- 1State Key Laboratory of Southwestern Chinese Medicine Resources, Hospital of Chengdu University of Traditional Chinese Medicine, School of Pharmacy, Chengdu University of Traditional Chinese Medicine, Chengdu, China
- 2State Key Laboratory of Biotherapy and Department of Urology, West China Hospital, Sichuan University, Chengdu, China
- 3School of Pharmacy and Sichuan Province College Key Laboratory of Structure-Specific Small Molecule Drugs, Chengdu Medical College, Chengdu, China
The activation of Ras small GTPases, including RalA and RalB, plays an important role in carcinogenesis, tumor progress, and metastasis. In the current study, we report the discovery of a series of 6-sulfonylamide-pyrano [2,3-c]-pyrazole derivatives as novel RalA inhibitors. ELISA-based biochemical assay results indicated that compounds 4k–4r suppressed RalA/B binding capacities to their substrates. Cellular proliferation assays indicated that these RalA inhibitors potently inhibited the proliferation of HCC cell lines, including HepG2, SMMC-7721, Hep3B, and Huh-7 cells. Among the evaluated compounds, 4p displayed good inhibitory capacities on RalA (IC50 = 0.22 μM) and HepG2 cells (IC50 = 2.28 μM). Overall, our results suggested that a novel small-molecule RalA inhibitor with a 6-sulfonylamide-pyrano [2, 3-c]-pyrazole scaffold suppressed autophagy and cell proliferation in hepatocellular carcinoma, and that it has potential for HCC-targeted therapy.
Introduction
Hepatocellular carcinoma (HCC) is a primary liver malignancy with one of the highest mortality rates worldwide (Jacobson et al., 2018; Zhou et al., 2018; Paludetto et al., 2019; Siegel et al., 2019). Although significant progress has been made to improve chemotherapy, transcatheter artery chemoembolization (TACE), and targeted HCC therapy, many patients still present recurrences and the development of drug resistance (Xue et al., 2019; Pan et al., 2020; Yu et al., 2020). Therefore, novel targeted therapeutic approaches are required to help overcome these problems. From a molecular-level perspective, an important HCC development factor is the imbalance of major signaling pathways, including Ras, p53, PI3K/Akt, and Wnt/β-catenin (Saha and Giri, 2019; Zhongqi et al., 2019). Ras—a small-molecule GTPase member—has important regulatory functions in cell signal transduction, cytoplasmic skeletal construction, and material transport (Ostrem et al., 2013; Lim et al., 2014; Lito et al., 2016). Notably, recent findings indicated that the Ras gene was mutated in more than 30% of HCC-afflicted patients sampled (Guichard et al., 2012; Lindsay and Blackhall, 2019; Suzuki et al., 2021). Mutations in the Ras gene have also been detected in various other types of cancers as well, including those for lung cancer, pancreatic cancer, and colon cancer (Zhang et al., 2018; Zhao et al., 2018; Chen et al., 2020). In the subseries of Ras signaling pathways, Ral has one of the most similar structures and functions compared to Ras, reaching up to 50% similarity for their sequences (Yan and Theodorescu, 2018). In the Ral small-GTPase branch, RalA and RalB are closely related G proteins with similar protein structures (Jiang et al., 2016). Their protein structures have an N-terminal free-drifting 11 amino acid sequence, a C-terminal membrane-targeting sequence, and a GTP- binding domain, which facilitate GDP/GTP binding (Zheng et al., 2016). The GTP- binding domain consists of five α-helices, six β-helices, and five loops. Their GTPase activity is low and requires the involvement of GTPase-activating proteins (GAPs) (Tracy et al., 2016). Additionally, the guanylate exchange factor (GEF) plays an important role in GTP/GDP binding and release (Hobbs et al., 2016). Usually, functional Ral members depend on their ability to cycle between activated (phosphorylated) and inactivated (dephosphorylated) Ral states. The Ral inhibitors can be divided into the following cases: targeted to Ral guanine exchange factors (RalGEFs); directly targeted to Ral; targeted to Ral effectors, and combinations of these therapies (Klose et al., 2016).
SCH-53239 was originally designed to inhibit guanine nucleotide exchange and control Ral signaling. A subsequent study elucidated the structure of SCH-53239 and led to the discovery of a derivative with a higher water solubility known as SCH-54292 (Gray et al., 2020). RBC8 has an efficient inhibitory effect on human and mouse platelets, preventing interactions with effector proteins and Ral-binding protein by binding to allosteric sites on GDP-bound Rals, leaving it in an inactive state. These types of compounds include currently reported Ral inhibitors, such as RBC6, RBC10, and BQU5726-27 (Figure 1). Prior structure analyses of BQU57 and RBC8 have suggested that the pyrano-fused pyrazole scaffold can bind to the RalA-GDP allosteric site (Alvarado and Giles, 2007; Hamada et al., 2011; Ezzeldin et al., 2014; Yan et al., 2016; Yan and Theodorescu, 2018; Walsh et al., 2019; Bum-Erdene et al., 2020; Xiong et al., 2020). In the current study, following a rationalized approach for strategic drug design, we aimed to develop methods to change the pyrazole ring N-methyl group into a substituted phenyl ring and add a sulfonyl group at the pyrano ring 2-amine group. These adjustments were performed to enhance RalA binding, via a 3-cyanide group and 4-aronmatic ring. The resultant pyrano[2,3-c]-pyrazole derivatives were assayed via their RalA and HCC cellular proliferation inhibitory capacities.
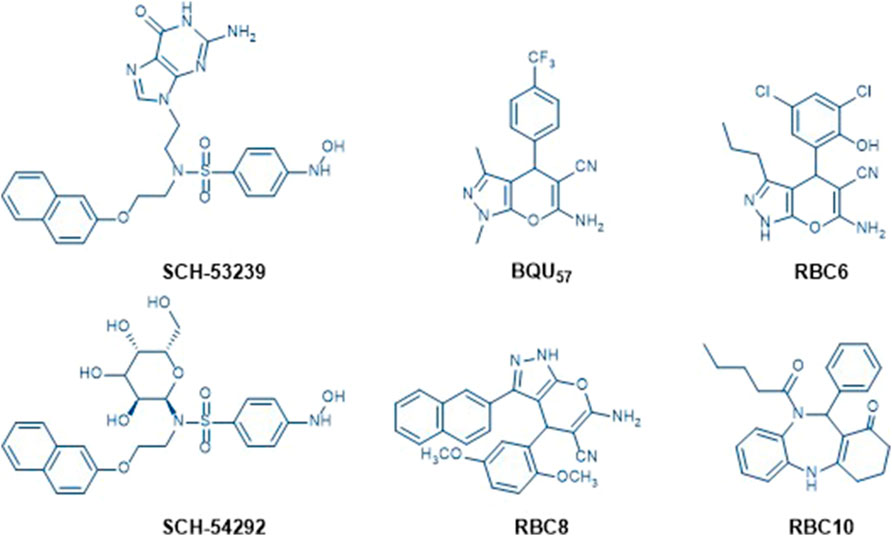
FIGURE 1. Chemical structures of known Ral inhibitors, including SCH-53239, SCH-54292, BQU57, RBC6, RBC8, and RBC10.
To achieve these objectives we designed and synthesized 6-sulfonamido-pyrano[2,3-c]-pyrazole–based RalA inhibitors. Then, we assayed their performance in HCC-based models in vitro and in vivo. We used HepG2 cells as vectors for in vitro ELISA-based biochemical assays, cell proliferation assays, and autophagy assays. We hypothesized that compound 4p would suppress RalA/B activation and cellular proliferation, as well as subsequently induce lethal autophagy in HCC-afflicted cells. Furthermore, we hypothesized that compound 4p would significantly suppress tumor growth using a HepG2 xenograft–based model. Overall, we expected that our findings and novel approach using RalA inhibitors will stimulate novel research that might achieve improved outcomes for targeted HCC therapies.
Results and Discussion
We observed that RalA mRNA levels were upregulated in the Oncomine database cancer subtype panels (Figure 2A). Results suggested that RalA was overexpressed in different cancer subtypes, including bladder cancer, breast cancer, liver cancer, and prostate cancer (He et al., 2019; Pan et al., 2018). Next, we assessed RalA mRNA expression patterns in the TCGA database. RalA was upregulated in ten TCGA subtype cohorts (fold change >2.0, p < 0.01) and was downregulated in two lung cancer cohorts (Figure 2B). The analysis of 369 HCC-afflicted tissues and 160 adjacent normal liver tissue samples from data in the TCGA and GTEx databases demonstrated that RalA mRNA expression in HCC-afflicted tissues was 2.67-fold higher than in adjacently sampled normal ones (Figure 2C) (Wang et al., 2018; Hao et al., 2019; Wang et al., 2019). Furthermore, the RalA mRNA levels were a significant marker to predict HCC-afflicted patients’ prognosis (Figure 2D). IHC-derived images of RalA proteins indicated that they were overexpressed in HCC vs. normal liver tissues (Figure 2E). Based on these results, we hypothesized that the upregulated RalA may be an attractive target for use in HCC-related therapies.
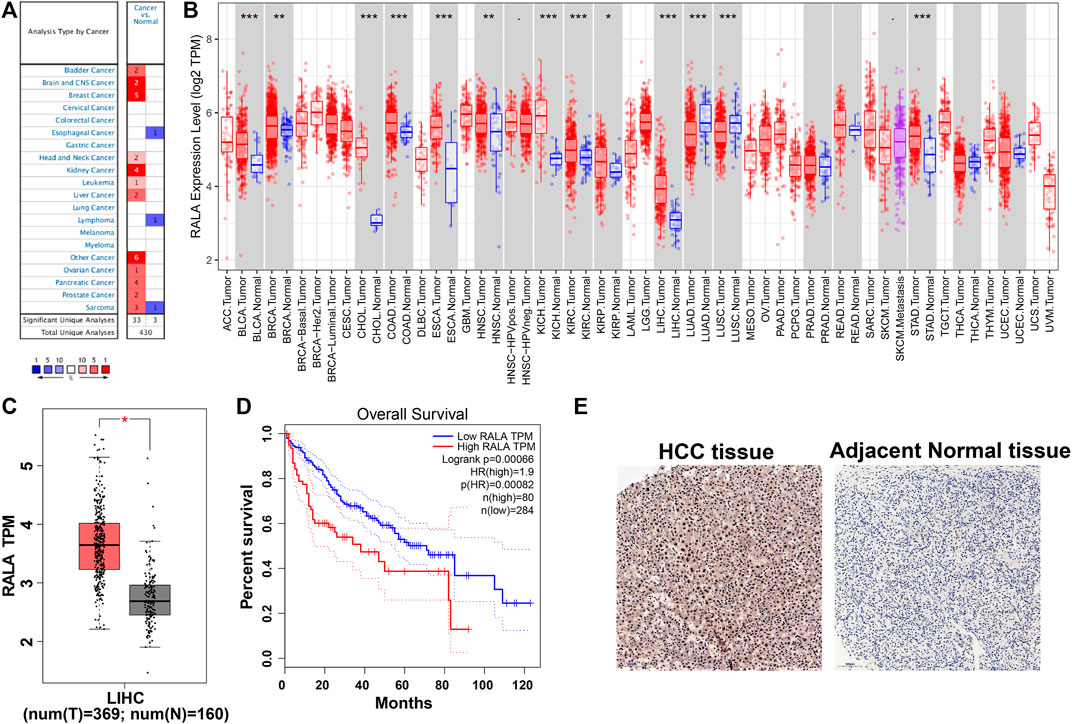
FIGURE 2. (A) Analysis of the differential RalA expression of various cancer types in the Oncomine database; (B) Differential expression profiles of various cancers and RalA in the TCGA database; (C) Differential expression of RalA protein in HCC and normal tissues; (D) Kalplan–Meier curves of RalA on the overall survival rate of HCC patients in the TCGA database; (E) Protein expression levels of RalA in HCC and adjacent normal tissues detected by IHC, scale bar: 100 μm.
BQU57 and RBC8 as RalA inhibitors were recently reported (Figure 3A) and suggested that they were characterized by a pyran-fused pyrazole scaffold. This type of a scaffold constitutes a core structure that can bind to the RalA-GDP allosteric site, with 2-amino and 3-cyanide groups on the pyran moiety, being key pharmacophores. Pyrano[2,3-c]-pyrazole–based RalA inhibitors’ effects on cancer cell proliferation have also been reported. However, we aimed to further develop efficient small-molecule allosteric RalA inhibitors based on these scaffold dynamics. Based on the necessary 5-nitrile-6-amino-pyrano[2, 3-c] pyrazole scaffold, variations in functional groups (R1 and R2) were introduced into the core structure, enriching the drug-like skeleton diversity (Figure 3B). The resulting compounds may, thus, have great potential to serve as foundations for exploring their uses as novel and efficient RalA inhibitors.
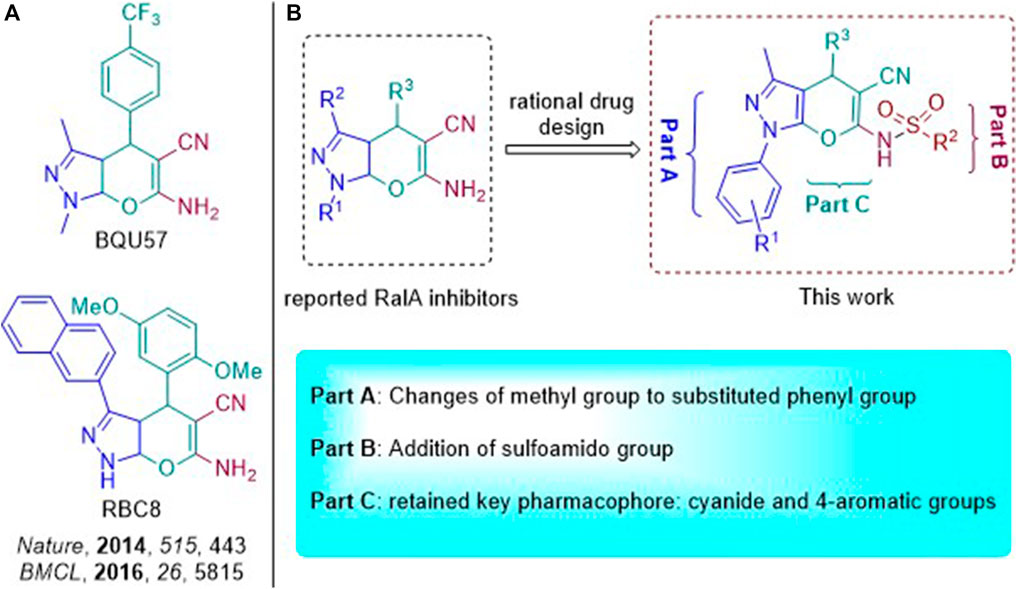
FIGURE 3. (A) Pyran-fused pyrazole derivatives reported to inhibit RalA; (B) Design strategy of novel RalA inhibitors.
Considering both feasible synthetic routes and the chemical diversity of novel RalA inhibitors, we employed the synthetic route depicted in Scheme 1. Briefly, the starting compound (1a–1o) was a cyano-olefin formed by aldehyde and Malononitrile reactions via Knoevenagel condensation in the presence of acetic acid and sodium acetate. The corresponding pyrazolone (2a–2f) was synthesized from ethyl acetoacetate and phenylhydrazine by heating and refluxing acetic acid constituents for 4 h. The 1a–1o and 2a–2f compounds were further used as raw materials. Then, morpholine was added into methanol (solvent) followed by heating and stirring for 30 min to form pyrano [2,3-c]pyrazole intermediates (3a–3x). Finally, intermediates 3a–3x reacted with a panel of sulfonyl chloride in the presence of DMAP using dichloromethane (DCM) as a solvent to obtain the target compound 4a–4ag (Scheme 1).
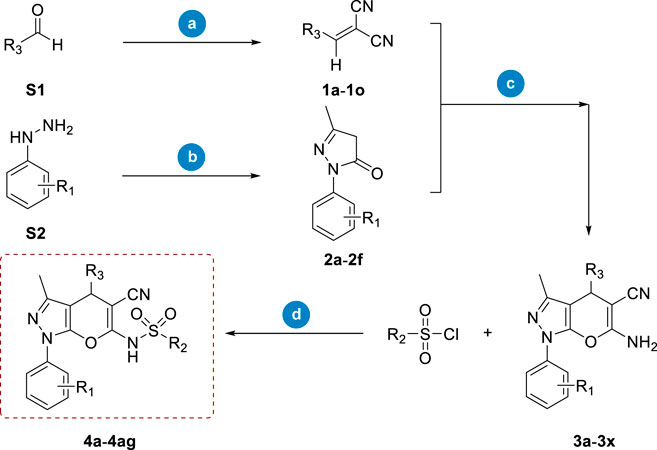
SCHEME 1. Synthetic route of compounds 4a–4ag. Reagents and conditions: (A). Malononitrile, acetic acid, and CH3COONa, overnight; (B). Ethyl acetoacetate, acetic acid, and reflux, 4 h; (C). Morpholine and methanol, 40°C, 30 min; (D). DMAP and DCM, 35°C, 12 h.
The structures of the synthesized target compounds (4a–4ag) were characterized by their HR-MS, 1H-NMR, and 13C-NMR spectra. Their RalA/B inhibitory activities at 1.0 μM and cellular proliferation data for HCC cell lines are shown in Table 1. When different pyrano [2,3-c]-pyrazole ring–type bioactivities were compared, the remaining RalA/B kinase activity was relatively high for pyran ring substituents with moderate steric hindrance (e.g. benzene rings or halogen-substituted phenyl groups). However, the corresponding HepG2 and Huh7 IC50 values were higher than 10 μM. When the 4-substitution of the pyran ring (R3 substitution) was a 4-trifluoromethyl phenyl or heteroaromatic ring, better activities were observed. Our findings also indicated that the hydrogen atom in the R1 group was preferred using 4s–4ag. Any changes at this site, such as halogen, methyl, or methoxyl substitutions led to a loss of RalA/B and HCC cell proliferation inhibitory activities. We found that the p-methoxybenzenesulfonyl group was the R2 -substituted fragment from compound 4n–4ab with the highest inhibitory activities against RalA/B and HCC cells. 1.0 μM of 4p was added before incubation, and only 13 and 22% of RalA and RalB kinase activities were detected, respectively. After compound 4p’s application, its IC50 values for HepG2 and Huh7 cells were 2.28 ± 0.23 and 4.31 ± 0.39 μM, respectively.
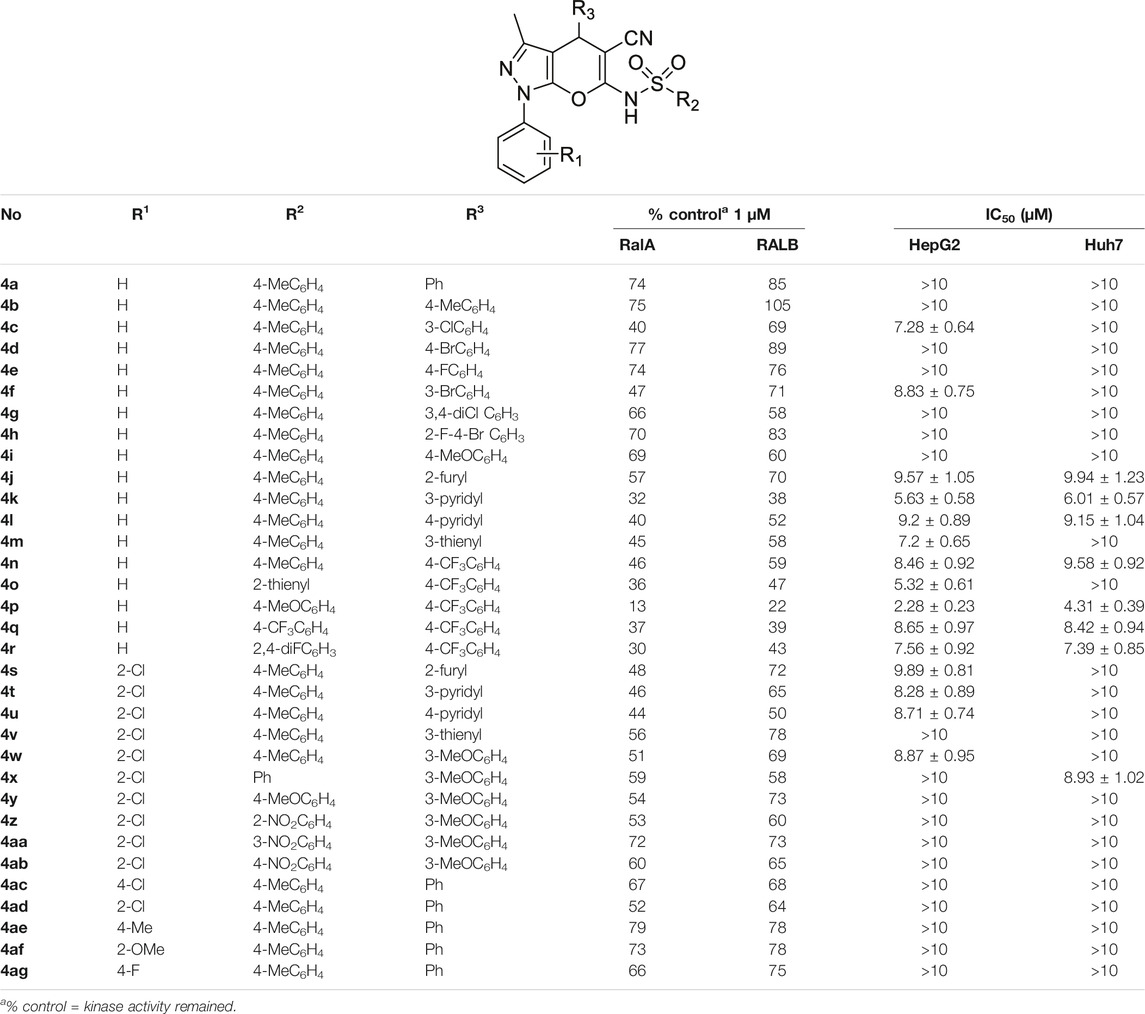
TABLE 1. Remaining kinase activities (%) after 1 μM compounds 4a–4ag incubation on RalA and RalB and cell proliferation inhibition.
In Table 2 we list the IC50 values for compounds 4k–4r for RalA/B and HepG2, Hep3B, Huh-7, and SMMC-7721 HCC cell proliferation. Compound 4p demonstrated the best RalA and RalB inhibitory activities with IC50 values of 0.22 and 0.41 μM, respectively. All eight compounds displayed good to moderately good inhibitory effects on RalA/B and HepG2 cell proliferation. Compounds 4l, 4m, and 4p exhibited potent cellular proliferation inhibitory capacities on the HCC cell lines. In the current study, HepG2 was the most sensitive cell line with IC50 values of 6.01, 9.15, and 2.28 μM for compounds 4l, 4m, and 4p, respectively. Therefore, 4p was selected as the best compound to increase the understanding of its RalA binding and its potential molecular mechanisms against HCC in subsequent experiments.
The 3D contours of 4p bounded to RalA allosteric sites are shown in Figure 4. We observed that the cyano group of 4p formed a hydrogen bond with the RalA Glu73 residue. The benzene ring at the R1 position and the pyrazole ring formed a π–π conjugation system with RalA Tyr82 residues, which might strengthen the stable binding. A cation–π interaction between 4p p-trifluoromethyl benzenesulfonyl fragment and RalA Arg79 residue was also detected.
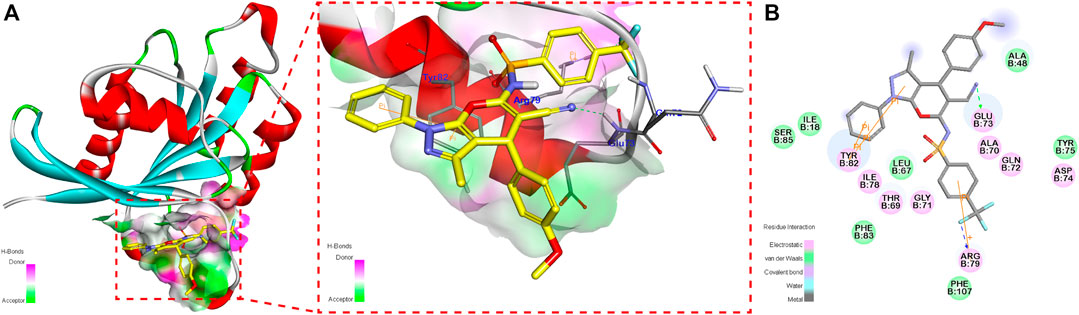
FIGURE 4. 3D contour (A) and 2D contour (B) of the binding mode of compound 4p in at the RalA-GDP allosteric site.
GST pulldown assays were performed to assess 4p RalA/B inhibitory capacities. 2.0 μM of compound 4p significantly suppressed activated RalA and RalB protein expression levels. Only activated RalA was suppressed after incubation with 0.5 μM of compound 4p (Figure 5A). Additionally, total RalA expression was unchanged, which suggested that 4p potentially interfered with RalBP1’s binding to RalA/B.
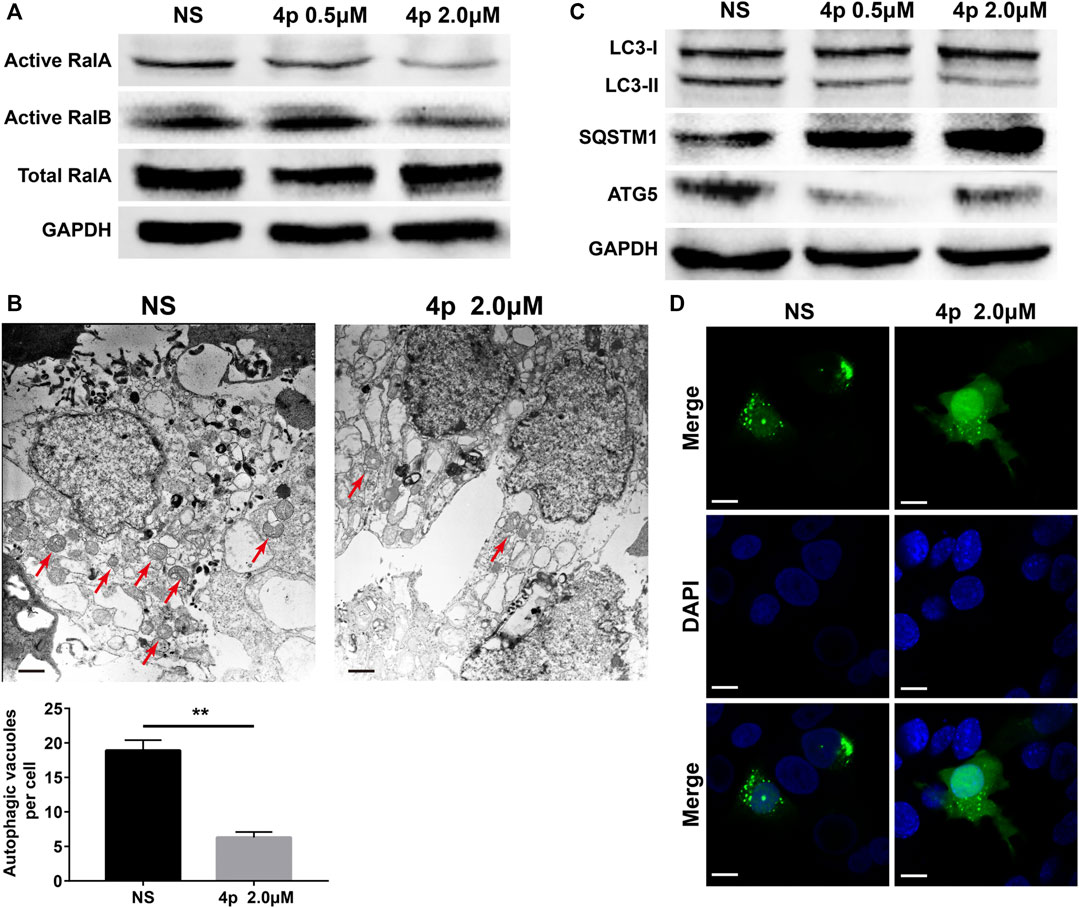
FIGURE 5. (A) Selective inhibition of 4p on RalA was observed by the GST pulldown test; (B) Transmission electron micrograph of HepG2 cells treated with 4p, scale bar: 500 nm; (C) Western blotting was used to detect the change of the LC3-II/I ratio; (D) Formation of autophagosome in GFP-LC3–transfected HepG2 cells induced by 2.0 μM 4p, scale bar: 6 μm.
Several reports have characterized RalA/B potential regulatory roles in autophagy (Zhang et al., 2019; Pang et al., 2020; Zhang et al., 2020). Considering the dual effect of HCC autophagy, we assessed changes in HepG2 cell autophagy levels after the addition of 0.5 or 2.0 μM of 4p, before incubation. Based on HepG2 cells’ TEM images with or without 4p (Figure 5B), autophagy vacuoles per cell decreased in the 4p-treated group (p < 0.01). After 4p treatments, LC3-II and ATG5 protein levels declined and SQSTM1 increased. These results suggested that 4p suppressed the autophagy flux in HepG2 cells (Figure 5C). In GFP-LC3–transfected HepG2 cells, cytoplasm LC3 fluorescent puncta numbers also declined after 4p treatments. These results indicated that the 4p addition suppressed autophagy in HepG2 cells. The in vivo antitumor capacities of 4p were determined on a HepG2 subcutaneous xenograft model. The 4p intraperitoneal injections were set to 15 mg/kg and 60 mg/kg, according to in vitro results. We observed that at a high dosage, the treatment group presented superior tumor growth inhibition (TGI) (63%), compared to the low-dosage group (39%) (Figure 6A). Moreover, mean tumor weights in both high- and low-dosage groups notably declined compared to the control (p < 0.01) without significant changes in body weights (Figures 6B,C). The potential 4p molecular mechanisms were further validated using immunofluorescence and IHC analyses of tissue sections stained by antibodies against TUNEL, Ki-67, RalA, LC3-II, and SQSTM1. In the 4p therapy groups, we found that a high 4p dosage group induced declined suppression levels of Ki-67 positive cells (p < 0.01), induced increased TUNEL and SQSTM1 levels, and decreased LC3-II expression (p < 0.01). No clear changes in total RalA levels were detected (Figure 6D). Therefore, the in vivo results were consistent with in vitro experiments.
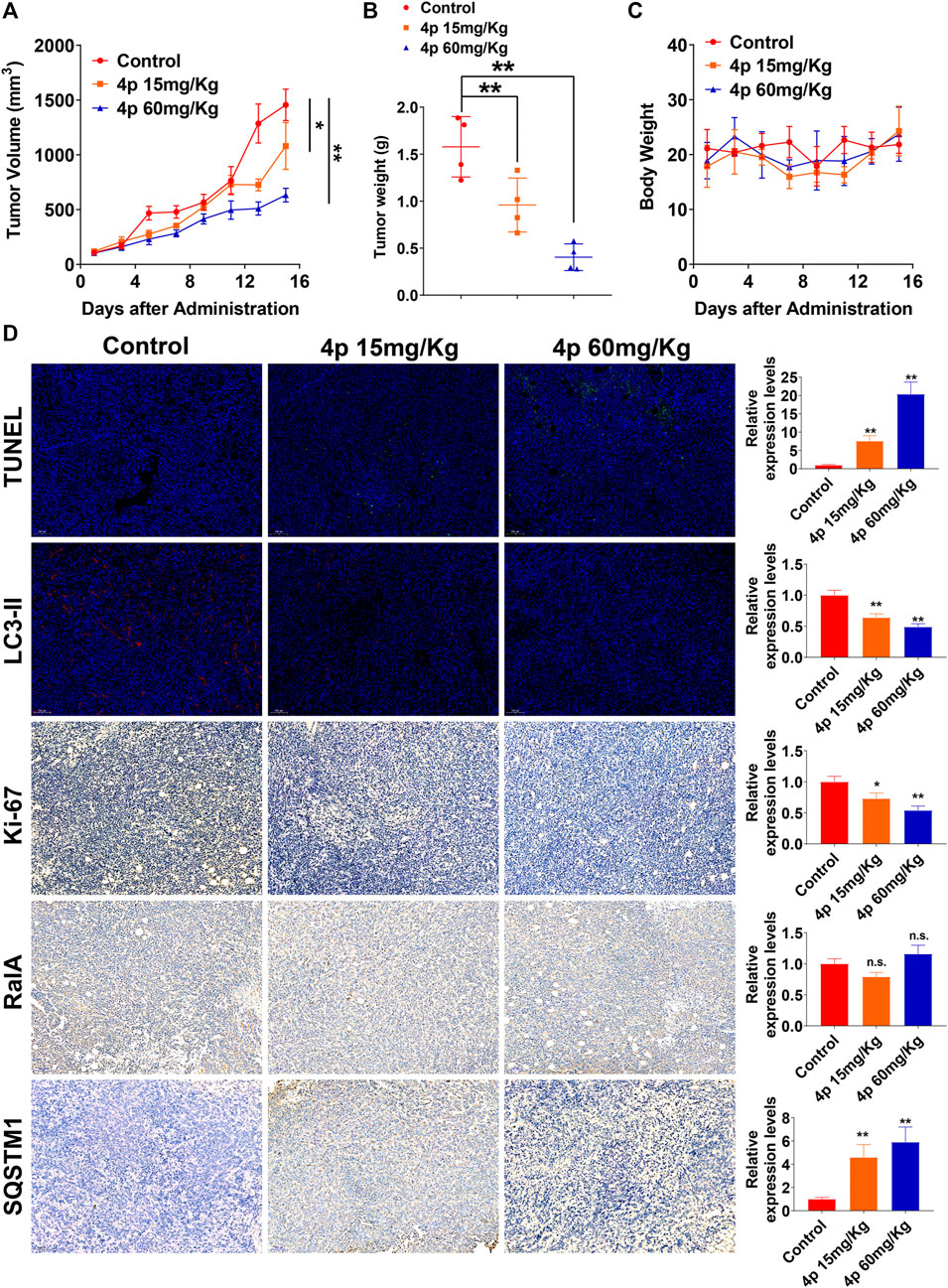
FIGURE 6. Compound 4p inhibited tumor growth in a HepG2 xenograft model. (A) Tumor volume changes were determined every 2 days after drug administration; (B) Tumor weight in each mouse in (A, C) The body weight of mice in each group; (D) Immunofluorescence and IHC images and analysis of tissue sections stained by TUNEL, Ki-67, RalA, LC3-II, and SQSTM1 in each group, scale bar: 100 μm.
Conclusion
Overall, we reported design, synthesis, and biologically based evaluation details of 6-sulfonamide-pyrano[2,3-c]-pyrazole as a novel RalA inhibitor against HCC, both in vitro and in vivo. Compound 4p was the most effective compound tested. It significantly inhibited cell proliferation and suppressed autophagy in HepG2 cells. These results can be a solid base for further exploration and development of novel RalA inhibitor candidates.
Experimental
General Information
Reactions were monitored using TLC purchased from a commercial supplier. Melting points were determined using a Reichert Thermovar apparatus. Proton nuclear magnetic resonance (1H NMR, 400 MHz) spectra and carbon nuclear magnetic resonance (13C NMR, 100 MHz) spectra were recorded on a Bruker Avance III NMR spectrometer. TMS (tetramethylsilane) was used as the internal standard and chloroform-d or DMSO-d6 was used to dissolve samples. Chemical shifts (d) were marked in ppm. ESI-HRMS spectrum data were collected using a Waters TOF-MS instrument (Waters, Milford, MA, United States). Nitrogen was used as the nebulizing gas, desolvation gas, and cone curtain gas. Chemicals received from commercial sources were used without further purification. Column chromatography was performed on silica gel (400–500 mesh) eluting with ethyl acetate and petroleum ether. TLC was performed on glass-backed silica plates. UV light and I2 were used to visualize products.
Reagents, Antibodies, and Cell Cultures
The antibodies recognizing LC3, p62/SQSTM1, RalA, RalB, and GAPDH were purchased from Proteintech (Wuhan, China). HCC cell lines, including HepG2, Hep3B, Huh-7, and SMMC-7721, were obtained from the Chinese Center for Type Culture Collection (Wuhan, China) and cultured in DMEM (Dulbecco’s modified Eagle’s medium) with 10% FBS (fetal bovine serum) and streptomycin. Cytotoxicity assays were performed by using the MTT method as previously described.
Biochemical and In Vitro Bioassays
The in vitro RalA/B bioactivity assays were performed following methods outlined in previous reports. (Yan et al., 2016; Zhang et al., 2018; Walsh et al., 2019; Bum-Erdene et al., 2020). In brief, the lysates of the test compound or vehicle-treated cells were cleared using cold centrifugation, and supernatants were collected. An RalBP1 solution was added to 96-well plates and incubated for 1–2 h, and then the plates were washed thrice using ELISA buffer. Next, cellular lysates were added, and samples were incubated overnight at 4°C. Then, ice-cold mouse anti-FLAG antibody was added, and samples were incubated for one more hour. After subsequent washing with ELISA buffer thrice, the HRP-conjugated anti-mouse antibody was added, and samples were incubated for another hour. Then, the HRP substrate was added. Antigen–antibody reactions were quenched by the addition of sulfuric acid (2 mol/L). Remaining RalA/B was detected by the OD450 values which were collected by using a plate reader. Cell proliferation assays, colony formation assays, apoptosis assays, GST pulldown, and Western blotting were used following methods outlined in our previous reports. (Zhou et al., 2015; Zhang et al., 2016; Yang et al., 2017; He et al., 2019). Autophagy levels and protein immunoblotting were used following the methods mentioned in our previous reports (Pan et al., 2018; Wang et al., 2018; Hao et al., 2019; Wang et al., 2019; Peng et al., 2020). Detailed experimental procedures are described in supporting materials.
Xenograft Models and In Vivo Evaluation
Female Balb/c nude mice aged 6–8 weeks were purchased from Beijing Huafukang Co. Ltd. (Beijing, China). Animal-based experiments complied with the guidelines of the Animal Ethics Committee (Sichuan University), and all aspects were approved by the Animal Ethics Committee of West China Hospital of Sichuan University (China). In brief, HepG2 cells were injected subcutaneously in the dorsal flank of Balb/c nude mice (5*105 cells per mice). Tumor volumes were recorded every 2 days post cancer cell injection. After the therapeutic endpoint, all mice were euthanized, and tumor tissues were stripped, fixed in formalin, embedded in paraffin, and sectioned. The IHC and immunofluorescent staining methods were performed following methods as per our previous research, (Zhang et al., 2019; Pan et al., 2020; Pang et al., 2020; Zhang et al., 2020), and detailed experimental procedures are also described in supporting materials.
General Procedure of Method for the Synthesis of 4a-4ag
To achieve the desired compound 2-benzylidenemalononitrile (1a), the following steps were completed. First, a mixture of benzaldehyde (10.0 mmol, 1.06 g), malononitrile (10.0 mmol, 0.66 g), sodium acetate (5.0 mmol, 0.41 g), and acetic acid (10 ml) was stirred overnight at room temperature. TLC was used to monitor whether or not the reaction was complete. When the basic reaction of raw materials was complete, ethyl acetate (30 ml) was added after the stirring was stopped, and glacial acetic acid was neutralized by NaHCO3 until pH values up to 7.0 were observed. The organic layer was separated and concentrated, and the crude product was purified using silica gel column chromatography using a mixed solvent of petroleum ether/ethylacetate (4:1) to give compound 1a.
To achieve the desired compound 2-benzylidene-malononitrile (2b), the following steps were completed. First, a mixture of 4-chlorophenylhydrazine salt (20 mmol, 3.6 g) and ethyl acetoacetate (30 mmol, 3.8 ml) was dissolved in 30 ml of glacial acetic acid and refluxed for 4 h. TLC was used to monitor whether or the reaction was complete. When the basic reaction of raw materials was complete, ethyl acetate (30 ml) was added on when the stirring was stopped. Then, glacial acetic acid was neutralized by NaHCO3 to a pH value = 7. The organic layer was separated and concentrated, and the crude product was purified using silica gel column chromatography using a mixed solvent of petroleum ether/ethylacetate (6:1) to give compound 2b.
6-Amino-3-methyl-1,4-diphenyl-1,4-dihydropyrano[2,3c]-pyrazole-5-carbonitrile (3a):A mixture of 1a (5 mmol, 0.77 g), 2a (5 mmol, 1.04 g) and morpholine (2.5 mmol, 0.22 g) in 10 ml methanol was stirred at 40°C for 30 min. The reaction mixture was filtered and washed with a small amount of ice-cold methanol to give the crude product, and then the crude product was purified using silica gel column chromatography using a mixed solvent of petroleum ether/ethylacetate (5:1) to give the compound 3a. Yield: 90%, white power solid, m. p. 170–172°C. 1H NMR (400 MHz, CDCl3) δ 7.65 (dt, J = 7.3, 1.4 Hz, 2H), 7.46 (tt, J = 7.5, 1.6 Hz, 2H), 7.39–7.30 (m, 3H), 7.30–7.24 (m, 3H), 4.67 (s, 2H), 4.66 (s, 1H), and 1.89 (s, 3H) ppm.
6-Amino-3-methyl-1-phenyl-4-(p-tolyl)-1,4-dihydropyrano-[2,3-c]-pyrazole-5-carbonitrile (3b): Synthesized with 1b and 2a according to the general procedure of 3a. Yield: 91%, white power solid, m. p. 169–170°C. 1H NMR (400 MHz, CDCl3) δ 7.68–7.63 (m, 2H), 7.45 (td, J = 8.3, 1.7 Hz, 2H), 7.30 (tt, J = 7.4, 1.6 Hz, 1H), 7.16–7.11 (m, 4H), 4.66 (s, 2H), 4.62 (s, 1H), 2.34 (s, 3H), 1.90 (s, 3H) ppm.
6-Amino-4-(3-chlorophenyl)-3-methyl-1-phenyl-1,4-dihydropyrano[2,3-c]-pyrazole-5-carbonitrile (3c): Synthesized with 1c and 2a according to the general procedure of 3a. Yield: 88%, white power solid, m. p. 155–157°C. 1H NMR (400 MHz, DMSO-d6) δ 7.80–7.78 (m, 2H), 7.49(tt, J = 8.6, 1.9 Hz, 2H), 7.41–7.37 (m, 3H), 7.34–7.30 (m, 3H)), 7.27–7.24 (m, 3H), 4.75 (s, 1H), 1.81 (s, 3H) ppm.
6-Amino-4-(4-bromophenyl)-3-methyl-1-phenyl-1,4-dihydropyrano[2,3-c]-pyrazole-5-carbonitrile (3d): Synthesized with 1d and 2a according to the general procedure of 3a. Yield: 90%, white power solid, m. p. 181–183°C. 1H NMR (400 MHz, CDCl3) δ 7.67–7.62 (m, 2H), 7.51–7.42 (m, 4H), 7.32 (t, J = 7.4 Hz, 1H), 7.14 (dt, J = 8.4, 2.6 Hz, 2H), 4.72 (s, 2H), 4.64 (s, 1H), 1.89 (s, 3H) ppm.
6-Amino-4-(4-fluorophenyl)-3-methyl-1-phenyl-1,4-dihydropyrano[2,3-c]-pyrazole-5-carbonitrile (3e): Synthesized with 1e and 2a according to the general procedure of 3a. Yield: 83%, yellow power solid, m. p. 173–176°C. 1H NMR (400 MHz, DMSO-d6) δ 7.79 (d, J = 8.0 Hz, 2H), 7.49 (t, J = 7.8 Hz, 2H), 7.34–7.29 (m, 3H), 7.22–7.15 (m, 4H), 4.73 (s, 1H), 1.79 (s, 3H) ppm.
6-Amino-4-(3-bromophenyl)-3-methyl-1-phenyl-1,4-dihydropyrano[2,3-c]-pyrazole-5-carbonitrile (3f): Synthesized with 1f and 2a according to the general procedure of 3a. Yield: 87%, white power solid, m. p. 161–164°C. 1H NMR (400 MHz, CDCl3) δ 7.66–7.63 (m, 2H), 7.47 (tt, J = 6.7, 2.0 Hz, 2H), 7.42 (dt, J = 6.5, 2.1 Hz, 1H), 7.36–7.30 (m, 2H), 7.23–7.22 (m, 2H), 4.75 (s, 2H), 4.63 (s, 1H), 1.91 (s, 3H) ppm.
6-Amino-4-(3,4-dichlorophenyl)-3-methyl-1-phenyl-1,4-dihydropyrano[2,3-c]-pyrazole-5-carbonitrile (3g): Synthesized with 1g and 2a according to the general procedure of 3a. Yield: 85%, white power solid, m. p. 198–200°C. 1H NMR (400 MHz, CDCl3) δ 7.66–7.63 (m, 2H), 7.49–7.42 (m, 3H), 7.34 (dt, J = 7.4, 1.1 Hz, 1H), 7.32 (d, J = 2.1 Hz, 1H), 7.13 (dd, J = 8.2, 2.1 Hz, 1H), 4.77 (s, 2H), 4.64 (s, 1H), 1.92 (s, 3H) ppm.
6-Amino-4-(4-bromo-2-fluorophenyl)-3-methyl-1-phenyl-1,4-dihydropyrano[2,3-c]-pyrazole-5-carbonitrile (3h): Synthesized with 1h and 2a according to the general procedure of 3a. Yield: 85%, white power solid, m. p. 200–203°C. 1H NMR (400 MHz, DMSO-d6) δ 7.81–7.75 (m, 2H), 7.56 (dd, J = 10.1, 2.0 Hz, 1H), 7.53–7.47 (m, 2H), 7.42 (dd, J = 8.3, 1.9 Hz, 1H), 7.37–7.26 (m, 4H), 4.97 (s, 1H), 1.83 (s, 3H) ppm.
6-Amino-4-(4-methoxyphenyl)-3-methyl-1-phenyl-1,4-dihydropyrano[2,3-c]-pyrazole-5-carbonitrile (3i): Synthesized with 1i and 2a according to the general procedure of 3a. Yield: 90%, white power solid, m. p. 176–178°C. 1H NMR (400 MHz, DMSO-d6) δ 7.82–7.76 (m, 2H), 7.49 (tt, J = 8.6, 2.0 Hz, 2H), 7.31 (tt, J = 7.4, 1.1 Hz, 2H), 7.18–7.15 (m, 4H), 6.90 (dt, J = 8.6, 2.0 Hz, 1H), 4.63 (s, 1H), 3.75 (s, 3H), 1.79 (s, 3H) ppm.
6-Amino-4-(furan-2-yl)-3-methyl-1-phenyl-1,4-dihydropyrano[2,3-c]-pyrazole-5-carbonitrile (3j): Synthesized with 1j and 2a according to the general procedure of 3a. Yield: 60%, yellow power solid, m. p. 225.1–225.5°C. 1H NMR (400 MHz, CDCl3) δ 7.62 (d, J = 7.8 Hz, 2H), 7.45 (t, J = 7.9 Hz, 2H), 7.37 (d, J = 0.9 Hz, 1H), 7.31 (t, J = 7.4 Hz, 1H), 6.34 (dd, J = 3.0, 1.9 Hz, 1H), 6.23 (d, J = 3.2 Hz, 1H), 4.83 (s, 1H), 4.75 (s, 2H), 2.09 (s, 3H) ppm.
6-Amino-3-methyl-1-phenyl-4-(pyridin-3-yl)-1,4-dihydropyrano[2,3-c]-pyrazole-5-carbonitrile (3k): Synthesized with 1k and 2a according to the general procedure of 3a. Yield: 80%, white power solid, m. p. 188.7–189.1°C. 1H NMR (400 MHz, DMSO-d6) δ 8.55 (d, J = 2.0 Hz, 1H), 8.49 (dd, J = 4.7, 1.5 Hz, 1H), 7.79 (d, J = 7.7 Hz, 2H), 7.66 (dt, J = 7.9, 1.8 Hz, 1H), 7.50 (t, J = 8.0 Hz, 2H), 7.38 (dd, J = 7.8, 4.8 Hz, 1H), 7.33 (t, J = 7.4 Hz, 1H), 7.29 (s, J = 7.0 Hz, 2H), 4.79 (s, 1H), 1.79 (s, 3H) ppm.
6-Amino-3-methyl-1-phenyl-4-(pyridin-4-yl)-1,4-dihydropyrano[2,3-c]-pyrazole-5-carbonitrile (3l): Synthesized with 1l and 2a according to the general procedure of 3a. Yield: 80%, white power solid, m. p. 192.2–192.6°C. 1H NMR (400 MHz, DMSO-d6) δ 8.59 (dd, J = 4.7, 1.3 Hz, 2H), 7.83–7.77 (m, 2H), 7.52–7.48 (m, 2H), 7.40–7.31 (m, 5H), 4.79 (s, 1H), 1.82 (s, 3H) ppm.
6-Amino-3-methyl-1-phenyl-4-(thiophen-3-yl)-1,4-dihydropyrano[2,3-c]-pyrazole-5-carbonitrile (3m): Synthesized with 1m and 2a according to the general procedure of 3a. Yield: 85%, yellow power solid, m. p. 178.2–178.6°C. 1H NMR (400 MHz, DMSO-d6) δ 7.81–7.75 (m, 2H), 7.53–7.45 (m, 3H), 7.37 (dd, J = 2.9, 1.2 Hz, 1H), 7.31 (t, J = 7.4 Hz, 1H), 7.18 (s, 2H), 6.95 (dd, J = 5.0, 1.2 Hz, 1H), 4.82 (s, 1H), 1.86 (s, 3H) ppm.
6-Amino-3-methyl-1-phenyl-4-(4-(trifluoromethyl) phenyl)-1,4-dihydropyrano[2,3-c]-pyrazole-5-carbonitrile (3n): Synthesized with 1n and 2a according to the general procedure of 3a. Yield: 85%, white power solid, m. p. 162.9–163.5°C. 1H NMR (400 MHz, DMSO-d6) δ 7.79 (d, J = 7.8 Hz, 2H), 7.73 (d, J = 8.1 Hz, 2H), 7.50 (t, J = 8.3 Hz, 4H), 7.33 (t, J = 7.6 Hz, 3H), 4.85 (s, 1H), 1.79 (s, 3H) ppm.
6-Amino-1-(2-chlorophenyl)-4-(furan-2-yl)-3-methyl-1,4-dihydropyrano[2,3-c]-pyrazole-5-carbonitrile (3o): Synthesized with 1j and 2b according to the general procedure of 3a. Yield: 62%, yellow power solid, m. p. 86.8–87.2°C. 1H NMR (400 MHz, DMSO-d6) δ 7.70 (dd, J = 7.8, 1.5 Hz, 1H), 7.59 (td, J = 7.5, 2.2 Hz, 2H), 7.57–7.48 (m, 2H), 7.12 (s, 2H), 6.42 (dd, J = 3.1, 1.9 Hz, 1H), 6.27 (d, J = 3.1 Hz, 1H), 4.90 (s, 1H), 1.96 (s, 3H) ppm.
6-Amino-1-(2-chlorophenyl)-3-methyl-4-(pyridin-3-yl)-1,4-dihydropyrano[2,3-c]-pyrazole-5-carbonitrile (3p): Synthesized with 1k and 2b according to the general procedure of 3a. Yield: 63%, white power solid, m. p. 165.9–166.4°C. 1H NMR (400 MHz, DMSO-d6) δ 8.56–8.47 (m, 2H), 7.71 (dd, J = 7.7, 1.6 Hz, 1H), 7.68–7.61 (m, 2H), 7.59–7.51 (m, 2H), 7.41 (dd, J = 7.8, 4.8 Hz, 1H), 7.15 (s, 2H), 4.80 (s, 1H), 1.78 (s, 3H) ppm.
6-Amino-1-(2-chlorophenyl)-3-methyl-4-(pyridin-4-yl)-1,4-dihydropyrano[2,3-c]-pyrazole-5-carbonitrile (3q): Synthesized with 1l and 2b according to the general procedure of 3a. Yield: 61%, white power solid, m. p. 200.1–200.7°C. 1H NMR (400 MHz, DMSO-d6) δ 8.57 (dd, J = 4.4, 1.5 Hz, 2H), 7.71 (dd, J = 7.8, 1.6 Hz, 1H), 7.66 (dd, J = 7.3, 2.2 Hz, 1H), 7.59–7.51 (m, 2H), 7.28 (dd, J = 4.5, 1.5 Hz, 2H), 7.19 (s, 2H), 4.77 (s, 1H), 1.80 (s, 3H) ppm.
6-Amino-1-(2-chlorophenyl)-3-methyl-4-(thiophen-3-yl)-1,4-dihydropyrano[2,3-c]-pyrazole-5-carbonitrile (3r): Synthesized with 1m and 2b according to the general procedure of 3a. Yield: 65%, yellow power solid, m. p. 167.0–167.5°C. 1H NMR (400 MHz, DMSO-d6) δ 7.70 (dd, J = 7.7, 1.7 Hz, 1H), 7.62 (dd, J = 7.2, 2.2 Hz, 1H), 7.58–7.48 (m, 3H), 7.35 (dd, J = 2.8, 1.0 Hz, 1H), 7.01 (s, 2H), 6.91 (dd, J = 5.0, 1.1 Hz, 1H), 4.83 (s, 1H), 1.85 (s, 3H) ppm.
6-Amino-1-(2-chlorophenyl)-4-(3-methoxyphenyl)-3-methyl-1,4-dihydropyrano[2,3-c]-pyrazole-5-carbonitrile (3s): Synthesized with 1o and 2b according to the general procedure of 3a. Yield: 80%, white power solid, m. p. 170.7–171.2°C. 1H NMR (400 MHz, DMSO-d6) δ 11.49 (s, 1H), 7.64–7.62 (m, 1H), 7.57–7.42 (m, 3H), 7.28 (t, J = 7.9 Hz, 2H), 7.16 (d, J = 7.2 Hz, 1H), 6.87 (dd, J = 8.2, 2.2 Hz, 1H), 5.85 (d, J = 9.2 Hz, 1H), 4.66 (d, J = 10.9 Hz, 1H), 3.74 (s, 3H), 2.14 (s, 3H) ppm.
6-Amino-1-(4-chlorophenyl)-3-methyl-4-phenyl-1,4-dihydropyrano[2,3-c]-pyrazole-5-carbonitrile (3t): Synthesized with 1a and 2c according to the general procedure of 3a. Yield: 80%, white power solid, m. p. 165.9–166.4°C. 1H NMR (400 MHz, DMSO-d6) δ 7.83 (dt, J = 8.9, 3.0 Hz, 2H), 7.53 (dt, J = 8.9, 3.2 Hz, 2H), 7.38–7.32 (m, 2H), 7.29–7.19 (m, 5H), 4.68 (s, 1H), 1.78 (s, 3H) ppm.
6-Amino-1-(2-chlorophenyl)-3-methyl-4-phenyl-1,4-dihydropyrano[2,3-c]-pyrazole-5-carbonitrile (3u): Synthesized with 1a and 2b according to the general procedure of 3a. Yield: 75%, white power solid, m. p. 188.9–189.2°C. 1H NMR (400 MHz, DMSO-d6) δ 11.64 (s, 1H), 7.67–7.56 (m, 3H), 7.51–7.46 (m, 3H) 7.37 (t, J = 7.4 Hz, 2H), 7.32–7.28 (m, 1H), 5.8 (d, J = 9.4 Hz, 1H), 4.70 (d, J = 10.2 Hz, 1H), 2.14 (s, 3H) ppm.
6-Amino-3-methyl-4-phenyl-1-(p-tolyl)-1,4-dihydropyrano-[2,3-c]-pyrazole-5-carbonitrile (3v): Synthesized with 1a and 2d according to the general procedure of 3a. Yield: 90%, white power solid, m. p. 167.0–167.5°C. 1H NMR (400 MHz, DMSO-d6) δ 7.65 (dt, J = 8.5, 2.8 Hz, 2H), 7.35 (tt, J = 7.3, 1.0 Hz, 2H), 7.30–7.23 (m, 5H), 7.17 (s, 2H), 4.67 (s, 1H), 2.35 (s, 3H), 1.77 (s, 3H) ppm.
6-Amino-1-(4-methoxyphenyl)-3-methyl-4-phenyl-1,4-dihydropyrano[2,3-c]-pyrazole-5-carbonitrile (3w): Synthesized with 1a and 2e according to the general procedure of 3a. Yield: 88%, white power solid, m. p. 186.2–187,3 °C. 1H NMR (400 MHz, DMSO-d6) δ 7.65 (dt, J = 8.5, 3.5 Hz, 2H), 7.38–7.32 (m, 2H), 7.29–7.23 (m, 3H), 7.14 (s, 2H), 7.06–7.01 (m, 2H), 4.67 (s, 1H), 3.80 (s, 3H), 1.77 (s, 3H) ppm.
6-Amino-1-(4-fluorophenyl)-3-methyl-4-phenyl-1,4-dihydropyrano[2,3-c]-pyrazole -5-carbonitrile (3x): Synthesized with 1a and 2f according to the general procedure of 3a. Yield: 80%, white power solid, m. p. 162.9–163.5°C. 1H NMR (400 MHz, DMSO-d6) δ 7.84–7.77 (m, 2H), 7.37–7.31 (m, 4H), 7.29–7.24 (m, 3H), 7.19 (s, 2H), 4.68 (s, 1H), 1.77 (s, 3H) ppm.
N-(5-cyano-3-methyl-1,4-diphenyl-1,4-dihydropyrano[2,3-c]-pyrazole-6-yl)-4-methylbenzenesulfonamide (4a): A mixture of 3a (5 mmol, 1.6 g), 4-methylbenzenesulfonyl chloride (5 mmol, 0.95 g), and N,N-dimethylpyridin-4-amine (DMAP) (5 mmol, 0.61 g) in 30 ml dichloromethane was stirred at room temperature overnight. TLC was used to monitor whether the reaction was complete. When the basic reaction of raw materials was complete, the stirring was stopped, and the reaction mixture was cooled to room temperature. The crude product was purified using silica gel column chromatography using a mixture solvent of petroleum ether/ethyl acetate (8:1) to give 4a. Yield: 66%, white power solid, m. p. 68.3–69.7°C. 1H NMR (400 MHz, CDCl3) δ 7.50–7.42 (m, 4H), 7.41–7.38 (m, 1H), 7.35 (dt, J = 8.4, 2.5 Hz, 2H), 7.24–7.18 (m, 3H), 7.17–7.13 (m, 2H), 7.04 (dt, J = 7.7, 0.8 Hz, 2H), 4.98 (d, J = 9.6 Hz, 1H), 4.85 (d, J = 9.6 Hz, 1H), 2.37 (s, 3H), 2.15 (s, 3H) ppm; 13C NMR (100 MHz, CDCl3) δ 147.7, 147.2, 140.4, 137.1, 136.3, 130.7 (2C), 129.9, 129.4 (2C), 129.4 (2C), 128.6, 128.5 (2C), 128.0, 127.9 (2C), 123.3 (2C), 114.0, 113.9, 108.3, 40.3, 27.3, 21.7, 14.9 ppm. HRMS (ESI): calculated for C27H22N4NaO3S+ [M + Na]+, 505.1305; found 505.1304.
N-(5-cyano-3-methyl-1-phenyl-4-(p-tolyl)-1,4-dihydropyrano[2,3-c]-pyrazol-6-yl)-4-methylbenzenesulfonamide (4b): Synthesized with 3b according to the general procedure of 4a. Yield: 70%, white power solid, m. p. 74.7–75.0°C. 1H NMR (400 MHz, CDCl3) δ 7.39–7.32 (m, 4H), 7.26–7.11 (m, 7H), 7.04 (d, J = 8.1 Hz, 2H), 4.96 (d, J = 9.7 Hz, 1H), 4.80 (d, J = 9.7 Hz, 1H), 2.37 (d, J = 1.7 Hz, 6H), 2.16 (s, 3H) ppm; 13C NMR (100 MHz, CDCl3) δ 147.7, 147.2, 140.4, 137.9, 137.1, 133.3, 130.7 (2C), 129.9 (2C), 129.9, 129.4 (2C), 128.5 (2C), 128.0, 127.7 (2C), 123.3 (2C), 114.0, 113.9, 108.4, 40.1, 27.3, 21.7, 21.1, 15.0 ppm. HRMS (ESI): calculated for C28H24N4NaO3S+ [M + Na]+, 519.1461; found 519.1462.
N-(4-(3-chlorophenyl)-5-cyano-3-methyl-1-phenyl-1,4-dihydropyrano[2,3-c]-pyrazol-6-yl)-4-methylbenzenesulfonamide (4c). Synthesized with 3c according to the general procedure of 4a. Yield: 68% white power solid. m. p. 68.4–69.0°C. 1H NMR (400 MHz, CDCl3) δ 7.45 (t, J = 1.6 Hz, 1H), 7.43–7.37 (m, 3H), 7.35 (dt, J = 8.4, 1.6 Hz, 2H), 7.25–7.12 (m, 5H), 7.05 (t, J = 7.8 Hz, 2H), 4.99 (d, J = 9.7 Hz, 1H), 4.81 (d, J = 9.7 Hz, 1H), 2.38 (s, 3H), 2.19 (s, 3H) ppm; 13C NMR (100 MHz, CDCl3) δ 148.0, 146.9, 139.9, 137.3, 137.1, 135.2, 130.4, 130.2, 129.9 (2C), 129.0, 128.8 (2C), 128.5 (2C), 128.2, 127.6, 125.9, 123.6 (2C), 112.0, 112.0, 107.0, 41.7, 27.1, 21.7, 14.3 ppm. HRMS (ESI): calculated for C27H21ClN4NaO3S+ [M + Na]+, 539.0915; found 539.0916.
N-(4-(4-bromophenyl)-5-cyano-3-methyl-1-phenyl-1,4-dihydropyrano[2,3-c]-pyrazol-6-yl)-4-methylbenzenesulfonamide (4d): Synthesized with 3d according to the general procedure of 4a. Yield: 70%, white power solid, m. p. 101.6–102.3°C. 1H NMR (400 MHz, CDCl3) δ 7.59 (dt, J = 8.5,2.8 Hz, 2H), 7.40–7.30 (m, 4H), 7.24–7.17 (m, 3H), 7.17–7.12 (m, 2H), 7.04 (dt, J = 7.8,1.8 Hz, 2H), 4.98 (d, J = 9.7 = Hz, 1H), 4.80 (d, J = 9.7 Hz, 1H), 2.37 (s, 3H), 2.17 (s, 3H) ppm; 13C NMR (100 MHz, CDCl3) δ 148.0, 146.9, 139.9, 137.1, 134.4, 132.4 (2C), 130.1, 129.9 (2C), 129.5 (2C), 128.8 (2C), 128.5 (2C), 127.6, 123.6 (2C), 122.9, 112.1, 112.1, 107.1, 41.7, 27.1, 21.8, 14.3 ppm. HRMS (ESI): calculated for C27H21BrN4NaO3S+ [M + Na]+, 583.0410; found 583.0410.
N-(5-cyano-4-(4-fluorophenyl)-3-methyl-1-phenyl-1,4-dihydropyrano[2,3-c]-pyrazol-6-yl)-4-methylbenzenesulfonamide (4e): Synthesized with 3e according to the general procedure of 4a. Yield: 70%, white power solid, m. p. 113.0–113.5°C. 1H NMR (400 MHz, CDCl3) δ 7.51–7.45 (m, 2H), 7.33 (dt, J = 8.4, 2.2 Hz, 2H), 7.24–7.18 (m, 3H), 7.17–7.11 (m, 4H), 7.05 (dt, J = 7.8, 0.8 Hz, 2H), 4.98 (d, J = 9.7 Hz, 1H), 4.82 (d, J = 9.7 Hz, 1H), 2.38 (s, 3H), 2.16 (s, 3H) ppm; 13C NMR (100 MHz, CDCl3) δ 162.1, 148.0, 146.9, 139.8, 137.1, 131.3, 130.2, 129.9 (2C), 129.7 (2C), 128.8 (2C), 128.5 (2C), 127.6, 123.7 (2C), 116.2 (2C), 112.2, 112.2, 107.4, 41.6, 27.4, 21.7, 14.3 ppm. HRMS (ESI): calculated for C27H21FN4NaO3S+ [M + Na]+, 523.1211; found 523.1216.
N-(4-(3-bromophenyl)-5-cyano-3-methyl-1-phenyl-1,4-dihydropyrano[2,3-c]-pyrazol-6-yl)-4-methylbenzenesulfonamide (4f): Synthesized with 3f according to the general procedure of 4a. Yield:75%, white power solid, m. p. 68.4–69.5°C. 1H NMR (400 MHz, CDCl3) δ 7.60 (t, J = 1.9 Hz, 1H), 7.54 (ddd, J = 8.0, 1.9, 1.0 Hz, 1H), 7.47 (ddd, J = 8.8, 1.9, 0.7 Hz, 1H), 7.38–7.30 (m, 3H), 7.24–7.18 (m, 3H), 7.18–7.12 (m, 2H), 7.05 (dt, J = 7.8, 0.8 Hz, 2H), 4.99 (d, J = 9.8 Hz, 1H), 4.80 (d, J = 9.8 Hz, 1H), 2.38 (s, 3H), 2.20 (s, 3H) ppm; 13C NMR (100 MHz, CDCl3) δ 148.0, 146.9, 139.9, 137.6, 137.1, 131.9, 131.1, 130.6, 130.1, 129.9 (2C), 128.8 (2C), 128.5 (2C), 127.6, 126.3, 123.6 (2C), 123.3, 112.0, 112.0, 107.0, 41.7, 27.1, 21.7, 14.3 ppm. HRMS (ESI): calculated for C27H21BrN4NaO3S+ [M + Na]+, 583.0410; found 583.0410.
N-(5-cyano-4-(3,4-dichlorophenyl)-3-methyl-1-phenyl-1,4-dihydropyrano[2,3-c]-pyrazol-6-yl)-4-methylbenzenesulfonamide (4g): Synthesized with 3g according to the general procedure of 4a. Yield: 69%, white power solid, m. p. 100.9–101.3°C. 1H NMR (400 MHz, CDCl3) δ 7.57 (d, J = 2.3 Hz, 1H), 7.55 (d, J = 8.4 Hz, 1H), 7.37 (dd, J = 8.4, 2.3 Hz, 1H), 7.33 (dt, J = 8.4, 2.3 Hz, 2H), 7.25–7.18 (m, 3H), 7.17–7.13 (m, 2H), 7.05 (dt, J = 7.7, 0.8 Hz, 2H), 4.99 (d, J = 9.9 Hz, 1H), 4.78 (d, J = 9.9 Hz, 1H), 2.38 (s, 3H), 2.21 (s, 3H) ppm; 13C NMR (100 MHz, CDCl3) δ 147.9, 147.0, 139.9, 137.0, 135.5, 133.5, 133.2, 131.1, 130.1, 130.1, 129.9 (2C), 128.9 (2C), 128.5 (2C), 127.7, 127.1, 123.7 (2C), 111.9, 111.8, 106.7, 41.4, 27.1, 21.8, 14.3 ppm. HRMS (ESI): calculated for C27H20Cl2N4NaO3S+ [M + Na]+, 573.0525; found 573.0523.
N-(4-(4-bromo-2-fluorophenyl)-5-cyano-3-methyl-1-phen-yl-1,4-dihydropyrano[2,3-c]-pyrazol-6-yl)-4-methylbenzenesulfonamide (4h): Synthesized with 3h according to the general procedure of 4a. Yield: 66%, white power solid, m. p. 73.6–77.4°C. 1H NMR (400 MHz, CDCl3) δ 7.52 (t, J = 8.1 Hz, 1H), 7.45 (dd, J = 8.5, 1.9 Hz, 1H), 7.35 (dd, J = 9.8, 1.9 Hz, 1H), 7.30 (dt, J = 8.4, 2.2 Hz, 2H), 7.22–7.14 (m, 3H), 7.12–7.06 (m, 2H), 7.02 (dt, J = 8.5, 2.0 Hz, 2H), 5.24 (d, J = 10.9 Hz, 1H), 5.03 (d, J = 10.9 Hz, 1H), 2.37 (s, 3H), 2.32 (s, 3H) ppm; 13C NMR (100 MHz, CDCl3) δ 160.0, 148.4, 146.9, 139.9, 137.0, 130.0, 129.9 (2C), 129.5, 128.8 (2C), 128.5 (2C), 128.2, 127.5, 123.6 (2C), 123.3, 122.0, 120.0, 111.9, 111.9, 105.9, 35.5, 26.4, 21.7, 13.8 ppm. HRMS (ESI): calculated for C27H20BrFN4NaO3S+ [M + Na]+, 601.0316; found 601.0311.
N-(5-cyano-4-(4-methoxyphenyl)-3-methyl-1-phenyl-1,4-dihydropyrano[2,3-c]-pyrazol-6-yl)-4-methylbenzenesulfonamide (4i): Synthesized with 3i according to the general procedure of 4a. Yield: 80%, white power solid, m. p. 145.2–146.0°C. 1H NMR (400 MHz, CDCl3) δ 7.40 (dt, J = 8.6, 3.4 Hz, 2H), 7.34 (dt, J = 8.4, 2.2 Hz, 2H), 7.24–7.18 (m, 3H), 7.17–7.13 (m, 2H), 7.04 (dt, J = 7.7, 1.6 Hz, 2H), 6.96 (dt, J = 8.8, 3.2 Hz, 2H), 4.95 (d, J = 9.7 Hz, 1H), 4.78 (d, J = 9.7 Hz, 1H), 3.83 (s, 3H), 2.37 (s, 3H), 2.16 (s, 3H) ppm; 13C NMR (100 MHz, CDCl3) δ 159.4, 147.8, 147.2, 140.3, 137.1, 130.8 (2C), 129.9, 129.5 (2C), 129.2 (2C), 128.6 (2C), 128.2, 128.1, 123.3 (2C), 114.80 (2C), 114.1, 114.0, 108.6, 55.7, 39.8, 27.6, 21.7, 15.0 ppm. HRMS (ESI): calculated for C28H24N4NaO4S+ [M + Na]+, 535.1410; found 535.1417.
N-(5-cyano-4-(furan-2-yl)-3-methyl-1-phenyl-1,4-dihydropyrano[2,3-c]-pyrazol-6-yl)-4-methylbenzenesulfonamide (4j): Synthesized with 3j according to the general procedure of 4a. Yield: 55%, yellow power solid, m. p. 153.8–154.3°C. 1H NMR (400 MHz, CDCl3) δ 7.49 (d, J = 1.4 Hz, 1H), 7.35 (d, J = 8.4 Hz, 2H), 7.26 (s, 1H), 7.24–7.18 (m, 3H), 7.4–7.12 (m, 2H), 7.01 (d, J = 8.2 Hz, 2H), 6.52 (d, J = 3.3 Hz, 1H), 6.45 (dd, J = 3.3, 1.9 Hz, 1H), 4.98 (d, J = 8.9 Hz, 1H), 4.84 (d, J = 8.9 Hz, 1H), 2.29 (s, 3H) ppm; 13C NMR (100 MHz, DMSO-d6) δ 149.1, 147.7, 147.5, 144.1, 140.5, 136.9, 130.7 (2C), 129.7, 129.4 (2C), 128.5 (2C), 128.0, 123.2 (2C), 113.7, 113.4, 111.4, 109.3, 106.0, 35.2, 27.2, 21.7, 14.4 ppm. HRMS (ESI): calculated for C25H20N4NaO4S+ [M + Na]+, 495.1097; found 495.1094.
N-(5-cyano-3-methyl-1-phenyl-4-(pyridin-3-yl)-1,4-dihydropyrano[2,3-c]-pyrazol-6-yl)-4-methylbenzenesulfonamide (4k): Synthesized with 3k according to the general procedure of 4a. Yield: 60%, white power solid, m. p. 136.3–137.0°C. 1H NMR (400 MHz, DMSO-d6) δ 7.98 (s, 1H), 7.87 (dd, J = 8.7, 1.0 Hz, 1H), 7.58 (d, J = 8.2 Hz, 2H), 7.52–7.38 (m, 5H), 7.34 (d, J = 8.0 Hz, 3H), 7.12 (d, J = 7.8 Hz, 3H), 2.36 (s, 3H), 2.29 (s, 3H) ppm; 13C NMR (100 MHz, DMSO-d6) δ 161.6, 151.9, 148.9, 147.7, 146.0, 145.0, 144.3, 142.4, 141.8, 138.2, 129.9 (2C), 129.4, 128.6 (2C), 126.0 (2C), 125.3 (2C), 123.4, 119.8, 118.9, 62.6, 21.4, 21.2, 13.5 ppm. HRMS (ESI): calculated for C26H21N5NaO3S+ [M + Na]+, 506.1257; found 506.1272.
N-(5-cyano-3-methyl-1-phenyl-4-(pyridin-4-yl)-1,4-dihydropyrano[2,3-c]-pyrazol-6-yl)-4-methylbenzenesulfonamide (4l): Synthesized with 3l according to the general procedure of 4a. Yield: 60%, white power solid, m. p. 100.5–101.1°C. 1H NMR (400 MHz, DMSO-d6) δ 8.70 (dd, J = 4.7, 1.4 Hz, 2H), 7.48 (d, J = 5.8 Hz, 2H), 7.43 (d, J = 8.4 Hz, 2H), 7.36–7.28 (m, 3H), 7.18 (d, J = 8.1 Hz, 2H), 7.14–7.07 (m, 2H), 5.95 (d, J = 8.9 Hz, 1H), 5.09 (d, J = 8.9 Hz, 1H), 2.34 (s, 3H), 2.03 (s, 3H) ppm; 13C NMR (100 MHz, DMSO-d6) δ 150.3, 147.7, 147.4, 145.7, 140.9, 136.9, 130.7 (2C), 129.7, 129.5 (2C), 128.6 (2C), 128.1, 123.3 (2C), 123.2 (2C), 113.7, 113.6, 107.0, 100.0, 39.5, 27.0, 21.7, 14.9 ppm. HRMS (ESI): calculated for C26H21N5NaO3S+ [M + Na]+, 506.1257; found 506.1253.
N-(5-cyano-3-methyl-1-phenyl-4-(thiophen-3-yl)-1,4-dihydropyrano[2,3-c]-pyrazol-6-yl)-4-methylbenzenesulfonamide (4m): Synthesized with 3m according to the general procedure of 4a. Yield: 65%, yellow power solid, m. p. 67.8–68.4°C. 1H NMR (400 MHz, DMSO-d6) δ 7.68 (dd, J = 5.0, 2.9 Hz, 1H), 7.61–7.57 (m, 1H), 7.43 (d, J = 8.4 Hz, 2H), 7.36–7.26 (m, 3H), 7.18 (d, J = 8.1 Hz, 2H), 7.15–7.05 (m, 3H), 5.82 (d, J = 8.9 Hz, 1H), 4.94 (d, J = 8.9 Hz, 1H), 2.34 (s, 3H), 2.00 (s, 3H) ppm; 13C NMR (100 MHz, DMSO-d6) δ 147.7, 147.4, 140.4, 137.0, 136.9, 130.7 (2C), 129.8, 129.4 (2C), 128.5 (2C), 128.1, 128.0, 127.8, 123.5, 123.2 (2C), 114.0, 113.8, 108.0, 36.8, 28.0, 21.7, 14.6 ppm. HRMS (ESI): calculated for C25H20N4NaO3S2+ [M + Na]+, 511.0869; found 511.0868.
N-(5-cyano-3-methyl-1-phenyl-4-(4-(trifluoromethyl)phenyl)-1,4-dihydropyrano[2,3-c]-pyrazol-6-yl)-4-methylbenzenesulfonamide (4n): Synthesized with 3n according to the general procedure of 4a. Yield: 73%, white power solid, m. p. 109.3–110.2°C. 1H NMR (400 MHz, DMSO-d6) δ 7.89 (d, J = 8.3 Hz, 2H), 7.70 (d, J = 8.2 Hz, 2H), 7.44 (d, J = 8.3 Hz, 2H), 7.36–7.30 (m, 3H), 7.18 (d, J = 8.2 Hz, 2H), 7.11 (dd, J = 7.6, 1.9 Hz, 2H), 5.97 (d, J = 9.3 Hz, 1H), 5.12 (d, J = 9.3 Hz, 1H), 2.34 (s, 3H), 2.07 (s, 3H) ppm; 13C NMR (100 MHz, DMSO-d6) δ 148.0, 147.1, 140.0, 139.4, 137.7, 137.0, 131.0, 130.0 (2C), 129.0 (2C), 128.5 (2C), 128.5 (2C), 127.7, 126.3 (2C), 123.8, 123.7 (2C), 112.1, 112.1, 107.0, 41.9, 27.1, 21.9, 14.4 ppm. HRMS (ESI): calculated for C28H21F3N4NaO3S+ [M + Na]+, 573.1179; found 573.1176.
N-(5-cyano-3-methyl-1-phenyl-4-(4-(trifluoromethyl)phenyl)-1,4-dihydropyrano[2,3-c]-pyrazol-6-yl)thiophene-2-sulfonamide (4o): Synthesized with 3n and 4-thiophene-2-sulfonyl chloride according to the general procedure of 4a. Yield: 54%, white power solid, m. p. 55.9–56.7°C. 1H NMR (400 MHz, DMSO-d6) δ 8.12 (dd, J = 5.0, 1.3 Hz, 1H), 7.89 (d, J = 8.3 Hz, 2H), 7.70 (d, J = 8.2 Hz, 2H), 7.55 (dd, J = 3.9, 1.3 Hz, 1H), 7.41–7.34 (m, 3H), 7.24–7.18 (m, 2H), 7.04 (dd, J = 4.9, 4.0 Hz, 1H), 5.98 (d, J = 9.2 Hz, 1H), 5.17 (d, J = 9.2 Hz, 1H), 2.07 (s, 3H) ppm; 13C NMR (100 MHz, DMSO-d6) δ 148.1, 139.9, 139.2, 137.3, 137.0, 136.9, 131.9, 131.0, 129.2 (2C), 128.4 (2C), 128.2, 128.0, 126.3 (2C), 123.8, 123.6 (2C), 112.0, 111.9, 107.1, 41.7, 27.0, 14.5 ppm. HRMS (ESI): calculated for C25H17F3N4NaO3S2+ [M + Na]+, 565.0586; found 565.0572.
N-(5-cyano-3-methyl-1-phenyl-4-(4-(trifluoromethyl) phenyl)-1,4-dihydropyrano[2,3-c]-pyrazol-6-yl)-4-methoxybenzenesulfonamide (4p): Synthesized with 3n and 4-methoxybenzenesulfonyl chloride according to the general procedure of 4a. Yield: 76%, white power solid, m. p. 72.0–72.7°C. 1H NMR (400 MHz, DMSO-d6) δ 7.89 (d, J = 8.3 Hz, 2H), 7.71 (d, J = 8.2 Hz, 2H), 7.47 (d, J = 9.0 Hz, 2H), 7.36–7.28 (m, 3H), 7.14 (dd, J = 7.8, 1.8 Hz, 2H), 6.86 (d, J = 9.0 Hz, 2H), 5.98 (d, J = 9.3 Hz, 1H), 5.15 (d, J = 9.3 Hz, 1H), 3.83 (s, 3H), 2.07 (s, 3H) ppm; 13C NMR (100 MHz, DMSO-d6) δ 165.1, 148.0, 140.2, 139.4, 137.1, 137.1, 131.0, 130.9 (2C), 129.0 (2C), 128.5 (2C), 127.8, 126.3 (2C), 123.8, 123.6 (2C), 114.6 (2C), 112.2, 112.1, 107.0, 55.9, 41.9, 27.1, 14.4 ppm. HRMS (ESI): calculated for C28H21F3N4NaO4S+ [M + Na]+, 589.1128; found 589.1129.
N-(5-cyano-3-methyl-1-phenyl-4-(4-(trifluoromethyl)phenyl)-1,4-dihydropyrano[2,3-c]-pyrazol-6-yl)-4-(trifluoromethyl)-benzenesulfonamide (4q): Synthesized with 3n and 4-(trifluoromethyl) benzenesulfonyl chloride according to the general procedure of 4a. Yield: 62%, yellow power solid, m. p. 153.7–154.1°C. 1H NMR (400 MHz, DMSO-d6) δ 7.86 (d, J = 8.3 Hz, 2H), 7.83–7.76 (m, 5H), 7.73–7.70 (m, 4H), 7.48–7.44 (m, 2H), 6.00 (d, J = 11.3 Hz, 1H), 4.94 (d, J = 11.3 Hz, 1H), 2.24 (s, 3H) ppm; 13C NMR (100 MHz, DMSO-d6) δ 148.2, 139.7, 139.1, 136.8, 136.7, 136.7, 131.1, 129.3 (2C), 129.1 (2C), 128.3 (2C), 128.2, 126.4 (4C), 123.8, 123.6 (2C), 120.9, 111.9, 111.9, 107.3, 41.8, 27.1, 14.5 ppm. HRMS (ESI): calculated for C28H18F6N4NaO3S+ [M + Na]+, 627.0896; found 627.0880.
N-(5-cyano-3-methyl-1-phenyl-4-(4-(trifluoromethyl)phenyl)-1,4-dihydropyrano[2,3-c]-pyrazol-6-yl)-2,4-difluorobenzenesulfonamide (4r): Synthesized with 3n and 2,4-difluorobenzenesulfonyl chloride according to the general procedure of 4a. Yield: 60%, white power solid, m. p. 65.8–66.5°C. 1H NMR (400 MHz, DMSO-d6) δ 7.89 (d, J = 8.3 Hz, 2H), 7.71 (d, J = 8.3 Hz, 2H), 7.67–7.61 (m, 1H), 7.37–7.27 (m, 4H), 7.20–7.11 (m, 3H), 5.96 (d, J = 9.0 Hz, 1H), 5.21 (d, J = 9.0 Hz, 1H), 2.05 (s, 3H) ppm; 13C NMR (100 MHz, DMSO-d6) δ 167.7, 160.5, 148.3, 139.5, 139.1, 136.8, 133.0, 132.9, 130.0, 129.2 (2C), 128.3 (2C), 128.2, 126.4 (2C), 123.8 (1C), 123.6 (2C), 112.6, 112.0, 111.8, 107.4, 106.4, 41.7, 27.0, 14.4 ppm. HRMS (ESI): calculated for C27H17F5N4NaO3S+ [M + Na]+, 595.0834; found 595.0822.
N-(1-(2-chlorophenyl)-5-cyano-4-(furan-2-yl)-3-methyl-1,4-dihydropyrano[2,3-c]-pyrazol-6-yl)-4-methylbenzenesulfonamide (4s): Synthesized with 3o according to the general procedure of 4a. Yield: 50%, yellow power solid, m. p. 62.5–63.9°C. 1H NMR (400 MHz, DMSO-d6) δ 7.77 (d, J = 1.2 Hz, 1H), 7.50 (dd, J = 15.6, 7.7, 1.4 Hz, 2H), 7.45 (dt, J = 8.4, 1.5 Hz, 2H), 7.34 (td, J = 7.7, 1.5 Hz, 1H), 7.30 (d, J = 8.2 Hz, 2H), 7.08 (d, J = 7.1 Hz, 1H), 6.55 (dd, J = 3.2, 1.9 Hz, 1H), 6.48 (d, J = 3.2 Hz, 1H), 5.69 (d, J = 9.1 Hz, 1H), 4.88 (d, J = 9.1 Hz, 1H), 2.40 (s, 3H), 2.12 (s, 3H) ppm; 13C NMR (100 MHz, DMSO-d6) δ 149.0, 148.1, 147.6, 144.0, 141.8, 134.3, 131.4, 131.0 (2C), 130.5, 130.5, 130.4, 129.7, 128.4, 128.3 (2C), 113.5, 113.3, 111.4, 109.3, 105.2, 35.1, 27.2, 21.7, 14.3 ppm. HRMS (ESI): calculated for C25H19ClN4NaO4S+ [M + Na]+, 529.0708; found 529.0703.
N-(1-(2-chlorophenyl)-5-cyano-3-methyl-4-(pyridin-3-yl)-1,4-dihydropyrano[2,3-c]-pyrazol-6-yl)-4-methylbenzenesulfonamide (4t): Synthesized with 3p according to the general procedure of 4a. Yield: 51%, white power solid, m. p. 195.8–196.3°C. 1H NMR (400 MHz, DMSO-d6) δ 8.66 (d, J = 1.6 Hz, 1H), 8.62 (d, J = 4.6 Hz, 1H), 7.87 (d, J = 8.1 Hz, 1H), 7.54 (dd, J = 7.9, 4.8 Hz, 1H), 7.51–7.42 (m, 4H), 7.37–7.32 (m, 1H), 7.29 (d, J = 8.3 Hz, 2H), 7.14 (d, J = 7.7 Hz, 1H), 5.89 (d, J = 9.8 Hz, 1H), 5.05 (d, J = 9.8 Hz, 1H), 2.39 (s, 3H), 2.10 (s, 3H) ppm; 13C NMR (100 MHz, DMSO-d6) δ 149.6, 149.1, 147.9, 147.6, 141.7, 135.9, 134.4, 132.3, 131.4, 131.0 (2C), 130.4 (2C), 129.8, 128.5, 128.5, 128.3 (2C), 124.3, 113.6, 113.5, 106.6, 38.2, 27.3, 21.7, 14.9 ppm. HRMS (ESI): calculated for C26H20ClN5NaO3S+ [M + Na]+, 540.0868; found 540.0870.
N-(1-(2-chlorophenyl)-5-cyano-3-methyl-4-(pyridin-4-yl)-1,4-dihydropyrano[2,3-c]-pyrazol-6-yl)-4-methylbenzenesulfonamide (4u): Synthesized with 3q according to the general procedure of 4a. Yield: 53%, white power solid, m. p. 79.0–80.0°C. 1H NMR (400 MHz, DMSO-d6) δ 8.69 (dd, J = 4.6, 1.5 Hz, 2H), 7.52–7.42 (m, 6H), 7.37–7.31 (m, 1H), 7.29 (d, J = 8.2 Hz, 2H), 7.11 (dd, J = 7.9, 1.3 Hz, 1H), 5.90 (d, J = 9.2 Hz, 1H), 5.03 (d, J = 9.2 Hz, 1H), 2.39 (s, 3H), 2.04 (s, 3H) ppm; 13C NMR (100 MHz, DMSO-d6) δ 150.5 (2C), 148.0, 147.6, 145.3, 142.0, 134.3, 131.4, 131.0 (2C), 130.4, 130.4, 130.4, 129.7, 128.4, 128.3 (2C), 123.1 (2C), 113.5, 113.5, 106.2, 39.3, 26.9, 21.7, 14.9 ppm. HRMS (ESI): calculated for C26H20ClN5NaO3S+ [M + Na]+, 540.0868; found 540.0867.
N-(1-(2-chlorophenyl)-5-cyano-3-methyl-4-(thiophen-3-yl)-1,4-dihydropyrano[2,3-c]-pyrazol-6-yl)-4-methylbenzenesulfonamide (4v): Synthesized with 3r according to the general procedure of 4a. Yield: 57%, yellow power solid, m. p. 67.4–68.8°C. 1H NMR (400 MHz, DMSO-d6) δ 7.67 (dd, J = 5.0, 2.9 Hz, 1H), 7.59–7.55 (m, 1H), 7.52–7.44 (m, 4H), 7.33 (td, J = 7.7, 1.6 Hz, 1H), 7.29 (d, J = 8.2 Hz, 2H), 7.11–7.05 (m, 2H), 5.79 (d, J = 9.3 Hz, 1H), 4.88 (d, J = 9.4 Hz, 1H), 2.40 (s, 3H), 2.00 (s, 3H) ppm; 13C NMR (100 MHz, DMSO-d6) δ 148.0, 147.6, 141.5, 136.8, 134.4, 131.3, 131.0 (2C), 130.4 (2C), 129.7, 128.4, 128.3 (2C), 128.2, 128.0, 127.8, 123.5, 113.9, 113.6, 107.1, 36.7, 27.9, 21.7, 14.6 ppm. HRMS (ESI): calculated for C25H19ClN4NaO3S2+ [M + Na]+, 545.0479; found 545.0485.
N-(1-(2-chlorophenyl)-5-cyano-4-(3-methoxyphenyl)-3-methyl-1,4-dihydropyrano[2,3-c]-pyrazol-6-yl)-4-methylbenzenesulfonamide (4w): Synthesized with 3s according to the general procedure of 4a. Yield: 68%, white power solid, m. p. 66.4–67.7°C. 1H NMR (400 MHz, CDCl3) δ 7.36–7.27 (m, 7H), 7.15 (d, J = 8.2 Hz, 2H), 7.06 (d, J = 7.8 Hz, 1H), 7.05 (t, J = 1.8 Hz, 1H), 6.91 (dd, J = 8.3, 2.2 Hz, 1H), 4.93 (d, J = 10.0 Hz, 1H), 4.69 (d, J = 10.0 Hz, 1H), 3.82 (s, 3H), 2.42 (s, 3H), 2.13 (s, 3H) ppm; 13C NMR (100 MHz, CDCl3) δ 160.2, 148.8, 146.6, 140.6, 136.9, 134.8, 131.9, 131.0, 130.2, 130.2, 130.1, 130.1 (2C), 129.8, 128.0 (2C), 127.3, 120.3, 114.6, 113.5, 112.3, 112.0, 106.7, 5.4, 42.4, 27.1, 21.8, 14.1 ppm. HRMS (ESI): calculated for C28H23ClN4NaO4S+ [M + Na]+, 569.1021; found 569.1022.
N-(1-(2-chlorophenyl)-5-cyano-4-(3-methoxyphenyl)-3-methyl-1,4-dihydropyrano[2,3-c]-pyrazol-6-yl)benzenesulfonamide (4x). Synthesized with 3s and benzenesulfonyl chloride according to the general procedure of 4a. Yield: 70%, white power solid, m. p. 66.0–67.5°C. 1H NMR (400 MHz, CDCl3) δ 7.63 (t, J = 7.4 Hz, 1H), 7.49–7.44 (m, 2H), 7.41–7.26 (m, 7H), 7.05 (dd, J = 13.9, 4.9 Hz, 2H), 7.07 (d, J = 7.6 Hz, 1H), 7.03 (t, J = 2.0 Hz, 1H), 4.92 (d, J = 10.1 Hz, 1H), 4.70 (d, J = 10.1 Hz, 1H), 2.14 (s, 3H), 1.56 (s, 3H) ppm; 13C NMR (100 MHz, DMSO-d6) δ 159.9, 148.0, 141.4, 137.7, 136.4, 134.4, 133.9, 133.7, 131.6, 130.6, 130.5 (2C), 129.9, 128.6, 128.2 (2C), 128.1, 120.1, 114.2, 113.8, 113.6, 107.4, 65.7, 55.6, 46.4, 27.2, 14.8 ppm. HRMS (ESI): calculated for C27H21ClN4NaO4S+ [M + Na]+, 555.0864; found 555.0869.
N-(1-(2-chlorophenyl)-5-cyano-4-(3-methoxyphenyl)-3-methyl-1,4-dihydropyrano[2,3-c]-pyrazol-6-yl)-4-methoxybenzenesulfonamide (4y): Synthesized with 3s and 4-methoxybenzenesulfonyl chloride according to the general procedure of 4a. Yield: 64%, white power solid, m. p. 67.6–68.2°C. 1H NMR (400 MHz, DMSO-d6) δ 7.53–7.45 (m, 4H), 7.42–7.34 (m, 2H), 7.19–7.16 (m, 1H), 7.07–6.93 (m, 5H), 5.86 (d, J = 10.1 Hz, 1H), 4.86 (d, J = 10.2 Hz, 1H), 3.87 (s, 3H), 3.78 (s, 3H), 2.05 (s, 3H) ppm; 13C NMR (100 MHz, DMSO-d6) δ 165.3, 159.9, 147.9, 141.7, 137.7, 134.5, 131.5, 130.7 (2C), 130.5, 130.4, 129.9, 128.5, 124.3, 120.1, 115.8 (2C), 115.1, 114.2, 113.9, 113.7, 113.6, 107.3, 65.7, 56.6, 55.6, 27.2, 14.8 ppm. HRMS (ESI): calculated for C28H23N4NaO5S+ [M + Na]+, 585.0970; found 585.0973.
N-(1-(2-chlorophenyl)-5-cyano-4-(3-methoxyphenyl)-3-methyl-1,4-dihydropyrano[2,3-c]-pyrazol-6-yl)-2-nitrobenzenesulfonamide (4z): Synthesized with 3s and 2-nitrobenzenesulfonyl chloride according to the general procedure of 4a. Yield: 68%, white power solid. m. p. 72.6–73.7°C. 1H NMR (400 MHz, CDCl3) δ 7.83 (dd, J = 7.9, 1.2 Hz, 1H), 7.79 (td, J = 7.1, 1.4 Hz, 1H), 7.79 (td, J = 7.9, 1.3 Hz, 1H), 7.51 (dd, J = 7.9, 1.0 Hz, 1H), 7.36 (t, J = 8.0 Hz, 1H), 7.31 (d, J = 7.1 Hz, 1H), 7.26–7.17 (m, 3H), 7.07 (d, J = 7.5 Hz, 1H), 6.99 (t, J = 1.9 Hz, 1H), 6.92 (dd, J = 8.2, 2.2 Hz, 1H), 4.82 (d, J = 10.3 Hz, 1H), 4.72 (d, J = 10.3 Hz, 1H), 3.82 (s, 3H), 2.18 (s, 3H) ppm; 13C NMR (100 MHz, DMSO-d6) δ 159.9, 148.1, 147.5, 141.3, 138.2, 137.5, 134.3, 134.1, 131.8, 131.6, 131.0, 130.5, 130.4, 130.3, 129.6, 128.4, 126.4, 126.3, 122.8, 120.0, 114.1, 113.8, 113.6, 107.7, 55.6, 27.3, 14.9 ppm. HRMS (ESI): calculated for C27H20N5NaO6S+ [M + Na]+, 600.0715; found 600.0720.
N-(1-(2-chlorophenyl)-5-cyano-4-(3-methoxyphenyl)-3-methyl-1,4-dihydropyrano[2,3-c]-pyrazol-6-yl)-3-nitrobenzenesulfonamide (4aa): Synthesized with 3s and 3-nitrobenzenesulfonyl chloride according to the general procedure of 4a. Yield: 73%, white power solid, m. p. 78.9–79.2°C. 1H NMR (400 MHz, CDCl3) δ 8.44 (ddd, J = 8.2, 2.1, 0.9 Hz, 1H), 8.14 (t, J = 1.8 Hz, 1H), 7.97 (dt, J = 7.9, 1.0 Hz, 1H), 7.67 (t, J = 8.1 Hz, 1H), 7.38 (t, J = 8.0 Hz, 1H), 7.32 (d, J = 9.0 Hz, 1H), 7.26–7.18 (m, 3H), 7.05 (d, J = 7.7 Hz, 1H), 7.00 (t, J = 2.0 Hz, 1H), 6.93 (dd, J = 8.2, 2.2 Hz, 1H), 4.83 (d, J = 10.1 Hz, 1H), 4.76 (d, J = 10.1 Hz, 1H), 3.83 (s, 3H), 2.16 (s, 3H) ppm; 13C NMR (100 MHz, DMSO-d6) δ 159.9, 148.2, 141.0, 137.5, 135.2, 134.2, 134.1, 132.8, 132.4, 131.6, 130.7, 130.5, 130.4, 130.2, 129.8, 128.7, 123.8, 122.8, 120.5, 120.1, 114.2, 113.8, 113.7, 107.6, 55.6, 27.4, 14.8 ppm. HRMS (ESI): calculated for C27H20N5NaO6S+ [M + Na]+, 600.0715; found 600.0717.
N-(1-(2-chlorophenyl)-5-cyano-4-(3-methoxyphenyl)-3-methyl-1,4-dihydropyrano[2,3-c]-pyrazol-6-yl)-4-nitrobenzenesulfonamide (4ab). Synthesized with 3s and 4-nitrobenzenesulfonyl chloride according to the general procedure of 4a. Yield: 60%, white power solid, m. p. 142.5–143.6°C. 1H NMR (400 MHz, CDCl3) δ 8.16 (dt, J = 8.8, 2.2 Hz, 2H), 7.67 (dt, J = 8.8, 2.2 Hz, 2H), 7.37 (t, J = 8.0 Hz, 1H), 7.32 (dd, J = 7.2, 2.0 Hz, 1H), 7.28–7.24 (m, 3H), 7.04 (d, J = 7.7 Hz, 1H), 6.99 (t, J = 2.0 Hz, 1H), 6.93 (dd, J = 8.2, 2.3 Hz, 1H), 4.82 (d, J = 10.0 Hz, 1H), 4.75 (d, J = 10.0 Hz, 1H), 3.83 (s, 3H), 2.16 (s, 3H) ppm; 13C NMR (100 MHz, DMSO-d6) δ 159.9, 151.6, 148.2, 138.8, 137.4, 134.3, 131.7, 130.6, 130.5, 130.4, 130.0 (2C), 129.9, 128.6, 127.4, 125.5 (2C), 123.8, 120.1, 114.3, 113.8, 113.7, 113.6, 107.6, 55.6, 27.4, 14.9 ppm. HRMS (ESI): calculated for C27H21N5O6S+ [M + H]+, 578.0896; found 578.0885.
N-(1-(4-chlorophenyl)-5-cyano-3-methyl-4-phenyl-1,4-dihydropyrano[2,3-c]-pyrazol-6-yl)-4-methylbenzenesulfonamide (4ac): Synthesized with 3t according to the general procedure of 4a. Compound (4ac) was synthesized from compound (3t) and 4-methylbenzenesulfonyl chloride, in a manner similar to (4a). Yield: 75%, white power solid. m. p. 181.4–181.7°C. 1H NMR (400 MHz, CDCl3) δ 7.49–7.36 (m, 7H), 7.19–7.07 (m, 6H), 4.95 (d, J = 9.6 Hz, 1H), 4.82 (d, J = 9.6 Hz, 1H), 2.43 (s, 3H), 2.15 (s, 3H) ppm; 13C NMR (100 MHz, CDCl3) δ 148.5, 147.2, 140.1, 135.6, 135.2, 133.4, 130.7, 129.9 (2C), 129.2 (2C), 128.9 (2C), 128.7, 128.4 (2C), 127.8 (2C), 124.7 (2C), 112.2, 112.2, 108.1, 42.0, 27.2, 21.8, 14.3 ppm. HRMS (ESI): calculated for C27H21ClN4NaO3S+ [M + Na]+, 539.0915; found 539.0914.
N-(1-(2-chlorophenyl)-5-cyano-3-methyl-4-phenyl-1,4-dihydropyrano[2,3-c]-pyrazol-6-yl)-4-methylbenzenesulfonamide (4ad). Synthesized with 3u according to the general procedure of 4a. Yield: 60%, white power solid, m. p. 77.4–78.0°C. 1H NMR (400 MHz, CDCl3) δ 7.50–7.41 (m, 4H), 7.41–7.33 (m, 3H), 7.32–7.29 (m, 2H), 7.16 (d, J = 8.1 Hz, 2H), 4.93 (d, J = 9.7 Hz, 1H), 4.74 (d, J = 9.6 Hz, 1H), 2.43 (s, 3H), 2.10 (s, 3H) ppm; 13C NMR (100 MHz, CDCl3) δ 148.8, 146.6, 140.7, 135.4, 134.4, 131.8, 131.0, 130.2, 130.1, 130.1 (2C), 129.7, 129.2 (2C), 128.6, 128.1 (2C), 127.9 (2C), 127.3, 112.3, 112.2, 106.9, 42.2, 27.1, 21.8, 14.2 ppm. HRMS (ESI): calculated for C27H21ClN4NaO3S+ [M + Na]+, 539.0915; found 539.0916.
N-(5-cyano-3-methyl-4-phenyl-1-(p-tolyl)-1,4-dihydro-pyrano[2,3-c]-pyrazol-6-yl)-4-methylbenzenesulfonamide (4ae). Synthesized with 3v according to the general procedure of 4a. Yield: 70%, white power solid. m. p. 142.5–143.0°C. 1H NMR (400 MHz, CDCl3) δ 7.50–7.42 (m, 4H), 7.40–7.33 (m, 3H), 7.07–6.97 (m, 6H), 4.97 (d, J = 9.6 Hz, 1H), 4.82 (d, J = 9.6 Hz, 1H), 2.39 (s, 3H), 2.34 (s, 3H), 2.14 (s, 3H) ppm; 13C NMR (100 MHz, CDCl3) δ 147.9, 147.6, 141.7, 136.3, 134.5, 131.5, 131.1 (2C), 130.7, 130.6, 130.5, 129.9, 129.4 (2C), 128.6, 128.5, 128.3 (2C), 128.0 (2C), 113.9, 113.8, 107.5, 40.6, 27.3, 21.8, 21.7, 14.9 ppm. HRMS (ESI): calculated for C28H24N4NaO3S+ [M + Na]+, 519.1461; found 519.1461.
N-(5-cyano-1-(4-methoxyphenyl)-3-methyl-4-phenyl-1,4-dihydropyrano[2,3-c]-pyrazol-6-yl)-4-methylbenzenesulfonamide (4af): Synthesized with 3w according to the general procedure of 4a. Yield: 70%, white power solid, m. p. 69.0–70.4°C. 1H NMR (400 MHz, CDCl3) δ 7.50–7.42 (m, 4H), 7.41–7.35 (m, 3H), 7.11–7.03 (m, 4H), 6.70 (dt, J = 8.9,3.4 Hz, 2H), 4.96 (d, J = 9.7 Hz, 1H), 4.81 (d, J = 9.7 Hz, 1H), 3.81 (s, 3H), 2.40 (s, 3H), 2.14 (s, 3H) ppm; 13C NMR (100 MHz, CDCl3) δ 147.7, 147.0, 140.5, 137.8, 136.5, 134.7, 130.7 (2C), 130.3, 129.9 (2C), 129.5 (2C), 128.6, 128.6 (2C), 127.9 (2C), 123.3 (2C), 114.0, 113.9, 108.1, 40.5, 27.4, 21.8, 21.1, 14.9 ppm. HRMS (ESI): calculated for C28H24N4NaO4S+ [M + Na]+, 535.1410; found 535.1412.
N-(5-cyano-1-(4-fluorophenyl)-3-methyl-4-phenyl-1,4-dihydropyrano[2,3-c]-pyrazol-6-yl)-4-methylbenzenesulfonamide (4ag): Synthesized with 3x according to the general procedure of 4a. Yield: 68%, white power solid, m. p. 138.6–139.3°C. 1H NMR (400 MHz, CDCl3) δ 7.50–7.37 (m, 7H), 7.17–7.10 (m, 4H), 6.93–6.86 (m, 2H), 4.95 (d, J = 9.6 Hz, 1H), 4.82 (d, J = 9.6 Hz, 1H), 2.41 (s, 3H), 2.14 (s, 3H) ppm; 13C NMR (100 MHz, CDCl3) δ 161.7 (1C), 148.2, 147.1, 134.0, 135.3, 133.3 (1C), 130.6, 129.9 (2C), 129.2 (2C), 128.7, 128.4 (2C), 127.8 (2C), 125.5 (2C), 115.7 (2C), 112.3, 112.2, 107.7, 42.0, 27.2, 21.7, 14.3 ppm. HRMS (ESI): calculated for C27H21FN4NaO3S+ [M + Na]+, 523.1211; found 523.1210.
Data Availability Statement
The original contributions presented in the study are included in the article/Supplementary Material; further inquiries can be directed to the corresponding authors.
Ethics Statement
The animal study was reviewed and approved by the Animal Ethics Committee of West China Hospital of Sichuan University.
Author Contributions
All synthetic research and data analysis were carried out by YW and MH. Biological assays were carried out by YW, MH, XL, and JC. The experiment design and manuscript preparation were performed by YW, QJ, CP, GH, and WH. All experimental research was carried out in the laboratory of GH and WH. All authors have approved the submitted manuscript.
Funding
This research was supported by grants from the National Natural Science Foundation (Nos. 21772131, 81773890, and 82073997), the China Postdoctoral Science Foundation, the Fundamental Research Funds of Science & Technology Department of Sichuan Province (Grant Nos. 2019YFSY0004, 2017JY0226, and 2020YFH0036), and Project First-Class Disciplines Development supported by Chengdu University of Traditional Chinese Medicine (Grant No. CZYJC1902).
Conflict of Interest
The authors declare that the research was conducted in the absence of any commercial or financial relationships that could be construed as a potential conflict of interest.
Publisher’s Note
All claims expressed in this article are solely those of the authors and do not necessarily represent those of their affiliated organizations, or those of the publisher, the editors, and the reviewers. Any product that may be evaluated in this article, or claim that may be made by its manufacturer, is not guaranteed or endorsed by the publisher.
Supplementary Material
The Supplementary Material for this article can be found online at: https://www.frontiersin.org/articles/10.3389/fchem.2021.700956/full#supplementary-material
References
Alvarado, Y., and Giles, F. J. (2007). Ras as a Therapeutic Target in Hematologic Malignancies. Expert Opin. Emerging Drugs 12 (2), 271–284. PubMed PMID: WOS:000247826100008. doi:10.1517/14728214.12.2.271
Bum-Erdene, K., Liu, D., Gonzalez-Gutierrez, G., Ghozayel, M. K., Xu, D., and Meroueh, S. O. (2020). Small-molecule Covalent Bond Formation at Tyrosine Creates a Binding Site and Inhibits Activation of Ral GTPases. Proc. Natl. Acad. Sci. USA 117 (13), 7131–7139. PubMed PMID: WOS:000523188100031. doi:10.1073/pnas.1913654117
Chen, F.-Y., Li, X., Zhu, H.-P., and Huang, W. (2020). Regulation of the Ras-Related Signaling Pathway by Small Molecules Containing an Indole Core Scaffold: A Potential Antitumor Therapy. Front. Pharmacol. 11, 280. Epub 2020/04/02PubMed PMID: 32231571; PubMed Central PMCID: PMCPMC7082308. doi:10.3389/fphar.2020.00280
Ezzeldin, M., Borrego-Diaz, E., Taha, M., Esfandyari, T., Wise, A. L., Peng, W., et al. (2014). RalA Signaling Pathway as a Therapeutic Target in Hepatocellular Carcinoma (HCC). Mol. Oncol. 8 (5), 1043–1053. PubMed PMID: WOS:000339774900014. doi:10.1016/j.molonc.2014.03.020
Gray, J. L., Delft, F., and Brennan, P. E. (2020). Targeting the Small GTPase Superfamily through Their Regulatory Proteins. Angew. Chem. Int. Ed. 59 (16), 6342–6366. PubMed PMID: WOS:000523764400004. doi:10.1002/anie.201900585
Guichard, C., Amaddeo, G., Imbeaud, S., Ladeiro, Y., Pelletier, L., Maad, I. B., et al. (2012). Integrated Analysis of Somatic Mutations and Focal Copy-Number Changes Identifies Key Genes and Pathways in Hepatocellular Carcinoma. Nat. Genet. 44 (6), 694–698. PubMed PMID: WOS:000304551100018. doi:10.1038/ng.2256
Hamada, M., Miki, T., Iwai, S., Shimizu, H., and Yura, Y. (2011). Involvement of RhoA and RalB in Geranylgeranyltransferase I Inhibitor-Mediated Inhibition of Proliferation and Migration of Human Oral Squamous Cell Carcinoma Cells. Cancer Chemother. Pharmacol. 68 (3), 559–569. PubMed PMID: WOS:000294345400002. doi:10.1007/s00280-010-1520-9
Hao, D., Wen, X., Liu, L., Wang, L., Zhou, X., Li, Y., et al. (2019). Sanshool Improves UVB-Induced Skin Photodamage by Targeting JAK2/STAT3-dependent Autophagy. Cell Death Dis 10, 10. PubMed PMID: WOS:000456089100008. doi:10.1038/s41419-018-1261-y
He, X. H., Ji, Y. L., Peng, C., and Han, B. (2019). Organocatalytic Asymmetric Synthesis of Cyclic Compounds Bearing a Trifluoromethylated Stereogenic Center: Recent Developments. Adv. Synth. Catal. 361 (9), 1923–1957. doi:10.1002/adsc.201801647
Hobbs, G. A., Der, C. J., and Rossman, K. L. (2016). RAS Isoforms and Mutations in Cancer at a Glance. J. Cel Sci. 129 (7), 1287–1292. PubMed PMID: WOS:000374949100001. doi:10.1242/jcs.182873
Jacobson, K. A., Merighi, S., Varani, K., Borea, P. A., Baraldi, S., Aghazadeh Tabrizi, M., et al. (2018). A3Adenosine Receptors as Modulators of Inflammation: From Medicinal Chemistry to Therapy. Med. Res. Rev. 38 (4), 1031–1072. Epub 2017/07/07PubMed PMID: 28682469; PubMed Central PMCID: PMCPMC5756520. doi:10.1002/med.21456
Jiang, Y., Sverdlov, M. S., Toth, P. T., Huang, L. S., Du, G., Liu, Y., et al. (2016). Phosphatidic Acid Produced by RalA-Activated PLD2 Stimulates Caveolae-Mediated Endocytosis and Trafficking in Endothelial Cells. J. Biol. Chem. 291 (39), 20729–20738. PubMed PMID: WOS:000384574800034. doi:10.1074/jbc.M116.752485
Klose, M., Duvall, L. B., Li, W., Liang, X., Ren, C., Steinbach, J. H., et al. (2016). Functional PDF Signaling in the Drosophila Circadian Neural Circuit Is Gated by Ral A-dependent Modulation. Neuron 90 (4), 781–794. PubMed PMID: WOS:000376255600012. doi:10.1016/j.neuron.2016.04.002
Lim, S. M., Westover, K. D., Ficarro, S. B., Harrison, R. A., Choi, H. G., Pacold, M. E., et al. (2014). Therapeutic Targeting of Oncogenic K-Ras by a Covalent Catalytic Site Inhibitor. Angew. Chem. Int. Ed. 53 (1), 199–204. PubMed PMID: WOS:000328714900026. doi:10.1002/anie.201307387
Lindsay, C. R., and Blackhall, F. H. (2019). Direct Ras G12C Inhibitors: Crossing the rubicon. Br. J. Cancer 121 (3), 197–198. Epub 2019/06/27PubMed PMID: 31239544; PubMed Central PMCID: PMC6738074. doi:10.1038/s41416-019-0499-1
Lito, P., Solomon, M., Li, L.-S., Hansen, R., and Rosen, N. (2016). Allele-specific Inhibitors Inactivate Mutant KRAS G12C by a Trapping Mechanism. Science 351 (6273), 604–608. PubMed PMID: WOS:000369291600041. doi:10.1126/science.aad6204
Ostrem, J. M., Peters, U., Sos, M. L., Wells, J. A., and Shokat, K. M. (2013). K-ras (K-Ras(G12C) Inhibitors Allosterically Control GTP Affinity and Effector Interactions. Nature 503 (7477), 548–551. PubMed PMID: WOS:000327464200048. doi:10.1038/nature12796
Paludetto, M. N., Puisset, F., Chatelut, E., and Arellano, C. (2019). Identifying the Reactive Metabolites of Tyrosine Kinase Inhibitors in a Comprehensive Approach: Implications for Drug‐drug Interactions and Hepatotoxicity. Med. Res. Rev. 39 (6), 2105–2152. Epub 2019/05/22PubMed PMID: 31111528. doi:10.1002/med.21577
Pan, Z., Chen, Y., Liu, J., Jiang, Q., Yang, S., Guo, L., et al. (2018). Design, Synthesis, and Biological Evaluation of polo-like Kinase 1/eukaryotic Elongation Factor 2 Kinase (PLK1/EEF2K) Dual Inhibitors for Regulating Breast Cancer Cells Apoptosis and Autophagy. Eur. J. Med. Chem. 144, 517–528. PubMed PMID: WOS:000425198100038. doi:10.1016/j.ejmech.2017.12.046
Pan, Z., Li, X., Wang, Y., Jiang, Q., Jiang, L., Zhang, M., et al. (2020). Discovery of Thieno[2,3-D]pyrimidine-Based Hydroxamic Acid Derivatives as Bromodomain-Containing Protein 4/Histone Deacetylase Dual Inhibitors Induce Autophagic Cell Death in Colorectal Carcinoma Cells. J. Med. Chem. 63 (7), 3678–3700. PubMed PMID: WOS:000526405300018. doi:10.1021/acs.jmedchem.9b02178
Pang, H., Wang, N., Chai, J., Wang, X., Zhang, Y., Bi, Z., et al. (2020). Discovery of Novel TNNI3K Inhibitor Suppresses Pyroptosis and Apoptosis in Murine Myocardial Infarction Injury. Eur. J. Med. Chem. 197, 112314. PubMed PMID: WOS:000533524000006. doi:10.1016/j.ejmech.2020.112314
Peng, F., Zhang, N., Wang, C., Wang, X., Huang, W., Peng, C., et al. (2020). Aconitine Induces Cardiomyocyte Damage by Mitigating BNIP3‐dependent Mitophagy and the TNFα‐NLRP3 Signalling axis. Cell Prolif 53 (1), 53. PubMed PMID: WOS:000492669400001. doi:10.1111/cpr.12701
Saha, S., and Giri, T. K. (2019). Breaking the Barrier of Cancer through Papaya Extract and Their Formulation. Acamc 19 (13), 1577–1587. PubMed PMID: WOS:000502339400002. doi:10.2174/1871520619666190722160955
Siegel, R. L., Miller, K. D., and Jemal, A. (2019). Cancer Statistics, 2019. CA A. Cancer J. Clin. 69 (1), 7–34. Epub 2019/01/09PubMed PMID: 30620402. doi:10.3322/caac.21551
Suzuki, S., Yonesaka, K., Teramura, T., Takehara, T., Kato, R., Sakai, H., et al. (2021). KRAS Inhibitor-Resistance in MET-Amplified KRASG12C Non-small Cell Lung Cancer Induced by RAS- and Non-RAS-mediated Cell Signaling Mechanisms. Clin. Cancer Res. 7, 856. Epub 2021/08/09PubMed PMID: 34365406. doi:10.1158/1078-0432.CCR-21-0856
Tracy, K., Velentzas, P. D., and Baehrecke, E. H. (2016). Ral GTP Ase and the Exocyst Regulate Autophagy in a Tissue‐specific Manner. EMBO Rep. 17 (1), 110–121. PubMed PMID: WOS:000369962300007. doi:10.15252/embr.201541283
Walsh, T. G., Wersäll, A., and Poole, A. W. (2019). Characterisation of the Ral GTPase Inhibitor RBC8 in Human and Mouse Platelets. Cell Signal. 59, 34–40. PubMed PMID: WOS:000468251000004. doi:10.1016/j.cellsig.2019.03.015
Wang, X., Wu, F., Li, G., Zhang, N., Song, X., Zheng, Y., et al. (2018). Lipid-modified Cell-Penetrating Peptide-Based Self-Assembly Micelles for Co-delivery of Narciclasine and siULK1 in Hepatocellular Carcinoma Therapy. Acta Biomater. 74, 414–429. PubMed PMID: WOS:000437998200032. doi:10.1016/j.actbio.2018.05.030
Wang, Y., Wen, X., Hao, D., Zhou, M., Li, X., He, G., et al. (2019). Insights into Autophagy Machinery in Cells Related to Skin Diseases and Strategies for Therapeutic Modulation. Biomed. Pharmacother. 113, 108775. PubMed PMID: WOS:000462864800052. doi:10.1016/j.biopha.2019.108775
Xiong, Y.-j., Song, Y.-z., Zhu, Y., Zuo, W.-q., Zhao, Y.-f., Shen, X., et al. (2020). Neuroprotective Effects of Olanzapine against Rotenone-Induced Toxicity in PC12 Cells. Acta Pharmacol. Sin 41 (4), 508–515. PubMed PMID: WOS:000517740600004. doi:10.1038/s41401-020-0378-6
Xue, B., Lei, M., Shi, K., Wang, M., Hao, Y., Xiao, Y., et al. (2019). Intratumoral Injection of Norcantharidin-Loaded Poly(D,L-lactide)-b-Poly(ethylene glycol)-b-Poly(D,L-lactide) Thermosensitive Hydrogel for the Treatment of Primary Hepatocellular Carcinoma. J. Biomed. Nanotechnol 15 (10), 2025–2044. Epub 2019/08/30PubMed PMID: 31462369. doi:10.1166/jbn.2019.2822
Yan, C., Theodorescu, D., Miller, B., Kumar, A., Kumar, V., Ross, D., et al. (2016). Synthesis of Novel Ral Inhibitors: An In Vitro and In Vivo Study. Bioorg. Med. Chem. Lett. 26 (23), 5815–5818. PubMed PMID: WOS:000389519100038. doi:10.1016/j.bmcl.2016.10.021
Yan, C., and Theodorescu, D. (2018). RAL GTPases: Biology and Potential as Therapeutic Targets in Cancer. Pharmacol. Rev. 70 (1), 1–11. PubMed PMID: WOS:000419220700001. doi:10.1124/pr.117.014415
Yang, M.-C., Peng, C., Huang, H., Yang, L., He, X.-H., Huang, W., et al. (2017). Organocatalytic Asymmetric Synthesis of Spiro-oxindole Piperidine Derivatives that Reduce Cancer Cell Proliferation by Inhibiting MDM2-P53 Interaction. Org. Lett. 19 (24), 6752–6755. PubMed PMID: WOS:000418392400074. doi:10.1021/acs.orglett.7b03516
Yu, M., Zeng, M., Pan, Z., Wu, F., Guo, L., and He, G. (2020). Discovery of Novel Akt1 Inhibitor Induces Autophagy Associated Death in Hepatocellular Carcinoma Cells. Eur. J. Med. Chem. 189, 112076. Epub 2020/02/03PubMed PMID: 32007668. doi:10.1016/j.ejmech.2020.112076
Zhang, N., Huang, Y., Wu, F., Zhao, Y., Li, X., Shen, P., et al. (2016). Codelivery of a miR-124 Mimic and Obatoclax by Cholesterol-Penetratin Micelles Simultaneously Induces Apoptosis and Inhibits Autophagic Flux in Breast Cancer In Vitro and In Vivo. Mol. Pharmaceutics 13 (7), 2466–2483. PubMed PMID: WOS:000379455800032. doi:10.1021/acs.molpharmaceut.6b00211
Zhang, N., Peng, F., Wang, Y., Yang, L., Wu, F., Wang, X., et al. (2020). Shikonin Induces Colorectal Carcinoma Cells Apoptosis and Autophagy by Targeting Galectin-1/JNK Signaling axis. Int. J. Biol. Sci. 16 (1), 147–161. PubMed PMID: WOS:000499624000012. doi:10.7150/ijbs.36955
Zhang, N., Zhong, Z., Wang, Y., Yang, L., Wu, F., Peng, C., et al. (2019). Competing Endogenous Network Analysis Identifies lncRNA Meg3 Activates Inflammatory Damage in UVB Induced Murine Skin Lesion by Sponging miR-93-5p/epiregulin axis. Aging 11 (22), 10664–10683. PubMed PMID: WOS:000499683800046. doi:10.18632/aging.102483
Zhang, Y., Wang, C., Huang, W., Haruehanroengra, P., Peng, C., Sheng, J., et al. (2018). Application of Organocatalysis in Bioorganometallic Chemistry: Asymmetric Synthesis of Multifunctionalized Spirocyclic Pyrazolone-Ferrocene Hybrids as Novel RalA Inhibitors. Org. Chem. Front. 5 (14), 2229–2233. PubMed PMID: WOS:000438245300014. doi:10.1039/c8qo00422f
Zhao, Q., Peng, C., Huang, H., Liu, S.-J., Zhong, Y.-J., Huang, W., et al. (2018). Asymmetric Synthesis of Tetrahydroisoquinoline-Fused Spirooxindoles as Ras-GTP Inhibitors that Inhibit colon Adenocarcinoma Cell Proliferation and Invasion. Chem. Commun. 54 (60), 8359–8362. PubMed PMID: WOS:000439702200014. doi:10.1039/c8cc04732d
Zheng, M., Zhang, X., Guo, S., Zhang, X., Min, C., Cheon, S. H., et al. (2016). Agonist-induced Changes in RalA Activities Allows the Prediction of the Endocytosis of G Protein-Coupled Receptors. Biochim. Biophys. Acta (Bba) - Mol. Cel Res. 1863 (1), 77–90. PubMed PMID: WOS:000366068400009. doi:10.1016/j.bbamcr.2015.10.007
Zhongqi, F., Xiaodong, S., Yuguo, C., and Guoyue, L. (2019). Can Combined Therapy Benefit Immune Checkpoint Blockade Response in Hepatocellular Carcinoma? Acamc 19 (2), 222–228. PubMed PMID: WOS:000469924800011. doi:10.2174/1871520618666181114112431
Zhou, F., Shang, W., Yu, X., and Tian, J. (2018). Glypican-3: A Promising Biomarker for Hepatocellular Carcinoma Diagnosis and Treatment. Med. Res. Rev. 38 (2), 741–767. Epub 2017/06/18PubMed PMID: 28621802. doi:10.1002/med.21455
Zhou, R., Wu, Q., Guo, M., Huang, W., He, X., Yang, L., et al. (2015). Organocatalytic cascade Reaction for the Asymmetric Synthesis of Novel Chroman-Fused Spirooxindoles that Potently Inhibit Cancer Cell Proliferation. Chem. Commun. 51 (66), 13113–13116. PubMed PMID: WOS:000359151100013. doi:10.1039/c5cc04968g
Keywords: RalA inhibitors, pyrano[2;3-c]-pyrazole, hepatocellular carcinoma, synthesis, autophagy
Citation: Wang Y, He M, Li X, Chai J, Jiang Q, Peng C, He G and Huang W (2021) Design, Synthesis, and Biological Evaluation of Pyrano[2,3-c]-pyrazole–Based RalA Inhibitors Against Hepatocellular Carcinoma. Front. Chem. 9:700956. doi: 10.3389/fchem.2021.700956
Received: 27 April 2021; Accepted: 29 September 2021;
Published: 15 November 2021.
Edited by:
Laurent G. Désaubry, INSERM U1260 Nanomedicine régénératrice (RNM), FranceCopyright © 2021 Wang, He, Li, Chai, Jiang, Peng, He and Huang. This is an open-access article distributed under the terms of the Creative Commons Attribution License (CC BY). The use, distribution or reproduction in other forums is permitted, provided the original author(s) and the copyright owner(s) are credited and that the original publication in this journal is cited, in accordance with accepted academic practice. No use, distribution or reproduction is permitted which does not comply with these terms.
*Correspondence: Qinglin Jiang, jql_cmc@163.com; Gu He, hegu@scu.edu.cn; Wei Huang, huangwei@cdutcm.edu.cn
†These authors have contributed equally to this work