESEARCH article
Front. Chem. , 16 July 2021
Sec. Organic Chemistry
Volume 9 - 2021 | https://doi.org/10.3389/fchem.2021.711257
A Brønsted acid-catalyzed domino ring-opening cyclization transformation of donor-acceptor (D-A) cyclopropanes and 2-naphthols has been developed. This formal [3+2] cyclization reaction provided novel and efficient access to the naphthalene-fused cyclopentanes in the absence of any transition-metal catalysts or additives. This robust procedure was completed smoothly on a gram-scale to afford the corresponding product with comparable efficiency. Furthermore, the synthetic application of the prepared product has been demonstrated by its transformation into a variety of synthetically useful molecules.
The demands for effective assembly of diverse molecular scaffolds are continuously growing along with the development of organic chemistry. Among various methods, domino ring-opening cyclization has recently emerged as a powerful tool for the rapid build-up of molecular complexity (Bhattacharyya et al., 2016; Lin et al., 2017; Sayyad et al., 2017; Yi et al., 2018; Wan and Liu, 2019). As a versatile class of three-atom building blocks, donor-acceptor (D-A) cyclopropanes have experienced an unexpected renaissance in the last 2 decades, which are widely exploited in methodology as well as natural product synthesis (Cavitt et al., 2014; Schneider et al., 2014; Grover et al., 2015; Novikov, 2015; Reiser, 2016; Ivanova and Trushkov, 2019; Werz and Biju, 2020). Due to their property of formation of 1,3-zwitterion intermediates with the help of the ring strain, D-A cyclopropanes could enter multitudinous kinds of chemical transformations with different counterparts in organic synthesis. Among the multiple reactions, Lewis acid-catalyzed (3 + n) ring-opening cyclization of D-A cyclopropanes represent the most convenient method to form the carbocycles and heterocycles, such as (3 + 2) cycloaddition with an unsaturated C-C multiple bond (Augustin et al., 2018; Ding et al., 2019; Huang et al., 2019; Mondal et al., 2019; Verma, et al., 2019; Xie et al., 2019), (3 + 3) cycloaddition with 1,3-dipoles (Dhote and Ramana, 2019; Petzold et al., 2019), and (3 + 4) cycloaddition with conjugated dienes (Ivanova et al., 2008; Garve et al., 2016; Wang et al., 2017; Zhang et al., 2017; Augustin et al., 2019a; Li et al., 2020) (Scheme 1A). In addition, the basic transformation of D-A cyclopropanes usually focuses on straightforward ring-opening reactions with nucleophiles, which allows ready access to 1,3-bifunctionalized derivatives (Garve et al., 2017; Lücht et al., 2017; Wallbaum et al., 2017; Das and DaniliucArmido, 2018; Augustin et al., 2019b; Lücht et al., 2019; Boichenko et al., 2020; Guin et al., 2020) (Scheme 1B). Moreover, the unexpected rearrangement of D-A cyclopropanes could lead to partially unsaturated five-membered heterocycles (Ivanova et al., 2018; Ortega, 2018; Shim et al., 2018) (Scheme 1C).
Typically, all the catalytic systems of D-A cyclopropanes employ high loadings of Lewis acidic catalysts, usually rare-earth triflates, with the reactions typically operating at elevated temperatures. Compared with those of Lewis acid-catalyzed reactions, the Brønsted acid-catalyzed conversion of donor-acceptor cyclopropanes has received only scant attention. In 2014, (3 + 2)-annulation of donor-acceptor cyclopropanes with alkynes induced by both Lewis and Brønsted acids was reported by Budynina (Rakhmankulov et al., 2015) (Scheme 2A). In 2018, Moran and co-workers presented an elegant nucleophilic ring opening of D-A cyclopropanes with nucleophiles in the presence of TfOH (Richmond et al., 2018) (Scheme 2B). Thus, developing sustainable alternative to achieve Brønsted acid-catalyzed reactions of donor-acceptor cyclopropanes is highly desirable. We notice that 2-naphthols commonly serve as important aromatic feedstocks in organic chemistry (Zhuo and You, 2013; Wang et al., 2015; Yang et al., 2015; Zheng et al., 2015; Cheng et al., 2016; Shen et al., 2017; Tu et al., 2017; Fang et al., 2018; Liu et al., 2018; Xia et al., 2019; Zhang et al., 2020), and Biju disclosed a formal (3 + 2) cyclopentannulation of 2-naphthols and D-A cyclopropanes catalyzed by Bi(OTf)3 and KPF6 (Kaicharla et al., 2016). But in the case of a reaction involving D-A cyclopropanes with vinyl as the only substrate, the cyclization product is obtained in an unsatisfactory yield (42%), which greatly inhibits the universality of the reaction. Given the versatility of the vinyl, here we report the successful realization of such a scenario, whereby TfOH acts as a highly active and general catalyst for the (3 + 2) dehydration annulation of D-A cyclopropanes and 2-naphthols (Scheme 2C). The salient features of this transformation include: (a) the use of nonmetallic, low-toxicity, and easily available TfOH as the catalyst, (b) simple and benign reaction conditions in the absence of additives, (c) a broad substrate scope with respect to 2-vinylcyclopropane-1,1-dicarboxylate in moderate to high yields, beyond the yields and scope disclosed in the previous work, and (d) the resulting product is easily transformed into synthetically useful compounds.
We commenced our investigation with 2-naphthol Scheme 1A and diethyl 2-vinylcyclopropane-1,1-dicarboxylate Scheme 2A as model substrates. To our delight, treatment of Scheme 1A and Scheme 2A with 20 mol% of TfOH without other additives in toluene at 0°C furnished the (3 + 2) annulation product Scheme 3A in a 40% yield (Table 1, entry 1). Encouraged by the initial result, we then focused on solvent screening, and typical solvents including CH3CN, iPrOH, DCE, hexane, and DCM were tested for the reaction (Table 1, entries 2–6). The results revealed that the solvents have great influence on the reaction outcome. Notably, DCM gave optimal results (77% yield, Table 1, entry 6) while others led to low yields of Scheme 3A. Next, the evaluation of a series of Brønsted acids were conducted, such as TsOH, MsOH, (±)-CSA, TFA, AcOH, HCl, H2SO4, and H3PO4. However, only under the catalysis of TsOH, MsOH, and TFA, the desired product was furnished at a 26–70% yield (Table 1, entries 7, 8, 10). Furthermore, efforts in running the reaction at room temperature proved to be unfruitful, as a slightly decreased yield (60%) of Scheme 3A was observed, and a complex reaction system was obtained when elevating the reaction temperature to 50°C (Table 1, entries 12–13).
TABLE 1. Optimization of reaction conditionsa.
With the optimized conditions determined, the generality of substrates with respect to 2-naphthols was then explored. As summarized in Scheme 3, an array of 2-naphthols underwent successful cyclization with diethyl 2-vinylcyclopropane-1,1-dicarboxylate Scheme 2A. First, 6-Br-2-naphthol was reacted with Scheme 2A, and the corresponding product Scheme 3B was obtained in an 83% yield. Whereas more electron-withdrawing cyano substituent decreased the performance of the reaction, providing almost no desirable product Scheme 3C. In addition, when the substrate with Br at the position of C7 of 2-naphthol was subjected to this reaction, it afforded Scheme 3D in a 76% yield. It is worth noting that when 2,7-dinaphthol bearing two reactive sites was chosen as the substrate, much to our surprise, monocyclic product Scheme 3E was isolated in a 62% yield. We speculated that a two-fold annulation product could be hampered by the unfavorable steric effect. Additionally, 2-naphthol with stronger electron-donating methoxy at the C7 position was also suitable for this reaction. Reaction of various 2-naphthol substrates bearing electron-donating or -withdrawing substituents at the phenyl residue provided the desired cyclization products in moderate to good yields (Schemes 3G–J, 60–72%). It is fascinating that the phenoxyphenyl substituent was also suitable to this condition, leading to a 65% yield of Scheme 3I. The structure of the Schemes 3A–J were characterized by 1H, 13C NMR, and HRMS (See Supplementary Material).
Next, we moved our attention to explore the scope of donor-acceptor cyclopropanes under the optimized conditions (Scheme 4). A series of 2-vinylcyclopropane-1,1-dicarboxylate (2, R = methyl, isopropyl, n-butyl) were compatible with the reaction conditions, leading to the corresponding dehydration annulation products in 77–87% yields. Unfortunately, D-A cyclopropane with tert-butyl shut down the desired transformation, presumably because the tert-butyl was readily hydrolyzed under strong acidic conditions. Similarly, when diisopropyl 2-vinylcyclopropane-1,1-dicarboxylate was reacted with substituted 2-naphthols, the desired products were isolated in 55–82% yields (Schemes 3O–3R). In addition, aromatic donors such as phenyl residues in this protocol were also successful, and an electron-donating substituent attached to the aromatic backbone worked in a moderate yield (Scheme 3T, 70% yield). Whereas more electron-withdrawing groups (F, Cl, Br) were also tolerated (Schemes 3U–W). Replacement of the benzene ring with a furan moiety in the substrate proved to be fine for the transformation (see Scheme 3X). The structure of the Schemes 3K–X were characterized by 1H, 13C NMR, and HRMS (See Supplementary Material).
Encouraged by the high efficiency of the domino ring-opening cyclization reaction of donor-acceptor cyclopropanes with 2-naphthols, this TfOH-catalyzed reaction was completed smoothly on a gram-scale to afford the corresponding naphthalene-fused cyclopentane Scheme 3O with comparable efficiency (75% yield, Scheme 5). Interestingly, an extraordinary ring-opening reaction initiated at the end of the double bond of D-A cyclopropane Scheme 2A could be accessed when phenol was used as the substrate, uncyclized product Scheme 5 was afforded in a 52% yield, which suggested that ring-opening occurred via an SN2′-like mechanistic pathway. The structure of the Scheme 5 was characterized in the Supplementary Material.
To illustrate the application of this protocol, the transformation reactions with respect to product Scheme 3K were investigated (Scheme 6). First, efforts were focused on the versatile vinyl functional group, and the epoxidation of Scheme 3K with m-CPBA gave Scheme 6A in a 78% yield. In the presence of 9-BBN, Scheme 3K underwent hydroboration-oxidation to deliver primary alcohol Scheme 6B (93% yield). Furthermore, the treatment of Scheme 3K with LiCl in DMSO and H2O (9:1) furnished the selective decarboxylic product Scheme 6C in a 70% yield. Finally, the hydrolysis/decarboxylation reaction of Scheme 3K under an alkaline condition led to monocarboxyl product Scheme 6D in a 45% yield. The structure of the Schemes 6A–D were characterized by 1H, 13C NMR, and HRMS (See Supplementary Material).
Based on the previous report, we proposed a plausible mechanism of this Brønsted acid-catalyzed reaction (Scheme 7). Initial protonation of the “acceptor-motif” of cyclopropane Scheme 2A by TfOH possibly generates the intermediate A, in which the polarization of C−C bond increases. Ring-opening reaction of Scheme 1A to A generates the intermediate B. The subsequent intermolecular aldol reaction generates the cyclopentane intermediate C, which eliminates a molecule of water, and then forms the final product Scheme 3A, along with the regeneration of the TfOH catalyst which enters the next catalytic cycle.
In summary, we have developed a robust strategy involving a Brønsted acid-facilitated domino ring-opening cyclization reaction, which provides efficient access to ubiquitous cyclopenta (a)naphthalene in moderate to good yields with high regioselectivity. Most importantly, this transformation avoids the use of metal-catalysts and external additives. Notably, a useful gram-scale reaction was completed smoothly via this protocol. Further applications involving Brønsted acid as a catalyst are under investigation in our laboratory and will be reported in due course.
The original contributions presented in the study are included in the article/Supplementary Material, further inquiries can be directed to the corresponding authors.
HuZ designed the work. HuZ and PS carried out the experimental part. HuZ, DS, HoZ, and YZ organized and wrote the manuscript.
We thank the National Natural Science Foundation of China (no. 22001137), Natural Science Foundation of Zhejiang Province (no. LQ20B020003), and Natural Science Foundation of Ningbo (no. 202003N4111) for financial support.
The authors declare that the research was conducted in the absence of any commercial or financial relationships that could be construed as a potential conflict of interest.
The Supplementary Material for this article can be found online at: https://www.frontiersin.org/articles/10.3389/fchem.2021.711257/full#supplementary-material
Augustin, A. U., Busse, M., Jones, P. G., and Werz, D. B. (2018). Formal Insertion of Thioketenes into Donor-Acceptor Cyclopropanes by Lewis Acid Catalysis. Org. Lett. 20, 820–823. doi:10.1021/acs.orglett.7b03961
Augustin, A. U., Jones, P. G., and Werz, D. B. (2019a). Ring‐Opening 1,3‐Aminochalcogenation of Donor-Acceptor Cyclopropanes: A Three‐Component Approach. Chem. Eur. J. 25, 11620–11624. doi:10.1002/chem.201902160
Augustin, A. U., Merz, J. L., Jones, P. G., Mlostoń, G., and Werz, D. B. (2019b). (4 + 3)-Cycloaddition of Donor-Acceptor Cyclopropanes with Thiochalcones: A Diastereoselective Access to Tetrahydrothiepines. Org. Lett. 21, 9405–9409. doi:10.1021/acs.orglett.9b03623
Bhattacharyya, A., Kavitha, C. V., and Ghorai, M. K. (2016). Stereospecific Synthesis of 2-Iminothiazolidines via Domino Ring-Opening Cyclization of Activated Aziridines with Aryl- and Alkyl Isothiocyanates. J. Org. Chem. 81, 6433–6443. doi:10.1021/acs.joc.6b01551
Boichenko, M. A., Andreev, I. A., Chagarovskiy, A. O., Levina, I. I., Zhokhov, S. S., Trushkov, I. V., et al. (2020). Ring Opening of Donor-Acceptor Cyclopropanes with Cyanide Ion and its Surrogates. J. Org. Chem. 85, 1146–1157. doi:10.1021/acs.joc.9b03098
Cavitt, M. A., Phun, L. H., and France, S. (2014). Intramolecular Donor-Acceptor Cyclopropane Ring-Opening Cyclizations. Chem. Soc. Rev. 43, 804–818. doi:10.1039/c3cs60238a
Cheng, Q., Wang, Y., and You, S.-L. (2016). Chemo-, Diastereo-, and Enantioselective Iridium-Catalyzed Allylic Intramolecular Dearomatization Reaction of Naphthol Derivatives. Angew. Chem. Int. Ed. 55, 3496–3499. doi:10.1002/anie.201511519
Das, S., DaniliucArmido, C. G., and Studer, A. (2018). Lewis Acid Catalyzed Stereoselective Dearomative Coupling of Indolylboron Ate Complexes with Donor-Acceptor Cyclopropanes and Alkyl Halides. Angew. Chem. Int. Ed. 57, 4053–4057. doi:10.1002/anie.201711923
Dhote, P. S., and Ramana, C. V. (2019). One-Pot Au[III]-/Lewis Acid Catalyzed Cycloisomerization of Nitroalkynes and [3 + 3]Cycloaddition with Donor-Acceptor Cyclopropanes. Org. Lett. 21, 6221–6224. doi:10.1021/acs.orglett.9b02035
Ding, W.-P., Zhang, G.-P., Jiang, Y.-J., Du, J., Liu, X.-Y., Chen, D., et al. (2019). Electron-Deficient Alkynes as Dipolarophile in Pd-Catalyzed Enantioselective (3 + 2) Cycloaddition Reaction with Vinyl Cyclopropanes. Org. Lett. 21, 6805–6810. doi:10.1021/acs.orglett.9b02431
Fang, X., Zeng, Y., Li, Q., Wu, Z., Yao, H., and Lin, A. (2018). Redox-Neutral Atom-Economic Pd(0)-Catalyzed Dearomatization of β-Naphthols with Alkynes toward Naphthalenones. Org. Lett. 20, 2530–2533. doi:10.1021/acs.orglett.8b00662
Garve, L. K. B., Jones, P. G., and Werz, D. B. (2017). Ring-Opening 1-Amino-3-Aminomethylation of Donor-Acceptor Cyclopropanes via 1,3-Diazepanes. Angew. Chem. Int. Ed. 56, 9226–9230. doi:10.1002/anie.201704619
Garve, L. K. B., Pawliczek, M., Wallbaum, J., Jones, P. G., and Werz, D. B. (2016). [4+3] Cycloaddition of Donor-Acceptor Cyclopropanes with Amphiphilic Benzodithioloimine as Surrogate for Ortho -Bisthioquinone. Chem. Eur. J. 22, 521–525. doi:10.1002/chem.201504013
Grover, H. K., Emmett, M. R., and Kerr, M. A. (2015). Carbocycles from Donor-Acceptor Cyclopropanes. Org. Biomol. Chem. 13, 655–671. doi:10.1039/c4ob02117g
Guin, A., Rathod, T., Gaykar, R. N., Roy, T., and Biju, A. T. (2020). Lewis Acid Catalyzed Ring-Opening 1,3-Aminothiolation of Donor-Acceptor Cyclopropanes Using Sulfenamides. Org. Lett. 22, 2276–2280. doi:10.1021/acs.orglett.0c00483
Huang, X.-B., Li, X.-J., Li, T.-T., Chen, B., Chu, W.-D., He, L., et al. (2019). Palladium-Catalyzed Highly Enantioselective Cycloaddition of Vinyl Cyclopropanes with Imines. Org. Lett. 21, 1713–1716. doi:10.1021/acs.orglett.9b00274
Ivanova, O. A., Budynina, E. M., Grishin, Y. K., Trushkov, I. V., and Verteletskii, P. V. (2008). Donor-Acceptor Cyclopropanes as Three-Carbon Components in a [4+3] Cycloaddition Reaction with 1,3-Diphenylisobenzofuran. Angew. Chem. Int. Ed. 47, 1107–1110. doi:10.1002/anie.200704438
Ivanova, O. A., Chagarovskiy, A. O., Shumsky, A. N., Krasnobrov, V. D., Levina, I. I., and Trushkov, I. V. (2018). Lewis Acid Triggered Vinylcyclopropane-Cyclopentene Rearrangement. J. Org. Chem. 83, 543–560. doi:10.1021/acs.joc.7b02351
Ivanova, O. A., and Trushkov, I. V. (2019). Donor‐Acceptor Cyclopropanes in the Synthesis of Carbocycles. Chem. Rec. 19, 2189–2208. doi:10.1002/tcr.201800166
Kaicharla, T., Roy, T., Thangaraj, M., Gonnade, R. G., and Biju, A. T. (2016). Lewis Acid Catalyzed Selective Reactions of Donor-Acceptor Cyclopropanes with 2-Naphthols. Angew. Chem. Int. Ed. 55, 10061–10064. doi:10.1002/anie.201604373
Li, B. Q., Qiu, Z.-W., Ma, A.-J., Peng, J.-B., Feng, N., Du, J.-Y., et al. (2020). Diastereoselective Synthesis of Cycloheptannelated Indoles via Lewis-Acid-Catalyzed (4 + 3)-Cyclization of Donor-Acceptor Cyclopropanes. Org. Lett. 22, 1903–1907. doi:10.1021/acs.orglett.0c00248
Lin, T.-Y., Wu, H.-H., Feng, J.-J., and Zhang, J. (2017). Divergent Access to Functionalized Pyrrolidines and Pyrrolines via Iridium-Catalyzed Domino-Ring-Opening Cyclization of Vinyl Aziridines with β-Ketocarbonyls. Org. Lett. 19, 6526–6529. doi:10.1021/acs.orglett.7b03232
Liu, X., Wang, P., Bai, L., Li, D., Wang, L., Yang, D., et al. (2018). Construction of Vicinal All-Carbon Quaternary Stereocenters Enabled by a Catalytic Asymmetric Dearomatization Reaction of β-Naphthols with 3-Bromooxindoles. ACS Catal. 8, 10888–10894. doi:10.1021/acscatal.8b03905
Lücht, A., Patalag, L. J., Augustin, A. U., Jones, P. G., and Werz, D. B. (2017). Reactions of Donor-Acceptor Cyclopropanes with Naphthoquinones: Redox and Lewis Acid Catalysis Working in Concert. Angew. Chem. Int. Ed. 56, 10587–10591. doi:10.1002/anie.201703732
Lücht, A., Sobottka, S., Patalag, L. J., Jones, P. G., Reissig, H. U., Sarkar, B., et al. (2019). New Dyes Based on Extended Fulvene Motifs: Synthesis through Redox Reactions of Naphthoquinones with Donor-Acceptor Cyclopropanes and Their Spectroelectrochemical Behavior. Chem. Eur. J. 25, 10359–10365. doi:10.1002/chem.201900764
Mondal, M., Panda, M., McKee, V., and Kerrigan, N. J. (2019). Asymmetric Synthesis of Tetrahydrofurans through Palladium(0)-Catalyzed [3 + 2]-Cycloaddition of Vinylcyclopropanes with Ketenes. J. Org. Chem. 84, 11983–11991. doi:10.1021/acs.joc.9b01854
Novikov, R. A., and Tomilov, Y. V. (2015). Dimerization of Donor-Acceptor Cyclopropanes. Mendeleev Commun. 25, 1–10. doi:10.1016/j.mencom.2015.01.001
Ortega, A., Manzano, R., Uria, U., Carrillo, L., Reyes, E., Tejero, T., et al. (2018). Catalytic Enantioselective Cloke-Wilson Rearrangement. Angew. Chem. Int. Ed. 57, 8225–8229. doi:10.1002/anie.201804614
Petzold, M., Jones, P. G., and Werz, D. B. (2019). (3+3)‐Annulation of Carbonyl Ylides with Donor-Acceptor Cyclopropanes: Synergistic Dirhodium(II) and Lewis Acid Catalysis. Angew. Chem. Int. Ed. 58, 6225–6229. doi:10.1002/anie.201814409
Rakhmankulov, E. R., Ivanov, K. L., Budynina, E. M., Ivanova, O. A., Chagarovskiy, A. O., Skvortsov, D. A., et al. (2015). Lewis and Brønsted Acid Induced (3 + 2)-Annulation of Donor-Acceptor Cyclopropanes to Alkynes: Indene Assembly. Org. Lett. 17, 770–773. doi:10.1021/ol5037562
Reiser, O. (2016). Catalytic Conversion of Furans and Pyrroles to Natural Products and Analogues Utilizing Donor-Acceptor Substituted Cyclopropanes as Key Intermediates. Isr. J. Chem. 56, 531–539. doi:10.1002/ijch.201500103
Richmond, E., Vuković, V. D., and Moran, J. (2018). Nucleophilic Ring Opening of Donor-Acceptor Cyclopropanes Catalyzed by a Brønsted Acid in Hexafluoroisopropanol. Org. Lett. 20, 574–577. doi:10.1021/acs.orglett.7b03688
Sayyad, M., Wani, I. A., Babu, R., Nanaji, Y., and Ghorai, M. K. (2017). A Synthetic Route to Chiral 1,4-Disubstituted Tetrahydro-β-Carbolines via Domino Ring-Opening Cyclization of Activated Aziridines with 2-Vinylindoles. J. Org. Chem. 82, 2364–2374. doi:10.1021/acs.joc.6b02719
Schneider, T. F., Kaschel, J., and Werz, D. B. (2014). A New Golden Age for Donor-Acceptor Cyclopropanes. Angew. Chem. Int. Ed. 53, 5504–5523. doi:10.1002/anie.201309886
Shen, D., Chen, Q., Yan, P., Zeng, X., and Zhong, G. (2017). Enantioselective Dearomatization of Naphthol Derivatives with Allylic Alcohols by Cooperative Iridium and Brønsted Acid Catalysis. Angew. Chem. Int. Ed. 56, 3242–3246. doi:10.1002/anie.201609693
Shim, S. Y., Choi, Y., and Ryu, D. H. (2018). Asymmetric Synthesis of Cyclobutanone via Lewis Acid Catalyzed Tandem Cyclopropanation/Semipinacol Rearrangement. J. Am. Chem. Soc. 140, 11184–11188. doi:10.1021/jacs.8b06835
Tu, H.-F., Zheng, C., Xu, R.-Q., Liu, X.-J., and You, S.-L. (2017). Iridium-Catalyzed Intermolecular Asymmetric Dearomatization of β-Naphthols with Allyl Alcohols or Allyl Ethers. Angew. Chem. Int. Ed. 56, 3237–3241. doi:10.1002/anie.201609654
Verma, K., Taily, I. M., and Banerjee, P. (2019). Exploitation of Donor-Acceptor Cyclopropanes and N-Sulfonyl 1-azadienes towards the Synthesis of spiro-cyclopentane Benzofuran Derivatives. Org. Biomol. Chem. 17, 8149–8152. doi:10.1039/c9ob01369e
Wallbaum, J., Garve, L. K. B., Jones, P. G., and Werz, D. B. (2017). Ring-Opening 1,3-Halochalcogenation of Cyclopropane Dicarboxylates. Org. Lett. 19, 98–101. doi:10.1021/acs.orglett.6b03375
Wan, S.-H., and Liu, S.-T. (2019). Functionalized Pyrroles from Vinylaziridines and Alkynes via Rhodium-Catalyzed Domino Ring-Opening Cyclization Followed by C C Bond Migration. Tetrahedron 75, 1166–1170. doi:10.1016/j.tet.2019.01.022
Wang, S. G., Liu, X. J., Zhao, Q. C., Zheng, C., Wang, S. B., and You, S. L. (2015). Asymmetric Dearomatization of β‐Naphthols through a Bifunctional‐Thiourea‐Catalyzed Michael Reaction. Angew. Chem. Int. Ed. 54, 14929–14932. doi:10.1002/anie.201507998
Wang, Z.-H., Zhang, H.-H., Wang, D.-M., Xu, P.-F., and Luo, Y.-C. (2017). Lewis Acid Catalyzed Diastereoselective [3+4]-annulation of Donor-Acceptor Cyclopropanes with Anthranils: Synthesis of Tetrahydro-1-Benzazepine Derivatives. Chem. Commun. 53, 8521–8524. doi:10.1039/c7cc04239f
Werz, D. B., and Biju, A. T. (2020). Uncovering the Neglected Similarities of Arynes and Donor-Acceptor Cyclopropanes. Angew. Chem. Int. Ed. 59, 3385–3398. doi:10.1002/anie.201909213
Xia, Z.-L., Zheng, C., Xu, R.-Q., and You, S.-L. (2019). Chiral Phosphoric Acid Catalyzed Aminative Dearomatization of α-naphthols/Michael Addition Sequence. Nat. Commun. 10, 3150. doi:10.1038/s41467-019-11109-9
Xie, M.-S., Zhao, G.-F., Qin, T., Suo, Y.-B., Qu, G.-R., and Guo, H.-M. (2019). Thiourea Participation in [3+2] Cycloaddition with Donor-Acceptor Cyclopropanes: a Domino Process to 2-Amino-Dihydrothiophenes. Chem. Commun. 55, 1580–1583. doi:10.1039/c8cc09595g
Yang, D., Wang, L., Han, F., Li, D., Zhao, D., and Wang, R. (2015). Intermolecular Enantioselective Dearomatization Reaction of β-Naphthol Usingmeso-Aziridine: A Bifunctional In Situ Generated Magnesium Catalyst. Angew. Chem. Int. Ed. 54, 2185–2189. doi:10.1002/anie.201410257
Yi, R., Li, X., and Wan, B. (2018). Ring-opening and Cyclization of Aziridines with Aryl Azides: Metal-free Synthesis of 6-(triflyloxy)quinolines. Org. Chem. Front. 5, 3488–3493. doi:10.1039/c8qo00984h
Zhang, C., Tian, J., Ren, J., and Wang, Z. (2017). Intramolecular Parallel [4+3] Cycloadditions of Cyclopropane 1,1-Diesters with [3]Dendralenes: Efficient Construction of [5.3.0]Decane and Corresponding Polycyclic Skeletons. Chem. Eur. J. 23, 1231–1236. doi:10.1002/chem.201605190
Zhang, H.-J., Gu, Q., and You, S.-L. (2020). Ni-Catalyzed Allylic Dearomatization Reaction of β-Naphthols with Allylic Alcohols. Org. Lett. 22 (8), 3297–3301. doi:10.1021/acs.orglett.0c01109
Zheng, J., Wang, S.-B., Zheng, C., and You, S.-L. (2015). Asymmetric Dearomatization of Naphthols via a Rh-Catalyzed C(sp2)-H Functionalization/Annulation Reaction. J. Am. Chem. Soc. 137, 4880–4883. doi:10.1021/jacs.5b01707
Keywords: donor−acceptor cyclopropane, 2-naphthol, brønsted acid, [3+2] cyclization, Naphthalene-fused cyclopentane
Citation: Zhao H, Shen P, Sun D, Zhai H and Zhao Y (2021) Transition-Metal-Free [3+2] Dehydration Cycloaddition of Donor-Acceptor Cyclopropanes With 2-Naphthols. Front. Chem. 9:711257. doi: 10.3389/fchem.2021.711257
Received: 18 May 2021; Accepted: 04 June 2021;
Published: 16 July 2021.
Edited by:
Guigen Li, Texas Tech University, United StatesReviewed by:
Daniel Werz, Technische Universitat Braunschweig, GermanyCopyright © 2021 Zhao, Shen, Sun, Zhai and Zhao. This is an open-access article distributed under the terms of the Creative Commons Attribution License (CC BY). The use, distribution or reproduction in other forums is permitted, provided the original author(s) and the copyright owner(s) are credited and that the original publication in this journal is cited, in accordance with accepted academic practice. No use, distribution or reproduction is permitted which does not comply with these terms.
*Correspondence: Hua Zhao, emhhb2h1YUBuYnUuZWR1LmNu; Hongbin Zhai, emhhaWhiQHBrdXN6LmVkdS5jbg==
Disclaimer: All claims expressed in this article are solely those of the authors and do not necessarily represent those of their affiliated organizations, or those of the publisher, the editors and the reviewers. Any product that may be evaluated in this article or claim that may be made by its manufacturer is not guaranteed or endorsed by the publisher.
Research integrity at Frontiers
Learn more about the work of our research integrity team to safeguard the quality of each article we publish.